DOI:
10.1039/D4RA02739F
(Paper)
RSC Adv., 2024,
14, 24765-24780
NADPH oxidase-dependent free radical generation and protein adduct formation in neutrophils†
Received
12th April 2024
, Accepted 31st July 2024
First published on 7th August 2024
Abstract
Neutrophils mediate the early innate immune response through extracellular traps comprising intracellular protein and DNA. These traps play a pivotal role in both immunity against invading pathogens and the development of immunopathological reactions through the production of reactive oxygen species (ROS). Proteins serve as the main target for ROS, resulting in the formation of protein adducts. Herein, we report that the superoxide anion radical (O2˙−) plays a vital role in neutrophil function through sequential events involving 5-lipoxygenase (5-LOX) and NADPH oxidase (NOX). More specifically, differences in NOX homologs expression were observed post-stimulation with PMA and LPS. Differentiation conditions and O2˙− generation were confirmed using flow cytometry. Immunoblotting analysis confirmed the time-dependent expression of NOX underlying its requirement and 5-LOX-mediated lipid peroxidation events in neutrophil function. Protein–malondialdehyde (MDA) adducts formed were detected using immunoblotting, and quercetin was evaluated for its ability to scavenge free radicals through electron paramagnetic resonance (EPR) spin-trapping spectroscopy and results were confirmed with blotting analysis. Free radical-mediated protein oxidation events influence neutrophil function and protein adducts formed serve as markers of neutrophil activation upon infection and inflammation. The study warrants further corroboration and the study of specific proteins involved in neutrophil activation and their role in inflammation.
1 Introduction
Neutrophils are terminally differentiated phagocytic cells that form the first line of defence against invading pathogens. Being an essential part of innate immune defence, neutrophils withhold a shorter lifespan and are directed towards the site of infection, inflammation, or damage caused by cytokines.1 Neutrophils employ a wide range of defence strategies, which include phagocytosis (engulfing microbes within a phagosome), degranulation (fusion of granules with the phagosome and release of antimicrobial peptides and enzymes), and recently reported neutrophil extracellular trap formation (NETs) by a process called NETosis.2 NETs are characterized as web-like structures consisting of long threads of DNA decorated with cytoplasmic and nuclear proteins, namely myeloperoxidase (MPO), neutrophil elastase (NE), histones, and cathepsin G. Although the molecular events underlying the formation of NETs are not yet completely known, recent studies support the involvement of various cellular processes, including reactive oxygen species (ROS) formation, autophagy, and histone citrullination.3 NETs are considered a ‘double-edged sword’ as they are reported to be involved in both inflammation resolution4 through innate immune defence mechanisms, as well as excessive formation or reduced clearance after resolution that contribute to various diseased conditions.5–7 The formation of NETs can be achieved by various physiological stimuli, such as recognition of bacteria (lipopolysaccharide) and molecular motifs of other pathogens (fungi, protozoan, parasites, and viruses) or specific cytokines such as interleukin 8 (IL-8), Tumour Necrosis Factor-alpha (TNF-α) and pharmacological stimuli such as phorbol 12-myristate 13-acetate (PMA), potassium, and calcium ionophores can induce the formation of NETs. Two different forms of NETosis have been described, which include classical or suicidal NETosis leading to cell death and vital NETosis wherein the cells retain their viability and effector functions.8 The nature of stimulation decides the NETosis process; thus PMA induces suicidal NETosis and microbial-specific molecular patterns recognised by toll-like receptors mediate vital NETosis.9
The formation of these extracellular traps was reported to be a novel antimicrobial mechanism used by neutrophils to kill invading pathogens, and it depends on ROS generation by NADPH oxidase.10 The involvement of ROS in NETs release is of apparent interest in recent times. These radical species are either generated as by-products of redox reactions in various cellular compartments, including mitochondria, peroxisomes, and the endoplasmic reticulum, or through oxidases and oxygenase enzyme functions. NADPH oxidase (NOX) enzymes are known to be associated with respiratory burst events of phagocytes; thus once activated, NOX transfers electrons from the cytoplasm across the membrane wherein one-electron reduction of molecular oxygen generates the superoxide anion radical (O2˙−), which gets dismutated into hydrogen peroxide (H2O2), either spontaneously or by superoxide dismutase (SOD). Myeloperoxidase (MPO) catalyzes the further formation of hypochlorous acid (HOCl). The presence of transition metals like Fe2+ mediates the formation of a highly reactive hydroxyl radical (HO˙) by the Fenton reaction.9 Detection of these ROS species is highly essential as early detection of changes in these ROS levels within cellular environment are considered to be essential for pathological studies and disease diagnosis. Techniques including EPR spectroscopy, mass spectrometry, and high performance liquid chromatography (HPLC) were employed for ROS detection; however, these techniques pose various limitations due to intrinsic properties of ROS, it diffusion rate, and site of generation. Recently, techniques involving fluorescence and electrochemical methods with high selectivity and sensitivity have been employed. More specifically, the role of scanning electrochemical microscopy in investigating the redox status of healthy and diseased condition remains a valuable tool for the redox process with enhanced sensitivity.10 ROS produced by NOX enzymes activates MPO and azurophilic granules resulting in NE release into the cytosol. NE disrupts plasma membrane integrity and, upon translocation into the nucleus, cleaves histones promoting chromatin decondensation. Peptidylarginine deiminase 4 (PAD 4) mediated citrullination of H3 histones and decondensation of chromatin along with NE.11 Among the seven reported NOX isoforms, NOX 2 and NOX 4 expressions varied with the severity of inflammation. The role of NOX 4 in extracellular neutrophil extracellular formation is largely unknown, and studies focusing on NOX4-dependent NET formation in neutrophils are essential. On the other hand, generated ROS (O2˙− and HO˙) can initiate lipid peroxidation by attacking polyunsaturated fatty acids (PUFAs) where free radicals mediate hydrogen abstraction from carbon and insert molecular oxygen, resulting in the formation of lipid peroxyl radicals (LOO˙) which abstract other hydrogen atoms forming hydroperoxides (LOOH) as primary products of lipid peroxidation. The lipid hydroperoxides decompose further, resulting in the formation of reactive aldehydes as secondary products, namely 4-hydroxynonenal (HNE) and malondialdehyde (MDA). Protein carbonylation results as a consequence of the covalent attachment of these electrophilic aldehydes to the lysine, histidine and cysteine residues or by direct interaction with HOCl and chloramines.12 Lipoxygenases (LOXs) mediate the formation of LOOH and are proposed to be the key regulator enzymes mediating pro-inflammatory and anti-inflammatory responses. Among the six reported human LOXs, the expression of 5-LOX is predominant in leukocytes that catalyze leukotrienes (LTs) biosynthesis, a group of lipid mediators of inflammation derived from arachidonic acid (AA).13 These lipid mediators promote neutrophil migration to the site of inflammation. Deciphering the role of NOX 4 and 5-LOX will help to understand ROS-dependent neutrophil extracellular trap formation during sterile and diseased inflammation conditions.
Investigations of free radical-mediated protein modification in activated neutrophils are limited, so the identification of susceptible protein targets that undergo oxidative modification will serve as a marker to distinguish the NETosis process involved, as well as neutrophil activation during infection and inflammation. Thus, the present study aims at understanding the lipid peroxidation-mediated protein oxidation events in neutrophil extracellular trap formation and the detection of free radical adducts using the immuno-spin trapping technique. With an average half-life of 6–8 h in the bloodstream and a lack of ability to proliferate, it is impossible to expand the neutrophil in vitro. Thus, HL-60 has been used as the most commonly used cell line model for neutrophil research. All-trans retinoic acid (ATRA), dimethylformamide (DMF), dimethyl sulfoxide (DMSO) and actinomycin D have been reported to induce differentiation of HL-60 into neutrophil-like cells.14 Flavonoids with their antioxidant capabilities and ability to modulate protein targets involved in the cell proliferation and cell death pathways are of much interest.14 Quercetin, a major dietary flavonoid, remains an integral part of the human diet, and its beneficial role in health is supported by various epidemiological data.15 These compounds have been reported to act as antioxidant and thus enhance immune functions both in vitro and in vivo.16–18 It exerts its anti-inflammatory activity by regulating the expression of pro-inflammatory factors, namely interleukin-6 (IL-6). Thus, the effect of quercetin on neutrophils stimulated for NETs formation was considered. In this study, protein carbonylation events in neutrophils stimulated for NETosis were analyzed, and protein targets undergoing MDA adduct formation were identified using malondialdehyde-specific antibodies. Both NETosis and protein carbonylation events are dependent on NOX and LOX activity, and inhibitor studies revealed the involvement of free radical-generated oxidation products in neutrophil function.
2 Materials and methods
2.1 Reagents
All-trans-retinoic acid (ATRA), PMA, lipopolysaccharide (LPS) from E. coli O111:B4, dihydroethidium (DHE) and diethylenediamine pentaacetic acid (DTPA) were procured from Sigma Aldrich (St. Louis, Missouri, USA). MTT Cell Proliferation Assay Kit, Rabbit polyclonal NOX4 antibody, rabbit polyclonal anti-malondialdehyde (MDA) antibody, and rabbit monoclonal anti-5 lipoxygenase antibody were obtained from Abcam (Cambridge, UK). CD11b Monoclonal Antibody (M1/70), FITC, and FVD-eFluor405 were purchased from eBioscience, Thermo Scientific (Massachusetts, USA). The FITC Annexin V/Dead cell Apoptosis Kit was obtained from Invitrogen, Thermo Scientific (Massachusetts, USA). NOX2 polyclonal antibody, Histone H3.3 polyclonal antibody, Mouse monoclonal CD63 antibody, MPO polyclonal antibody, Goat polyclonal HRP-conjugated Affinipure Goat Anti-Mouse IgG(H + L) and HRP-conjugated Affinipure Goat Anti-Rabbit IgG (H + L) were purchased from Proteintech (Martinsried, GmbH Germany). Protease and phosphatase inhibitors were received from Roche (Mannheim, Germany). 1-Hydroxy-3-carboxy-pyrrolidine (CPH). Hydrochloride was purchased from Enzo Life Sciences (New York, USA). Cell culture media and antibiotics were procured from Biosera (Nuaille, France).
2.2 Cell culture and differentiation conditions
The HL-60 cell line was purchased from American Type Culture Collection (ATCC; Rockville, Maryland, USA) and cultured in RPMI 1640 medium supplemented with 10% fetal bovine serum, 1% penicillin–streptomycin solution (v/v). Cells were passaged twice a week and maintained in a humidified atmosphere of 5% CO2 at 37 °C. HL-60 cells were seeded at 0.5 × 106 mL−1 in complete media for experimentation and differentiated with ATRA (0.5 μM) for three days. Cell viability followed by treatment with inducers (PMA, LPS, and ATRA) was determined by the MTT assay.
2.3 Flow cytometric analysis
Cell differentiation was assessed by evaluating the expression of surface CD markers using an SP6800 spectral cell analyzer (Sony Biotechnology, San Jose, California, USA) equipped with 405 nm, 488 nm and 637 nm lasers. The surface expression of CD11b, a neutrophil surface marker, was evaluated to investigate the differentiation efficacy and the FITC Annexin V Apoptosis Detection Kit was used to determine the viability of differentiated cells using a SP6800 spectral cell analyzer. For the analysis of CD11b, cells were collected and subjected to centrifugation at 250g for 5 min at RT and the pellets were resuspended in 1× DPBS (Dulbecco's phosphate buffered saline). The washing procedure was repeated twice to remove residual media. The resulting pellets were resuspended in 1× DPBS solution containing 5% FBS, viability dye (0.5 μL) FVD-eFluor450 (eBioscience, San Diego, California, USA) and FITC-labeled CD11b monoclonal antibody (5 μL). The mixture was incubated in the dark for 30 min and centrifuged at 250g for 5 min to collect cell pellets. The pellets were washed twice with 1× DPBS to remove unbound antibodies and 200 μL of the sample was subjected to analysis. The expression of the CD11b marker was evaluated for a week to optimize the differentiation condition.
Similarly, the identification of apoptotic and necrotic cells was achieved by the FITC Annexin V Apoptosis Detection Kit with propidium iodide (PI). Cell pellets washed with 1× DPBS were resuspended in Annexin V Binding Buffer at 0.5 × 107 cells per mL. 100 μL of resuspended mixture was transferred into flow cytometry tubes and incubated for 15 min at RT in the dark along with 5 μL of Annexin V and 10 μL PI solution. Post incubation, 400 μL of Annexin V Binding Buffer was added to the mixture and subjected to flow cytometry analysis. Data were analyzed with FlowJo software (Becton Dickinson, Franklin Lakes, New Jersey, USA).
2.4 Neutrophil differentiation and stimulation
Differentiated neutrophils were seeded at 2–5 × 104 cells per well in six-well plates and allowed to settle for 30 min at 37 °C, 5% CO2 in the absence or presence of inhibitors. Following incubation, cells were stimulated with 50 nM PMA or 100 ng mL−1 LPS for 3 h in serum-free RPMI media.19,20 Samples were collected at two time intervals of 1.5 and 3 hours and subjected to further experimental studies. Unstimulated cells serve as a control for further experiments. Quercetin and apocynin were used as inhibitors at a concentration of 20 μM and 100 μM respectively.21,22
2.5 Detection of intracellular superoxide anion radicals by flow cytometry and electron paramagnetic resonance spectroscopy
Retinoic acid differentiated neutrophils were centrifuged at 250g for 5 min and the cell suspension was prepared in 1× PBS. DHE-based detection of intracellular O2˙− was carried out using flow cytometry23 wherein RA-differentiated neutrophils were resuspended in 1× PBS and incubated at 37 °C with 5 μM DHE and respective stimulus for 30 min in the dark. Data were collected using an SP6800 spectral cell analyzer and analyzed using FlowJo software. 30 mM DHE stock solution was prepared in DMSO.
Cell permeable spin probe CPH was employed to study the O2˙− generation within stimulated neutrophils. As mentioned by,24 the samples were prepared with slight modifications as described below. 0.5 M CPH stock solution was prepared in 1× PBS. The stock solution was diluted to a working concentration of 0.5 mM in 1× PBS along with metal chelator DTPA (1 mM). PMA and LPS-stimulated cells were collected at 250g for 5 min and washed twice with PBS. Washed pellets were collected in 1× PBS containing 1 mM DTPA (pH 7.4) and subjected to centrifugation. The resulting pellet was resuspended in PBS + DTPA solution containing a 0.25 mM CPH probe. Samples were collected at regular time intervals and were kept at 37 °C. Sample tubes are gently mixed at regular intervals to avoid cell settlement at the bottom. ROS production in stimulated samples was analyzed in comparison to that in unstimulated samples. Apocynin and quercetin were employed as inhibitors to evaluate the NOX requirement for extracellular trap formation. The measurements were done using the following EPR parameters: 10 mW microwave power, 1 G modulation amplitude, 100 kHz modulation frequency, 100 G sweep width, and 1.62 G s−1 scan rate.
2.6 Detection of MDA protein adducts and inflammatory response by immunoblotting analysis
Based on the protocol outlined by Handzlik and co-workers, whole cell protein lysate for western blot analysis was prepared.25 Post-stimulation, samples were collected at two different intervals (1.5 h and 3 h). Samples were washed twice with 1× PBS to remove residual media and pellets were resuspended in Radio-Immunoprecipitation Assay (RIPA) buffer supplemented with protease inhibitor. Cell lysis was achieved by sonication and the whole cell lysate containing supernatant was collected by centrifugation at 12
000g for 20 min. Before SDS PAGE electrophoresis, sample concentrations was quantified by bicinchoninic acid (BCA) assay. An equal concentration of samples was prepared with 5× Laemeli buffer and boiled for 10 min at 70 °C. Followed by centrifugation, the protein supernatant was loaded onto 10% SDS-PAGE, separated and transferred onto a nitrocellulose membrane. 5% BSA prepared in 1× tris-buffered saline – Tween 20 (TBST) was used to block the membranes for 2 h at RT. Blocked membranes were incubated with primary antibodies [anti-MDA (1
:
5000)/anti-5-LOX (1
:
2000)/CD63 (1
:
5000)/histone H3.3 (1
:
2000)] overnight at 4 °C and subsequently with HRP conjugated anti-mouse or anti-rabbit secondary antibodies (1
:
10
000) for 1 h at RT. β-actin (1
:
1000) antibodies were used as a control to evaluate the expression of target genes. Densitometric analysis was performed using ImageJ software (National Institute of Health, Maryland, USA).
2.7 Evaluation of NOX2 and NOX4 expression by immunoblotting analysis
Western blot analysis was performed to study the difference in NOX2 and NOX4 protein expression along with neutrophil function. To achieve this, samples were prepared as mentioned above and membranes were incubated overnight using anti-NOX2 and anti-NOX4 antibodies at a dilution of 1
:
5000 in 5% BSA prepared in 1× TBST. Proteins were detected by goat anti-rabbit secondary antibody. Incubation with antibody for β-actin (1
:
5000) acted as an internal loading control and was detected by rabbit anti-mouse secondary antibodies (1
:
10
000). Quantitative analysis of the blots was performed using ImageJ software.
2.8 Statistical analysis
Quantitative data are expressed as mean ± standard deviations determined from duplicates and P values < 0.05 were considered statistically significant. Statistical analysis and data evaluation were performed using Multiplot (Magnettech GmbH – Berlin, Germany), GraphPad Prism and Origin Lab (version 8.5.1).
3 Results
3.1 ATRA-mediated differentiation of HL60 into neutrophil and stimulation for trap formation
Differentiation protocol varies between studies reported so far;26,27 thus the present study aims to determine an optimized differentiation protocol with enhanced neutrophil expression marker levels along with cell viability. 0.5 μM of ATRA was used to differentiate HL-60 cells into neutrophils and the differentiation efficacy was analyzed based on CD11b expression, a neutrophil surface marker. Expression of CD11b was observed to be evident between three to five days and started to decline at the end of the seventh day (Fig. 1A and B). Based on these results, an optimized differentiation condition of three days (72 h) was employed to achieve neutrophil-like cells for further experimentation. Additionally, the expression of the CD14 marker was evaluated and found to be negative post-differentiation with ATRA, which confirms the neutrophil-like characteristics of differentiated HL-60 cells (ESI 1†). Fig. 1C represents CD11b expression post-differentiation with RA measured on alternate days for a week. Expression of CD11b markers was retained post-stimulation for NETosis with PMA and LPS (Fig. 1D). Neutrophils were evaluated for their differentiation states, namely live, apoptotic and dead cells post differentiation with ATRA 72 h and stimulation with PMA and LPS for 3 h. The expression of CD63 and MPO (ESI 2†) confirms MPO derived ROS produced within neutrophils mediates lipid peroxidation and protein adduct formation.
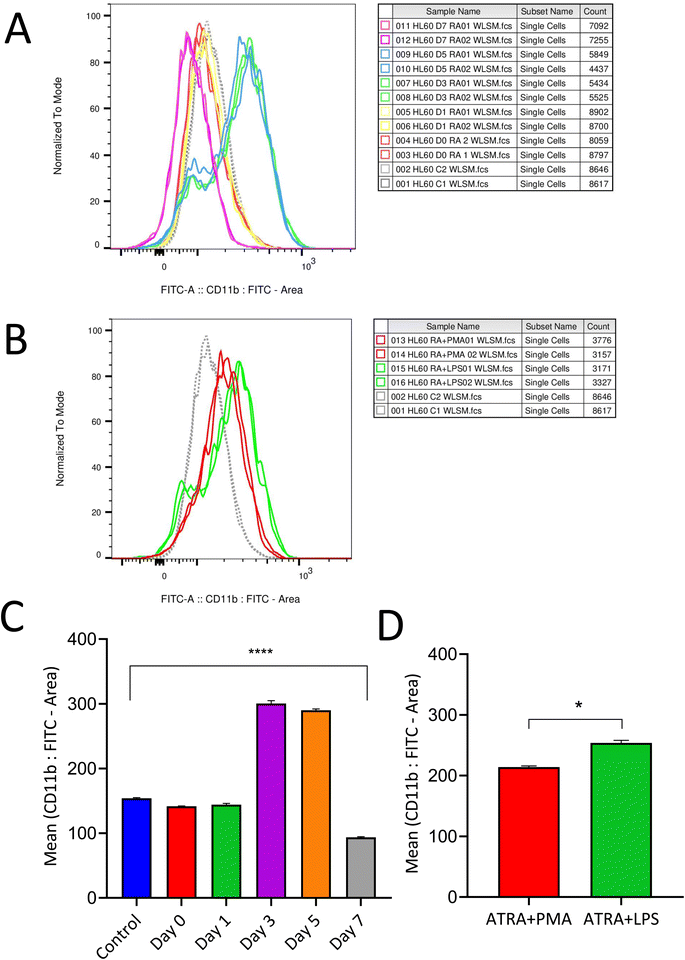 |
| Fig. 1 ATRA-mediated differentiation of HL-60 cells into neutrophil. Evaluation of CD11b expression (A) post differentiation with ATRA from days 0–7 followed by (B) stimulation with PMA and LPS for 3 h; (C) and (D) represent bar graphs with values expressed as mean ± SD calculated from two independent experiments; the results obtained were statistically significant (****P ≤ 0.0001). | |
3.2 Detection of intracellular ROS formation by flow cytometry and EPR
DHE in the presence of superoxide anion radical (O2˙−) undergoes further oxidation resulting in the formation of red fluorescent product 2-hydroxy-ethidium (2OH-Et+) with a distinct excitation wavelength. The generated product 2OH-Et+ has specific spectral properties with an excitation peak around 400 nm and emission peak at 580 nm which has been utilized to measure and quantify intracellular O2˙− generation in neutrophils using flow cytometry. From Fig. 2, it is evident stimulation of neutrophils with PMA (Fig. 2A) and LPS (Fig. 2B) induces O2˙− production. Measurements were carried out in triplicates and the mean fluorescence intensity for the experimental condition was presented in Fig. 2C.
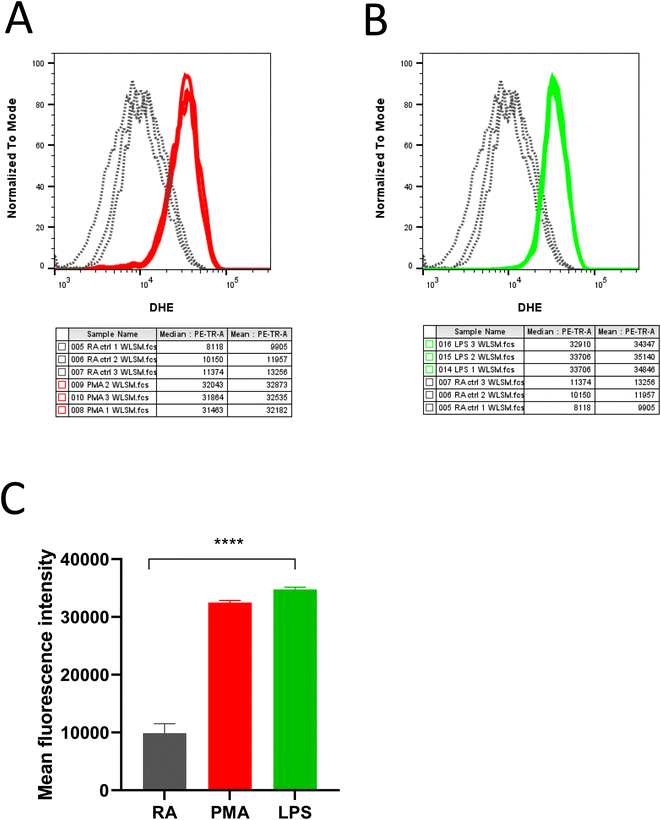 |
| Fig. 2 Intracellular O2˙− detection using flow cytometry. Utilization of DHE to detect O2˙− generation in neutrophils post-stimulation with (A) PMA and (B) LPS. Measurements were carried out in triplicates, (C) represent bar graphs with values expressed as mean ± SD calculated from three independent experiments; the results obtained were statistically significant (****P ≤ 0.0001). | |
The results were further validated wherein O2˙− formation in stimulated neutrophils was monitored using cyclic hydroxylamine spin probe CPH by EPR spectroscopy. Cyclic hydroxylamine spin probe reacts with O2˙− to form stable nitroxide radical. Inhibitors namely Apocynin (NOX inhibitor) and Quercetin (as an antioxidant) were added 30 min before stimulation to evaluate ROS role in neutrophil stimulation. Following collection and washes, cells were resuspended in PBS solution containing 0.5 mM CPH and 1 mM DTPA with a cell concentration ranging from 0.1–0.5 × 106 cells per mL. Differentiation of HL-60 with ATRA resulted in the generation of O2˙− which reacts with CPH, forming the stable nitroxide radical 3-carboxy-2,2,5,5-tetramethylpyrrolidine 1-oxyl (CP˙) (Fig. 3A). Post stimulation with PMA and LPS, a two-fold increase in the CP˙ was observed compared to unstimulated neutrophils. The efficacy of NETs formation correlates with the intracellular ROS production by NOX. Thus, the addition of Apocynin, a known inhibitor of NOX prior to stimulation reduced the adduct formation with both PMA and LPS-treated samples. Similarly, the addition of quercetin reduced CP˙ formation confirming its antioxidant activity (Fig. 3B and C). Results from this study underlie activating O2˙− generation aids in neutrophil differentiation and activation to form extracellular traps. Experiments were carried out in duplicates and a bar graph representing ± SD values was included as Fig. 3D.
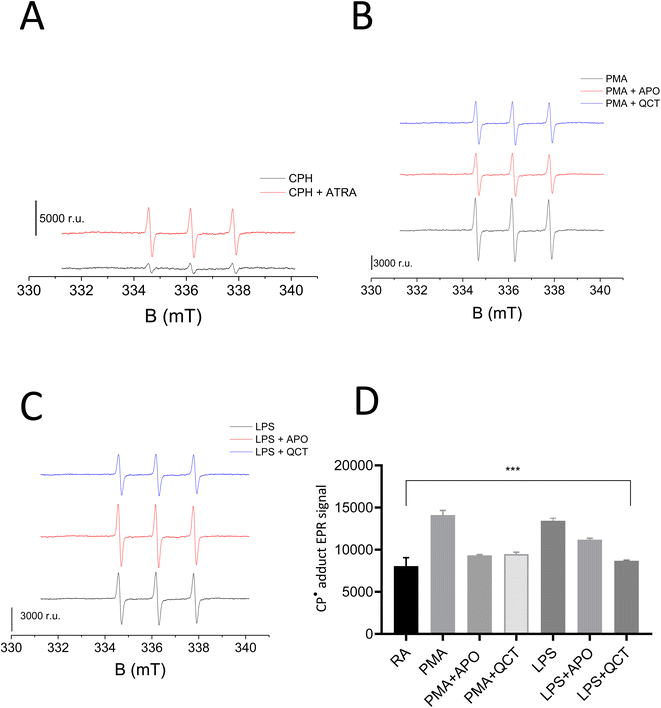 |
| Fig. 3 EPR spin trapping analysis of CP˙ formation in neutrophils. Detection of O2˙− generation in neutrophils stimulated with PMA and LPS in the presence and absence of inhibitors. EPR spectra were obtained in the presence of 0.25 mM CPH, 1 mM DTPA and 0.01 M PBS in (A) control (lower trace) and ATRA-differentiated HL-60 cells (upper trace), (B) PMA (lower trace), PMA + apocynin (middle trace) and PMA + quercetin (upper trace), (C) LPS (lower trace), LPS + apocynin (middle trace), LPS + quercetin (upper trace). (D) Bar chart representing values ± SD of EPR spectra measurements; the results obtained were statistically significant (***P ≤ 0.001) (n = 3). | |
3.3 Detection of lipid peroxidation-induced protein adducts formation during NETosis
Lipoxygenase (LOX) are involved in the oxidation of PUFA resulting in the production of O2˙− which mediates further signaling events involving neutrophil differentiation and activation. The expression of 5-LOX has been evaluated under treatment conditions. In comparison to control, PMA stimulation (Fig. 4A) enhances 5-LOX expression with a period of 1.5 h and gradually decreases at 3 h, whereas, with LPS, 5-LOX expression enhances and remains the same after 1.5 and 3 h (Fig. 4C). Quantification of protein band intensities was performed using ImageJ software and presented as a Densitogram as a right panel for both the blots (Fig. 4B and D). The expression of 5-LOX confirms its sequential activation in response to inflammatory stimuli underlying neutrophil function.
 |
| Fig. 4 Expression analysis of inflammatory mediators. Expression analysis of 5-lipoxygenase by western blot. 5-LOX expressions were analyzed post-stimulation with PMA (A) and LPS (C) in comparison to unstimulated differentiated control. Desnitogram analysis of PMA (B) and LPS (D) blots are presented as separate right panels. | |
Additionally, LOX5 expression confirms the role of lipid peroxidation events and thus protein adduct formation was evaluated. Malondialdehyde, a secondary by-product of lipid peroxidation, is a highly reactive aldehyde capable of covalently modifying proteins resulting in MDA–protein adduct formation. Detection of MDA–protein adducts formation along the NETosis process was carried out wherein post-treatment conditions whole cell homogenates were subjected to SDS-PAGE electrophoresis, blotted onto nitrocellulose membrane and probed with anti-MDA antibody. Three evident bands of 45 kDa, 15 kDa and 12 kDa (Fig. 5A) were observed with western blot analysis, as the anti-malondialdehyde antibody used in this present study specifically binds to protein targets undergone modification with MDA. Quantification of protein band intensities was performed using ImageJ software and presented as a densitogram (Fig. 5B). Since posttranslational modification of histones plays a pivotal role in the process of extracellular trap formation,28 the anti-MDA blots were stripped and probed with histone (H3) antibodies and expression of protein around 15 kDa were confirmed to be as histones (Fig. 5C). A significant decrease in MDA–protein adduct formation was observed in samples pretreated with quercetin underlying its anti-inflammatory properties. Proteins detected with anti-MDA blots have to be further characterized to identify targets and post-translational events they undergo along the process of extracellular trap formation. These identified protein targets can serve as biomarkers for studying NETosis and its associated inflammation.
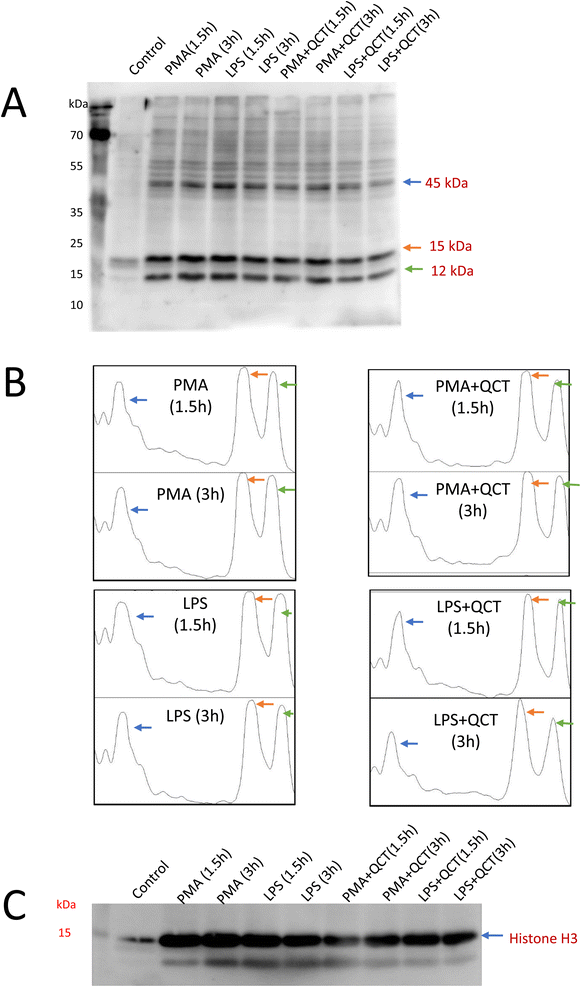 |
| Fig. 5 Aldehyde-derived protein adduct formation. Detection of malondialdehyde-modified proteins along neutrophil activation (A) in the presence of respective stimuli (PMA, LPS) and inhibitors (quercetin) using an anti-MDA antibody. (B) Densitogram analysis displaying the difference in band intensity of protein targets identified by the anti-MDA antibody. (C) Reprobing with anti-histone H3 antibody and identification of 15 kDa protein from MDA blot as histone. | |
3.4 NOX4-dependent extracellular trap formation and inhibitory studies
NADPH oxidases' generation of intracellular ROS regulates the extracellular trap formation by neutrophils. NOX-mediated intracellular ROS generation during neutrophil stimulation and the antioxidant potential of quercetin to inhibit NOX expression were evaluated. More specifically, the expression of NADPH oxidase 4 and 2 in response to PMA and LPS was considered. To achieve this, differentiated neutrophils were stimulated with PMA and LPS for a 3 h and expression was evaluated at intervals of 1.5 and 3 hours. Upon stimulation with PMA, NOX4 expression increased rapidly within 1.5 h and reduced with time. PMA is the non-physiological agent known to activate protein kinase C (PKC), producing ROS through NADPH oxidase. The addition of quercetin before PMA stimulation reduced NOX4 expression as evident from Fig. 6A. However, in the case of LPS stimulation, NOX-4 expression was enhanced with 3 h and reduced after treatment with quercetin (Fig. 7A). Quercetin is known to downregulate inflammatory factors through the TLR-NFκB signalling pathway, which can be correlated to its ability to regulate NOX expression. Densitometric quantification of NOX-4 expression was performed with β-actin as the control for both PMA and LPS-stimulated conditions (Fig. 6C and 7C). Concerning NOX2 expression, no evident time-dependent expression patterns were observed with both PMA (Fig. 6B) and LPS (Fig. 7B) stimulation and inhibitory effects of quercetin were evident. Quantification of NOX-2 expression was performed with β-actin as the control for both PMA and LPS-stimulated and presented as graphs (Fig. 6D and 7D).
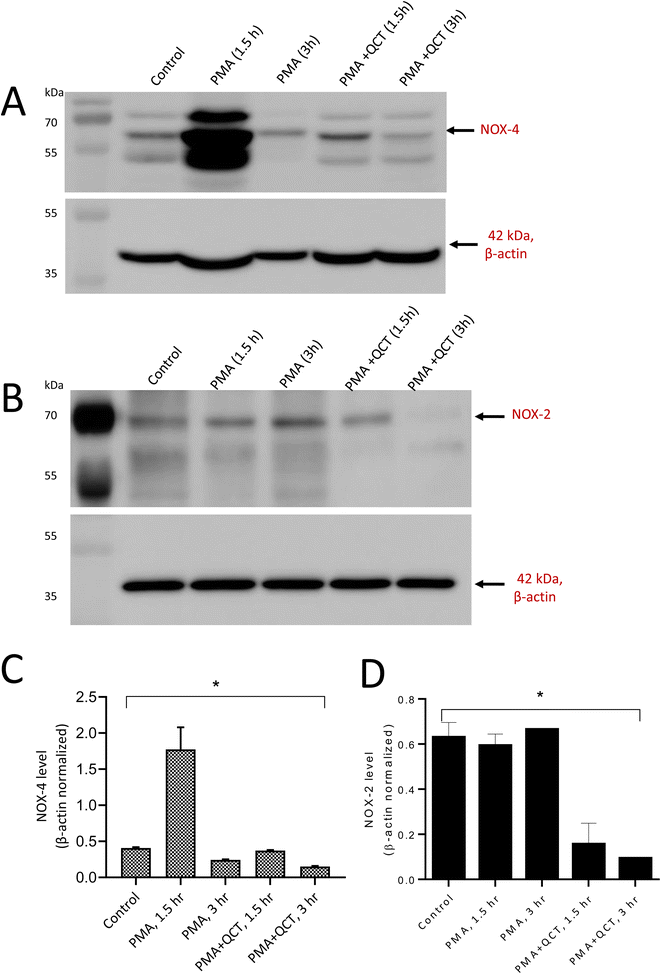 |
| Fig. 6 NOX4 expression in response to PMA stimulation. (A) NOX4 protein expression analysis post-stimulation with PMA in the absence and presence of quercetin. (B) NOX2 protein expression analysis post-stimulation with PMA in the absence and presence of quercetin. Quantification of protein bands from NOX4 (C) and NOX2 (D) blots with values normalized to β-actin as control. Values are expressed as mean ± SD calculated from two independent experiments; the results obtained were statistically significant (*P ≤ 0.05). | |
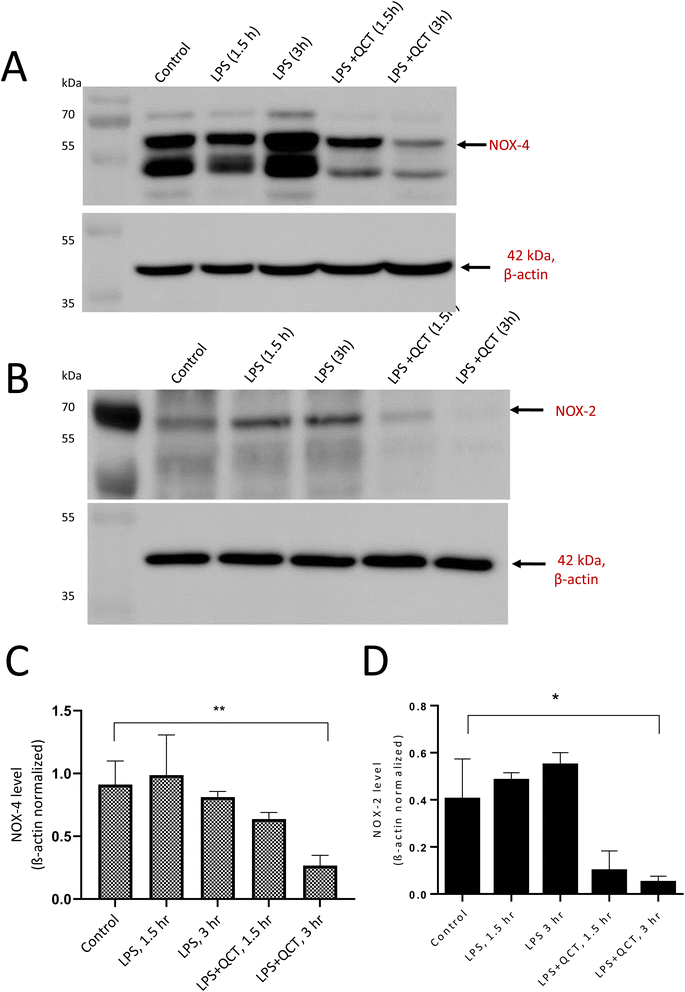 |
| Fig. 7 NOX4 expression in response to LPS stimulation (A). NOX4 protein expression analysis post-stimulation with LPS in the absence and presence of quercetin (B). NOX2 protein expression analysis post-stimulation with LPS in the absence and presence of quercetin. Quantification of protein bands from NOX4 (C) and NOX2 (D) blots with values normalized to β-actin as control. Values are expressed as mean ± SD calculated from two independent experiments; the results obtained were statistically significant (**P ≤ 0.01 and *P ≤ 0.05). | |
With NOX-4, the presence of multiple bands can be correlated to post-translational modification events. For example, four putative sites have been reported in the extracellular loop regions of NOX4 and additionally, recent reports support glycosylation of splice variants of NOX4. The amino acid sequence analysis of NOX4 reveals the presence of numerous PKC phosphorylation sites.27 As NOX4 are constitutively active and regulated in an inducible manner, post-translational events associated with NOX4 activities should be studied in detail.29
4 Discussion
Neutrophils are recognised as vital effector cells that play a key regulatory role in both innate and adaptive immune responses. Upon production in the bone marrow, these neutrophils enter the bloodstream and are recruited to the site of infection. Neutrophil effector functions are regulated through three main strategies: phagocytosis, degranulation, and the release of NETs. These extracellular traps are web-like structures comprising cytosolic and granular proteins assembled on decondensed chromatin. These traps can neutralise and kill pathogens however if dysregulated contribute to immune-related pathogenesis.30 Neutrophils express various inflammatory mediators, including cytokines, chemokines, and NETs that trigger inflammatory cascades.31 Several stimuli, including bacteria, viruses, cytokines, calcium ionophores, and PMA are known to induce NET formation. As the composition of NETs varies between stimuli, apart from protein targets such as NE, MPO, and histones, a list of proteins undergoing oxidation events must be considered. PMA and LPS have been used in this study to differentiate free radical-mediated oxidation events associated with suicidal and vital NETosis processes. MTT assays were performed and the concentrations of ATRA, PMA, and LPS were optimised using cell viability data (ESI 1†).
The present study uses ATRA (a vitamin A derivative) to differentiate HL-60 cells into neutrophils using receptor-dependent or receptor-independent pathways. ATRA preferentially binds to the retinoic acid receptor A (RARα), initiating multiple signaling mechanisms, namely MAPK and PI3K/Akt pathways resulting in cytoskeletal reorganizations necessary for differentiation.32 Differentiation conditions varied across the literature;33 thus, optimisation of differentiation conditions is highly conducive to achieving effective differentiation. The results of this study indicate that the incubation of HL-60 with ATRA for four days was the most suitable, justified by the expression of the neutrophil surface marker CD11b and cell viability. The absence of CD14 expression confirms the neutrophil state and avoids confusion regarding differentiation status into monocyte/macrophage. Neutrophils contain four different granules, namely azurophilic granules, specific granules, gelatinase granules, and secretory vesicles, to mediate antimicrobial mechanisms through degranulation events. Extracellular trap formation activates the exocytosis of granules which fuses with the plasma membrane upon activation, confirming the neutrophil activation.30 CD63 is present in azurophilic granules, and its expression enhances post-stimulation compared to unstimulated control neutrophils.
Research carried out so far tries to decipher the molecular mechanism associated with NET formation, and so far, identified numerous pathways and molecules involved in this process. However, another aspect of this process namely the oxidative stress-mediated inflammatory process remains unclear.34 In this study, we have demonstrated that ROS-induced neutrophil activation is carried out through O2˙− generation. We employed the use of redox redox-sensitive fluorescent probe, DHE with flow cytometry as a detection method. We further advanced our study by EPR measurements wherein hydroxylamine probes were employed to detect O2˙− generation in differentiated and stimulated neutrophils in the presence and absence of inhibitors. CPH probes readily react with O2˙− forming CP˙ radicals that form stable adducts as detected by EPR spectroscopy. Since NADPH oxidase activity is essential for the generation of O2˙− and in turn extracellular trap formation, cells were treated with Apocynin a known inhibitor of NOX. The addition of apocynin reduced the O2˙− generation in comparison to PMA and LPS-stimulated cells. Apocyanin served as a standard to evaluate the efficacy of quercetin in reducing free radical generation along the differentiation and stimulation process. Quercetin treatment reduced free radical generation similar to Apocynin. This confirms the antioxidant properties of quercetin and its ability to regulate the process of neutrophil-mediated inflammation.35
During inflammation along with local increase of vascular permeability, increased production of oxidants by neutrophils leads to either local or generalised oxidative stress conditions. Oxidants thus produced attacks on biomolecules including proteins and lipids. Under normal metabolic conditions, approximately 10
000 oxidative hits (per cell per day) were reported to take place and the count substantially increases under respiratory burst or during depletion of cellular antioxidants.36 Thus, induction of lipid peroxidation events and concomitant formation of lipid peroxidation products of PUFAs namely aldehydes play a significant role in the pathophysiology of various chronic and acute inflammatory diseases. These aldehydes are capable of forming stable adducts with proteins that are involved in the intracellular signaling events that can modulate pro-inflammatory and anti-inflammatory activities and are potential biomarkers of oxidative stress.37 ATRA-induced differentiation of phagocytes upregulates the expression of Toll-like receptors and NF-κB and additional stimulation with PMA or LPS results in enhanced expression of cytokines like TNF-α, and other co-stimulatory molecules contributing to the difference in the levels of protein MDA adduct formation between samples.38,39 With both PMA and LPS, the formation of MDA protein adduct formation was quite evident in comparison to unstimulated control. The difference can be attributed to an increase in free radical generation in neutrophils undergoing NETosis. Among the three evident bands noticed in anti-MDA blots, protein bands with a molecular weight of 15 kDa were identified as histone proteins with anti-H3 antibodies (ESI 2†). Post-translational modification of histones including citrullination, methylation and acetylation events are reported to play a critical role in regulating neutrophil function and NET-mediated diseased conditions.40 Recent studies carried out, demonstrated the biphasic effect of histone acetylation on NETosis via both NOX-dependent and independent manner.41 Histones are composed of mostly positively charged amino acid residues with abundant lysine and arginine residues. MDA pose a high affinity towards the formation of the adduct with lysine amino acids through the Schiff base reaction. The MDA-adduct formed binds to PKC facilitates kinase activation and stimulates the secretion of pro-inflammatory cytokines IL-6 and 8. The MDA thus formed may activate or silence various signalling pathways through oxidoreductases and hydrolases. While oxidoreductase influences NFκB activation and inflammatory cytokine expression, hydrolases mediate inflammatory signaling events through interleukin resulting in systemic inflammation and autoimmune diseases.42
Within a cellular environment, sources of intracellular ROS signaling include NOX, LOX, and mitochondria. Lipoxygenases (LOX) include six isoforms in humans and leukotrienes (LTs) serve as inflammatory mediators involved in chemotaxis and increased vascular permeability in phagocytes. 5-LOX is predominantly expressed in neutrophils and catalyses the production of leukotrienes and ROS from arachidonic acid. LOX is reported to be essential for intracellular ROS production mediated by pro-inflammatory stimuli including LPS.43 The results of this study demonstrate that the gradient increase in 5-LOX expression post-stimulation suggests that LOX-generated arachidonic acid (AA) metabolites namely leukotrienes (LT) induce ROS generation by stimulating NOX. Among the reported ROS, O2˙− is a key redox signaling molecule generated by NOX enzymes. These enzymes include seven isoforms with a wide tissue distribution and different activation mechanisms. NOX4-mediated ROS generation primarily depends on its interaction with p22phox and does not require depends on the cytosolic subunits and Rac. Thus, NOX4 is constitutively active and up-regulated during oxidative stress conditions. They control various cellular processes including cell proliferation, migration, and metabolic reprogramming in different physio-pathological processes in several tissues and cell types.44 PMA and LPS induced a time-dependent difference in NOX4 expression (ESI 3†) and are on par with the expression observed with CD63 and 5-LOX. This confirms the role of NOX4 in physiological innate immune defense through neutrophil extracellular trap formation. NOX-2 expression remained the same with both stimuli and time intervals (ESI 4†). β-Actin blots used for quantification were presented as ESI 5.†
Numerous studies have reported the antioxidant properties of flavonols, a subclass of flavonoids.45,46 The present study confirms quercetin's free radical scavenging ability through EPR spin trapping spectroscopy, and the results are on par with the reduction of NOX-4 expression in samples treated with quercetin prior to stimulation. Thus, quercetin inhibits oxidative stress by reducing the expression of NOX4. Quercetin is also reported to significantly reduce the inflammatory response through NF-κB translocation. Our study demonstrates O2˙− produced by NOX4-induced neutrophils to form extracellular traps. Quercetin reduces oxidative stress by preventing O2˙− generation which can be employed as a potential candidate for treating neutrophil-associated immunopathological conditions. Understanding the molecular mechanism associated with the regulation of NOX isoforms in cellular metabolism is highly essential as NOX becoming an attractive point of intervention for the pharmacological manipulation of ROS biology.
5 Conclusion
Neutrophils play a central role in host defence against pathogens and, in the past few years, the formation of neutrophil extracellular traps and its role in infection and inflammation remain elusive. This unique form of cell death depends on the generation of ROS wherein diffusion of reactive free radical species enables protein modification in activated neutrophils. Relatively few studies were carried out to interpret the role of free radical-induced protein oxidation in mediating neutrophil functions. Our study employed two different stimuli to analyse oxidative stress-mediated protein modification events and explored the role of NOX4 in neutrophil functions. A significant time-dependent expression difference was observed in NOX-4 compared to NOX-2 between stimuli underlying NOX-4 role in neutrophil function. Flow cytometry and EPR spectroscopy analysis reveal superoxide generation and Immuno-spin trapping technique underlie protein modification through covalent attachment of electrophilic aldehydes (MDA) produced during lipid peroxidation respectively. The anti-inflammatory effects of the flavonoid quercetin on neutrophil activation were confirmed by EPR and western blot analysis. From our preliminary study, three prominent protein targets that have undergone ROS-mediated oxidation were detected and a more detailed proteomic analysis is necessary to identify and study the post-translation modifications the protein has undergone. These proteins may serve as biomarkers for neutrophil activation during infection or inflammation and functional implications in neutrophil extracellular trap formation.
Abbreviations
ROS | Reactive oxygen species |
NETs | Neutrophil extracellular trap |
EPR spectroscopy | Electron paramagnetic resonance spectroscopy |
PMA | Phorbol 12-myristate 13-acetate |
ATRA | All-trans retinoic acid |
LPS | Lipopolysaccharides |
NOX 4 | NADPH oxidase 4 |
5-LOX | 5-Lipoxygenase |
Data availability
All data generated or analysed during this study are included in this published article [and its ESI files†].
Author contributions
Renuka Ramalingam Manoharan: conceptualization, data curation, formal analysis, methodology, validation, writing – original draft. Kateřina Zachová: methodology, data curation and validation. Marek Buzáš: methodology. Michal Křupka: editing the final version. Pavel Pospíšil: editing the final version. Ankush Prasad: conceptualization, data curation, formal analysis, methodology, project administration, validation, writing – original draft.
Conflicts of interest
The authors declare that they have no known competing financial interests or personal relationships that could have appeared to influence the work reported in this paper.
Acknowledgements
This work was funded by the European Regional Development Fund project “Plants as a tool for sustainable global development” (CZ.02.1.01/0.0/0.0/16_019/0000827) and grant no. IGA_PrF_2023_023 and IGA_LF_2024_013 of Palacký University. We would like to thank Dr Marek Rác, Department of Biophysics, Faculty of Science, Palacký University for his valuable discussion and technical assistance and Prof. Claudio Rossi, Department of Biotechnology, Chemistry and Pharmacy, University of Siena for providing antibodies.
References
- N. de Buhr and M. von Köckritz-Blickwede, How Neutrophil Extracellular Traps Become Visible, J. Immunol. Res., 2016, 2016, 4604713, DOI:10.1155/2016/4604713.
- E. Kolaczkowska and P. Kubes, Neutrophil recruitment and function in health and inflammation, Nat. Rev. Immunol., 2013, 13, 159–175, DOI:10.1038/nri3399.
- I. Neeli and M. Radic, Opposition between PKC isoforms regulates histone deimination and neutrophil extracellular chromatin release, Front. Immunol., 2013, 4, 38, DOI:10.3389/fimmu.2013.00038.
- C. Schauer, C. Janko, L. E. Munoz, Y. Zhao, D. Kienhöfer, B. Frey, M. Lell, B. Manger, J. Rech and E. Naschberger, et al., Aggregated neutrophil extracellular traps limit inflammation by degrading cytokines and chemokines, Nat. Med., 2014, 20, 511–517, DOI:10.1038/nm.3547.
- J. Clarke, NETs directly injure cartilage in RA, Nat. Rev. Rheumatol., 2020, 16, 410, DOI:10.1038/s41584-020-0459-4.
- M. Dwyer, Q. Shan, S. D'Ortona, R. Maurer, R. Mitchell, H. Olesen, S. Thiel, J. Huebner and M. Gadjeva, Cystic Fibrosis Sputum DNA Has NETosis Characteristics and Neutrophil Extracellular Trap Release Is Regulated by Macrophage Migration-Inhibitory Factor, J. Innate Immun., 2014, 6, 765–779, DOI:10.1159/000363242.
- H. Björnsdottir, A. Welin, E. Michaëlsson, V. Osla, S. Berg, K. Christenson, M. Sundqvist, C. Dahlgren, A. Karlsson and J. Bylund, Neutrophil NET formation is regulated from the inside by myeloperoxidase-processed reactive oxygen species, Free Radicals Biol. Med., 2015, 89, 1024–1035, DOI:10.1016/j.freeradbiomed.2015.10.398.
- N. V. Vorobjeva and B. V. Chernyak, NETosis: Molecular Mechanisms, Role in Physiology and Pathology, Biochemistry, 2020, 85, 1178–1190, DOI:10.1134/S0006297920100065.
- S. Masuda, D. Nakazawa, H. Shida, A. Miyoshi, Y. Kusunoki, U. Tomaru and A. Ishizu, NETosis markers: Quest for specific, objective, and quantitative markers, Clin. Chim. Acta, 2016, 459, 89–93, DOI:10.1016/j.cca.2016.05.029.
- J. Petroniene, I. Morkvenaite-Vilkonciene, R. Miksiunas, D. Bironaite, A. Ramanaviciene, K. Rucinskas and A. Ramanavicius, Scanning electrochemical microscopy for the investigation of redox potential of human myocardium-derived mesenchymal stem cells grown at 2D and 3D conditions, Electrochim. Acta, 2020, 360, 136956, DOI:10.1016/j.electacta.2020.136956.
- V. Brinkmann, U. Reichard, C. Goosmann, B. Fauler, Y. Uhlemann, D. S. Weiss, Y. Weinrauch and A. Zychlinsky, Neutrophil Extracellular Traps Kill Bacteria, Science, 2004, 303, 1532–1535, DOI:10.1126/science.1092385.
- W. Stoiber, A. Obermayer, P. Steinbacher and W.-D. Krautgartner, The Role of Reactive Oxygen Species (ROS) in the Formation of Extracellular Traps (ETs) in Humans, Biomolecules, 2015, 5, 702–723, DOI:10.3390/biom5020702.
- M. Ravindran, M. A. Khan and N. Palaniyar, Neutrophil Extracellular Trap Formation: Physiology, Pathology, and Pharmacology, Biomolecules, 2019, 9, 365, DOI:10.3390/biom9080365.
- R. P. Wilkie-Grantham, N. J. Magon, D. T. Harwood, A. J. Kettle, M. C. Vissers, C. C. Winterbourn and M. B. Hampton, Myeloperoxidase-dependent Lipid Peroxidation Promotes the Oxidative Modification of Cytosolic Proteins in Phagocytic Neutrophils, J. Biol. Chem., 2015, 290, 9896–9905, DOI:10.1074/jbc.M114.61342.
- O. Rådmark and B. Samuelsson, 5-Lipoxygenase: mechanisms of regulation, J. Lipid Res., 2009, 50, S40–S45, DOI:10.1194/jlr.R800062-JLR200.
- M. Blanter, M. Gouwy and S. Struyf, Studying Neutrophil Function in vitro: Cell Models and Environmental Factors, J. Inflammation Res., 2021, 14, 141–162, DOI:10.2147/JIR.S284941.
- L. Gibellini, M. Pinti, M. Nasi, S. De Biasi, E. Roat, L. Bertoncelli and A. Cossarizza, Interfering with ROS Metabolism in Cancer Cells: The Potential Role of Quercetin, Cancers, 2010, 2, 1288–1311, DOI:10.3390/cancers2021288.
- N. M. Hartung, J. Fischer, A. I. Ostermann, I. Willenberg, K. M. Rund, N. H. Schebb and U. Garscha, Impact of food polyphenols on oxylipin biosynthesis in human neutrophils, Biochim. Biophys. Acta, Mol. Cell Biol. Lipids, 2019, 1864, 1536–1544, DOI:10.1016/j.bbalip.2019.05.002.
- S. Hougee, A. Sanders, J. Faber, Y. M. F. Graus, W. B. Van Den Berg, J. Garssen, H. F. Smit and M. A. Hoijer, Decreased pro-inflammatory cytokine production by LPS-stimulated PBMC upon in vitro incubation with the flavonoids apigenin, luteolin or chrysin, due to selective elimination of monocytes/macrophages, Biochem. Pharmacol., 2005, 69, 241–248, DOI:10.1016/j.bcp.2004.10.002.
- M. Comalada, D. Camuesco, S. Sierra, I. Ballester, J. Xaus, J. Gálvez and A. Zarzuelo, In vivo quercitrin anti-inflammatory effect involves release of quercetin, which inhibits inflammation through down-regulation of the NF-κB pathway, Eur. J. Immunol., 2005, 35, 584–592, DOI:10.1002/eji.200425778.
- S. Bhaskar, V. Shalini and A. Helen, Quercetin regulates oxidized LDL induced inflammatory changes in human PBMCs by modulating the TLR-NF-κB signaling pathway, Immunobiology, 2011, 216, 367–373, DOI:10.1016/j.imbio.2010.07.011.
- D. Awasthi, S. Nagarkoti, A. Kumar, M. Dubey, A. K. Singh, P. Pathak, T. Chandra, M. K. Barthwal and M. Dikshit, Oxidized LDL induced extracellular trap formation in human neutrophils via TLR-PKC-IRAK-MAPK and NADPH-oxidase activation, Free Radicals Biol. Med., 2016, 93, 190–203, DOI:10.1016/j.freeradbiomed.2016.01.004.
- Y. Guo, F. Gao, Q. Wang, K. Wang, S. Pan, Z. Pan, S. Xu, L. Li and D. Zhao, Differentiation of HL-60 cells in serum-free hematopoietic cell media enhances the production of neutrophil extracellular traps, Exp. Ther. Med., 2021, 21, 353, DOI:10.3892/etm.2021.9784.
- A. A. Abubaker, D. Vara, I. Eggleston, I. Canobbio and G. Pula, A novel flow cytometry assay using dihydroethidium as redox-sensitive probe reveals NADPH oxidase-dependent generation of superoxide anion in human platelets exposed to amyloid peptide β, Platelets, 2019, 30(2), 181–189, DOI:10.1080/09537104.2017.1392497.
- O.-J. Sul and S. W. Ra, Quercetin Prevents LPS-Induced Oxidative Stress and Inflammation by Modulating NOX2/ROS/NF-kB in Lung Epithelial Cells, Molecules, 2021, 26, 6949, DOI:10.3390/molecules26226949.
- J. P. Gotham, R. Li, T. E. Tipple, J. R. Lancaster, T. Liu and Q. Li, Quantitation of spin probe-detectable oxidants in cells using electron paramagnetic resonance spectroscopy: To probe or to trap?, Free Radicals Biol. Med., 2020, 154, 84–94, DOI:10.1016/j.freeradbiomed.2020.04.020.
- A. Manda-Handzlik, W. Bystrzycka, M. Wachowska, S. Sieczkowska, A. Stelmaszczyk-Emmel, U. Demkow and O. Ciepiela, The influence of agents differentiating HL-60 cells toward granulocyte-like cells on their ability to release neutrophil extracellular traps, Immunol. Cell Biol., 2018, 96, 413–425, DOI:10.1111/imcb.12015.
- A. Prasad, R. R. Manoharan, M. Sedlářová and P. Pospíšil, Free Radical-Mediated Protein Radical Formation in Differentiating Monocytes, Int. J. Mater. Sci., 2021, 22, 9963, DOI:10.3390/ijms22189963.
- E. Rincón, B. L. Rocha-Gregg and S. R. Collins, A map of gene expression in neutrophil-like cell lines, BMC Genomics, 2018, 19, 573, DOI:10.1186/s12864-018-4957-6.
- D. Gupta, H. P. Shah, K. Malu, N. Berliner and P. Gaines, Differentiation and Characterization of Myeloid Cells, Curr. Protoc. Immunol., 2014, 104 DOI:10.1002/0471142735.im22f05s104.
- F. Chen, S. Haigh, S. Barman and D. J. R. Fulton, From form to function: the role of Nox4 in the cardiovascular system, Front. Physiol., 2012, 3, 412, DOI:10.3389/fphys.2012.00412.
- K. Yuan, Q. Zhu, Q. Lu, H. Jiang, M. Zhu, X. Li, G. Huang and A. Xu, Quercetin alleviates rheumatoid arthritis by inhibiting neutrophil inflammatory activities, J. Nutr. Biochem., 2020, 84, 108454, DOI:10.1016/j.jnutbio.2020.108454.
- V. Papayannopoulos, Neutrophil extracellular traps in immunity and disease, Nat. Rev. Immunol., 2018, 18, 134–147, DOI:10.1038/nri.2017.105.
- O. Di Martino and J. S. Welch, Retinoic Acid Receptors in Acute Myeloid Leukemia Therapy, Cancers, 2019, 11, 1915, DOI:10.3390/cancers11121915.
- A. Manda-Handzlik, W. Bystrzycka, A. Cieloch, E. Glodkowska-Mrowka, E. Jankowska-Steifer, E. Heropolitanska-Pliszka, A. Skrobot, A. Muchowicz, O. Ciepiela and M. Wachowska, et al., Nitric oxide and peroxynitrite trigger and enhance release of neutrophil extracellular traps, Cell. Mol. Life Sci., 2020, 77, 3059–3075, DOI:10.1007/s00018-019-03331-x.
- D. Dömer, T. Walther, S. Möller, M. Behnen and T. Laskay, Neutrophil Extracellular Traps Activate Proinflammatory Functions of Human Neutrophils, Front. Immunol., 2021, 12, 636954, DOI:10.3389/fimmu.2021.636954.
- E. Lorne, J. W. Zmijewski, X. Zhao, G. Liu, Y. Tsuruta, Y.-J. Park, H. Dupont and E. Abraham, Role of extracellular superoxide in neutrophil activation: interactions between xanthine oxidase and TLR4 induce proinflammatory cytokine production, Am. J. Physiol.: Cell Physiol., 2008, 294, C985–C993, DOI:10.1152/ajpcell.00454.2007.
- Y. Sasaki, Does oxidative stress participate in the development of hepatocellular carcinoma?, J. Gastroenterol., 2007, 41, 1135–1148, DOI:10.1007/s00535-006-1982-z.
- G. Li, C. Ye, Y. Zhu, T. Zhang, J. Gu, J. Pan, F. Wang, F. Wu, K. Huang and K. Xu, et al., Oxidative Injury in Ischemic Stroke: A Focus on NADPH Oxidase 4, Oxid. Med. Cell. Longevity, 2022, 1–12, DOI:10.1155/2022/1148874.
- R. R. Manoharan, M. Sedlářová, P. Pospíšil and A. Prasad, Detection and characterization of free oxygen radicals induced protein adduct formation in differentiating macrophages, Biochim. Biophys. Acta, Gen. Subj., 2023, 1867, 130324, DOI:10.1016/j.bbagen.2023.130324.
- P. Hamam, Post-Translational Modifications in NETosis and NETs-Mediated Diseases, Biomolecules, 2019, 9, 369, DOI:10.3390/biom9080369.
- H. J. Hamam and N. Palaniyar, Histone Deacetylase Inhibitors Dose-Dependently Switch Neutrophil Death from NETosis to Apoptosis, Biomolecules, 2019, 9, 184, DOI:10.3390/biom9050184.
- A. Wroński, A. Gęgotek and E. Skrzydlewska, Protein adducts with lipid peroxidation products in patients with psoriasis, Redox Biol., 2023, 63, 102729, DOI:10.1016/j.redox.2023.102729.
- S. Y. Kim, T.-B. Kim, K. Moon, T. J. Kim, D. Shin, Y. S. Cho, H.-B. Moon and K.-Y. Lee, Regulation of pro-inflammatory responses by lipoxygenases via intracellular reactive oxygen species in vitro and in vivo, Exp. Mol. Med., 2008, 40, 461, DOI:10.3858/emm.2008.40.4.461.
- D. Awasthi, S. Nagarkoti, S. Sadaf, T. Chandra, S. Kumar and M. Dikshit, Glycolysis dependent lactate formation in neutrophils: A metabolic link between NOX-dependent and independent NETosis, Biochim. Biophys. Acta, Mol. Basis Dis., 2019, 1865, 165542, DOI:10.1016/j.bbadis.2019.165542.
- A. Prasad, C. Rossi, R. R. Manoharan, M. Sedlářová, L. Cangeloni, D. Rathi, G. Tamasi, P. Pospíšil and M. Consumi, Bioactive Compounds and Their Impact on Protein Modification in Human Cells, Int. J. Mol. Sci., 2022, 23, 7424, DOI:10.3390/ijms23137424.
|
This journal is © The Royal Society of Chemistry 2024 |
Click here to see how this site uses Cookies. View our privacy policy here.