DOI:
10.1039/D4RA00790E
(Paper)
RSC Adv., 2024,
14, 14296-14302
Metabolomic profiling of Medicago sativa-derived fungal endophytes and evaluation of their biological activities†
Received
1st February 2024
, Accepted 7th April 2024
First published on 30th April 2024
Abstract
This study aimed to discover the potential of Medicago sativa-derived fungal endophytes as a prospective source of bioactive metabolites. In the present study, three different strains of fungal endophyte Aspergillus terreus were isolated from leaves L, roots T and stems St of Medicago sativa to explore their biological and chemical diversity. These isolated fungi were exposed to different fermentation conditions by adding various chemical elicitors to their solid fermentation media. According to LC-HRESIMS-based metabolomics and multivariate analysis, each chemical treatment had a different effect on the chemical profiles of the fungi. Orthogonal Projections to Latent Structures Discriminant Analysis (OPLS-DA) proposed several compounds with anticancer action against MCF-7 (a human breast cancer cell line) and MDA-MB-231 (a human epithelial breast cancer cell line).
1 Introduction
Endophytic fungi and bacteria are capable of colonizing the internal tissues of plants in a non-pathogenic manner, exhibiting no discernible symptoms of disease. They can live outside or inside cells for a short length of time or their entire life. These endophytes represent an untapped natural resource for bioactive chemicals. They can generate metabolites that are chemically similar to those of their hosts, or they can develop metabolites that are unique to themselves.1 Altering the environment in which fungal endophytes are grown allows for the production of a wide variety of novel metabolites. The OSMAC approach (One Strain Many Compounds) utilizes the change in the microorganism's culture conditions and fermentation parameters to activate silent biosynthetic gene clusters to produce new secondary metabolites.2 OSMAC is widely and successfully used nowadays in the induction of new metabolites as well as in drug discovery campaigns.3
M. sativa (Family Fabaceae) is one of the most cultivated plant around the world, and has been recognized as “the king of forage”.4 Many ancient Chinese medical books, such as the Compendium of Materia Medica, mentioned M. sativa as a food supplement. The ancients called it alfac-facah (the father of all food).5 It has many bioactive ingredients, such as flavonoids, saponins, alkaloids, coumarins, fatty acids and essential oils. The flavonoids are the main chemical constituents.6,7 It can help treat various diseases, such as diabetes, ulcers, arthritis pain, fluid retention and more.7–9
Metabolomics is a technique that can identify the chemical fingerprint of complex crude extracts and the marker metabolites related to specific biological activities. This can save time and effort for purification and re-isolation procedures.10,11 It can also help in optimizing fungal fermentation methods and isolating certain bioactive compounds.12 By using multivariate analysis and analytical tools such as Nuclear Magnetic Resonance (NMR) and Liquid Chromatography coupled with High-Resolution Mass Spectrometry (LC-HRMS), a wide range of metabolites in a crude extract and their pharmacological efficacy can be determined.13 LC-HRMS-based metabolomic profiling has the capability to effectively identify novel metabolites by distinguishing between recognized and unknown. This way, we can quickly track and characterize the unwanted non-bioactive or known metabolites from a given extract.14 The large amount of metabolomics data generated by a chosen analytical tool requires multivariate analysis (MVA) for grouping samples into different categories and studying the distribution of metabolites among these categories.15 The most common MVA and key tools for this purpose are Principal Component Analysis (PCA), Partial Least Square-Discriminant Analysis (PLS-DA) and Orthogonal Partial Least Square-Discriminant Analysis (OPLS-DA).16
As a part of our ongoing research on the fungal endophytes isolated from M. sativa,17 this study focused on three endophytic fungal strains isolated from M. sativa using an LC-MS-based metabolomics and multivariate analyses to investigate these fungi and studying the effect of adding different chemical elicitors on their metabolic profiles. Additionally, each produced extract was tested for its anticancer activity against two breast cancer cell lines; MCF-7 and MDA-MB-231. Using a number of statistical analyses of the generated Mass Spectroscopy (MS) data, a number of metabolites were to be linked to the anticancer activity of the active extracts.
2 Material and methods
2.1 Plant material
The leaves, stems, and roots of M. sativa were harvested fresh from the botanical garden at the Science Faculty of Minia University in Minia, Egypt. Professor Naser Barakat of Minia University's Botany and Microbiology Department, Faculty of Science, was able to positively identify the plant under study. The Pharmacognosy Department, Faculty of Pharmacy, Minia University, Minia, Egypt, maintained a voucher specimen (Mn-Ph-Cog-040).
2.2 Molecular inspection of purified endophytic fungi
The taxonomic identification of the three isolated endophytic fungal strains from M. sativa was achieved through DNA amplification and sequencing of the fungal internal transcribed spacer (ITS) area using the universal primers ITS1 and ITS4.18,19 In their native form for BLAST homology searches in GenBank. After evaluating the fungal strains to other sequences in GenBank, we found that they were 99.9% similar to A. terreus (Genbank accession No. MT300182, MT300174, MT300177). The identified fungal strains can be found at Microbiology Department, Faculty of Science, Minia University, Egypt.
2.3 Fermentation
The three isolated endophytic fungi were fermented under different culturing conditions. Six different elicitors were added to solid malt-agar media (glucoseamine E1, nicotinamide E2, sodium butyrate E3, erythromycin E4, cycloheximide E5 and tetracycline E6), which consisted of 10 g agar, 7.5 g malt and 0.5 L distilled water. The solid media were sterilized by autoclave and then the elicitors were added under aseptic conditions before pouring the media into Petri dishes. There were 5 Petri dishes per strain for each treatment. The Petri dishes were inoculated with the pure fungal strains and then incubated for 15 days at 30 °C.
2.4 Fungal culture extraction
Each Petri plate from each treatment was extracted with EtOAc (100 mL × 3). The extraction efficiency was maximized by employing an ultrasonic equipment to slice and homogenize the agar cultures in advance to extraction. Extracts were then vacuum evaporated to remove moisture and get dry extracts. In order to perform the LC-HRMS analysis, the dried extracts were re-dissolved in MeOH (1 mg mL−1).
Chemicals and reagents, isolation and identification of entophytic fungi, LC-HRMS analysis, statistical and multivariate analysis, selected cell lines for anti-cancer activity of endophytic fungal extract and method of MTT assay are available at ESI.†
3 Results and discussion
3.1 Metabolomic profiling and multivariate analysis
The twenty one extracts produced from the three fungal strains derived from M. sativa upon their fermentation using different elicitors (i.e., same culture media with different elicitors) were analyzed by LC-HRMS to reveal their chemical diversity, similarity, and uniqueness of secondary metabolites among different groups. Both positive and negative ionization modes were used to detect the maximum possible metabolites, considering their different physical nature and ionization potential in each extract. Using LC-HRESIMS analysis with both ionization modes, 9350 peaks were found in the 21 extracts. The HRESIMS data were subjected to principal component analysis (PCA) (Fig. 1A) to observe the chemical variation among the three fungi under different fermentation conditions. The PCA-2D scores plot showed that the chemical profiles of the examined extracts varied substantially, and the model was validated by a permutation test of 1000 permutations, (p < 0.001). The three fungal strains A. terreus (L, St and R) under specific treatments clustered separately from each other. A. terreus R (A1B) in media with elicitor 0.1 M sodium butyrate E3 and elicitor 0.1 M erythromycin E4 had distinct chemical profiles and clustered more separately from other groups. However, the same strain A. terreus R (A1B) in media with elicitor 0.1 M glucosamine E1 and in elicitor 0.1 M nicotinamide E2 had no significant difference in their chemical profiles as they clustered together despite being obtained from different treatments (Fig. 1A). The HRESIMS data was used to partial least squares discriminant analysis (PLS-DA) to identify which metabolites could distinguish between the various culture medium types. The highly predictive PLS-DA model (R2 = 0.9 and Q2 = 0.923) and its score plots were used to divide 21 culture media into five groups (Fig. 1B). The PLS-DA VIP scores (Table 1) were used to study the metabolites arising from each of the five unique groups (variables with a VIP score greater than 1.5).
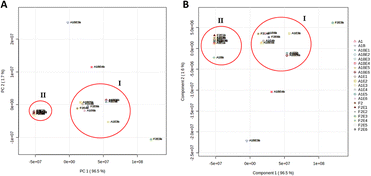 |
| Fig. 1 The A and B score plots depict PCA and PLS-DA results based on LC-HRMS data for the three fungal strains under study. | |
Table 1 Table of induced metabolites that are notable for each set of three fungal strains under varied culturing conditionsa
Groups |
Culture condition |
Distinguished metabolites for each group |
Mass |
Molecular formula |
Vip score |
A1 = Aspergillus terreus (L), A1B = Aspergillus terreus (R), F2 = Aspergillus terreus (St), E1 = glucoseamine, E2 = nicotinamide, E3 = sodium butyrate, E4 = erythromycin, E5 = cycloheximide, E6 = tetracycline. |
Group I |
A. terreus R (A1B) E6 |
Echinulin [1] |
461.3047 |
C29H39N3O2 |
2.12 |
A. terreus L (A1) E51 |
A. terreus St (F2) E5 |
Neoechinulin A [2] |
323.1639 |
C19H21N3O2 |
2.04 |
A. terreus L (A1) E32 |
A. terreus St (F2) E4 |
Questin [3] |
284.0681 |
C16H12O5 |
1.94 |
A. terreus L (A1) E63 |
A. terreus St (F2) E6 |
Flavoglaucin [4] |
304.2032 |
C19H28O3 |
1.71 |
A. terreus L (A1) E44 |
A. terreus R (A1B) E55 |
Group II |
A. terreus R (A1B) |
Cladosporin [5] |
292.1306 |
C16H20O5 |
1.92 |
A. terreus St (F2) E16 |
A.terreus L (A1) E17 |
A. terreus St (F2) |
Lovastatin [6] |
404.2567 |
C24H36O5 |
1.89 |
A. terreus R (A1B) E28 |
A. terreus L (A1)9 |
A. terreus R (A1B) E1 |
Itaconicacid [7] |
130.0262 |
C5H6O4 |
1.77 |
A. terreus L (A1) E210 |
A. terreus St (F2) E311 |
Group III |
A. terreus St (F2) E3 |
Penicillic acid [8] |
170.0575 |
C8H10O4 |
1.81 |
Kojic acid [9] |
142.0269 |
C6H6O4 |
1.96 |
Group IV |
A. terreus R (A1B) E4 |
Parietin [10] |
284.0689 |
C16H12O5 |
1.99 |
Emodin [11] |
270.0528 |
C15H10O5 |
1.92 |
Group V |
A. terreus R (A1B) E3 |
Mycophenolic acid [12] |
320.1265 |
C17H20O6 |
2.21 |
The induced metabolites of the three fungal strains are summarized in Table 1 and Fig. 2–6 for each treatment. During the dereplication procedure, a taxonomic filter was used to select only hits that were related to the A. terreus strains investigated. The culture conditions were divided into five groups (Table 1) based on the similar induced metabolites in each group. The present analysis will prioritize the selection of distinctive metabolites for each group and afterwards discuss the noteworthy metabolites associated with each group. Previous studies have shown that echinulin [1] (Fig. 2) one of the metabolmics present in group I has modest cytotoxic effect against both drug-resistant and drug-sensitive human prostate cancer cells20. Echinulin-related compounds have diverse biological activities, such as antimicrobial,21 cytotoxic,22 antiviral22 and antioxidant.23 Neoechinulin A [2] has garnered the most interest among the alkaloids related to echinulin on account of its neuroprotective characteristics.24 Questin [3] showed strong antibacterial and bactericidal effects against Vibrio harveyi through the disruption of the bacterial cell wall and membrane.25 Flavoglaucin [4] was an excellent antioxidant and synergist.26 Many other fungi, including Cladosporium, Aspergillus, Eurotium, and Chaetomium, have been found producing cladosporin [5] in group II (Fig. 3).27 It had antifungal,27 insecticidal,28 antibacterial29 and plant growth regulatory effects30 as well as anti-inflammatory31 and antiparasitic activity in both blood and liver stages.32 Lovastatin [6] isolated from A. terreus had diverse biological effects such as antihyperlipidemic,33 anti-depressant, anti-inflammatory, neuroprotective and anticancer.34 Itaconic acid [7] mainly produced by fungi35 such as A. terreus and exert different bioactivities such as antimicrobial36 and anti-inflammatory activities.37 Group III (Fig. 4) contained 5 discriminant metabolites identified as penicillic acid [8], which showed cytotoxic activity as reported by Suzuki, et al., 1971.38
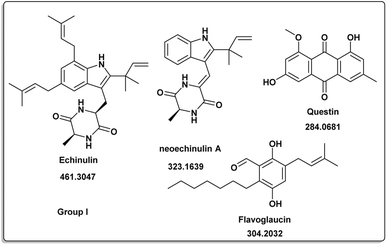 |
| Fig. 2 Induced metabolites of group I (Table 1) which is characterized for the different culture media (different culture with different treatment but has same secondary metabolites). | |
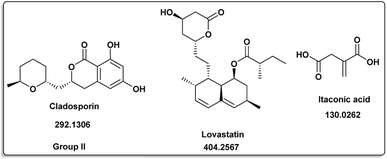 |
| Fig. 3 Induced metabolites of group II (Table 1) which is characterized for the different culture media (different culture with different treatment but has same secondary metabolites). | |
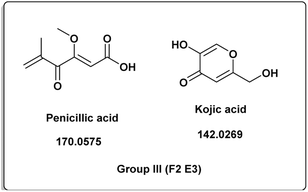 |
| Fig. 4 Induced metabolites of group III (Table 1) which is characterized for the different culture media (different culture with different treatment but has same secondary metabolites). | |
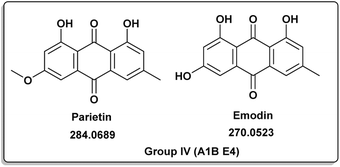 |
| Fig. 5 Induced metabolites of group IV (Table 1) which is characterized for the different culture media (different culture with different treatment but has same secondary metabolites). | |
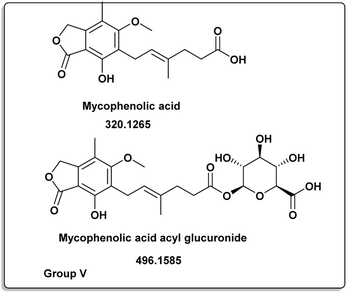 |
| Fig. 6 Induced metabolites of group V (Table 1) which is characterized for the different culture media (different culture with different treatment but has same secondary metabolites). | |
Penicillic acid [8] revealed its cytotoxicity (cell growth inhibitions >95%) against the tumor melanoma (MDA-MB-435) and colon cancer (HCT-8) cell lines.39 The natural product kojic acid [9] produced by the fungus Aspergillus, Penicillium, and Acetobacter40 exhibited a wide range of biological activities, including powerful tyrosine-inhibiting capabilities, antioxidant, and anticancer activities.41,42 Kojic acid [9] could act as an immunomodulatory agent by promoting the differentiation of monocytes into macrophages.43 Group IV (Fig. 5) was distinguished by the presence of emodin [11] and parietin [10], a derivative of emodin.
Antitumor, antiviral, antioxidant, anti-osteoporotic, anti-inflammatory, and depressive actions were just some of the many pharmacological effects they exhibited.44,45 Parietin [10] successfully suppressed cancer cell proliferation by reducing 6-phosphogluconate dehydrogenase activity or inducing cell death.45,46 Mycophenolic acid (12) was distinct for group V (Fig. 6). It had a variety of biological properties including antifungal, antiviral, antibacterial, antitumor or immunosuppressive activities.47–52
3.2 Anti-cancer activity
For our cytotoxicity experiments, we have used two different human breast cancer cell lines: MCF-7 and MDA-MB-231. It was found that extracts of A. terreus isolated from stems of M. sativa in different elicitor treatments E6, E3, E5 and E1 exhibited significant inhibitory activity against both tested cancer cell lines, with IC50 values comparable to the positive control, doxorubicin.
3.3 Bioactivity–metabolites correlation
The anticancer activity results were analyzed using an OPLS-DA-based approach to identify possible metabolites linked to the anticancer activity of extracts derived from A. terreus spp. (i.e., those obtained via the standard solid fermentation and with different elicitors, Table 2). Very high levels of performance and predictive ability (R2 = 0.91, Q2 = 0.89) were displayed by the model. Furthermore, our OPLS-DA model is not likely over fitted because a permutation test (1000 permutations) was performed to cross-validate it and showed that none of the permutation-result models (i.e., based on random data) were superior to the original one (p 0.001). Breast carcinoma extracts with IC50 values ≤ 20 μg mL−1 were regarded to have anticancer activity. Higher levels were considered inactive, and only two breast cancer types (MCF-7) and (MDA-MB-231) were evaluated. In Fig. 7A, the active and inactive extracts were clearly separated, with the active extracts clustering together, indicating the existence of common metabolites that may be associated to their anticancer activity. Consequently, we utilized the OPLS-DA-derived S-plots (Fig. 7B) to isolate the bioactive discriminating metabolites. Table 3 and Fig. 8 illustrate the most significant metabolites (P-value ≤ 0.01) in the functional extracts. The most promising candidate metabolite associated with the active extracts' anticancer action is porritoxin (accurate mass = 305.1624, P = 2.28 × 10−4). Porritoxin was discovered as a benzoxazocine derivative initially from the culture broth of Alternaria porri, the causal pathogen of black spot disease in stone-leek and onion.53 Porritoxin's structure was subsequently changed as isoindol-1-one congener.54 At 10 μg mL−1, this chemical reduced shoot and root growth in lettuce seedlings.53 The porritoxin [1] from A. porri has been reported to show anti-tumor-promoting activity.55 Giluterrin [2] (accurate mass = 484.1994, P = 2.67 × 10−5) a cytotoxic prenylated indole alkaloid with a novel carbon skeleton.56 When tested against a normal cell line, giluterrin showed cytotoxic effects, very similar to those of the gold standard doxorubicin.57 Giluterrin also showed specific cytotoxicity, with IC50 values of 22.93 μM and 48.55 μM, against kidney cancer cell line 786-0 and prostate cancer cell line PC-3, respectively.58 Dehydroaltenusin [3] (accurate mass = 288.0631, P = 3.74 × 10−4) also has been reported as a selective inhibitor of eukaryotic DNA polymerases.59 Asperlicin [4] (accurate mass = 535.2222, P = 5.23 × 10−3). Asperlicin is a potent antagonist of cholecystokinin.59 Cholecystokinin is a neurotransmitter hormone that regulates digestive processes like pancreas secretion and gallbladder contraction.59
Table 2 In vitro cytotoxicity of A. terreus fermentation extracts with various elicitorsa
Sample |
IC50 (MCF7) |
IC50 (MDAMB231) |
L = leaves, R = root, St = stem, E1 = glucoseamine, E2 = nicotinamide, E3 = sodium butyrate, E4 = erythromycin, E5 = cycloheximide, E6 = tetracycline. |
A. terreus R |
86.26 ± 1.3 |
97.29 ± 2.1 |
A. terreus L |
73.81 ± 0.97 |
56.19 ± 0.86 |
A. terreus St |
32.29 ± 2.7 |
21.19 ± 0.73 |
A. terreus R E1 |
64.22 ± 2.6 |
> 100 |
A. terreus L E1 |
75.54 ± 1.3 |
83.26 ± 0.74 |
A. terreus St E1 |
14.19 ± 2.2 |
11.11 ± 1.3 |
A. terreus R E2 |
24.75 ± 1.4 |
17.25 ± 2.9 |
A. terreus L E2 |
>100 |
>100 |
A. terreus St E2 |
20.15 |
15.29 ± 2.7 |
A. terreus R E3 |
93.26 ± 2.1 |
89.29 ± 0.4 |
A. terreus LE3 |
48.83 ± 1.7 |
66.29 ± 1.3 |
A. terreus St E3 |
9.32 ± 0.8 |
6.24 ± 1.2 |
A. terreus R E4 |
>100 |
>100 |
A. terreus L E4 |
>100 |
>100 |
A. terreus St E4 |
43.27 ± 2.1 |
26.2 ± 1.8 |
A. terreus R E5 |
37.26 ± 1.1 |
24.45 ± 1.8 |
A. terreus L E5 |
>100 |
>100 |
A. terreus St E5 |
12.25 ± 1.9 |
8.3 ± 0.7 |
A. terreus RE6 |
>100 |
>100 |
A. terreus L E6 |
>100 |
>100 |
A. terreus St E6 |
5.31 ± 1.5 |
7.21 ± 1.2 |
Doxorubicin |
2.62 ± 0.71 |
1.53 ± 0.24 |
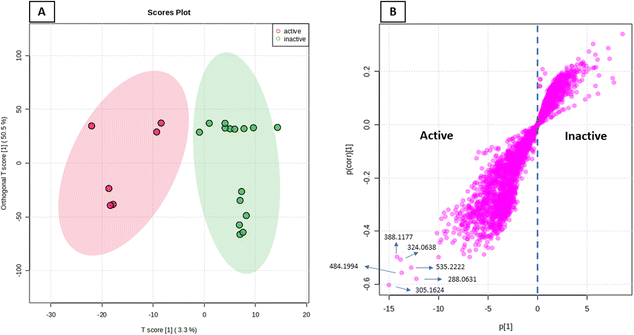 |
| Fig. 7 OPLS-DA score plot of active vs. inactive extracts (A), together with its S-plot showing the highly correlated metabolites to the anticancer activity (B). | |
Table 3 Significant and highly associated putatively active metabolites were dereplicated and ranked by P-value
No |
Dereplication |
Mass |
Molecular formula |
Reported activity |
Probability P |
1 |
Porritoxin |
305.1624 |
C17H23NO4 |
Anti-tumer activity55 |
2.28 × 10−4 |
2 |
Giluterrin |
484.1994 |
C29H28N2O5 |
Cytotoxicity towards 786-0 (kidney) and PC-3 (prostate) cancer cells56 |
2.67 × 10−5 |
3 |
Dehydroaltenusin |
288.0631 |
C15H12O |
Selective inhibitor of eukaryotic DNA polymerases59 |
3.74 × 10−4 |
4 |
Asperlicin |
535.2222 |
C31H29N5O4 |
Potent antagonist of cholecystokinin59 |
5.23 × 10−3 |
5 |
Atromentin |
324.0638 |
C18H12O6 |
Induce apoptosis in human leukemia u937 cells60 |
4.17 × 10−3 |
6 |
Serantrypinone |
388.1177 |
C21H16N4O4 |
Insecticide61 |
3.18 × 10−3 |
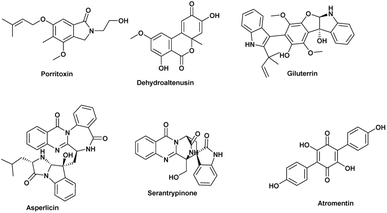 |
| Fig. 8 The most possibly observed metabolites for their activity against two types of breast cancer (MCF-7) and (MDA-MB-231) according to OPLS-DA. | |
Atropentin [5] (exact mass = 324.0638, P = 4.17 × 10−3) has been found to trigger apoptosis in human leukemia u937 cells, and is thus another metabolite associated with anticancer action.60 Serantrypinone [6] (acuret mass = 388.1177, P = 3.18 × 10−3), was previously isolated from Penicillium thymicola in 2001 by the Larsen group.62 Additionally, an assay aimed at identifying insect GABA receptor inhibitors led to the isolation of serantrypinone from A. terreus culture broth.61 Later on, it was demonstrated through a comparison between synthetic and natural samples that both Penicillium thymicola and A. terreus produce the same enantiomer of serantrypinone.63 GABA receptors are susceptible to a variety of insecticides due to their involvement in neurotransmission processes within the insect nervous system. The selectivity of serantrypinone for insect GABA receptors compared to rat GABA receptors was reported to be 61-fold higher by Ozoe and coworkers. This data implies that mammals may be more resistant to the alkaloid's hazardous effects than insects are.61,63
4 Conclusions
Three endophytic fungi isolated from Medicago sativa (leaves L, roots T and stems St) have been examined for their diversity and anticancer potential. To broaden the spectrum of metabolites the fungi could produce, they were grown in a variety of media (OSMAC strategy). Also, the biological activities of the fungal extracts under each condition were tested and found that they varied depending on the culture conditions. This reflected the different chemical composition of each extract. MVA (PLS-DA and OPLS-DA) used to analyze the LC-HRMS data and the biological activity results, This assisted in the identification of biomarkers in each extract and the suggestion of metabolites that showed anticancer activities in human breast cancer cell lines MCF-7 and MDA-MB-231. This strategy would be of a great help in discovering natural products with bioactive properties from their natural sources and save time and effort for isolating certain metabolites with high biological potential.
Author contributions
Conceptualization: DEA, AMS, SHE, OMS, URA and RM. Formal analysis: AMS, OMS and URA. Investigation: AMS, DEA, OMS and RM. Resources: OMS, AMS, URA and EZA. Supervision: SHE, URA and RM. Writing original data: DEA, AMS, OMS, URA and RM. Writing-review and editing DEA, AMS, SHE, OMS, EZA, URA and RM. All authors have agreed to this published version.
Conflicts of interest
There are no conflicts to declare.
References
- H. Nisa, A. N. Kamili, I. A. Nawchoo, S. Shafi, N. Shameem and S. A. Bandh, Microb. Pathog., 2015, 82, 50–59 CrossRef CAS PubMed.
- R. T. Hewage, T. Aree, C. Mahidol, S. Ruchirawat and P. Kittakoop, Phytochemistry, 2014, 108, 87–94 CrossRef CAS PubMed.
- R. Pan, X. Bai, J. Chen, H. Zhang and H. Wang, Front. Microbiol., 2019, 10, 294 CrossRef PubMed.
- Q.-G. Ma, T. Li, R.-R. Wei, W.-M. Liu, Z.-P. Sang and Z.-W. Song, J. Agric. Food Chem., 2016, 64, 8138–8145 CrossRef CAS PubMed.
- A. A. Ehsanpour and R. Razavizadeh, Am. J. Biochem. Biotechnol., 2005, 1, 107–110 CrossRef CAS.
- P. Abbruscato, S. Tosi, L. Crispino, E. Biazzi, B. Menin, A. M. Picco, L. Pecetti, P. Avato and A. Tava, J. Agric. Food Chem., 2014, 62, 11030–11036 CrossRef CAS PubMed.
- K. Rafińska, P. Pomastowski, O. Wrona, R. Górecki and B. Buszewski, Phytochem. Lett., 2017, 20, 520–539 CrossRef.
- K. S. Bora and A. Sharma, Pharm. Biol., 2011, 49, 211–220 CrossRef PubMed.
- L. Chen, J. Liu, Y. Zhang, Y. Niu, B. Dai and L. Yu, J. Agric. Food Chem., 2015, 63, 2970–2978 CrossRef CAS PubMed.
- E. M. Mohamed, M. H. Hetta, M. E. Rateb, M. A. Selim, A. M. AboulMagd, F. A. Badria, U. R. Abdelmohsen, H. A. Alhadrami and H. M. Hassan, Molecules, 2020, 25, 1714 CrossRef CAS PubMed.
- M. S. Hifnawy, M. A. Aboseada, H. M. Hassan, A. M. AboulMagd, A. F. Tohamy, S. H. Abdel-Kawi, M. E. Rateb, E. M. B. El Naggar, M. Liu and R. J. Quinn, Metabolites, 2020, 10, 31 CrossRef CAS PubMed.
- A. F. Tawfike, R. Tate, G. Abbott, L. Young, C. Viegelmann, M. Schumacher, M. Diederich and R. Edrada-Ebel, Chem. Biodiversity, 2017, 14, e1700040 CrossRef PubMed.
- N. D. Yuliana, A. Khatib, Y. H. Choi and R. Verpoorte, Phytother. Res., 2011, 25, 157–169 CrossRef CAS PubMed.
- S. S. El-Hawary, A. M. Sayed, R. Mohammed, H. M. Hassan, M. E. Rateb, E. Amin, T. A. Mohammed, M. El-Mesery, A. Bin Muhsinah and A. Alsayari, Mar. Drugs, 2019, 17, 465 CrossRef CAS PubMed.
- R. Madsen, T. Lundstedt and J. Trygg, Anal. Chim. Acta, 2010, 659, 23–33 CrossRef CAS PubMed.
- J. Trygg, E. Holmes and T. Lundstedt, J. Proteome Res., 2007, 6, 469–479 CrossRef CAS PubMed.
- A. M. Sayed, N. H. Sherif, A. O. El-Gendy, Y. I. Shamikh, A. T. Ali, E. Z. Attia, M. M. H. El-Katatny, B. A. Khalifa, H. M. Hassan and U. R. Abdelmohsen, Nat. Prod. Res., 2022, 36, 2404–2408 CrossRef CAS PubMed.
- S. El-Hawary, R. Mohammed, S. AbouZid, W. Bakeer, R. Ebel, A. Sayed and M. Rateb, J. Appl. Microbiol., 2016, 120, 900–911 CrossRef CAS PubMed.
- S. S. El-Hawary, A. M. Sayed, M. E. Rateb, W. Bakeer, S. F. AbouZid and R. Mohammed, Nat. Prod. Res., 2017, 31, 2568–2571 CrossRef CAS PubMed.
- O. F. Smetanina, A. N. Yurchenko, E. V. Girich, P. T. H. Trinh, A. S. Antonov, S. A. Dyshlovoy, G. von Amsberg, N. Y. Kim, E. A. Chingizova and E. A. Pislyagin, Molecules, 2019, 25, 61 CrossRef PubMed.
- L. Xu, W. Meng, C. Cao, J. Wang, W. Shan and Q. Wang, Mar. Drugs, 2015, 13, 3479–3513 CrossRef CAS PubMed.
- X. Chen, L. Si, D. Liu, P. Proksch, L. Zhang, D. Zhou and W. Lin, Eur. J. Med. Chem., 2015, 93, 182–195 CrossRef CAS PubMed.
- Y. Miyake, C. Ito, T. Kimura, A. Suzuki, Y. Nishida and M. Itoigawa, Food Sci. Technol. Res., 2014, 20, 139–146 CrossRef CAS.
- K. Kimoto, T. Aoki, Y. Shibata, S. Kamisuki, F. Sugawara, K. Kuramochi, A. Nakazaki, S. Kobayashi, K. Kuroiwa and N. Watanabe, J. Antibiot., 2007, 60, 614–621 CrossRef CAS PubMed.
- L. Guo, F. Zhang, X. Wang, H. Chen, Q. Wang, J. Guo, X. Cao and L. Wang, 3 Biotech, 2019, 9, 1–7 Search PubMed.
- Y. Ishikawa, K. Morimoto and T. Hamasaki, J. Am. Oil Chem. Soc., 1984, 61, 1864–1868 CrossRef CAS.
- P. M. Scott, W. van Walbeek and W. M. MacLean, J. Antibiot., 1971, 24, 747–755 CrossRef CAS PubMed.
- J. Grove and M. Pople, Mycopathologia, 1981, 76, 65–67 CrossRef CAS.
- H. Anke, J. Antibiot., 1979, 32, 952–958 CrossRef CAS PubMed.
- J. P. Springer, H. G. Cutler, F. G. Crumley, R. H. Cox, E. E. Davis and J. E. Thean, J. Agric. Food Chem., 1981, 29, 853–855 CrossRef CAS.
- J. Miller, M. Sun, A. Gilyan, J. Roy and T. Rand, Chem.–Biol. Interact., 2010, 183, 113–124 CrossRef CAS PubMed.
- D. Hoepfner, C. W. McNamara, C. S. Lim, C. Studer, R. Riedl, T. Aust, S. L. McCormack, D. M. Plouffe, S. Meister and S. Schuierer, Cell Host Microbe, 2012, 11, 654–663 CrossRef CAS PubMed.
- A. Alberts, J. Chen, G. Kuron, V. Hunt, J. Huff, C. Hoffman, J. Rothrock, M. Lopez, H. Joshua and E. Harris, Proc. Natl. Acad. Sci., 1980, 77, 3957–3961 CrossRef CAS PubMed.
- L. Xie, G. Zhu, J. Shang, X. Chen, C. Zhang, X. Ji, Q. Zhang and Y. Wei, Cell. Signalling, 2021, 87, 110122 CrossRef CAS PubMed.
- M. Sano, T. Tanaka, H. Ohara and Y. Aso, Appl. Microbiol. Biotechnol., 2020, 104, 9041–9051 CrossRef CAS PubMed.
- T. Cordes, A. Michelucci and K. Hiller, Annu. Rev. Nutr., 2015, 35, 451–473 CrossRef CAS PubMed.
- A. Hooftman and L. A. O'Neill, Trends Immunol., 2019, 40, 687–698 CrossRef CAS PubMed.
- S. Suzuki, T. Kimura, F. SAITO and K. Ando, Agric. Biol. Chem., 1971, 35, 287–290 CrossRef CAS.
- T. G. Montenegro, F. A. Rodrigues, P. C. Jimenez, A. L. Angelim, V. M. Melo, E. Rodrigues Filho, M. d. C. F. de Oliveira and L. V. Costa-Lotufo, Chem. Biodiversity, 2012, 9, 2203–2209 CrossRef CAS PubMed.
- M. Saeedi, M. Eslamifar and K. Khezri, Biomed. Pharmacother., 2019, 110, 582–593 CrossRef CAS PubMed.
- A. d. J. Gomes, C. N. Lunardi, S. Gonzalez and A. C. Tedesco, Braz. J. Med. Biol. Res., 2001, 34, 1487–1494 CrossRef CAS PubMed.
- G. Karakaya, A. Ercan, S. Öncül and M. Aytemir, J. Res. Pharm., 2019, 23, 596–607 CAS.
- J. P. Da Costa, A. P. D. Rodrigues, L. H. S. Farias, P. C. R. Frade, B. J. M. Da Silva, J. L. M. Do Nascimento and E. O. Silva, Biomed. Pharmacother., 2018, 101, 100–106 CrossRef CAS PubMed.
- X. Dong, J. Fu, X. Yin, S. Cao, X. Li, L. Lin, Huyiligeqi and J. Ni, Phytother. Res., 2016, 30, 1207–1218 CrossRef CAS PubMed.
- Y. Liu, S. Chu, S. Yang, Y. Peng, S. Ren, B. Wen and N. Chen, Chem.–Biol. Interact., 2019, 310, 108722 CrossRef PubMed.
- R. Lin, S. Elf, C. Shan, H.-B. Kang, Q. Ji, L. Zhou, T. Hitosugi, L. Zhang, S. Zhang and J. H. Seo, Nat. Cell Biol., 2015, 17, 1484–1496 CrossRef CAS PubMed.
- M. S. Diamond, M. Zachariah and E. Harris, Virology, 2002, 304, 211–221 CrossRef CAS PubMed.
- D. R. Kuypers, Y. Le Meur, M. Cantarovich, M. J. Tredger, S. E. Tett, D. Cattaneo, B. Tönshoff, D. W. Holt, J. Chapman and T. Van Gelder, Clin. J. Am. Soc. Nephrol., 2010, 5, 341–358 CrossRef CAS PubMed.
- R. Nicoletti, M. De Stefano, S. De Stefano, A. Trincone and F. Marziano, Mycopathologia, 2004, 158, 465–474 CrossRef CAS PubMed.
- R. J. Tressler, L. J. Garvin and D. L. Slate, Int. J. Cancer, 1994, 57, 568–573 CrossRef CAS PubMed.
- R. Torrenegra, J. Baquero and J. Calderon, Rev. Latinoam. Quim., 2005, 33, 76–81 CAS.
- B. Mouhamadou, L. Sage, S. Périgon, V. Séguin, V. Bouchart, P. Legendre, M. Caillat, H. Yamouni and D. Garon, Fungal Biol., 2017, 121, 103–111 CrossRef CAS PubMed.
- R. Suemitsu, K. Ohnishi, M. Horiuchi, A. Kitaguchi and K. Odamura, Phytochemistry, 1992, 31, 2325–2326 CrossRef.
- M. Horiuchi, T. Maoka, N. Iwase and K. Ohnishi, J. Nat. Prod., 2002, 65, 1204–1205 CrossRef CAS PubMed.
- M. T. Horiuchi, H. Ohnishi, K. Yamashita, M. Nishino, H. Maoka and T. Porritoxins, Nat. Prod., 2006, 20, 161–166 CrossRef CAS PubMed.
- J. R. Gubiani, M. C. Oliveira, R. A. Neponuceno, M. J. Camargo, W. S. Garcez, A. R. Biz and H. L. Teles, Phytochem. Lett., 2019, 32, 162–167 CrossRef CAS.
- M.-R. Ke, S.-F. Chen, X.-H. Peng, Q.-F. Zheng, B.-Y. Zheng, C.-K. Yeh and J.-D. Huang, Eur. J. Med. Chem., 2017, 127, 200–209 CrossRef CAS PubMed.
- J. R. Gubiani, M. C. Oliveira, R. A. Neponuceno, M. J. Camargo, W. S. Garcez, A. R. Biz, M. A. Soares, A. R. Araujo, V. d. S. Bolzani and H. C. Lisboa, Phytochem. Lett., 2019, 32, 162–167 CrossRef CAS.
- N. Maeda, Y. Kokai, S. Ohtani, H. Sahara, I. Kuriyama, S. Kamisuki, S. Takahashi, K. Sakaguchi, F. Sugawara and H. Yoshida, Biochem. Biophys. Res. Commun., 2007, 352, 390–396 CrossRef CAS PubMed.
- J. H. Kim and C. H. Lee, J. Microbiol. Biotechnol., 2009, 19, 946–950 CrossRef CAS PubMed.
- T. Kuriyama, E. Kakemoto, N. Takahashi, K.-i. Imamura, K. Oyama, E. Suzuki, K. Harimaya, T. Yaguchi and Y. Ozoe, J. Agric. Food Chem., 2004, 52, 3884–3887 CrossRef CAS PubMed.
- M. R. Ariza, T. O. Larsen, B. O. Petersen, J. Ø. Duus, C. Christophersen and A. F. Barrero, J. Nat. Prod., 2001, 64, 1590–1592 CrossRef CAS PubMed.
- D. J. Hart, ARKIVOC, 2010, IV, 32–65 Search PubMed.
Footnotes |
† Electronic supplementary information (ESI) available: Material and methods for isolation and identification of endophytic fungi, methodology and chromatograms of LC-HRMS analysis. See DOI: https://doi.org/10.1039/d4ra00790e |
‡ Two authors are equally contributed to the work and they are corresponding authors. |
|
This journal is © The Royal Society of Chemistry 2024 |
Click here to see how this site uses Cookies. View our privacy policy here.