Implementing team-based learning in a large environmental chemistry course and its impact on student learning and perceptions
Received
25th June 2023
, Accepted 2nd October 2023
First published on 4th October 2023
Abstract
Team-based learning (TBL) is an instructional strategy where students participate in a set of activities including, applying course concepts to real-life case studies in instructor-selected teams. Here, we describe how TBL has been incorporated into a 3rd year, large, environmental chemistry course and investigate the benefits of using this strategy. A combination of pre/post survey and coursework data were analyzed to understand: (1) What were student perceptions of TBL? (2) How did using TBL to deliver content influence student learning, measured by exam performance? (3) How did students’ team skills evolve? Post-survey results indicate that students perceived TBL as enhancing their interest in course content, creating real-world connections, and most helpful for achieving practical critical thinking skills. Student performance on TBL-related final exam items was significantly better (Mean = 73%, SD = 21%) than non TBL-related final exam items, (Mean = 65%, SD = 21%), despite the level of complexity being similar between the two categories. The pre/post survey results indicate that, as compared to the start of term, students reported being significantly more comfortable expressing opinions in group meetings (t(78) = 4.25, p < 0.001, Cohen's d = 0.48), and leading group discussions (t(78) = 3.11, p = 0.003, Cohen's d = 0.35), by the end of the term. The one-minute reflections (completed following the first and fifth TBL activities) indicated that there was a 14% increase (77% vs. 91%) in the number of students reporting on collective team decision making. This study demonstrates the wide-ranging positive impacts of TBL to student learning in a large Environmental Chemistry course all while enhancing active learning and applying chemistry concepts to relevant and real-life case studies.
Introduction
Societies face collective decisions which require scientific knowledge and citizens are encouraged to acquire knowledge about science so they are able to participate in these decisions responsibly (European Commission, 2015). More recently, Pew Research found that the majority of people in all 20 countries investigated agree that being a world leader in scientific achievement is at least somewhat important (Funk et al., 2020). At the same time, chemistry courses are perceived as unpopular and difficult (Osborne et al., 2003; Guerris et al., 2020), partially because chemistry is perceived to lack relevance to the real world (Stuckey and Eilks, 2014). When a student enrolls in a chemistry course, there is an opportunity to enhance perceptions of chemistry and to inspire interest in learning chemistry, not only with the individual students but with each student's broader community. Classroom practices can play a large role in impacting student perceptions and motivations. For example, classrooms that use more active learning strategies, such as peer instruction, group work, and in-class problem solving (Crouch et al., 2007; Deslauriers et al., 2011; Agwu and Nmadu, 2023; Brady and Voronova, 2023), are more likely to create positive perceptions (Osborne et al., 2003; Wieman and Perkins, 2005; Watkins and Mazur, 2013; Donnelly and Hernández, 2018; Kressler and Kressler, 2020). Further, teaching chemistry topics through the lens of relevant contexts and/or applications can positively influence student motivation in learning Chemistry (Yalçinkaya et al., 2012; Broman and Parchmann, 2014; Stuckey and Eilks, 2014; Mahaffy et al., 2017). Context-based learning has also been shown to enhance higher order thinking (Broman and Parchmann, 2014) and interconnected content knowledge (Mahaffy et al., 2017). One classroom practice that could be used to enhance active learning via use of relevant and real-life applications is team-based learning (TBL). In TBL, students participate in a set of learning activities, such as analyzing case studies that apply course content to real-life scenarios, in instructor-selected teams (Michaelsen et al., 2004; Michaelsen and Sweet, 2008). The goal behind this research project was to evaluate the impact of TBL on student learning and perceptions of chemistry in the context of a large Environmental Chemistry course where TBL was implemented to connect classroom concepts with real-life applications.
While there are numerous studies that have shown the benefits of various active learning techniques on student performance in undergraduate science courses (Freeman et al., 2014) and in chemistry courses (Rahman and Lewis, 2020), documentation specifically of TBL in undergraduate chemistry courses are limited (Comeford, 2016; Hagen, 2016; Lekhi and Nashon, 2016). This may be due to Chemistry being viewed as factual, with either right or wrong answers so designing a case study that merits discussion is challenging (Capel et al., 2021). TBL has been widely used in the fields of Medicine, Health Sciences, and Management and documented benefits of TBL include: improvements in student perceptions and engagement (Davidson, 2011), academic performance (Koles et al., 2010; Rania et al., 2015), and teamwork skills (Michaelsen, 2002). Using course evaluation and survey data, Davidson (2011) describes significantly higher ratings of medical student satisfaction with their learning experiences in a course that was redesigned from using a didactic approach to TBL. In regard to academic performance, Koles et al. (2010) found that medical students performed better on exam questions which tested material they learned using TBL compared to other teaching strategies and Rania et al. (2015) found that psychology students performed better on exams of courses that implemented TBL compared to exams of courses that did not. TBL has been found to increase undergraduate medical students’ ‘‘understanding of the principles of group work’’ (Davidson, 2011) and encourages involvement of team members and collaboration since, by design, all team members share the same grade for the group activities (Morrison et al., 2010). The current study aims to describe how TBL was implemented in a large 3rd year Environmental Chemistry course and to evaluate TBL based on the benefits observed in other disciplines. The research questions include:
1. What were student perceptions of TBL?
2. How did using TBL to deliver content influence student learning, measured by exam performance?
3. How did students’ teamwork skills evolve over the course?
Team-based learning instructional strategy
Following Michaelsen's design, students in a TBL class are strategically assigned into permanent teams of 4–6 (Michaelsen et al., 2004). Before coming to class, students are expected to complete assigned readings. Once in class, students complete an individual Readiness Assurance Test (iRAT) which consists of a short multiple-choice test on the key ideas from the pre-readings. Then, the students take the same test again as a team, referred to as the Group Readiness Assurance Test (gRAT). Group members come to a consensus on the answers and receive immediate feedback on their response choices, working together until all of the answers are correct. Students have the opportunity to write evidence-based appeals for their answer to questions marked as incorrect. The instructor may choose to give a summary lecture to clarify any misconceptions arising from the RAT. The pre-reading, iRAT, gRAT, appeals and summary lecture are all referred to as the Readiness Assurance Process (RAP). Once the RAP is completed, the students work in the same teams during class to solve real-life application problems, or case studies, that are based on the material that has been learned. The case studies are designed to have the following characteristics: they are the same for all of the groups, the stories are interesting and complicated enough to stimulate debate and the groups must make a decision about the case study. The latter is referred to as the decision task (e.g., a multiple-choice question). The results of the decision task are simultaneously reported by all groups and then, the instructor facilitates discussion between teams (Michaelsen and Sweet, 2008). Students complete a peer assessment of their team members’ contributions to the activities of the team (Michaelsen et al., 2004).
Team-based learning is similar to other case-based activities, such as Problem-based learning (PBL). Like TBL, PBL is widely used in the medical sciences. Readers may be more familiar to PBL since there are more studies on PBL in chemistry education compared to TBL (Rahman and Lewis, 2020). There are three main differences between TBL and PBL. First, in PBL students are initially provided with the problem before deciding what concepts need to be discovered on a ‘need-to-know’ basis. Second, the problems in PBL tend to be more complex and integrate multiple, and perhaps, interdisciplinary concepts whereas TBL applications tend to be more specific and an extension of the RAP. Finally, PBL usually requires a facilitator or tutor per group to guide the students through the problem. In contrast, TBL relies on the working of the team, and the RAP (Capel et al., 2021).
Team-based learning is similar but distinct to cooperative learning, which is another form of in-class small group learning that has been shown to be effective in chemistry education (Rahman and Lewis, 2020). Cooperative learning applies to a series of independent small group learning activities where the types of tasks assigned to the groups vary (Johnson et al., 1998). In contrast, TBL uses a semester-long series of small group activities with the same design (RAP plus case study and decision task). The implications of this fundamental distinction leads to other practical differences. For example, it is important for instructors in cooperative learning to spend class time teaching and analyzing cooperative skills and to assign roles (e.g., “encourager”, “explainer”, etc.) for students in groups (Johnson et al., 1998). In TBL, these elements are not required and, in the case of assigning roles, is discouraged (Michaelsen et al., 2004). The belief in TBL is that with prompt feedback on the gRAT and the decision task, teams naturally engage in an analysis of what went wrong or right and make the necessary improvements to their group process. Further, the case study should be designed to require discussion among team members. In TBL, consistent expectations of the teams’ function and composition encourages members of the team to, over time, naturally decide what functions of the task each member will manage (pages 12 to 26 of Michaelsen et al., 2004).
The primary author was drawn to implementing TBL in a large Environmental Chemistry course for a few reasons. First, it is apt to apply context-based teaching in Environmental Chemistry since environmental challenges have been increasingly publicized across the globe, and knowledge of the role of chemistry deepens student understanding of these challenges. While the majority of students in the course are not pursing a degree in chemistry, it is important for them to see the relevance of chemistry concepts to function as well-educated citizens. With TBL, students have an opportunity to connect the chemistry learned in the course to real-life environmental topics. Second, TBL facilitates student learning in teams. This is beneficial because teamwork skills has become a key factor in hiring graduates (Fair et al., 2014; Kondo and Fair, 2017). Third, TBL seemed relatively easy to incorporate into a large lecture context where there are no resources for additional tutors, as required by PBL, and it is a structured pedagogy, which helps the students understand the expectations.
Theoretical framework
Team-based learning is based on a constructivist approach stemming from the learning theories of Jean Piaget and Lev Vygotsky (Cakir, 2008; Hrynchak and Batty, 2012). In his theory of intellectual development, Piaget asserts that mental patterns (schemes) are used to guide human behavior or cognition, and interpret new experiences or material in relation to existing schemes (Cakir, 2008). This theory underlies a primary idea of constructivism that learners “construct” their own knowledge on the basis of what they already know. Learning is active, rather than passive, with learners making judgments about when and how to modify their knowledge (Matthews, 1993). When using TBL, passive learning strategies, such as lecturing, are minimized. Students are expected to construct their own understanding of course content from the pre-reading material and are given opportunity to compare their current understanding with their team-mates during the gRAT and case study discussions (Hrynchak and Batty, 2012). This process is consistent with a constructivist view that learning occurs by integrating information obtained by new experiences into existing mental schemes.
Team-based learning is also heavily based on Vygotsky's theories of the Zone of Proximal Development (ZPD) and social constructivism (Taber, 2011). Vygotsky's ZPD describes what the learner is incapable of doing unaided but capable when supported by a more knowledgeable individual. Learning occurs when individuals are exposed to experiences beyond what they already know but within reach of their current understanding. Thus, scaffolding is an important concept related to Vygotsky's ideas (Taber, 2011). Scaffolding is key in TBL because the readiness assurance process is meant to “cover” low-level course material that students can learn on their own facilitated by the pre-reading and discussions of the gRAT with their teammates (Michaelsen and Sweet, 2008). If necessary, there is also the opportunity for the instructor to address difficult concepts during the summary lecture. Students then tackle the case studies that are meant for higher-level learning after the RAP (Michaelsen and Sweet, 2008). The in-class team problem-solving component of TBL case studies applies Vygotsky's Social Constructivism theory (Jensen et al., 2015). Vygotsky viewed learning as a social process and categorized learning into two types: (1) everyday learning and (2) learning that occurs in a formal setting (Cakir, 2008). When a student learns in a formal setting, he/she eventually come to see how his/her everyday experiences fits into the system he/she has been taught and vice versa. Dialogue, with the teacher and peers of a real-life case studies applying course content plays crucial role in learning to bridge the gap between the two categories of learning (Cakir, 2008).
IRB approval
This study followed ethical protocol and institutional guidelines, and the Behavioural Research Ethics Board at the University of British Columbia Vancouver provided ethical approval for the present study (H17-02890). Written, informed consent was obtained from participants when they completed the online surveys.
Implementation of TBL in CHEM 301
The present study took place in a 3rd year Aqueous Environmental Chemistry course (CHEM 301) delivered between January–April 2018 at a large research institution, the University of British Columbia in Vancouver, Canada. CHEM 301 is an elective course, with a prerequisite requirement of first-year Chemistry only. In January–April 2018, CHEM 301 classes occurred twice per week for 90 minutes per class in a traditional lecture-style classroom with fixed seating. At the start of term, all students (n = 108) were given the course schedule which indicated that six of the twenty-four 90 minute class sections would be transformed into “TBL Events”, where students would be required to participate in TBL activities before and during those classes. The pre-readings were excerpts from Manahan's 8th edition of Environmental Chemistry textbook, available through the university's online library reserve, as well as other sources. Students were not required to purchase any textbook for the course. Table 1 outlines the learning objectives for the pre-reading assignments as well as the titles of the case studies for the six TBL events.
Table 1 Case studies and associated pre-reading learning objectives
Case study title |
Learning objectives for pre-readings |
Pre-reading resource |
1. Limnic eruption of lake Nyos: an application of Henry's law |
(a) Define stratification, autotrophic, heterotrophic, productivity, epilimnion, hypolimnion, thermocline, BOD, DO, eutrophic, primary producers. |
Chapter 3.3 to 3.6 (Manahan, 2005) |
(b) Describe photosynthesis and respiration and the role of dissolved oxygen in these processes. |
(c) Identify the sources and sinks of dissolved oxygen in natural waters. |
(d) Define Henry's Law and factors that affect solubility of gases. |
|
2. Ocean acidification: effect of atmospheric CO2 on oceanic buffer system |
(a) Define alkalinity, acidity, and total dissolved inorganic carbon |
Chapter 3.7 and 3.8 (Manahan, 2005) |
(b) Identify the sources of dissolved CO2 in natural waters |
(c) Interpret a distribution diagram for CO2 system. |
(d) Describe the equilibrium system of dissolved CO2 with water |
(e) Define Ka1 and Ka2 for the carbonate system. |
|
3. Mining in bristol bay, alaska: role of complexation in concerns of copper toxicity |
(a) Define: ligand, unidentate (or monodentate) ligand, chelating ligand, complexes and speciation. |
Chapter 3.10 and 3.11 of (Manahan, 2005) and Pages 197–198 of (Mahaffy, Bucat and Tasker, 2014). |
(b) Explain the bonding strength of metal complexes with different ligands. |
(c) Recognize Lewis acids and bases in a complexation reaction |
(d) Draw Lewis structures of common ligands to identify binding sites in a ligand. |
(e) Define a coordination bond and determine the coordination number of a metal complex. |
(f) Recognize the importance of complexation and speciation of metals in natural and waste waters. |
(g) Give examples of common chelating ligands. |
|
4. Acid rock drainage in britannia beach, BC: role of microbial transformation |
(a) Identify the most common form of sulfur in: oxic environments, organic matter and reducing environments and identify the role of bacteria in some of these transformations of sulfur. |
Chapter 6.12 and 6.14 of (Manahan, 2005). |
(b) Explain the redox reactions which occur when metal sulphides are exposed to oxygen (which species is oxidized/reduced and how acid is produced). |
(c) Explain what happens to metals in the aquatic environment when acid is produced. |
(d) Define “yellowboy” deposits |
(e) Discuss what happens when acid mine waters enter oxygenated environments. |
|
5. Arsenic contamination of drinking water in Bangladesh: the importance of speciation in heavy metal pollution |
(a) Describe the sources, transformations and problems associated with heavy metal and metalloid pollution, for: Cd, Pb, Hg, As |
Chapter 19.3 of (Tro, 2010) and instructor-created content. |
(b) Define: volatile, bioaccumulation and biomagnification. |
(c) Describe 3 types of radioactivity (alpha decay, beta decay and gamma radiation) |
(d) Use symbols to represent elements, mass number, atomic number, alpha particle, beta particle and gamma ray. |
(e) When given a nuclear process represented by symbols, categorize the process as alpha decay, beta decay or gamma radiation. |
(f) Compare the energy levels and potential harmfulness of three types of radioactivity (alpha radiation, beta radiation and gamma radiation). |
|
6. Water Crisis in Flint, MI USA: water treatment procedures |
(a) Identify the sources of drinking water and treatment differences between ground water and lakes or rivers. |
Instructor-created content. |
(b) Describe the treatments (methods and function of each method) used in treating drinking water |
(c) Identify the drawbacks of using chlorine and discuss some of the alternatives |
Students were assigned into teams of 4–6. Teams were created to have a mix of academic performance and gender. Each team had at least one student who achieved an “A” standing in first year Chemistry and no team had members who were all the same gender. During a TBL event, students were assigned seats based on their team IDs (Fig. 1). When students arrived to the class, they sat in their teams and were given a team envelope. The envelope contained items for the TBL activity, including a multiple-choice test that assessed their mastery of the pre-reading objectives. First, students would individually complete this test (the iRAT) for approximately 10 minutes. Then, the students completed the same multiple-choice test again in their teams (the gRAT), using IF-AT multiple-choice cards purchased from Epstein Educational Enterprises (https://www.if-at.com/home/). The IF-AT cards consist of rectangles labelled “A”, “B”, “C”, etc. and each rectangle has a covering, which can be scratched away. The correct answer is pre-marked but it is hidden by a cover. Teams reach consensus on the correct answer to a question, scratch the corresponding cover on the IF-AT card, and can immediately see whether their first choice was correct, without giving away the correct answer. Teams who did not choose the correct answer have the opportunity to try again. If a team chooses the correct answer on their first attempt, they receive 4 points, 2 points if the team chooses the correct answer on their second attempt, and 1 point if they choose the correct answer on their third attempt. Teams are instructed to sum up their scores themselves on the gRAT and to submit their IF-AT cards. During the gRAT, the instructor and teaching assistant (TA) walk around the lecture hall to help facilitate discussions in the teams if needed, and to look for questions that are the most difficult. Once the gRAT is completed, teams move on to the application activity. The instructor may provide clarifications on any of the questions from the gRAT, if necessary and this would happen once all the IF-AT cards are submitted. Students would read the case study and complete a worksheet as a team. The first task on the worksheet is to make a decision about the case, in the form of a multiple-choice question. The first question after the decision task in the worksheet is always the same: “Give your decision and provide a rationale based on the case as well as course content. Include information that opposes the other options as well as information that supports your argued position.” Additional questions on the worksheet are intended to be hints to get students to dig deeper into the case study and/or help teams make the correct decision. Table 2 provides the components of the team worksheet used for case study 1. Before the 90 minute lecture is over, teams will simultaneously reveal their choice for the decision task using colored paper labelled “A”, “B, “C”, “D” that can be found in the team envelopes. Once the decision is revealed, the instructor facilitates a discussion in the large class, asking questions such as, “D seems to be the least popular answer. Why did you NOT pick “D”? and “Why did you pick “A”?. Before the end of the 90 minute class, the decision answer is discussed and the best answer is provided. The other questions on the worksheet are answered either in the same lecture, time permitting, or in the next lecture. In this way, discussions of a case study can span over 2 lectures but all marked team activities are completed during the lecture scheduled to be the “TBL event”.
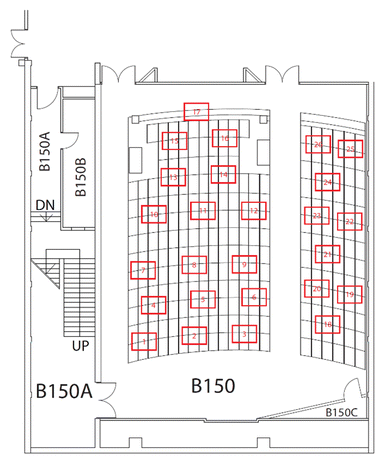 |
| Fig. 1 Floorplan of CHEM 301 Lecture Hall with Assigned Seating for Teams. | |
Table 2 Case study 1 components of the team worksheet
Case study |
Lake Nyos is located in Cameroon, West Africa (Fig. 1). A disaster occurred in the evening of August 21st, 1986 around Lake Nyos where people and animals suddenly collapsed and died. Sadly, 1746 people and thousands of cattle and other livestock were found dead on the morning of August 22. It was heart-breaking, shocking, and mysterious. There was no obvious cause of the disaster such as war or a fire or an earthquake. The water is normally blue in Lake Nyos, but turned red after the event. |
|
Location of Cameroon. Taken from public domain: https://worldpopulationreview.com/countries/cameroon/location |
It was later determined that a limnic eruption had occurred. A fountain of CO2 gas and water shot up suddenly from the lake. The carbon dioxide in the air surrounding Lake Nyos poisoned people and animals. At the bottom of Lake Nyos (∼200 m deep) are volcanic vents that supply CO2 (g) to the bottom water. Other sources of dissolved CO2 (g) include the atmosphere and respiration processes, which produce CO2 (g). |
|
Decision task (multiple choice question) |
Why are CO2 limnic eruptions NOT a problem in British Columbia? |
A. There is little volcanic activity in BC lakes to supply CO2 to the bottom waters. |
B. The population is not as dense in BC as it is in in Cameroon. As a result, less atmospheric CO2 dissolves in British Columbian lakes. |
C. There are larger temperature changes in BC throughout the year. |
D. Lakes in BC are generally not as deep as Lake Nyos and winds are generally stronger in BC, leading to more vertical mixing. |
|
First question (the same for all TBLs) |
1. Give your choice and provide a rationale based on the case as well as course content. Include information that opposes the other options as well as information that supports your argued position. |
|
Additional questions |
• How does pressure compare between surface water and deep water? What effect does this have on concentration of dissolved gas between the surface and deep water? |
• Why did the lake turn red? (Hint: the red was the same red color of rust). |
• Carbon dioxide caused the death of people near Lake Nyos in 1986. Why don’t we worry about being poisoned when we open a bottle of a carbonated drink? |
• Imagine that you are an environmental engineer who has been commissioned to prevent a limnic eruption in lake Nyos from occurring ever again. What ideas do you have on how to do this? |
At the end of TBL Event #1 and Event #5, students were asked to complete a “1 minute Reflection”, which asked students to rank their satisfaction working with their assigned team and provide the opportunity for students to let the instructor know of any concerns (Fig. 2). The 1 minute reflections re-iterated that it was important to reach consensus for team decisions, and allowing a space for students to let the instructor know of any problems in the teams. On the last day of class, students completed a summative peer evaluation of their team members (Fig. 3). Students were told repeatedly in the semester that all members of a team receive the same grade on the group TBL activities UNLESS a member receives poor scores from multiple members of their team. In that situation, the member in question would have their scores on the group activities adjusted (lowered) to reflect their lack of contribution. Fig. 3 is a copy of the form used, with the names of the team members replaced by “Member #X”.
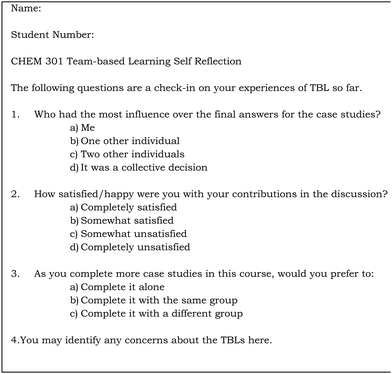 |
| Fig. 2 Copy of 1 minute Reflection Forms Used After TBL 1 and TBL 5. | |
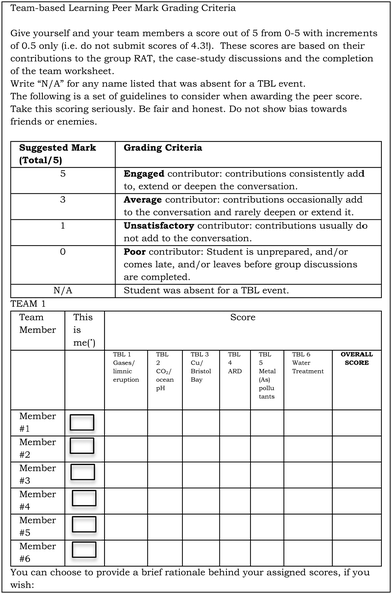 |
| Fig. 3 summative peer evaluation of their team members. | |
Non-TBL classes
Non-TBL classes consisted of lectures with active learning components. While classroom observations were not conducted in this study, instructor reflections based on criteria of existing observational tools, provide general characterizations of the non-TBL classes. One such classroom observation tool, the three-dimensional learning observation protocol (3D LOP) (Bain et al., 2020), uses criteria of which teaching activities were used (administration, lecture, tasks, and clicker questions), as well as, the nature of what was taught. Applying this criteria, a large percentage (at least 67%) of a non-TBL class was devoted to lecturing (i.e. the instructor presents information) while the remaining time consisted of tasks and clicker questions (Crouch et al., 2007). The tasks were mainly limited to open-ended and numerical questions that students either solved individually or through discussions with peers sitting near them. The nature of what was taught in a non-TBL class can be characterized as mainly building knowledge of course concepts and performing calculations. In contrast, the nature of what was taught in a TBL class can be characterized as applying knowledge of course content to real-life case study, making connections between course concepts, constructing explanations, and engaging in consensus building using evidence.
Grading
The components of the TBL were counted towards 18% of students’ final grades, as summarized in Table 3.
Table 3 CHEM 301 assessment in 2018
Assessment |
Weight towards Final Grade |
Midterms (2): |
30% (15% each midterm) |
In class participation |
2% (using iClickers) |
On-line quizzes |
5% (two quizzes) |
iRAT |
12% (6 iRATs) |
Team activities |
6% (gRATs plus team worksheets) |
Final Exam |
45% |
Evaluation of TBL on student learning: methods
To address the research questions, a combination of pre/post survey, administered at the start and end of the semester, and coursework data was analyzed. Table 4 summarizes the alignment between the TBL research questions and the data.
Table 4 Summary of TBL research questions and data collection
Research questions: |
Data collected |
What were students’ perceptions of TBL? |
• Pre/post survey |
How did using TBL to deliver content influence student learning measured by exam performance? |
• Final exam |
How did students’ team skills evolve over the course? |
• Pre/post survey |
|
• In-class 1 minute reflections |
Participant recruitment
All students registered in the CHEM 301 course (n = 108) during the study dates were eligible to be participants in the study. Course work was collected for all those enrolled in CHEM 301 and participation in the pre/post survey was voluntary. Participation in the surveys was advertised by one of the co-authors (Varao-Sousa) in her role as Research Specialist for the project. In the first week of class, students were informed of the survey and an active link was posted via the classroom management system (Canvas). In the final week of class, a second announcement was made for the post-term survey, at which time the survey became active for students to complete.
Pre/post survey
We acquired student survey reports twice throughout the course: (1) a pre-course survey, and (2) an end of term survey. The surveys were all conducted online via the Qualtrics platform. By having students complete a survey at two points during the term we were able to conduct a within-subjects analyses to examine how individual students’ perceptions changed as the course progressed. 108 students were enrolled in the course, and 79 completed both surveys. The surveys were completed for course credit; students were given one bonus mark allocated to their final exam for completing both surveys. Students who did not want to complete the surveys were provided with the option of a research paper summary, for an equivalent one mark bonus (no students chose this option). Student identifiers were removed prior to analyzing the data and each student was given an anonymous unique identifier. Traditionally, both Science and Engineering students enroll in CHEM 301, in the present sample of students, 12 were Chemistry Majors, 54 were Combined Majors, 13 were from the Environmental Science program, 6 were in Engineering and the remaining 20 were in other Faculty of Science programs.
The start of term survey was made available to students in the first week of class, and remained active for 10 days. The survey consisted of 42 questions (Appendix A), and students took an average of 5 minutes to complete the survey. Most of the questions were developed by the authors intended to probe student perceptions of TBL. Majority of the questions required multiple-choice or Likert scale responses. The final survey was made available to students in the final 2 weeks of class, and remained available for 9 days. This survey consisted of the same questions from the start of term survey, with an additional 7 questions developed by the authors specifically related to student engagement working with teams (average completion time was 7 minutes). Questions created by authors are not validated measures. Questions 6 to 10 of the pre/post survey consisted of items from established research assessments to address an additional research question that is not directly assessing TBL: How did student students’ attitudes towards the discipline of Chemistry evolve over the course? This question was assessed via selected categories from the Chemistry Attitudes and Experiences Questionnaire (CAEQ) (Coll et al., 2002) and the Colorado Learning Attitudes about Science Survey (CLASS) (Adams et al., 2006). Categories from these assessments that related to the real-life environmental chemistry problems, and careers discussed in the course were included. The CAEQ categories “attitudes towards chemistry” was included because it consisted of items measuring perceptions of chemistry careers and chemists, as well as interest in chemistry websites and in talking to friends about chemistry. Items from the CAEQ “self-efficacy” included ranking confidence on the item, “Explaining something you learned in this chemistry course to another person”. The CLASS-Chemistry category “Real-world Connection” was included in the survey as it assesses student views on the relevance of chemistry to their lives. Additional items created by the authors were added to the survey which further assessed student experiences in teams. Items included self-reported comfort level in discussing opinions in group settings and leading group discussions as well as questions asking whether students preferred to learn in groups or individually. The post survey also included reflection questions probing student experiences with TBL in the course.
In-class coursework
Two course tasks were analyzed as part of the study. These included in-class 1 minute reflections and the final exam.
1 minute reflections
At the end of TBL Event #1 and Event #5, students were asked to complete a form reflecting on their team experiences during TBL (Fig. 2). Students were asked to rank their satisfaction working with their assigned team and given the opportunity to let the instructor know of any concerns. These forms were collected and kept by the instructor. After the course was completed and the final grades were submitted, the co-researcher (Varao-Sousa) analyzed the forms for changes in student satisfaction working in teams across the term.
Final exam
Long after the course was completed and the final scores were submitted, the instructor and co-researcher (Lekhi), as well as an independent faculty member who has also taught the course (Kristin Orians) served as raters for the final exam. The rating process began with each rater categorizing the questions independently, then the raters met and discussed each question where there was disagreement until they were able to come to a consensus rating for all questions. The exam questions were categorized in two ways. First, if the exam question tested an objective that was mainly learned through TBL (including the pre-readings and case studies), it was categorized as “TBL-related” while questions that tested an objective that was mainly learned in other class activities were categorized as “non-TBL related”. Using the method by Wieman and Holmes (2015), we referred to the objectives from the pre-readings (Table 1), or the team worksheets, including the case study to guide us in this categorization. Between the two raters, there was 96% agreement on categorizing exam questions as TBL-related and non-TBL related and then consensus was reached on the remaining 4%. To evaluate the equivalence of complexity in the sub categories of exam questions, we followed the method outlined in Freeman et al. (2011) to calculate a Weighted Bloom's Index for TBL-related questions and for non-TBL-related questions, which is a weighted sum of the points per question, the weight being the assigned Bloom's level:
where n is the number of parts on the test, P is the points per question, B is Bloom's rank (1–6) for that question. T is the maximum score on test (Freeman et al., 2011).
To help the raters with ‘Blooming’ the questions, we followed the six descriptors and examples as detailed in Dunham et al. (2015): (1) knowledge/remembering, (2) comprehend/understand, (3) apply, (4) analyze, (5) create and (6) synthesize. The higher two levels (“Create” and “Synthesize”) were not tested in the CHEM 301 final exam, which is typical of final exam questions in introductory courses (Dunham et al., 2015). The two raters had 75% agreement on categorizing the question according to Bloom's taxonomy before reaching consensus on all questions. The Weight Bloom's Index was computed to be 40.35 for TBL-related questions, and 43.9 for non-TBL related questions, indicating that the complexity of the questions was similar for TBL-related questions and non-TBL related questions. Table 5 provides examples of final exam questions in each domain. Appendix B contains all questions with Bloom levels from each rater.
Table 5 Examples of final exam questions in each category
|
TBL-related |
Non-TBL related |
Level 1: remembering |
(Refer to Table 1; TBL Event 5; Objective (d) |
Which two species primarily cause acid rain? |
An alpha particle, once it leaves an atom, is the same as: |
(a) NO and CO2 |
(a) A neutron |
(b) SO2 and CO2 |
(b) An electron |
(c) SO2 and O2 |
(c) A hydrogen nucleus |
(d) H2 and CO2 |
(d) A deuterium nucleus |
(e) NO and SO2 |
(e) A helium nucleus |
|
|
Level 2: comprehension |
(Refer to Table 1; TBL Event 5; Objective d) |
Addition of a polyelectrolyte causes the precipitation of colloidal material primarily by: |
Fill in the missing species in the following nuclear equation: |
(a) Decreasing the electric double layer |
234Pa → 234U + — |
(b) Increasing the ionic strength of solution |
(a)  |
(c) Changing the pH to match the zero point charge of the colloid. |
(b)  |
(d) Bridging colloids |
(c)  |
(e) Modifying the size of the colloids so that they exhibit the Tyndall effect. |
d)  |
|
(e)  |
|
|
Level 3: apply |
The Flint water crisis occurred because the Flint river water was corrosive and that resulted in leaching of lead into the drinking water from pipes. Which of the following statements is FALSE? |
Complete the following sentence: In seawater, the hydrolysis product of Al3+ would be the complex but in acid mine waters, Al3+ would be mainly found as its complex |
(a) The Flint river water was more corrosive due to high levels of chloride (Cl−) ions. |
(a) Aqua, hydroxo |
(b) It is important to perform the aeration step of water treatment of the Flint river before adding Cl2 as a disinfectant because the presence of Fe2+ will react with Cl2 to form Cl−. During aeration, Fe2+ is oxidized to Fe3+. |
(b) Aqua, oxo |
(c) The presence of trimethylhalides in the Flint drinking water was due to Cl2 reacting with organic matter. |
(c) Hydroxo, aqua |
(d) Alum was used as a coagulant during water treatment in Flint. |
(d) Hydroxo, oxo |
(e) Both b and c are false. |
(e) Oxo, aqua |
(f) Both b and d are false. |
(f) Oxo, hydroxo |
(g) Both c and d are false. |
|
|
Level 4: analyze |
(Refer to Table 1; TBL Event 3) |
Determine the solubility product constant (Ksp) for silver chloride at 25 °C, using the equations and pε° values provided below. |
Bristol Bay in Alaska was initially protected from mining in 2014 partially due to concerns over copper toxicity to fish and other aquatic organisms. |
Ag+(aq) + e− → Ag(s) |
Copper has been found to be more toxic in soft waters compared to hard waters. Why would copper toxicity increase in soft waters? |
pε° = 13.52 |
|
AgCl(s) + e− →Ag(s) + Cl−(aq) |
|
pε° = 3.76 |
Statistical analysis
Statistical analyses were conducted using RStudio statistical software. The online surveys, the 1 minute reflection questions and performance on the final exam questions were analyzed using within-subject t-tests, comparing changes in individual responses across the term. The critical thinking scores from the application worksheets were analyzed via a within-subjects ANOVA.
Results and discussion
The results and discussion are structured around the research questions (RQ) presented in the introduction. The authors also had an additional research question that is not directly evaluating TBL: How did student students’ attitudes towards the discipline of Chemistry evolve over the course? Survey data was analyzed for changes in student perceptions of chemistry, described in this study as seeing chemistry as important and relevant in daily life. The selected categories from the CAEQ and CLASS in the pre/post survey did not differ significantly across the term although it should be noted that scores indicated that students began (and ended) the course with relatively positive perceptions of chemistry (all ps > 0.05).
RQ 1: What were students’ perceptions of TBL?
Post-survey results suggest that students positively perceived TBL. Seventy percent of students (n = 56) in the post-survey indicated that they would recommend using TBL for Chemistry 301 in the future, with key reasons for supporting this decision being: creating real-world connections; enhancing interest and perceived increases in critical thinking. A follow-up open-ended question in the post-survey, asking students to explain their reasoning for why they would recommend TBL in a future course included a variety of responses such as:
“Connecting what we learned in the lecture to the real world is what I am expecting in a university course.” (Student 1).
“The TBLs were a great way to think more critically about what we learned and to practice these skills in a low stress setting.” (Student 2).
“It allows students to interact with each other and also able to see the different perspectives other students take on certain topics.” (Student 3).
Students indicated in a separate item that their interest in the course improved as a result of the TBL events (Mean = 2.86; 1 = Strongly Agree; 7 = Strongly Disagree). A follow-up to this item in the post-survey asked students to explain their rating. Sample responses from those who's interest increased included statements such as:
“Discussing real world applications of the subjects presented in the course gave a different perspective on the chemistry involved.” (Student 4).
“Got to learn about current real-world problems involving chemistry and the thought process that goes in to them.” (Student 5).
“The TBLs showed me current events, and how chemistry plays a significant role in solving problems and health hazards” (Student 6).
“TBLs were a nice application of the course. They taught about several issues which I had heard about but only knew at a surface level.” (Student 7).
“The TBLs sparked my interest in applying chemistry to problems outside the classroom.” (Student 8).
Students reported that of all the course components (lectures, quizzes, TBL, text readings and discussion forums), TBL was the most helpful for achieving practical critical thinking skills (Fig. 4).
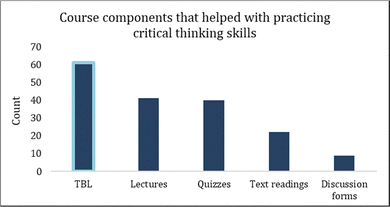 |
| Fig. 4 Post-survey results. | |
RQ 2: How did using TBL to deliver content influence student learning, measured by exam performance?
To assess the influence of TBL on test performance, final exam scores were divided into TBL-related scores and non-TBL related scores (outlined in the Methods section). Since the level of complexity was approximately the same for both categories, we were able to compare average scores between TBL-related and non-TBL related questions. A paired samples t-test indicated that students performed significantly better on questions which tested material from TBL sessions (Mean = 73%, SD = 21%) compared to questions testing content learned through other teaching strategies (Mean = 65%, SD = 21%), t(108) = 6.64, p < 0.001, Cohen's d = 0.63. This finding is consistent with Koles et al. (2010) who determined that that medical students performed better on exam questions which tested material they learned using TBL compared to other teaching strategies.
Research question 3: How did students’ teamwork skills evolve over the course?
Chemistry students’ teamwork skills, including consensus building and encouraging opinions from members, has become more important in recent years as a key factor in hiring graduates (Fair et al., 2014; Kondo and Fair, 2017). Previous research shows that students prefer to form their own groups (Chapman et al., 2006). Despite the fact that the teams are instructor-selected, we saw a positive evolution student comfort level and other team skills in both the survey and the 1 minute reflections. Two pre/post survey questions assessed comfort with expressing opinions in teams and leading group discussions. As compared to the start of term, students reported being significantly more comfortable expressing opinions in group meetings (t(78) = 4.25, p < 0.001, Cohen's d = 0.48), and leading group discussions (t(78) = 3.11, p = 0.003, Cohen's d = 0.35), by the end of the term.
Although no class time was spent teaching and analyzing team cooperation skills or assigning roles to students, as in done in Cooperative Learning (Johnson et al., 1998), teams functioned better over time. When comparing the one-minute reflections (completed following the first and fifth TBL activities) we found a 14% increase (77% vs. 91%) in number of students reporting on collective team decision making, a 21% increase (48% vs. 69%) in number of reports of satisfaction with their personal contributions and a 25% increase (66 vs. 91%) in number of students reporting a preference for continue to work in the same group.
In the post survey, students were asked to rate their experience with the team in TBL (Fig. 5). On average, more than 50% of students reported agreeing or strongly agreeing with all elements of the team experience.
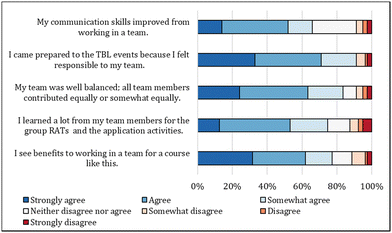 |
| Fig. 5 Team Experiences in the TBL Activities. | |
Limitations and future work
In this study is it is not known if students’ responses to the pre/post survey or 1 minute reflections are completely honest and not influenced by a desire to please the researchers. It is possible that students were more likely to rate TBL highly because they were in a study about TBL. It is also unknown if the changes in pre/post survey results are solely based on students’ participation in the TBL events. Further, generalizations are presented here-TBL is beneficial for the majority of students but there may be students with life experiences that do not benefit from TBL. An area of future research for those interested in pursuing TBL would be to look at the experiences of students from equity-deserving groups with TBL. It is possible that students with neurodivergence, mental health barriers, or those from historically marginalized backgrounds may have different experiences in team settings. Results presented here are from a single national context, characterized by a relatively low power distance and high individualism (Hofstede, 2011). Generalizing the findings presented here to other culturally distinct contexts may be limited.
Conclusions
Team-based learning combines context-based teaching with active learning in a highly structured way and was implemented in a large Environmental Chemistry course. This study indicates that the benefits of TBL described in existing literature in the fields of health and business (Michaelsen and Sweet, 2008; Hrynchak and Batty, 2012; Hagen, 2016) also extends to a large environmental chemistry course. As seen for other active learning techniques, TBL enhances interest in Chemistry and student performance. Results shown here indicate that students perceived that TBL helped to: create connections between course content and the real-world, enhance student interest in the course, and enhance student critical thinking skills. Final exam analysis reveals that students performed significantly better on questions which tested material that students learned using TBL compared to questions testing content learned through other teaching strategies despite the complexity of questions being similar between the two categories. In addition, TBL enhances team skills without spending class time on teaching team skills. Students reported that their team skills, such as ability to communicate and work collaboratively to come to consensus improved as a result of TBL. Developing materials and trying a new pedagogy is daunting and time-consuming but Chemistry educators of large classes should feel assured by the evidence presented here that TBL can be used to apply in-class chemistry concepts to relevant and real-life scenarios, all while channelling the same, if not greater, benefits of other active learning pedagogies.
Conflicts of interest
There are no conflicts to declare.
Appendices
Appendix A
Pre and post survey questions
Question used for both pre/post survey.
1. Would you be interested in taking more Environmental Chemistry courses in the future? (yes/no/maybe)
2. If given the choice, how would you prefer to learn in the classroom? (always alone/mostly alone/a mix of alone and in a group/mostly in a group/always in a group)
3. How do you prefer to learn outside the class? (always alone/mostly alone/a mix of alone and in a group/mostly in a group/always in a group)
4. How comfortable are you expressing your opinions in groups? (extremely comfortable/moderately comfortable/slightly comfortable/neither comfortable nor uncomfortable/slightly uncomfortable/moderately uncomfortable/extremely uncomfortable)
5. How comfortable are you leading a group discussion in a team? (extremely comfortable/moderately comfortable/slightly comfortable/neither comfortable nor uncomfortable/slightly uncomfortable/moderately uncomfortable/extremely uncomfortable)
6. Please indicate to what extent do you agree or disagree with the following statements about chemistry: (strongly agree/agree/somewhat agree/neither agree nor disagree/somewhat disagree/disagree/strongly disagree)
a. Learning chemistry changes my ideas about how the world works.
b. Reasoning skills used to understand chemistry can be helpful to me.
c. The subject of chemistry has little relation to what I experience in the real world.
d. To understand chemistry, I sometimes think about my personal experiences and relate them to the topic being analyzed.
7. To what extent do you feel that Chemistry improves our lives? (improves a lot/improves a little/neither improves nor does not improve/does not improve/does not improve at all)
8. To what extent do you feel that Chemistry is relevant to everyday life? (very relevant/a little relevant/neither relevant nor irrelevant/barely relevant/very irrelevant)
9. This part of the questionnaire investigates the perceptions you have about chemistry and related topics. For example: If you feel chemistry is mostly about the study of natural substances, and only a little bit about the study of synthetic material then you would answer the following question as shown:
a. Chemists are:
Athletic |
Unfit |
Socially aware |
Socially unaware |
Environmentally aware |
Environmentally unaware |
Flexible in their ideas |
Fixed in their ideas |
Care about the effects of their results |
Only care about their results |
Imaginative |
Unimaginative |
Friendly |
Unfriendly |
Inquisitive |
Indifferent |
Patient |
Impatient |
b. Science documentaries are:
c. Chemistry websites are:
d. Chemistry jobs are:
Challenging |
Easy |
Varied |
Repetitive |
Interesting |
Boring |
Satisfying |
Unsatisfying |
Exciting |
Tedious |
e. Talking to my friends about chemistry:
f. Science fiction movies:
Excitingtedious
10. This part of the questionnaire investigates the confidence you have in undertaking different tasks. Please indicate how CONFIDENT you feel about (very confident/confident/a little confident/neither confident nor unconfident/a little unconfident/unconfident/very unconfident)
a. Tutoring another student in a first year chemistry course
b. Determining what answer is required from a written description of a chemistry problem
c. Explaining something that you learned in this chemistry course to another person
d. Choosing an appropriate formula to solve a chemistry problem
e. After reading an article about a chemistry experiment, writing a summary of the main points
f. Learning chemistry theory
g. Determining the appropriate units for a result determined using a formula
h. After watching a television documentary dealing with some aspect of chemistry, writing a summary of its main points
i. After listening to a public lecture regarding some chemistry topic, explaining its main ideas to another person
Question used for post-survey only.
1. Which of the following course components helped you achieve the required goal? Select all that apply (lectures/quizzes/team-based learning/case studies/discussion and forums)?
a. Practicing critical thinking skills
b. Practicing problem solving skills
c. Enhancing interest in Chemistry
d. Understanding of course material
2. What did you like most about Team-based Learning (TBL) in Chemistry 301?
3. What did you like least about TBL in Chemistry 301?
4. My interest in the course improved as a result of the TBL events (strongly disagree/disagree/somewhat disagree/neither agree or disagree/somewhat agree/agree/strongly agree)
5. Please explain your answer to the previous question.
6. Would you recommend using TBL for Chemistry 301 in the future? Why or why not?
7. Please indicate the extent to which you agree to disagree with the following statements regarding the team work in TBL (strongly disagree/disagree/somewhat disagree/neither disagree nor agree/somewhat agree/agree/strongly agree)
a. I learned a lot from my team members for the group RATs and the application activities
b. My team was well balanced; all team members contributed equally or somewhat equally.
c. I came prepared to the TBL events because I felt responsible to my team.
d. My communication skills improved from working in a team.
e. I see benefits to working in a team for a course like this.
Appendix B
Blooming final exam questions
Non-TBL related questions |
Bloom level (1–4) |
Rater 1 |
Rater 2 |
Consensus rating |
Water has a high boiling point primarily because: |
1 |
2 |
2 |
(a) It forms covalent bonds with other water molecules |
(b) It forms H-bonds with other water molecules |
(c) It has a large molecular weight relative to other solvents |
(d) It is a polar molecule |
(e) All of the above |
|
Ammonium, NH4+ can be oxidized to form nitrate, NO3− in a 1 : 1 ratio. If we have a 10 ppm solution of NH4+, which of the following is true? |
4 |
4 |
4 |
(a) This process is called nitrification and the concentration of NO3− formed would be 34 ppm |
(b) This process is called nitrification and the concentration of NO3− formed would be 10 ppm. |
(c) This process is called nitrification and the concentration of NO3− formed would be 9.0 × 10−3 ppm. |
(d) This process is called ammonification and the concentration of NO3− formed would be 10 ppm. |
(e) This process is called ammonification and the concentration of NO3− formed would be 9.0 × 10−3 ppm. |
(f) This process is called ammonification and the concentration of NO3− formed would be 34 ppm. |
(g) This process is called nitrate assimilation and the concentration of NO3− formed would be 10 ppm. |
(h) This process is called nitrate assimilation and the concentration of NO3− formed would be 9.0 × 10−3 ppm. |
(i) This process is called nitrate assimilation and the concentration of NO3− formed would be 34 ppm. |
|
Which of the following statements are true? |
3 |
2 |
3 |
(a) At 0 °C, fresh water is at its maximum density. |
(b) At 0 °C, the kinetic energy of fresh water is at its maximum. |
(c) At 4 °C, the hydrogen bonding in fresh water is at its maximum. |
(d) At 4 °C, the kinetic energy of fresh water is at its maximum. |
(e) At 4 °C, seawater is at its maximum density. |
(f) More than one statement is true. |
(g) None of the above statements are true. |
|
Given [HCO3−] = 0.04 mM, [SO42−] = 0.08 mM, [NO3−] = 0.20 mM, and pH = 7, what is the total alkalinity of the system (in meq)? |
4 |
4 |
4 |
(a) 0.04 |
(b) 0.08 |
(c) 0.12 |
(d) 0.16 |
(e) 0.28 |
(f) 0.30 |
(g) 0.32 |
(h) 0.36 |
(i) 0.40 |
(j) 0.44 |
(k) 0.52 |
|
Addition of a polyelectrolyte causes the precipitation of colloidal material primarily by: |
2 |
2 |
2 |
(a) decreasing the electric double layer |
(b) Increasing the ionic strength of solution |
(c) Changing the pH to match the zero point charge of the colloid. |
(d) Bridging colloids |
(e) Modifying the size of the colloids so that they exhibit the Tyndall effect. |
|
Complete the following sentence: In seawater, the hydrolysis product of Al3+ would be the _________ complex but in acid mine waters, Al3+ would be mainly found as its________ complex. |
3 |
3 |
3 |
(a) Aqua, hydroxo |
(b) Aqua, oxo |
(c) Hydroxo, aqua |
(d) Hydroxo, oxo |
(e) Oxo, aqua |
(f) Oxo, hydroxo |
|
Manganese may precipitate as MnCO3 from aqueous solution according to the reaction: Mn2+ (aq) + CO2 (aq) + H2O (l) ⇌ MnCO3 (s) + 2H3O+ (aq) |
3 |
3 |
3 |
Which element is being reduced and which is being oxidized in this reaction? |
(a) Mn is oxidized, C is reduced |
(b) Mn is oxidized, O is reduced |
(c) C is oxidized, Mn is reduced |
(d) H is oxidized, Mn is reduced |
(e) C is oxidized, O is reduced |
(f) H is oxidized, C is reduced |
(g) None – the reaction does not involve an electron transfer |
|
Which of the following ligands do you expect to form the strongest complexes with Ti4+? |
4 |
3 |
4 |
(a) Cl− |
(b) OH− |
(c) SO42− |
(d) Cysteine: |
|
|
(e) Serine: |
(f) B and C would both form equally the strongest complexes |
(g) D and E would both form equally the strongest complexes |
|
Which two species primarily cause acid rain? |
1 |
1 |
1 |
(a) NO and CO2 |
(b) SO2 and CO2 |
(c) SO2 and O2 |
(d) H2 and CO2 |
(e) NO and SO2 |
|
Which of the following statements is FALSE? |
2 |
2 |
2 |
(a) Increasing temperature speeds up the rate of microbial-mediated reactions at all temperatures. |
(b) Bioaccumulation can occur when a toxic species is more soluble in fatty tissue than in water. |
(c) Chelation of metals in the aqueous environment can increase the solubility and decreases the bioavailability of the metal |
(d) The solubility of CaSO4(s) is best determined by measuring [Ca2+(aq)] that goes into solution as well as the amount of CaSO04(aq) that goes into solution. |
(e) Both a and b are false. |
(f) Both a and d are false. |
|
Consider the reduction of sulfur, shown in the equation below: |
3 |
3 |
3 |
1/8SO42− (aq) + 5/4H+ (aq) + e− ⇌ 1/8 H2S (aq) + 1/2H2O (l) pε° = 5.1 |
What is the pε of an environment controlled by this reaction, if pH = 8, and [H2S] = 10[SO42−]? |
(a) −5.0 |
(b) 5.1 |
(c) −5.9 |
(d) 15.2 |
(e) −10.1 |
|
During the final step of nitrate assimilation, which of the following oxidation state transformations of nitrogen can occur? |
2 |
2 |
2 |
(a) +5 to 0 |
(b) 0 to +5 |
(c) + 3 to +5 |
(d) + 5 to +3 |
(e) + 3 to −3 |
(f) −3 to +5 |
|
Determine the solubility product constant (Ksp) for silver chloride at 25 °C, using the equations and pε° values provided below. |
4 |
4 |
4 |
Ag+(aq) + e− → Ag(s) pε° = 13.52 |
AgCl(s) + e− → Ag(s) + Cl−(aq) pε° = 3.76 |
(a) Ksp = 5.25 × 10−18 |
(b) Ksp = 3.02 × 10−14 |
(c) Ksp = 1.74 × 10−10 |
(d) Ksp = 5.75 × 103 |
(e) Ksp = 5.75 × 109 |
(f) Ksp = 3.31 × 1013 |
(g) Ksp = 1.91 × 1017 |
|
Vancouver area has mainly granite bedrock, not calcium carbonate. Which of the following statements is FALSE? |
2 |
2 |
2 |
(a) Vancouver is more sensitive to the effects of acid rain. |
(b) Vancouver's lakes will be generally more acidic than other areas that have calcium carbonate bedrock. |
(c) Vancouver's water is harder than other areas that have calcium carbonate bedrock. |
(d) If two lakes in Vancouver area have the same pH, they may not have the same total alkalinity. |
(e) Both a and b |
(f) Both b and c |
|
Which of the following is the most likely structure of an organic pollutant? |
2 |
2 |
2 |
|
|
Which of the following statements is true about the organic pollutants, PCB's, dioxins and DDT? |
2 |
2 |
2 |
(a) PCB's are a byproduct produced during dioxin degradation. |
(b) DDT is a common byproduct of the pulp and paper industry in BC. |
(c) Dioxins are produced for use as coolants and insulators. |
(d) Dioxins are a byproduct produced during degradation of PCB's. |
(e) all of the above |
|
When an organic pollutant is added to a stream, under what conditions are you most likely to find a septic zone downstream? |
1 |
2 |
2 |
(a) When BOD > dissolved O2 and there is little turbulent mixing |
(b) When BOD > dissolved O2 and there is a lot of turbulent mixing |
(c) When BOD < dissolved O2 and there is little turbulent mixing |
(d) When BOD < dissolved O2 and there is a lot of turbulent mixing |
|
In Bangladesh, 30–80 million people were exposed to arsenic polluted drinking water. What was the source of arsenic? |
1 |
1 |
1 |
(a) Industrial wastes |
(b) Pesticides |
(c) Fertilizers |
(d) The bedrock in Bangladesh |
(e) Acid rock drainage (ARD) |
|
Given the following distribution diagram for a ligand “L”, which can bind with 4 H's, the curve labeled “X” represents ____, the pKa for this species is approximately ____: |
4 |
3 |
4 |
|
|
When anoxic sediments containing FeS(s) are exposed to oxygenated surface water: |
2 |
3 |
3 |
(a) Fe oxidizes and S oxidizes |
(b) Fe oxidizes, and S reduces |
(c) Fe does not oxidize and S oxidizes |
(d) Fe does not oxidize and S reduces |
(e) Fe oxidizes, S does not oxidize |
(f) Fe does not oxidize, S does not oxidize |
|
Equal amounts of Pb2+ and Mn2+ ions were added simultaneously to a sample of fresh water containing excess CO32− and excess NTA3−. Which of the following statements is TRUE? |
3 |
3 |
3 |
(a) There will be more “free” Pb2+ ions than “free” Mn2+ ions |
(b) There will be less “free” Pb2+ ions than “free” Mn2+ ions |
(c) There will be less Pb–NTA complex than Mn–NTA complex |
(d) Both a and c |
(e) Both b and c |
|
When chlorine, Cl2(g) is added as a disinfectant at pH = 6 to oxygenated water, it is mainly converted to the form: |
2 |
2 |
2 |
(a) Cl2 (aq); dissolved chlorine |
(b) Cl− (aq); chloride ion |
(c) HOCl (aq); hypochloric acid |
(d) OCl− (aq); hypochlorite ion |
(e) A 50 : 50 mix of Cl− and Cl2 (aq) |
(f) A 50 : 50 mix of HOCl (aq) and OCl− (aq) |
|
The Henry's Law constant for O2 in water at 25 °C is 1.28 × 10−3 mole L−1 atm−1. Which of the following is a reasonable prediction of the constant at 500C? |
3 |
4 |
3 |
(a) 6.28 × 10−4 mole L−1 atm−1 |
(b) 2.51 × 10−3 mole L−1 atm−1 |
(c) 1.16 × 10−3 mole L−1 atm−1 |
(d) 6.28 × 10−3 mole L−1 atm−1 |
|
1. The pε-pH diagram below shows an alternate look at Fe speciation, compared to the one we derived in class, where the presence of carbonate is also considered, as well as multiple forms of the iron hydroxides. It is also unlike most others we have seen in that the lines are extended beyond the region of water stability, and hatch marks are drawn around the regions that show the dominance of a solid form. Take a minute to look at the diagram carefully and then answer the following questions: |
2 |
2 |
2 |
|
(a) What form of Fe in an aquatic system do you expect to dominate under anoxic conditions (controlled by the CO2/CH4 couple) at pH 2.5? |
|
(b) List ONE transition of Fe in an aquatic system that is only a proton transfer (does not depend on pε). You do not need to write a balanced chemical equation (e.g. H2O (l) → O2 (g) is a transition) |
2 |
2 |
2 |
|
(c) List ONE transition of Fe in an aquatic system that is only an electron transfer (does not depend on pH). You do not need to write a balanced chemical equation (e.g. H2O (l) → O2 (g) is a transition) |
2 |
2 |
2 |
|
(d) List ONE transition of Fe in an aquatic system that is dependent on both pε and pH. You do not need to write a balanced chemical equation (e.g. H2O (l) → O2 (g) is a transition) |
2 |
2 |
2 |
|
(e) What is corrosion? |
1 |
1 |
1 |
|
(f) Explain why iron is easily corroded in aqueous environments. |
4 |
3 |
3 |
|
2. Wastewater containing 8.0 mg L−1 O2, 1.00 × 10−3 M NO3− and 1.00 × 10−2 M soluble organic matter, {CH2O}, is stored and isolated from the atmosphere in a container richly seeded with a variety of bacteria. Assume that respiration using oxygen and/or nitrate can occur during storage. |
1 |
2 |
2 |
Respiration using O2: |
O2 + {CH2O} ⇌ CO2 + H2O |
Respiration using NO3−: |
4 NO3− + 4H+ + 5{CH2O} ⇌ 5CO2 + 7H2O + 2N2 |
(a) Which respiration reaction (with O2 or NO3−) is more energy efficient (has a larger negative ΔG° value)? |
|
(b) In what order will respiration occur? |
2 |
2 |
2 |
i. By O2 first then by NO3− |
ii. By NO3−, then O2 |
iii. Both reactions will occur simultaneously |
iv. Only respiration using O2 is needed. |
|
(c) Which oxidant (O2 or NO3−) will consume more of the organic matter? Support your answer with calculations. |
4 |
4 |
4 |
|
(d) Under these conditions, will ALL the soluble organic matter in the wastewater sample be degraded? (yes/no). |
3 |
3 |
3 |
|
3. Given: in a fresh water system, the total NTA = 1.0 × 10−2 M, [HCO3−] = 1.0 × 10−3 M and pH = 6.8 |
2 |
3 |
3 |
(a) Calculate the concentration of CO32− at this pH: |
|
(b) What is the dominant form of uncomplexed NTA? |
2 |
2 |
2 |
|
(c) What are the dominant soluble forms of Pb in this solution? |
3 |
3 |
3 |
|
(d) Assume total Pb ≪< total NTA. Calculate the total soluble Pb in this solution. |
4 |
4 |
4 |
4. A radioisotope has a biological half-life of 24 hours and a nuclear half-life of 12 hours. |
3 |
3 |
3 |
(a) What percentage of the total concentration will be the daughter element inside of a person's body after 24 hours? |
|
(b) In general, how is the half-life of a radioisotope (short, intermediate or long) related to its impact on the environment? Explain the impact: |
2 |
2 |
2 |
Impact of short half-life: |
Impact of intermediate half-life: |
Impact of long half-life: |
|
(c) If a radioisotope was unstable because its nucleus contained too many neutrons relative to protons, predict the type of radiation that would occur: |
1 |
2 |
2 |
i. Alpha radiation |
ii. Beta radiation |
iii. Gamma radiation |
|
(d) Provide a rationale for your choice in (c). |
3 |
2 |
2 |
TBL-related questions |
Bloom level (1–4) |
Rater 1 |
Rater 2 |
Consensus rating |
Nutrients do not get trapped in the bottom of temperate lakes but they can in tropical lakes. Why? |
2 |
2 |
2 |
(a) Respiration does not occur at the bottom of temperate lakes |
(b) Temperate lakes overturn when the surface water reaches 0 degrees in the fall or spring |
(c) Temperate lakes overturn when the surface water reaches 4 degrees in the fall or spring |
(d) In tropical lakes, the surface and deep water are not well stratified. |
(e) In tropical lakes, the entire lake can freeze. The deep water reaches 0 degrees in the fall or spring |
|
Which of the following statements is FALSE? |
2 |
1 |
1 |
(a) Cadmium can replace zinc in enzymes. |
(b) Lead has, in the past, been used in pipes. |
(c) Mercury in its methylated form is less toxic than its inorganic form. |
(d) Arsenite, AsO33−, is a toxic form of arsenic. |
(e) Copper can be both limiting and toxic. |
|
Before the limnic eruption in Lake Nyos, a parcel of water containing CO2 from the bottom was brought up closer to the surface. Which of the following is true? |
3 |
4 |
4 |
(a) CO2 is more soluble near the surface primarily because of the increase in salinity near the surface |
(b) CO2 is less soluble near the surface primarily because of the increase in salinity near the surface |
(c) CO2 is more soluble near the surface primarily because of the wind action at the surface |
(d) CO2 is less soluble near the surface primarily because of the wind action at the surface |
(e) CO2 is more soluble near the surface primarily because of the lower pressure at the surface |
(f) CO2 is less soluble near the surface primarily because of the lower pressure at the surface |
(g) All of A, C and E |
(h) All of B, D, and F |
|
Fill in the missing species in the following nuclear equation: |
2 |
2 |
2 |
234Pa → 234U + — |
(a)  |
(b)  |
(c)  |
(d)  |
(e)  |
|
In the aerobic treatment of acid mine drainage, often Ca(OH)2 and/or CaCO3 are used to treat the acid. What happens under these conditions? |
3 |
3 |
3 |
(a) The pH increases and metals precipitate as metal hydroxides. |
(b) The pH increases and metals precipitate as hydrated (aqua) metal complexes. |
(c) Yellowboy is removed from the area. |
(d) It causes Fe3+ to reduce to Fe2+. |
(e) It causes methanogenesis, which increases the pH of the system. |
|
An alpha particle, once it leaves an atom, is the same as: |
1 |
1 |
1 |
(a) A neutron |
(b) An electron |
(c) A hydrogen nucleus |
(d) A deuterium nucleus |
(e) A helium nucleus |
|
The Flint water crisis occurred because the Flint river water was corrosive and that resulted in leaching of lead into the drinking water from pipes. Which of the following statements is FALSE? |
3 |
3 |
3 |
(a) The Flint river water was more corrosive due to high levels of chloride (Cl−) ions. |
(b) It is important to perform the aeration step of water treatment of the Flint river before adding Cl2 as a disinfectant because the presence of Fe2+ will react with Cl2 to form Cl−. During aeration, Fe2+ is oxidized to Fe3+. |
(c) The presence of trimethylhalides in the Flint drinking water was due to Cl2 reacting with organic matter. |
(d) Alum was used as a coagulant during water treatment in Flint. |
(e) Both b and c are false. |
(f) Both b and d are false. |
(g) Both c and d are false. |
|
1. Bristol Bay in Alaska was initially protected from mining in 2014 partially due to concerns over copper toxicity to fish and other aquatic organisms. |
1 |
1 |
1 |
(a) Many of the lakes in Bristol Bay are oligotrophic. Define oligotrophic. |
|
(b) Copper has been found to be more toxic in oligotrophic waters than in eutrophic waters. Why would copper toxicity increase in oligotrophic waters? |
4 |
4 |
4 |
|
(c) Many of the lakes in Bristol Bay are soft waters. What is hard water? |
1 |
1 |
1 |
|
(d) Copper has been found to be more toxic in soft waters compared to hard waters. Why would copper toxicity increase in soft waters? |
4 |
4 |
4 |
Acknowledgements
We would like to thank the UBC Scholarship of Teaching and Learning Seed fund for supporting this study and the CHEM 301 students for participating in this study. We also wish to express our appreciation to Dr Kristin Orians for serving an independent rater of the team worksheets and final exam questions. Thank you to Dr Joss Ives for his remarks and encouragement of this paper.
References
- Adams W. et al., (2006), New instrument for measuring student beliefs about physics and learning physics: The Colorado Learning Attitudes about Science Survey, Phys. Rev. Spec. Top. – Phys. Educ. Res., 2(010101), 1–18 DOI:10.1103/PhysRevSTPER.2.010101.
- Agwu U. D. and Nmadu J., (2023), Students’ interactive engagement, academic achievement and self concept in chemistry: an evaluation of cooperative learning pedagogy, Chem. Educ. Res. Pract., 24(2), 688–705 10.1039/d2rp00148a.
- Bain K. et al., (2020), Characterizing college science instruction: The Three-Dimensional Learning Observation Protocol, PLoS One, 15(6), 1–20 DOI:10.1371/journal.pone.0234640.
- Brady D. and Voronova K., (2023), Flipped Online Active Learning Environment for Large General Chemistry Courses, J. Chem. Educ., 100(3), 1109–1117 DOI:10.1021/acs.jchemed.2c00602.
- Broman K. and Parchmann I., (2014), Students’ application of chemical concepts when solving chemistry problems in different contexts, Chem. Educ. Res. Practice, 15(4), 516–529 10.1039/c4rp00051j.
- Cakir M., (2008), Constructivist Approaches to Learning in Science and Their Implications for Science Pedagogy: A Literature Review, Int. J. Environ. Sci. Educ., 3(4), 193–206.
- Capel N. J. et al., (2021), Using Team Based Learning to Promote Problem Solving Through Active Learning, in Tsaparlis G. (ed.) Problems and Problem Solving in Chemistry Education: Analysing Data, Looking for Patterns and Making Deductions, Croyden, UK: The Royal Society of Chemistry, pp. 279–319.
- Chapman K. J. et al., (2006), Can’t we pick our own groups? the influence of group selection method on group dynamics and outcomes, J. Manage. Educ., 30(4), 557–569 DOI:10.1177/1052562905284872.
- Coll R. K., Dalgety J. and Salter D., (2002), The development of the Chemistry Attitudes and Experiences Questionnaire (CAEQ), Chem. Educ.: Res. Pract. Eur., 3(1), 19–32.
- Comeford L., (2016), Team-Based Learning Reduces Team-Based Learning Reduces Attrition in a First-Semester General Chemistry Course, J. College Sci. Teach., 46(2), 2016. Available at: https://www.ncbi.nlm.nih.gov/pubmed/26849997%0Ahttps://doi.wiley.com/10.1111/jne.12374.
- Crouch C. H. et al., (2007), Peer Instruction: Engaging Students One-on-One, All At Once, in E. F. Redish and P. Cooney (eds) Research-Based Reform of University Physics. College Park: American association of physics teachers, 1–55 DOI:10.1364/OPN.9.9.000037.
- Davidson L. K., (2011), A 3 year exper),ience implementing blended TBL: active instructional methods can shift student attitudes to learning, Medical Teacher, 33(9 750–753 DOI:10.3109/0142159X.2011.558948.
- Deslauriers L., Schelew E. and Wieman C., (2011), Improved learning in a large-enrollment physics class., Science, 332(6031), 862–864 DOI:10.1126/science.1201783.
- Donnelly J. and Hernández F. E., (2018), Fusing a reversed and informal learning scheme and space: student perceptions of active learning in physical chemistry, Chem. Educ. Res. Pract., 19(2), 520–532 10.1039/c7rp00186j.
- Dunham B., Yapa G. and Yu E., (2015), Calibrating the difficulty of an assessment tool: the blooming of a statistics examination, J. Statist. Educ., 23(3), 1–33 DOI:10.1080/10691898.2015.11889745.
- European Commission, Directorate-General for Research and Innovation, (2015), Science education for responsible citizenship – Report to the European Commission of the expert group on science education, Publications Office, https://data.europa.eu/doi/10.2777/12626.
- Fair J. D., Kleist E. M. and Stoy D. M., (2014), A survey of industrial organic chemists: Understanding the chemical industry's needs of current bachelor-level graduates, J. Chem. Educ., 91(12), 2084–2092 DOI:10.1021/ed400570f.
- Freeman S., Haak D. and Wenderoth M. P., (2011), Increased course structure improves performance in introductory biology, CBE Life Sci. Educ., 10(2), 175–186 DOI:10.1187/cbe.10-08-0105.
- Freeman S. et al., (2014), Active learning increases student performance in science, engineering, and mathematics, Proc. Natl. Acad. Sci. U. S. A., 111(23), 8410–8415 DOI:10.1073/pnas.1319030111.
- Funk C. et al., (2020), Science and scientists held in high esteem across global publics, Pew Res. Center Rep., 1–133. Available at: https://www.pewresearch.org/science/2020/09/29/science-and-scientists-held-in-high-esteem-across-global-publics/.
- Guerris M. et al., (2020), Describing the public perception of chemistry on twitter, Chem. Educ. Res. Pract., 21(3), 989–999 10.1039/c9rp00282k.
- Hagen J. P., (2016), Team-Based Learning in Physical Chemistry, in Muzyka J. L. and Luker C. S. L. (ed.) The Flipped Classroom: Background and Challenges, American Chemical Society, vol. 1, pp. 55–72 DOI:10.1021/bk-2016-1223.ch005.
- Hofstede G., (2011), Dimensionalizing Cultures: The Hofstede Model in Context, Online Read. Psychol. Culture, 2(1), 1–26 DOI:10.9707/2307-0919.1014.
- Hrynchak P. and Batty H., (2012), The educational theory basis of team-based learning, Medical Teacher, 34(10), 796–801 DOI:10.3109/0142159X.2012.687120.
- Jensen J. L., Kummer T. and Godoy P. D. M., (2015), Improvements from a Flipped Classroom May Simply Be the Fruits of Active Learning, CBE—Life Sciences Education, 14, 1–12 DOI:10.1187/10.1187/cbe.14-08-0129.
- Johnson D. W., Johnson R. and Smith K., (1998), Active Learning: Cooperation in the College Classroom, 2nd edn, Edina, MN: Interaction Book Company.
- Koles P. G. et al., (2010), The impact of team-based learning on medical students’ Academic Performance, Academic Medicine, 85(11), 1739–1745 DOI:10.1097/ACM.0b013e3181f52bed.
- Kondo A. E. and Fair J. D., (2017), Insight into the Chemistry Skills Gap: The Duality between Expected and Desired Skills, J. Chem. Educ., 94(3), 304–310 DOI:10.1021/acs.jchemed.6b00566.
- Kressler B. and Kressler J., (2020), Diverse Student Perceptions of Active Learning in a Large Enrollment STEM Course, J. Scholarship Teach. Learn., 20(1), 40–64 DOI:10.14434/josotl.v20i1.24688.
- Lekhi P. and Nashon S. M., (2016), How a Flipped Classroom Promotes Sophisticated Epistemology: Example from a Large Analytical Chemistry Course, in Muzyka J. L. and Luker C. S. (ed.) The Flipped Classroom: Background and Challenges, American Chemical Society, vol. 1, pp. 93–104 DOI:10.1021/bk-2016-1223.ch007.
- Mahaffy P. G., Bucat B. and Tasker R., (2014), Chemistry: Human Activity, Chemical Reactivity, 2nd edn, Nelson.
- Mahaffy P. G. et al., (2017), Beyond “inert”
Ideas to Teaching General Chemistry from Rich Contexts: Visualizing the Chemistry of Climate Change (VC3), J. Chem. Educ., 94(8), 1027–1035 DOI:10.1021/acs.jchemed.6b01009.
- Manahan S. E., (2005), Environmental Chemistry, 8th edn, Boca Raton Florida: CRC Press.
- Matthews M. R., (1993), Constructivism and Science Education: Some Epistemological Problems, J. Sci. Educ. Technol., 2(1), 359–370.
- Michaelsen L. K., (2002), in Michaelsen L. K., Knight A. B. and Fink Westport L. D. (ed.) Team-based learning: A transformative use of small groups, CT: Greenwood publishing group.
- Michaelsen L. K. and Sweet M., (2008), The Essential Elements of Team-based Learning, New Directions for Teaching and Learning, Wiley Periodicals DOI:10.1002/tl.330.
- Michaelsen L. K., Bauman Knight A. and Fink L. D., (2004) Team-based Learning: A Transformative Use of Small Groups. Sterling, VA: Stylus Publishing.
- Morrison G., Goldfarb S. and Lanken P. N., (2010), Team training of medical students in the 21st century: would Flexner approve?, Acad. Med., 85(2), 254–259 DOI:10.1097/ACM.0b013e3181c8845e.
- Osborne J., Simon S. and Collins S., (2003), Attitudes towards science: a review of the literature and its implications, Int. J. Sci. Educ., 25(9), 1049–1079 DOI:10.1080/0950069032000032199.
- Rahman T. and Lewis S. E., (2020), Evaluating the evidence base for evidence-based instructional practices in chemistry through meta-analysis, J. Res. Sci. Teach., 57(5), 765–793 DOI:10.1002/tea.21610.
- Rania N., Rebora S. and Migliorini L., (2015), Team-based Learning: Enhancing Academic Performance of Psychology Students, Proc.- Soc. Behav. Sci., 174, 946–951 DOI:10.1016/j.sbspro.2015.01.716.
- Stuckey M. and Eilks I., (2014), Increasing student motivation and the perception of chemistry's relevance in the classroom by learning about tattooing from a chemical and societal view, Chem. Educ. Res. Practice, 15(2), 156–167 10.1039/c3rp00146f.
- Taber K. S., (2011), Constructivism as educational theory: Contingency in learning, and optimally guided instruction, in Hassaskhah J. (ed.) Educational Theory, New York, NY: Nova Science Publishers INC, pp. 39–61 DOI:10.5785/21-2-73.
- Tro N. J., (2010) Chemistry: A molecular Approach, 2nd edn Pearson.
- Watkins J. and Mazur E., (2013), Retaining Students in Science, Technology, Engineering, and Mathematics (STEM) Majors, J. College Sci. Teach., 42(5), 36–41 DOI:10.2505/4/jcst13_042_05_36.
- Wieman C. and Perkins K., (2005), Transforming Physics Education, Phys. Today, 36–41.
- Wieman C. and Holmes N. G., (2015), Measuring the impact of an instructional laboratory on the learning of introductory physics, Am. J. Phys., 83(11), 972–978 DOI:10.1119/1.4931717.
- Yalçinkaya E. et al., (2012), Is case-based learning an effective teaching strategy to challenge students’ alternative conceptions regarding chemical kinetics?, Res. Sci. Technol. Educ., 30(2), 151–172 DOI:10.1080/02635143.2012.698605.
|
This journal is © The Royal Society of Chemistry 2024 |