DOI:
10.1039/D4RA01021C
(Paper)
RSC Adv., 2024,
14, 14758-14766
Swelling behaviors of superabsorbent composites based on acrylic acid/acrylamide copolymer and acid-modified attapulgite
Received
9th February 2024
, Accepted 20th March 2024
First published on 7th May 2024
Abstract
The swelling behaviors and water retention of superabsorbent materials prepared from acrylic acid/acrylamide copolymer and acid-modified attapulgite under ultrasonic treatment and different pH conditions were investigated. The results demonstrated that a suitable amount of attapulgite can effectively improve the absorption capacity. The superabsorbent achieved the highest absorptions of 1257.54 g g−1 and 209.45 g g−1 in distilled water and a 0.9 wt% NaCl solution, and a higher water absorbency occurred over a wide pH range of 5–9 when the ultrasonic power was 200 W, and the attapulgite content was 10%. Addition of attapulgite could significantly increase the water absorption and retention of this kind of material, which can be applied as a sand-fixing material.
1. Introduction
Sand-fixing materials (SFMs) have been suggested as a solution for controlling desertification in arid and semiarid regions because they could influence the configuration and function of the desert surface by enhancing soil stability and increasing moisture retention. Superabsorbent polymers are always major components of SFMs since they can absorb and retain huge amounts of water or aqueous solution and are difficult to remove, even under high pressure or temperature.1,2 Superabsorbent composites have a three-dimensional network structure of crosslinked hydrophilic polymer. Because of their unique properties, such as ability to swell, insolubility and hydrophilicity, applications of superabsorbent materials have been expanded to diverse fields, such as agriculture, horticulture, and environmental protection. The preparation methods and swelling behaviors of superabsorbent composites have attracted considerable attention from many researchers;3–7 however, most superabsorbents are expensive and environmentally toxic. Hence, to reduce the cost of production and improve biocompatibility, renewable, eco-friendly, non-toxic, biodegradable, natural organic materials, such as starch, sodium alginate, chitosan, guar gum and inorganic clay minerals, are often introduced into the synthesis process of superabsorbent composites.8–12
Superabsorbent composites containing organic–inorganic minerals have received extensive attention. Attapulgite is a type of magnesium aluminum silicate clay mineral. A large number of hydrophilic Si–OH bonds are present on the surface of attapulgite, each of which could undergo a graft copolymerization reaction with a hydrophilic vinyl monomer, and graft copolymerization occurs between hydrophilic vinyl monomers. When attapulgite was added to resins, it improved the salt tolerance and absorbent properties of newly formed composite materials and also effectively reduced the production cost.8,13–18 The new material formed by copolymerization grafting of attapulgite with acrylamide monomers and 2-acrylamido-2-methylpropanesulfonic acid has strong anionic traits, salt resistance, and water absorption.19 In addition, it has saline alkali tolerance, and when added into high-water-absorbency polymer materials, it could not only improve the absorbency, salt tolerance and other properties of the materials but also effectively reduce the production cost, so it is suitable for preparing inorganic–organic composite superabsorbents.20–22
Considering the strong impact of attapulgite on the properties of corresponding superabsorbent composites, a composite superabsorbent was prepared by grafting hydrophilic monomer acrylic acid and acrylamide onto acid-modified attapulgite clay in aqueous solution. Potassium persulfate was used as the initiator and N,N-methylenebisacrylamide was used as a crosslinker. The acid-modified attapulgite was incorporated into the polyacrylamide polymeric network to investigate its effects in terms of the swelling behaviour, pH-sensitivity, salt-resistance properties and water retention of the superabsorbent composites. Increasing the proportion of attapulgite clay in the material preparation process is more conducive to bonding between the material and desert surface and helps to form a soil biocrust, achieving the goal of biological sand fixation. Sand-fixing materials synthesized with resins and attapulgite are explored under attapulgite addition and ultrasonic treatment.
2. Materials and methods
2.1. Materials
Attapulgite clay was obtained from Linze county of Gansu province, China. The main composition was as follows: SiO2, 63.47%; Al2O3, 18.01%; MgO4, 20%; Fe2O3, 5.16% and K2O, 3.64%. It contains mineral impurities, such as attapulgite, quartz, dolomite and montmorillonite (Fig. 1). Acrylic acid (AA), acrylamide (AM), potassium persulfate, N,N-methylenebisacrylamide (MBA), sodium hydroxide (NaOH) and sodium bisulphite were all of analytical grade. In electron microscope images, the rod-shaped structure of attapulgite is very obvious, and it is connected with other crystal minerals and amorphous silicates, consistent with the characteristics of clay minerals in terms of adhesion and cementation (Fig. 1).
 |
| Fig. 1 SEM image and XRD pattern of attapulgite. | |
2.2. Acid-modified treatment of attapulgite
First, 10 g of attapulgite clay was soaked in 4 mol L−1 H2SO4 solution at a temperature of 25 °C for 72 hours, and then, the material was washed with distilled water to a pH of 7 and placed in an oven at a temperature of 105 °C to dry. A certain amount of NaOH was added to distilled water in a beaker, and then, a certain amount of AA was slowly added to the beaker. During this process, the beaker was placed in an ice-water bath. The degree of neutralization was maintained at 80%. A predetermined amount of AM was added into the beaker with vigorous stirring to produce the monomer solution. Based on the performance the adsorbents were prepared by the addition of acrylic acid and acrylamide,7,12,18 where AA and AM were added according to the mass ratio 15.0
:
4.0. A variable amount of acid-modified attapulgite, and later, 30 mL of distilled water were added to a beaker with vigorous stirring to produce acid-modified attapulgite.
2.3. Synthesizing experiment
First, 5 g of NaOH was first dissolved in 10 mL of distilled water in a beaker. Then, 15 mL of AA was slowly added to the NaOH solution, and the resultant solution was neutralized in an ice-water bath with a neutralization degree of 80%. Further, 15 mL of AA and 4 g of AM were then added to the neutralized solution to prepare a monomer solution. Acid-modified attapulgite samples with different mass fractions of 0%, 3%, 5%, 8%, 10%, and 15% (denoted ATP0, ATP3, ATP5, ATP8, ATP10, and ATP15, respectively) were taken and dissolved in 30 mL of distilled water to prepare attapulgite colloids. In addition, the amount of N,N′-methylene bisacrylamide (acting as the crosslinker) was 0.05%, and the dosage of potassium persulfate and sodium bisulfite (molar ratio = 1
:
1; acting as the reaction initiator) was 0.6%. N′-methylene bisacrylamide, potassium persulfate, and sodium bisulfite were dissolved in 10 mL of distilled water to prepare an initiator-crosslinker solution (Table 1). The temperature of the water bath was set to 80 °C. Until the temperature reached the specified value, the attapulgite colloids and initiator-crosslinker solutions were added to the monomer solution. After the reaction, the obtained product was crushed and washed with aqueous ethanol, then dried at 90 °C in an oven and ground to 100 mesh to obtain the sand-fixing materials.
Table 1 Proportions (%) of prepared materials for the preparation of sand-fixing materials
Material |
ATP0 |
ATP3 |
ATP5 |
ATP8 |
ATP10 |
ATP15 |
Acid-modified attapulgite |
0% |
3.00% |
5.00% |
8.00% |
10.00% |
15.00% |
AA |
77.30% |
74.98% |
73.44% |
71.12% |
69.57% |
65.71% |
AM |
9.80% |
9.50% |
9.31% |
9.02% |
8.82% |
8.33% |
Potassium persulfate |
0.17% |
0.17% |
0.16% |
0.16% |
0.15% |
0.14% |
Sodium bisulfite |
0.43% |
0.42% |
0.41% |
0.40% |
0.39% |
0.37% |
NaOH |
12.25% |
11.88% |
11.64% |
11.27% |
11.03% |
10.41% |
N,N′-Methylene bisacrylamide |
0.05% |
0.05% |
0.05% |
0.05% |
0.05% |
0.04% |
2.4. Ultrasonic treatment
The beaker with the acid-modified attapulgite was put into an ultrasonic reaction device with a variable amount of ultrasonic power (200 W, 300 W, 400 W, or 500 W) and heated at 80 °C. The monomer solution and initiator-crosslinker solution were added into the beaker with stirring. Approximately 10 min later, the product was washed several times with water and alcohol (1
:
9 in volume) to remove any unreacted reactants. The product was dried in an oven at 90 °C until the weight of the product was constant. The product was crushed to a particle size of 100 mesh to obtain the sand-fixing materials under ultrasonic treatment.
2.5. Measurement of swelling properties
First, 0.5 g of dried sample was mixed with 800 mL of distilled water in a beaker. The water absorption rate (WAR) and saline absorption rate (SAR) were calculated with eqn (1) and (2): |
 | (1) |
|
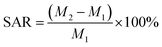 | (2) |
where M1 is the mass of the sand-fixing material before swelling (g), and M2 is the mass of the sand-fixing material after swelling (g).
Further, 0.5 g of sample was weighed and put into a container. The container was kept in a constant-temperature oven at 90 °C after saturation. The container was taken out of the oven at a certain time interval and weighed. The water retention rate (WRC) was calculated with eqn (3):
|
 | (3) |
where
W2 signifies the mass after dehydration (g), and
W1 denotes the mass after saturation with water (g). Then, 0.5 g of sample was further added to 500 mL of distilled water, and 0.1 M HCl or NaOH was used to adjust the initial pH value of the resultant solution. Five treatments of pH 6–10, were shaken at room temperature for 2 h until the sand-fixing materials reached equilibrium for swelling. The influence of pH change on water absorption rate was examined.
2.6. Statistical analysis
Two-way analysis of variance (ANOVA), followed by a multiple-comparison test (Duncan's test), was used to analyze differences among treatments; all treatments had six repetitions.
3. Results and discussion
3.1. Effect of attapulgite addition on water absorbency
The ratio of attapulgite to resins determined the absorbent performance when attapulgite was used as a comonomer for the synthesis of sand-fixing materials. The influence of attapulgite on water absorbency was investigated by varying the mass ratio from 3% to 15%. The addition of attapulgite obviously enhanced the water absorbency of the sand-fixing materials. The water absorbency increased with the addition of attapulgite. The addition of attapulgite could enhance the water absorbency, and when 10 wt% of attapulgite was added, the WAR for distilled water and saline absorption rate reached maxima of 955.36 g g−1 at 80 min and 91.47 g g−1 at 60 min, respectively (Fig. 2 and 3).
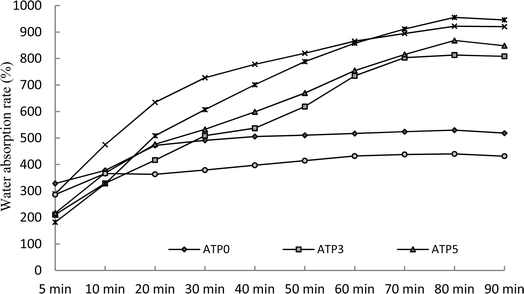 |
| Fig. 2 Water absorption rate of sand-fixing materials synthesized with polymer and acid-modified attapulgite for distilled water. | |
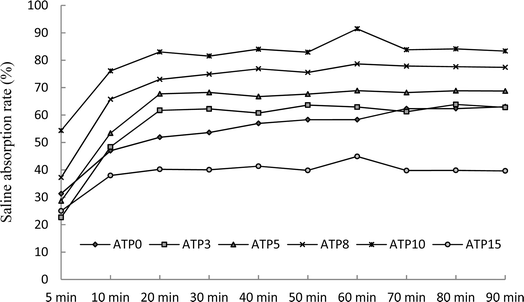 |
| Fig. 3 Saline absorption rate of the sand-fixing materials for salt solution (0.9 NaCl). | |
This result may be because attapulgite was easily dispersed into the polymetric gel and enhanced its hydrophilicity, and attapulgite participated in the construction of a three-dimensional hydrophilic network through its active –OH groups reacting and crosslinking with polymeric chains. Attapulgite nanoparticles can alleviate the entanglement of grafted polymer chains and weaken the hydrogen bonding interaction between –COOH groups, thereby reducing the degree of physical crosslinking and promoting the formation of a polymer network structure.23,24 With higher attapulgite addition (15%), water absorbency was remarkably reduced, because the attapulgite nanoparticles may become key crosslinking points in the polymers. Increasing the amount of attapulgite may cause a higher density of crosslinking and network space, making it difficult for water molecules to penetrate and gradually reducing water absorption. Conversely, an excessive amount of attapulgite may fill the physical space of the network, reduce the proportion of hydrophilic groups per unit volume, and lead to a decrease in the hydrophilicity of the composite material and cause a diminishment in water absorbency. This may be because attapulgite is combined with the polymer monomer via a chemical bond, and attapulgite serves as a crosslinking point in the resin, which is favorable for the formation of a porous three-dimensional network structure of the resin.21,24 The gelation reaction between the attapulgite nanoparticles and monomers changes the network structure of the polymer materials,13,30,36 further improving water absorption. The proportion was relatively low, or excess attapulgite only plays a role in filling the pores, which results in a decrease in the water absorption ratio.16,32
3.2. Effect of ultrasonic power on water absorbency
Two-way ANOVA showed that the WAR and SAR of the sand-fixing materials were significantly affected by ultrasonic treatment and the addition of attapulgite and their interaction (Table 2). The WAR was significantly different among the five ultrasonic treatments for all five sand-fixing materials with added attapulgite. Attapulgite addition significantly promoted the increasing WAR of the sand-fixing materials. The lowest WAR always appeared in ATP0 and ATP3 for all the ultrasonic treatments, which suggested that the lower dosages of attapulgite had no significant influence on the WAR of the SRA. Under 200 W of the ultrasonic treatment, ATP10 and ATP15 performed with larger WAR compared with other sand-fixing materials, and 300 W of the ultrasonic treatment could significantly improve the WAR of ATP10: the WAR of ATP10 reached a maximum value of 1168.55 g g−1 in distilled water (Table 3). The SAR was performed with almost coincident responses under the five ultrasonic treatments and different attapulgite additions. The SAR of the sand-fixing materials increased with the dosage of added attapulgite. A lower dosage (3%) of attapulgite had no significant influence on the SAR under all the ultrasonic treatments. ATP10 and ATP15 always showed a larger SAR compared to the other sand-fixing materials, and ATP10 performed with the largest SAR, reaching 212.60 g g−1 in saline solution (Table 4).
Table 2 Analysis of variance for the effects of attapulgite addition and ultrasonic power, attapulgite addition and solution pH on water absorption rate, saline absorption rate and water retention capacity of the sand-fixing materials
Traits |
Source of variation |
df |
F-value |
P |
Water absorption rate |
Attapulgite |
5 |
45.68 |
<0.001 |
Ultrasonic power |
4 |
11.24 |
<0.01 |
Attapulgite × ultrasonic power |
20 |
235.49 |
<0.001 |
Attapulgite |
5 |
75.64 |
<0.001 |
pH |
4 |
6.27 |
<0.05 |
Attapulgite × pH |
20 |
164.78 |
<0.001 |
Saline absorption rate |
Attapulgite |
5 |
36.54 |
<0.001 |
Ultrasonic power |
4 |
21.34 |
<0.001 |
Attapulgite × ultrasonic power |
20 |
86.27 |
<0.001 |
Water retention capacity |
Attapulgite |
5 |
11.25 |
<0.01 |
Ultrasonic power |
4 |
8.26 |
<0.01 |
Attapulgite × ultrasonic power |
20 |
65.24 |
<0.001 |
Table 3 Water absorption rate of the sand-fixing materials under different ultrasonic treatments at 60 min. Values with same lowercase letters were not significantly different among the ultrasonic treatments (column) and sand-fixing materials with addition of different contents of attapulgite (row) at P < 0.05 or according to Duncan's multiple comparison testsa a* at 0.05 level, ** at 0.01 level, *** at 0.001 level for significant difference by ANOVA
Ultrasonic |
ATP0 |
ATP3 |
ATP5 |
ATP8 |
ATP10 |
ATP15 |
F-value |
CK |
508.55E |
606.99bD |
849.89C |
903.34B |
989.77bA |
849.89bC |
14.16** |
200 W |
481.88D |
621.01aC |
1005.81B |
1012.56B |
1119.98bA |
1005.81aB |
65.37*** |
300 W |
501.75D |
586.93bC |
962.98B |
1092.77A |
1168.55aA |
971.23aB |
36.57*** |
400 W |
494.12D |
558.73bD |
900.04C |
1014.10B |
1107.71bA |
904.34bC |
24.38** |
500 W |
463.67D |
563.85bC |
856.61B |
942.10A |
996.45bA |
843.67bB |
12.39** |
F-value |
3.65 |
8.67* |
4.36 |
4.52 |
9.41* |
12.34* |
|
Table 4 Saline absorption rate of the sand-fixing materials under different ultrasonic treatments at 60 min. Values with the same lowercase letters were not significantly different among the ultrasonic treatments (column) and sand-fixing materials with addition of different contents of attapulgite (row) at P < 0.05 or according to Duncan's multiple comparison testsa a* at 0.05 level, ** at 0.01 level, *** at 0.001 level for significant difference by ANOVA
Ultrasonic |
ATP0 |
ATP3 |
ATP5 |
ATP8 |
ATP10 |
ATP15 |
F-value |
CK |
54.35C |
76.1cB |
83.03cA |
81.53cA |
83.34dA |
81.47cA |
16.54** |
200 W |
54.83C |
85.93bB |
184.79aA |
194.18aA |
209.45aA |
206.36aA |
46.58*** |
300 W |
65.11C |
98.53bB |
194.56aA |
198.23aA |
212.60aA |
200.19aA |
35.46** |
400 W |
58.11C |
113.85aB |
151.66bA |
166.43bA |
171.33bA |
162.83bA |
13.68* |
500 W |
68.47C |
93.15bB |
134.01bAB |
153.27bA |
158.95cA |
152.04bA |
26.54** |
F-value |
4.29 |
8.54* |
16.87** |
11.34* |
32.46** |
28.64** |
|
The results could be explained on the basis that when the power and reaction rate were low. The lower water absorbency of the superabsorbent composites could not been responded the reaction completely. With increasing ultrasonic power, the formation of free radicals would be easier. When the ultrasonic power increased to 300 W, the number of active radicals distinctly increased, leading to the growth of long chains in the grafted polymer and formation of a regular three-dimensional network, thereby improving the water absorption of the composite material. When the ultrasonic power is greater than 300 W, the water absorption of the composite material does not further improve (Tables 3 and 4). Excessive ultrasonic power may lead to self-crosslinking between the polymer and attapulgite nanoparticles, thereby enhancing the degree of crosslinking. During this process, a large number of free radicals may terminate the growth of grafting chains in the three-dimensional network, which may be another reason for the loss of water absorption by the composite material under conditions of higher ultrasonic power. In addition, high-power ultrasound treatment can easily cause liquid splashing, making the reaction difficult to control. Therefore, this study chose 300 W as the optimal ultrasound power.
3.3. Effect of solution pH on water absorbency
The sensitivity of the sand-fixing materials to pH value was explored by investigating the WAR for various pH values ranging from 5.0 to 9.0 in water. Two-way ANOVA showed that water absorbency showed significant differences among the five pH treatments of water for ATP3, ATP10 and ATP15. The WAR for all water with five pH values was performed with significant differences among the six sand-fixing materials. Attapulgite addition significantly enhanced the increase in the WAR of the sand-fixing materials. The lowest WAR always appeared in ATP0 and ATP3, showing that higher dosages of attapulgite had a significant enhancement effect on the WAR of the sand-fixing materials for water with various pH values. ATP10 always performed with the largest WAR compared to the other sand-fixing materials and arrived at a maximum value of 1114.46 g g−1 in water with pH 6 (Table 5). The water absorbency of the six sand-fixing materials increased in the pH range for water of 5–6 and decreases in the pH range of 7–9. According to previous reports, repulsive electrostatic interaction and hydrogen bonding have been recognized as the main reasons for water absorbency at various pH values of water.
Table 5 Water absorption rate of the sand-fixing materials for water in different pH at 60 min. Values with same lowercase letters were not significantly different among pH values of water (column) and sand-fixing materials with addition of different contents of attapulgite (row) at P < 0.05 or according to Duncan's multiple comparison testsa
pH |
ATP0 |
ATP3 |
ATP5 |
ATP8 |
ATP10 |
ATP15 |
F-value |
* at 0.05 level, ** at 0.01 level, *** at 0.001 level for significant difference by ANOVA. |
5 |
427.3C |
505.24aC |
870.48B |
914.82abA |
966.64bA |
938.26abA |
45.68*** |
6 |
432.86D |
527.22aC |
908.84B |
931.54aAB |
1114.46aA |
966.64aA |
123.56*** |
7 |
439.26D |
536.26aC |
926.36B |
957.64aB |
1074.40aA |
990.62aB |
56.87*** |
8 |
465.12D |
523.94aC |
906.90B |
937.06aB |
1025.66aA |
985.3aAB |
231.45*** |
9 |
414.2D |
435.16bC |
846.08B |
866.74bB |
971.4bA |
886.10bB |
65.32*** |
F-value |
3.48 |
6.87* |
4.12 |
3.66 |
12.45* |
8.54* |
|
Under acidic conditions, most carboxylate anions are protonated, which limits the electrostatic repulsion between atoms in the composite material, enhances the hydrogen bonding between polymer chains, and leads to a contraction of the three-dimensional network structure in the composite material, thereby reducing water absorption. As the external pH value increases (5 < pH < 6), the ionization level of grafted chains in the composite material increases, leading to disruption of hydrogen bonding interactions, an increase in osmotic expansion pressure between the hydrophilic network structure and external solution, and an increase in electrostatic repulsion energy between polymer –COO functional groups. The three-dimensional network structure space of the composite sand-fixing material may expand and transform into neutrality, thereby enhancing water absorption. This behavior is very beneficial for the stability of superabsorbers in various agricultural water conservation applications and desert surfaces. However, in alkaline water (pH > 8), excess sodium cations shield the complexation of –COO functional groups through charge shielding, inhibiting effective anion repulsion and reducing the water absorption of the composite material.25,26 Repulsive electrostatic interaction and hydrogen bonding have been considered the main causes affecting the water absorption of the materials under various environmental conditions.34 At higher pH, hydrogen bonding interactions are eliminated, electrostatic repulsion between anionic groups increases, and the polymer network structure of the composite material tends to have a higher expansion rate. Due to the charge shielding effect of counter ions (Na+), the swelling rate in alkaline solution decreases. In other hydrogel systems, the dependence of the swelling properties of similar composites on pH has also been reported.25 As the concentration of salt solution increases, the swelling ability significantly decreases. This well-known phenomenon is usually attributed to the “shared shielding effect” of additional cations, which leads to a decrease in anion–anion electrostatic repulsion, leading to a decrease in osmotic pressure (ion pressure) difference between the hydrogel network structure and external solution.13,37
3.4. Effect of attapulgite addition and ultrasonic power on water retention
WRC is an important indicator for the sand-fixing materials in view of practical applications. In this research, the sand-fixing materials prepared with different dosages of attapulgite under various ultrasonic treatments were evaluated with the WRC. It was remarkable that ATP8 and ATP10 under 200 W and 300 W of the ultrasonic treatment performed with an outstanding WRC compared to other SRA. The WRC of ATP10 was significantly higher than those of other sand-fixing materials, and no (ATP0) and lower addition of attapulgite (ATP3) always restrained the lowest WRC (Table 6). This could give a result where the water retention was improved upon the incorporation of attapulgite. Moreover, the swollen water in the composite materials can be divided into bound water, half-bound water, and free water. Compared with bound water and half-bound water, free water has higher transferability and is easily lost under ultrasonic treatment.27–29 Ultrasonic treatment can effectively improve the stability and water absorption of the composite materials.30 Adding a certain amount of attapulgite to the composite material increases the number of hydrophilic functional groups, such as –COOH and –COOH, resulting in a higher percentage of bound and half-bound water in the composite superabsorbent than free water, which significantly enhances the water retention ability of the formed polymer.31–35 Higher ultrasonic power (400 W and 500 W) could cause liquid splashes. Therefore, ultrasonic power of 300 W was chosen as the optimum treatment. The WRC of all SRA was always performed with a downward trend over time, and ATP8 and ATP10 presented better water retaining capacity (Fig. 4). Ultrasonication has a strong stirring effect on the liquid, where the localized high-temperature and high-pressure microenvironment that was formed via cavitation could lead to breaking and decomposition of polymer chains, and free radicals have an important effect on the solution polymerization.30 However, the excessive cavitation that was generated by the excessive ultrasonic power would cause polymer chain breakage and hinder the formation of the three-dimensional network structure. In addition, high cavitation produced high temperatures and led to an increase in the reaction speed. The initiation of burst polymerization led to an increase in the number of oligomers, which increased the number of soluble components of the resin, namely, the number of short polymer chains.27,28
Table 6 Water retention capacity of the sand-fixing materials under the different ultrasonic treatments at 24 h. Values with same lowercase letters were not significantly different among the ultrasonic treatments (column) and among addition of different contents of attapulgite (row) at P < 0.05 or according to Duncan's multiple comparison testsa
Ultrasonic |
ATP0 |
ATP3 |
ATP5 |
ATP8 |
ATP10 |
ATP15 |
F-value |
* at 0.05 level, ** at 0.01 level, *** at 0.001 level for significant difference by ANOVA. |
CK |
11.83B |
13.14bB |
19.4b |
17.48bA |
20.56cA |
18.81bA |
5.66* |
200 W |
14.63C |
25.96aB |
27.55aB |
36.45aA |
40.87aA |
29.70aAB |
36.52*** |
300 W |
15.66C |
28.51aB |
29.84aB |
32.58aAB |
40.08aA |
28.78aB |
29.64*** |
400 W |
12.84C |
20.04bB |
19.3bB |
22.56abB |
30.38bA |
17.45bB |
30.26*** |
500 W |
11.54C |
16.14bBC |
19.43bB |
19.48bB |
29.68bA |
13.58cC |
18.26** |
F-value |
3.61 |
19.58** |
7.47** |
28.66*** |
17.38** |
9.68** |
|
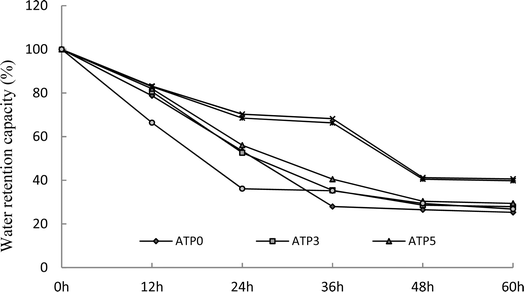 |
| Fig. 4 Water retention capacity (WRC) of SRA. | |
4. Conclusions
The composite superabsorbent yielded the best absorption when the attapulgite content was 10% (mass fraction). Attapulgite clay has a significant effect on the water absorption rate and water retention when it is used as a framework structure matrix for preparing water absorbing materials. As the content of acid-modified attapulgite clay increased from 5% to 10%, the water absorption rate increased. When the content of attapulgite was too large, the increase in the number of crosslinking points led to an increase in the degree of crosslinking of the resin material and a decrease in the three-dimensional network structure. Excessive crosslinking agent leads to the formation of additional crosslinking points in the composite material, resulting in an additional adhesive network structure, thereby reducing the amount of space available for water to enter the composite material.
pH conditions had no significant effect on the water absorption of composite superabsorbers, and composite materials exhibit higher swelling ratios over a wider pH range. Due to the “charge shielding effect” of counter ions (Na+), the swelling capacity in alkaline solutions of composite materials shows a decreasing trend.
The composite superabsorbent exhibited the highest absorption when the ultrasonic power was 200 W and 300 W. Attapulgite became more uniform in the monomer solution because of the ultrasonication. Ultrasonication facilitated the reaction of attapulgite and polymerized monomers and acted as an initiator. The combined effect caused the composites that were prepared under ultrasonic action to have higher water-absorption and brine-absorption rates than those that were prepared via conventional methods. High cavitation produced high temperatures and led to an increase in the reaction speed. The water absorption capacity of the polymer material increased with ultrasonic power.
Author contributions
Hanru Ren: visualization, methodology, resources, supervision, data processing. Ling Tao: conceptualization, investigation, data processing, formal analysis, visualization, supervision, funding acquisition. Jun Ren: methodology, resources, formal analysis, laboratory analyses. Xuechang Ren: conceptualization, investigation.
Conflicts of interest
The authors declare that they have no known competing financial interests or personal relationships that could have appeared to influence the work reported in this paper.
Acknowledgements
This research was supported by the National Natural Science Foundation of China (No. 31560234).
References
- T. Chock, A. J. Antoninka, A. M. Faist, M. A. Bowker, J. Belnap and N. N. Barger, Responses of biological soil crusts to rehabilitation strategies, J. Arid Environ., 2019, 163, 77–85 CrossRef.
- C. H. Park, X. R. Li, R. L. Jia and J. S. Hur, Combined application of cyanobacteria with soil fixing chemicals for rapid induction of biological soil crust formation, Arid Land Res. Manage., 2017, 31(1), 81–93 CrossRef CAS.
- X. H. Qi, M. Z. Liu, F. Zhang, Z. B. Chen, F. Zhang and L. Zhao, Preparation and properties of macroporous superabsorbent composite, Polym. Adv. Technol., 2010, 21(3), 196–204 CrossRef CAS.
- G. B. Marand, G. R. Mahdavinia and S. H. Ghafary, Swelling behavior of novel protein-based superabsorbent nanocomposite, J. Appl. Polym. Sci., 2011, 120, 1170–1179 CrossRef.
- S. K. Patra and S. K. Swain, Swelling Study of Superabsorbent PAA-co-PAM/Clay nanohydrogel, J. Appl. Polym. Sci., 2011, 120, 1533–1538 CrossRef CAS.
- X. X. Su, Y. Y. Li, Z. J. Li, M. J. Li, Y. Zhao and M. D. Hao, Preparation and characterization of XG-g-P(AA-co-AM)/AC composite super absorbent resin, Chem. Ind. Eng. Prog., 2011, 30(7), 1556–1561 CAS.
- X. N. Shi, W. Wang, Y. Kang and A. Wang, Enhanced swelling properties of a novel sodium alginate-based superabsorbent composites: NaAlg-g-poly (NaA-co-St)/PT, J. Appl. Polym. Sci., 2012, 125(3), 1822–1832 CrossRef CAS.
- W. B. Wang and A. Q. Wang, Preparation, characterization and properties of superabsorbent nanocomposites based on natural guar gum and modified rectorite, Carbohydr. Polym., 2009, 77(4), 891–897 CrossRef CAS.
- W. B. Wang and A. Q. Wang, Synthesis and swelling properties of guar gum-g-poly (sodium acrylate)/Na-montmorillonite superabsorbent nanocomposite, J. Compos. Mater., 2009, 43(23), 2805–2819 CrossRef CAS.
- A. Li, J. P. Zhang and A. Q. Wang, Utilization of starch and clay for the preparation of superabsorbent composite, Bioresour. Technol., 2007, 98, 327–332 CrossRef CAS PubMed.
- H. X. Yang, W. B. Wang and A. Q. Wang, A pH-sensitive biopolymer-based superabsorbent nanocomposite from sodium alginate and attapulgite: synthesis, characterization, and swelling behaviors, J. Dispersion Sci. Technol., 2012, 33, 1154–1162 CrossRef CAS.
- X. H. Qi, M. Z. Liu, F. Zhang and Z. B. Chen, Synthesis and properties of poly(sodium acrylateco-2-acryloylamino-2-methyl-1-propanesulfonic acid)/Attapulgite as a salt-resistant superabsorbent composite, Polym. Eng. Sci., 2009, 10, 182–188 CrossRef.
- J. F. Mukerabigwi, S. J. Lei, L. Fan, H. L. Wang, S. Y. Luo, X. Y. Ma, J. Qin, J. Q. Huang and Y. Cao, Eco-friendly nano-hybrid superabsorbent composite from hydroxyethyl cellulose and diatomite, R. Soc. Chem., 2016, 6, 31607–31618 CAS.
- W. B. Wang, Y. Zheng and A. Q. Wang, Syntheses and properties of superabsorbent composites based on natural guar gum and attapulgite, Polym. Adv. Technol., 2008, 19(12), 1852–1859 CrossRef CAS.
- A. Li, Y. G. Zhao and A. Q. Wang, Study on superabsorbent composite. XII. effect of ion-exchanged attapulgite on water absorbency of poly(acrylic acid)/attapulgite superabsorbent composites, J. Appl. Polym. Sci., 2007, 105, 3476–3482 CrossRef CAS.
- A. Li, A. Q. Wang and J. M. Chen, Studies on poly(acrylic acid)/attapulgite superabsorbent composites. II. swelling behaviors of superabsorbent composites in saline solutions and hydrophilic solvent-water mixtures, J. Appl. Polym. Sci., 2004, 94(5), 1869–1876 CrossRef CAS.
- A. Li, A. Q. Wang and J. M. Chen, Studies on poly(acrylic acid)/attapulgite superabsorbent composite. I. synthesis and characterization, J. Appl. Polym. Sci., 2004, 92(4), 1596–1603 CrossRef CAS.
- S. K. Swain, B. B. Shur and S. K. Patra, Poly(acrylamide-co-vinyl alcohol)-superabsorbent materials reinforced by modified clay, Polym. Compos., 2013, 10, 1794–1800 CrossRef.
- E. R. Kenawy, H. H. Elnaby and M. M. Azaam, Synthesis of superabsorbent composite based on chitosan-g-poly (acrylamide)/attapulgite, Polym. Bull., 2024, 81, 3527–3543 CrossRef CAS.
- Y. Liu, H. Chen, J. P. Zhang and A. Q. Wang, Effect of number of grindings of attapulgite on enhanced swelling properties of the superabsorbent nanocomposites, J. Compos. Mater., 2012, 47(8), 969–978 CrossRef.
- X. N. Shi, W. B. Wang and A. Q. Wang, Effect of surfactant on porosity and swelling behaviors of guar gum-g-poly(sodium acrylate-co-styrene)/attapulgite superabsorbent hydrogels, Colloids Surf., B, 2011, 88, 279–286 CrossRef CAS PubMed.
- X. Y. Zhang, X. P. Wang, L. Li, S. S. Zhang and R. N. Wu, Preparation and swelling behaviors of a high temperature resistant superabsorbent using tetraallylammonium chloride as crosslinking agent, React. Funct. Polym., 2015, 87, 15–21 CrossRef CAS.
- L. Q. Peng, L. C. Zhou, Y. F. Li, F. Pan and S. D. Zhang, Synthesis and properties of waterborne polyurethane/attapulgite nanocomposites, Compos. Sci. Technol., 2011, 71, 1280–1285 CrossRef CAS.
- J. K. An, W. B. Wang and A. Q. Wang, Preparation and swelling behavior of a pH-responsive psyllium-g-poly(acrylic acid)/attapulgite superabsorbent nanocomposite, Int. J. Polym. Mater., 2012, 61, 906–918 CrossRef CAS.
- W. B. Wang and A. Q. Wang, Nanocomposite of carboxymethyl cellulose and attapulgite as a novel pH-sensitive superabsorbent: synthesis, characterization and properties, Carbohydr. Polym., 2010, 82, 83–91 CrossRef CAS.
- S. L. Shi, H. R. Zhang, C. Huang, X. Q. Lin and X. D. Chen, Purification of lignocellulose hydrolysate by org-attapulgite/(divinyl benzene-styrene-methyl acrylate) composite adsorbent, BioResources, 2016, 11(4), 8664–8675 CAS.
- J. H. Xu, T. Y. Gan, C. S. Peng and Z. Li, Synthesis and swelling behaviours of APT-gPAMPS superabsorbent composites by microwave irradiation, Mater. Res. Innovations, 2014, 18, 377–381 CAS.
- J. H. Xu, D. X. Tan, Z. Li, L. Y. Yan and W. J. Si, Characterization of attapulgite clay grafted AMPS/AM superabsorbent resin prepared by microwave irradiation, Polym. Mater. Sci. Eng., 2012, 28(12), 17–20 CAS.
- G. F. Ma, F. T. Ran, E. K. Feng, Z. Zhang and Z. Q. Lei, Preparation and properties of an organic–inorganic composite superabsorbent based on attapulgite, J. Compos. Mater., 2016, 50(14), 1865–1874 CrossRef CAS.
- Z. Djamaa, R. Chebout, K. Bachari and D. Lerari, Microwave-assisted soft method for synthesis of poly (acrylic acid)/organo-clay composites: application as superabsorbent for phenol and Cr (VI), ChemistrySelect, 2023, 8(42), e202302459 CrossRef CAS.
- J. F. Mukerabigwi, S. J. Lei, L. Fan, H. L. Wang, S. Y. Luo, X. Y. Ma, J. Qin, X. Y. Huang and Y. Cao, Eco-friendly nano-hybrid superabsorbent composite from hydroxyethyl cellulose and diatomite, RSC Adv., 2016, 6(38), 31607–31618 RSC.
- H. F. Li, L. R. Fan, L. R. Jing, J. Q. Song, M. S. Li and H. Q. Tian, Swelling behavior of palygorskite/poly(sodium acrylate) superabsorbent composites, Acta Mater. Compositae Sin., 2009, 26, 24–28 CAS.
- X. N. Shi, W. B. Wang and A. Q. Wang, Swelling behavior of guar gum-g-poly(sodium acrylate-co-styrene)/attapulgite superabsorbent composites, J. Macromol. Sci., 2011, 50, 1847–1863 CAS.
- J. M. Zhang, H. Chen and A. Q. Wang, Study on superabsorbent composite. III. Swelling behaviors of polyacrylamide/attapulgite composite based on acidified attapulgite and organo-attapulgite, Eur. Polym. J., 2005, 41(10), 2434–2442 CrossRef CAS.
- W. B. Wang and A. Q. Wang, Synthesis and swelling behaviors of guar gum-g-poly(sodium acrylate-co-styrene)/vermiculite superabsorbent composites, J. Compos. Mater., 2011, 45(21), 2189–2198 CrossRef.
- M. Sharma and A. Bajpai, Superabsorbent nanocomposite from sugarcane bagasse, chitin and clay: Synthesis, characterization and swelling behaviour, Carbohydr. Polym., 2018, 193, 281–288 CrossRef CAS PubMed.
- Y. Liu, Y. F. Zhu, B. Mu, X. C. Wang and A. Q. Wang, Fenton-like redox-initiated synthesis of superabsorbent composites with excellent water retention and swelling properties based on green tea and oil shale semi-coke, Eur. Polym. J., 2023, 182, 111716 CrossRef CAS.
|
This journal is © The Royal Society of Chemistry 2024 |
Click here to see how this site uses Cookies. View our privacy policy here.