DOI:
10.1039/D4RA00517A
(Paper)
RSC Adv., 2024,
14, 5975-5980
Ir(III)/Ag(I)-catalyzed directly C–H amidation of arenes with OH-free hydroxyamides as amidating agents†
Received
20th January 2024
, Accepted 9th February 2024
First published on 15th February 2024
Abstract
A versatile Ir(III)-catalyzed C–H amidation of arenes by employing readily available and stable OH-free hydroxyamides as a novel amidation source. The reaction occurred with high efficiency and tolerance of a range of functional groups. A wide scope of aryl OH-free hydroxyzamides, including conjugated and challenging non-conjugated OH-free hydroxyzamides, were capable of this transformation and no addition of an external oxidant is required. This protocol provided a simple, straightforward and economic method to a variety N-(2-(1H-pyrazol-1-yl)alkyl)amide derivates with good to excellent yield. Mechanistic study demonstrated that reversible C–H bond functionalisation might be involved in this reaction.
Introduction
Pyrazole and its derivatives play crucial roles in organic and pharmaceutical fields, because of their unique five-membered nitrogen-containing heterocyclic structures.1 In synthetic chemistry, pyrazole and its derivatives are not only efficiently and conveniently synthesized, but also further transformed into more complex artificial molecules.2 In the continuous development of coordination chemistry, various complexes containing pyrazole ligands have also been synthesized, characterized, and further applied.3 In the field of biomedicine, pyrazole and its derivatives have also been proven to have good antibacterial, anti-inflammatory, and anti-tumor activities.4 Because of the multi-reactivity and practicality profile, there is a continued strong demand for efficient and selective synthesis of pyrazole and its derivatives for theoretical and practical research. The most common synthesis methods mainly focus on the following parts: (1) Knorr pyrazole synthesis reaction and further expanded to α,β-unsaturated carbonyl compounds;5 (2) pyrazole and its derivatives can also be obtained from the reaction of hydrazones and ketones, alkynes, and isonitriles catalyzed by metal-free conditions;6 (3) direct functionalization reactions of pyrazole and its derivatives.7 Based on previous work, there has been good progress in the research of transition metal catalyzed pyrazole synthesis methodologies.8 Actually, cost-effective, and feasibility degree synthesis methods are still needful, in view of the broad range of applications of pyrazole in biology and chemistry.
Transition-metal-catalyzed C–H functionalization has become powerful tools for synthesis of non-cyclic or cyclic artificial molecule, which complementary to traditional synthesis methods.9 C–H functionalization reaction has unparalleled advantages in the construction of chemical bonds and compounds.10 Particularly, significant progress has been made in C–H bond amidation reactions in recent years.11 Heterocyclic compounds represented by pyrazole are excellent directing groups in C–H bond activation reactions, which catalyzed by Cp*Rh(III), Cp*Ir(III), Ru(II) and other metal.12 At the same time, a series of amidation reagents, such as N-substituted hydroxylamines,13N-methoxyamide,14 dioxazolones,15 organic azide,16 chloramines and other substrates,17 have been widely used as C–N coupling partners for construction of structurally complex scaffolds. However, the application of OH-free hydroxyamide as a novel amidation reagent in C–H bond activation are relatively limited. Therefore, the development of an efficient one pot method to give ortho-functionalized pyrazole derivatives via Ir(III)/Ag(I)-catalyst C–H bond amination reactions of N-arylpyrazoles and OH-free hydroxyamides. In this methodology, OH-free hydroxyamides were innovatively used as the amidation source, which has excellent selectivity, stability and reactivity compared to organic azides via comparative experiment (Scheme 1).
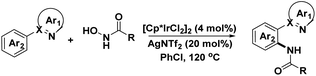 |
| Scheme 1 Ir(III)/Ag(I)-catalyzed C–H directly amination with N-hydroxy amide. | |
Results and discussion
We started our investigation by submitting the model substrate N-phenyl-pyrazole (1a) and N-hydroxybenzamide (2a). The reaction carried out with [Cp*IrCl2]2 (4 mol%) as catalyst, AgNTf2 as an (20 mol%) additive, without any external base and oxidant, in 1,2-DCE at 90 °C for 8 h under air. As result, the desired amidated product (3aa) was obtained in 57% isolated yield without any bisamidated product was detected. After constructing this feasible concept, the confirmed results encourage us to further conduct optimization studies with an initial focus on the catalyst, among the various catalyst investigated, the [Cp*IrCl2]2 was found most efficient, other metal catalyst such as[Cp*RhCl2]2, Pd(OAc)2 and Pd(PPh3)4 could not promoted this transformation (Table 1, entry 1–4). The control experiment further confirmed no amidating product could be obtained without [Cp*IrCl2]2 or AgNTf2 (Table 1, entry 5–6). In order to improve the yield of the reaction, several solvents were taken into consideration, such as toluene, PhCl, MeCN, DMSO and 1,4-dioxane were screened (Table 1, entry 7–11), wherein PhCl was the best choice with 67% isolated yield. Subsequently, a variety of additive were used, unfortunately, none of them worked (Table 1, entry 12–17). The effect of temperate variation was also investigated and increasing it to 120 °C could assisted the transformation. However, further increase of temperature the yield with slightly decrease (Table 1, entry 18–21). In addition, reducing or increasing catalyst, additive and time were detrimental to the yield (Table 1, entry 22–28).
Table 1 Optimization of the reaction conditionsa
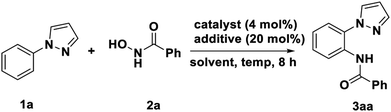
|
Entry |
Catalyst |
Additive |
Solvent |
Temp (oC) |
Yieldb (%) |
Reaction conditions: N-phenylpyrazole 1a (0.25 mmol), N-hydroxybenzamide 2a (0.25 mmol), solvent (1.5 mL), and catalyst (4.0 mol%) for 8 h.
Isolated yields.
2 mol% of catalyst was used.
8 mol% of catalyst was used.
10 mol% of additive was used.
40 mol% of additive was used.
60 mol% of additive was used.
reaction time for 4 h.
reaction time for 12 h.
|
1 |
[Cp*IrCl2]2 |
AgNTf2 |
1,2-DCE |
90 |
57 |
2 |
[Cp*RhCl2]2 |
AgNTf2 |
1,2-DCE |
90 |
Trace |
3 |
Pd(OAc)2 |
AgNTf2 |
1,2-DCE |
90 |
Trace |
4 |
Pd(PPh3)4 |
AgNTf2 |
1,2-DCE |
90 |
Trace |
5 |
— |
AgNTf2 |
1,2-DCE |
90 |
Trace |
6 |
[Cp*IrCl2]2 |
— |
1,2-DCE |
90 |
Trace |
7 |
[Cp*IrCl2]2 |
AgNTf2 |
Toluene |
90 |
46 |
8 |
[Cp*IrCl2]2 |
AgNTf2 |
PhCl |
90 |
67 |
9 |
[Cp*IrCl2]2 |
AgNTf2 |
MeCN |
90 |
61 |
10 |
[Cp*IrCl2]2 |
AgNTf2 |
DMSO |
90 |
Trace |
11 |
[Cp*IrCl2]2 |
AgNTf2 |
1,4-Dioxane |
90 |
23 |
12 |
[Cp*IrCl2]2 |
AgSbF6 |
PhCl |
90 |
Trace |
13 |
[Cp*IrCl2]2 |
AgOAc |
PhCl |
90 |
Trace |
14 |
[Cp*IrCl2]2 |
Ag2CO3 |
PhCl |
90 |
Trace |
15 |
[Cp*IrCl2]2 |
Ag2O |
PhCl |
90 |
Trace |
16 |
[Cp*IrCl2]2 |
CsOPiv |
PhCl |
90 |
Trace |
17 |
[Cp*IrCl2]2 |
HOAc |
PhCl |
90 |
Trace |
18 |
[Cp*IrCl2]2 |
AgNTf2 |
PhCl |
50 |
28 |
19 |
[Cp*IrCl2]2 |
AgNTf2 |
PhCl |
70 |
54 |
20
|
[Cp*IrCl
2
]
2
|
AgNTf
2
|
PhCl
|
120
|
86
|
21 |
[Cp*IrCl2]2 |
AgNTf2 |
PhCl |
140 |
84 |
22c |
[Cp*IrCl2]2 |
AgNTf2 |
PhCl |
120 |
58 |
23d |
[Cp*IrCl2]2 |
AgNTf2 |
PhCl |
120 |
83 |
24e |
[Cp*IrCl2]2 |
AgNTf2 |
PhCl |
120 |
42 |
25f |
[Cp*IrCl2]2 |
AgNTf2 |
PhCl |
120 |
67 |
26g |
[Cp*IrCl2]2 |
AgNTf2 |
PhCl |
120 |
62 |
27h |
[Cp*IrCl2]2 |
AgNTf2 |
PhCl |
120 |
56 |
28i |
[Cp*IrCl2]2 |
AgNTf2 |
PhCl |
120 |
86 |
With the optimized reaction conditions in hand, we next examined the tolerance of this methodology, and the corresponding results are summarized in the Table 2. We first explored the scope of N-aryl pyrazole with 2a as coupling partner. It was found that a series of para-position substituted underwent smoothly amidation with 2a deliver the corresponding products in good to excellent yields (83–94%) with high tolerance of functional groups. Even for sensitive iodine-substituted substrates also could provide the corresponding product 3la in 87% yield. Especially, the substrates containing trifluoromethyl, Cyano group with 2a deliver the corresponding products 3ma and 3na in 84% and 83% yield, respectively. For the meta-substituted substrates, the yields show slightly decreases 3ba, 3ga and 3ha compared with para-substituents 3ca, 3ja and 3ka. In the end, we also investigated the reaction activity of naphthalene ring, the product 3oa in 94% yield was obtained and confirmed the structure by single crystal diffraction. A gram-level experiment was conducted, and the corresponding product can still be obtained in 88% yield (1.37 g).
Table 2 Scope of N-aryl pyrazoles in C–H bond amidationa,b
Reaction conditions: N-aryl pyrazoles 1 (0.25 mmol), N-hydroxybenzamide 2a (0.25 mmol), [Cp*IrCl2]2 (4 mol%), and AgNTf2 (40 mol%) in PhCl (3 mL) were stirred at 120 °C under air for 8 h.
Isolated yields.
|
|
Encouraged by these positive outcomes, we further examined the selective of the Ir(III)/Ag(I)-catalyzed C–H bond amidation reaction with N-phenyl-pyrazole (1a), a variety of substituted aryl N-hydroxyzamide 2 were examined, as shown in Table 3. N-hydroxyzamide 2 bearing both electron-donating as well as electron-withdrawing aryl substituents were reacted well with 1a to give the desired amidated products (3ab–3ao) in good to excellent yields. To our delight, bisubstituted aryl N-hydroxyzamide could be successfully converted to the corresponding products 3ai and 3aj with more than 80% yield, respectively. What we need to mention that ortho-position substituted substrates also provided the corresponding product 3ab and 3ag in 91 and 95% yield, respectively. In addition, when we employed N-hydroxycarbamate as amidating agent, there are no amidated products generated under Ir(III)/Ag(I)-catalyst system.
Table 3 Substrates scope of N-hydroxyamidea,b
Reaction conditions: N-phenyl-pyrazole 1 (0.25 mmol), N-hydroxyamide 2 (0.25 mmol), [Cp*IrCl2]2 (4 mol%), and AgNTf2 (40 mol%) in PhCl (3 mL) were stirred at 120 °C under air for 8 h.
Isolated yields.
|
|
To access the general applicability of the protocol, other N-heterocycles-DGs substrates were also took into consideration, such as 2-phenylpyridine, 2-phenylpyrimidine and 1-(pyrimidin-2-yl)-1H-indole, they could be smoothly transformed into desired amidated products in excellent yield (81–95%) (Scheme 2. 9aa–11am). Unfortunately, other DGs derived from pyrazoles did not work well in the transformation (Scheme 2, 12aa, 13aa).
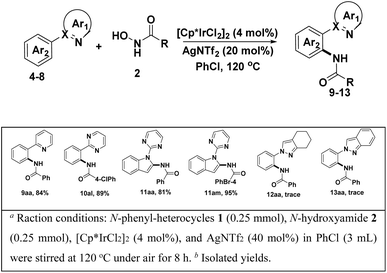 |
| Scheme 2 Scope of other N-heterocycles-DGs substrates.a,b | |
To illustrate the stability and reactivity of N-hydroxyamide, we also applied acyl azides under Ir(III)/Ag(I)-catalyst system. To our delight, desired amidated products were obtained under suitable condition in accepted yield, without any Curtius rearrangement product,18 and the results shown in Scheme 5. In the selected example, the yield of the acyl azide as the amidation reagent is lower than that of the N-hydroxylamine as the amidation reagent (Scheme 3).
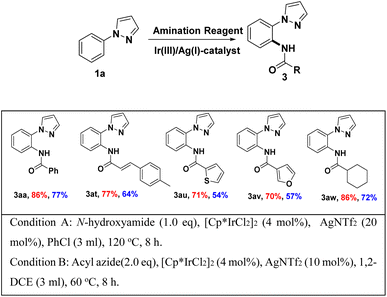 |
| Scheme 3 Comparison between acyl azides and N-hydroxylamine as amidating agents.a,b | |
To investigate the mechanism of this reaction, we carried out a series of control experiments. First, substrate 1a was treated with CD3OD under standard reaction conditions. As a result, no D-exchange was detected, suggesting that C–H bond metalation is irreversible (Scheme 4, eqn (a)). Coupling of 1a and 2a under the standard conditions in the presence of CD3OD afforded 3aa with 24% H/D exchange was detected at the ortho-position of the product 3aa, indicative of the irreversibility of the C–H activation process under the catalytic conditions (Scheme 4, eqn (a)). By employing deuterium-labeled compound [D5]-1a as substrate, we further determined the kinetic isotope effect (KIE) value of the parallel and intermolecular experiments to be 2.0 and 3.0 respectively, indicating that the cleavage of C–H bond at the ortho-position of N-phenyl-pyrazole might be involved in the rate-determining step (Scheme 4, eqn (b and c)).
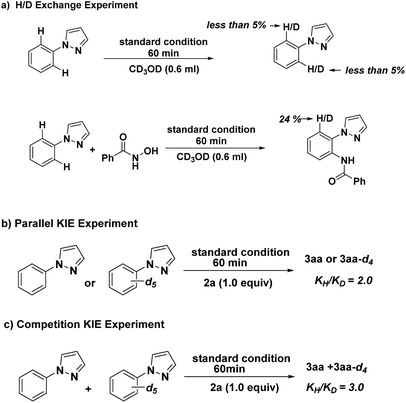 |
| Scheme 4 Control experiments. | |
Based on the above results and literature precedence,19 a plausible mechanism is presented in Scheme 5. First, the treatment of [Cp*RhCl2]2 with AgNTf2 gives rise to the cationic Rh(III) species A, which undergo C–H bond activation with 1 to generated HNTf2 and five-membered rhodacycle B, then coordinated to N-hydroxylamine 2 to afford intermediate C followed by migratory insertion, resulting in the formation of complex D and the release of H2O. Finally, proto-demetalation step lead to final product 3 and regenerated the Rh(III)-catalyst.
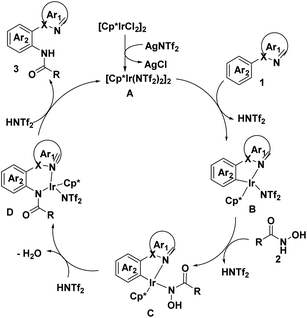 |
| Scheme 5 Plausible mechanism. | |
Conclusions
In summary, the combination of iridium catalyst and AgNtf2 additive deliver a highly efficient catalytic system for the C–H activation of N-aryl pyrazole with N-hydroxyamide, which allows the high efficient synthesis 2-amide substituted N-aryl pyrazole derivatives without any bisamidated products. This C–H bond amidation protocol is applicable to the coupling of a wide range of OH-free hydroxylamine, the reaction proceeds under oxidant-free or base-free conditions, enabling facile access to amidated products in good to high yields with a broad functional group tolerance. We believe that this strategy has potential applications in organic synthesis, as well as medicinal chemistry.
Conflicts of interest
There are no conflicts to declare.
Acknowledgements
This project was supported by the Natural Science Research Key Project of Education Department of Anhui Province (2022AH051381), the Doctoral Scientific Research Foundation (2021BSK009, 2021BSK040, 2020BS019) of Suzhou University.
References
-
(a) S. Fustero, M. Sánchez-Roselló, P. Barrio and A. Simón-Fuentes, Chem. Rev., 2011, 111, 6984–7034 CrossRef CAS;
(b) P. K. Mykhailiuk, Chem. Rev., 2021, 121, 1670–1715 CrossRef CAS PubMed;
(c) S. P. Chandrasekharan, A. Dhami, S. Kumara and K. Mohanan, Org. Biomol. Chem., 2022, 20, 8787–8817 RSC;
(d) E. David, K. Thirumoorthy and N. Palanisami, Mater. Chem. Front., 2021, 5, 8290–8307 RSC;
(e) R. Lan, Q. Liu, P. Fan, S. Lin, S. R. Fernando, D. McCallion, R. Pertwee and A. Makriyannis, J. Med. Chem., 1999, 42, 769–776 CrossRef CAS PubMed;
(f) A. Olyaei and M. Sadeghpour, New J. Chem., 2020, 44, 14791–14813 RSC;
(g) V. L. M. Silva and A. M. S. Silva, Molecules, 2023, 28, 5873 CrossRef CAS PubMed.
-
(a) L. Y. Janin, Chem. Rev., 2012, 112, 3924–3958 CrossRef PubMed;
(b) P. Majumdar, A. Pati, M. Patra, R. K. Behera and A. B. Kumar, Chem. Rev., 2014, 114, 2942–2977 CrossRef CAS;
(c) L. D. Shirtcliff, S. P. McClintock and M. M. Haley, Chem. Soc. Rev., 2008, 37, 343–364 RSC;
(d) T. Deb, J. Tu and R. M. Franzini, Chem. Rev., 2021, 121, 6850–6914 CrossRef CAS PubMed;
(e) T. Pavithra, E. S. Devi and U. C. Maheswari, Asian J. Org. Chem., 2021, 10, 1861–1883 CrossRef CAS.
-
(a) Á. Vivancos, C. Segarra and M. Albrecht, Chem. Rev., 2018, 118, 9493–9586 CrossRef;
(b) Y. Chi and P. T. Chou, Chem. Soc. Rev., 2010, 39, 638–655 RSC;
(c) S. Liu, Chem. Soc. Rev., 2004, 33, 445–461 RSC;
(d) J. C. Bernhammera and H. V. Huynh, Dalton Trans., 2012, 41, 8600–8608 RSC.
-
(a) J. Yang, P. Gharagozloo, J. Yao, V. I. Ilyin, R. B. Carter, P. Nguyen, S. Robledo, R. M. Woodward and D. J. Hogenkamp, J. Med. Chem., 2004, 47, 1547–1552 CrossRef CAS;
(b) S. Roy, H. Raj, J. Roberts, J. Hastings, D. F. Gilmore, R. C. Shields and M. A. Alam, J. Med. Chem., 2023, 66, 13622–13645 CrossRef CAS;
(c) X. Wu, Z. Yang, M. Bu, J. Duan and A. Zhang, Molecules, 2023, 28, 7509 CrossRef CAS PubMed;
(d) H. M. Alkahtani, A. A. Almehizia, M. A. Al-Omar, A. J. Obaidullah, A. A. Zen, A. S. Hassan and W. M. Aboulthana, Molecules, 2023, 28, 7125 CrossRef CAS;
(e) G. Poce, S. Consalvi, G. Venditti, S. Alfonso, N. Desideri, R. Fernandez-Menendez, R. H. Bates, L. Ballell, D. B. Aguirre, J. Rullas, A. De Logu, M. Gardner, T. R. Ioerger, E. J. Rubin and M. Biava, ACS Med. Chem. Lett., 2019, 10, 1423–1429 CrossRef CAS PubMed;
(f) M. Chalkha, M. Akhazzane, F. Z. Moussaid, O. Daoui, A. Nakkabi, M. Bakhouch, S. Chtita, S. Elkhattabi, A. I. Housseinic and M. E. Yazidi, New J. Chem., 2022, 46, 2747–2760 RSC.
-
(a) T. Dai, Q. Li, X. Zhang and C. Yang, J. Org. Chem., 2019, 84, 5913–5921 CrossRef CAS;
(b) B. Sarmah and R. Srivastava, Ind. Eng. Chem. Res., 2017, 56, 515017–515029 Search PubMed;
(c) S. R. Stauffer and J. A. Katzenellenbogen, J. Comb. Chem., 2000, 2, 318–329 CrossRef CAS;
(d) A. Kowalczyk, G. Utecht-Jarzyńska, G. Mlostoń and M. Jasiński, Org. Lett., 2022, 24, 2499–2503 CrossRef CAS PubMed;
(e) C. Guo, B. Sahoo, C. G. Daniliuc and F. Glorius, J. Am. Chem. Soc., 2014, 136, 17402–17405 CrossRef CAS PubMed;
(f) Q. Wang, Z. Zhang, Z. Du, H. Hua and S. Chen, Green Chem., 2013, 15, 1048–1054 RSC.
-
(a) J. Wen, H. Tang, K. Xiong, Z. Ding and Z.-P. Zhan, Org. Lett., 2014, 16, 5940–5943 CrossRef CAS PubMed;
(b) P. V. Khairnar, T.-H. Lung, Y. Lin, C. Wu, S. R. Koppolu, A. Edukondalu, P. Karanam and W. Lin, Org. Lett., 2019, 21, 4219–4223 CrossRef CAS PubMed;
(c) L. Wei, S. Ding, M. Liu, Z. Yu and Y. Xiao, Org. Lett., 2021, 23, 7718–7723 CrossRef CAS;
(d) Y. Tu, Z. Zhang, T. Wang, J. Ke and J. Zhao, Org. Lett., 2017, 19, 3466–3469 CrossRef CAS;
(e) S. Choudhary, M. K. Muthyala, K. Parang and A. Kumar, Org. Chem. Front., 2014, 1, 683–688 RSC;
(f) S. Katiyar, A. Kumara and K. V. Sashidhara, Chem. Commun., 2022, 58, 7297–7300 RSC.
-
(a) S. J. Han, H. T. Kim and J. M. Joo, J. Org. Chem., 2016, 81, 689–698 CrossRef CAS PubMed;
(b) M. Wang, J. C. Simon, M. Xu, S. A. Corio, J. S. Hirschi and V. M. Dong, J. Am. Chem. Soc., 2023, 145, 14573–14580 CrossRef CAS;
(c) O. S. Kim, J. H. Jang, H. T. Kim, S. J. Han, G. C. Tsui and J. M. Joo, Org. Lett., 2017, 19, 1450–1453 CrossRef CAS PubMed;
(d) V. Garg, P. Kumar and A. K. Verma, J. Org. Chem., 2017, 82, 10247–10262 CrossRef CAS;
(e) E. Kang, H. T. Kim and J. M. Joo, Org. Biomol. Chem., 2020, 18, 6192–6210 RSC;
(f) Y. Zuo, X. He, Y. Ning, Q. Tang, M. Xie, W. Hu and Y. Shang, Org. Biomol. Chem., 2019, 17, 9766–9771 RSC;
(g) Z. Chen, Z. Ding, T. Chen, L. Meng and G. Wang, Org. Biomol. Chem., 2020, 18, 8486–8490 RSC.
-
(a) A. J. Pearce, R. P. Harkins, B. R. Reiner, A. C. Wotal, R. J. Dunscomb and I. A. Tonks, J. Am. Chem. Soc., 2020, 142, 4390–4399 CrossRef CAS;
(b) J. S. Chen, D. L. Golden, S. W. Krska and S. S. Stahl, J. Am. Chem. Soc., 2021, 143, 14438–14444 CrossRef PubMed;
(c) W. Yang, S. Ye, D. Fanning, T. Coon, Y. Schmidt, P. D. Stamos and J.-Q. Yu, Angew. Chem., Int. Ed., 2014, 54, 2501–2504 CrossRef PubMed;
(d) N. Gulia and O. Daugulis, Angew. Chem., Int. Ed., 2016, 56, 3630–3634 CrossRef PubMed;
(e) A. M. Haydl, K. Xu and B. Breit, Angew. Chem., Int. Ed., 2015, 55, 7149–7153 CrossRef.
-
(a) P. Gandeepan, T. Müller, D. Zell, G. Cera, S. Warratz and L. Ackermann, Chem. Rev., 2019, 119, 2192–2452 CrossRef CAS PubMed;
(b) T. Dalton, T. Faber and F. Glorius, ACS Cent. Sci., 2021, 7, 245–261 CrossRef CAS;
(c) Z. Zeng, H. Gao, Z. Zhou and W. Yi, ACS Catal., 2022, 12, 14754–14772 CrossRef CAS;
(d) J. Zhang, X. Lu, C. Shen, L. Xu, L. Ding and G. Zhong, Chem. Soc. Rev., 2021, 50, 3263–3314 RSC;
(e) S. K. Sinha, P. Ghosh, S. Jain, S. Maiti, S. A. Al-Thabati, A. A. Alshehri, M. Mokhtar and D. Maiti, Chem. Soc. Rev., 2023, 52, 7461–7503 RSC;
(f) G. Song, F. Wang and X. Li, Chem. Soc. Rev., 2012, 41, 3651–3678 RSC.
-
(a) C. Sambiagio, D. Schönbauer, R. Blieck, T. Dao-Huy, G. Pototschnig, P. Schaaf, T. Wiesinger, M. F. Zia, J. Wencel-Delord, T. Besset, B. U. W. Maes and M. Schnürch, Chem. Soc. Rev., 2018, 47, 6603–6743 RSC;
(b) S. Rana, J. P. Biswas, S. Paul, A. Paika and D. Maiti, Chem. Soc. Rev., 2021, 50, 243–472 RSC;
(c) Z. Dong, Z. Ren, S. J. Thompson, Y. Xu and G. Dong, Chem. Rev., 2017, 117, 9333–9403 CrossRef CAS;
(d) N. K. Mishra, S. Sharma, J. Park, S. Han and I. S. Kim, ACS Catal., 2017, 7, 2821–2847 CrossRef CAS.
-
(a) J. Jiao, K. Murakami and K. Itami, ACS Catal., 2016, 6, 610–633 CrossRef CAS;
(b) D. Hazelard, P.-A. Nocque and P. Compain, Org. Chem. Front., 2017, 4, 2500–2521 RSC;
(c) Y. N. Timsina, B. F. Gupton and K. C. Ellis, ACS Catal., 2018, 8, 5732–5776 CrossRef CAS;
(d) J. Ma, X. Zhou, J. Chen, J. Shi, H. Cheng, P. Guo and H. Ji, Org. Biomol. Chem., 2022, 20, 7554–7576 RSC;
(e) M. V. K. Rao, S. Kareem, S. R. Vali and B. V. S. Reddy, Org. Biomol. Chem., 2023, 21, 8426–8462 RSC.
-
(a) L. Cao, Y. Hua, H. Cheng and Q. Zhou, Org. Chem. Front., 2021, 8, 3883–3914 RSC;
(b) S. A. Babu, Y. Aggarwal, P. Patela and R. Tomara, Chem. Commun., 2022, 58, 2612–2633 RSC;
(c) M. Moselage, J. Li and L. Ackermann, ACS Catal., 2016, 6, 498–525 CrossRef CAS;
(d) W. Liu and L. Ackermann, ACS Catal., 2016, 6, 3743–3752 CrossRef CAS;
(e) S. Nakanowatari, R. Mei, M. Feldt and L. Ackermann, ACS Catal., 2017, 7, 2511–2515 CrossRef CAS;
(f) M. Zhang, Z. Zhong, L. Liao and A. Q. Zhang, Org. Chem. Front., 2022, 9, 3882–3896 RSC;
(g) B. Liu, L. Yang, P. Li, F. Wang and X. Li, Org. Chem. Front., 2021, 8, 1085–1101 RSC;
(h) S. V. Kumar, S. Banerjeea and T. Punniyamurthy, Org. Chem. Front., 2020, 7, 1527–1569 RSC;
(i) G. Rani, V. Luxami and K. Paul, Chem. Commun., 2020, 56, 12479–12521 RSC.
-
(a) P. Patel and S. Chang, Org. Lett., 2014, 16, 3328–3331 CrossRef CAS PubMed;
(b) P. Patel and S. Chang, ACS Catal., 2015, 5, 853–858 CrossRef CAS;
(c) X. Wang, T. Gensch, A. Lerchen, C. G. Daniliuc and F. Glorius, J. Am. Chem. Soc., 2017, 139, 6506–6512 CrossRef CAS PubMed.
-
(a) G. Ju, C. Yuan, D. Wang, J. Zhang and Y. Zhao, Org. Lett., 2019, 21, 9852–9855 CrossRef CAS PubMed;
(b) C. Zhou, J. Zhao, W. Guo, J. Jiang and J. Wang, Org. Lett., 2019, 21, 9315–9319 CrossRef CAS PubMed;
(c) G. Ju, G. Li, G. Qian, J. Zhang and Y. Zhao, Org. Lett., 2019, 21, 7333–7336 CrossRef CAS PubMed;
(d) T. Ban, H.-M. Vu, J. Zhang, J.-Y. Yong, Q. Liu and X.-Q. Li, J. Org. Chem., 2022, 87, 5543–5555 CrossRef CAS PubMed;
(e) H. Xu, Y. Zhu, J. Yang, X. Chaia and L. Dong, Org. Chem. Front., 2020, 7, 1230–1234 RSC.
-
(a) J. Park and S. Chang, Angew. Chem., Int. Ed., 2015, 54, 14103–14107 CrossRef CAS PubMed;
(b) Y. Liang, Y. Liang, C. Tang, Y. Yuan and N. Jiao, Chem.–Eur. J., 2015, 21, 16395–16399 CrossRef CAS PubMed;
(c) Q. Ma, X. Yu, R. Lai, S. Lv, W. Dai, C. Zhang, X. Wang, Q. Wang and Y. Wu, ChemSusChem, 2018, 11, 3672–3678 CrossRef CAS PubMed;
(d) Z. Zhou, J. Kweon, H. Jung, D. Kim, S. Seo and S. Chang, J. Am. Chem. Soc., 2022, 144, 9161–9171 CrossRef CAS PubMed.
-
(a) C. Pan, C. He, J. Wang, J. Tang and X. Zhang, Org. Biomol. Chem., 2024, 22, 1181–1185 RSC;
(b) T. A. Shah, P. B. De, S. Pradhan, S. Banerjee and T. Punniyamurthy, J. Org. Chem., 2019, 84, 16278–16285 CrossRef CAS PubMed;
(c) K. Yoo, J. Lee, M. H. Park, Y. Kim, H. J. Kim and M. Kim, J. Org. Chem., 2020, 85, 6233–6241 CrossRef CAS;
(d) M. V. K. Rao, S. Kareem, S. R. Vali and B. V. S. Reddy, Org. Biomol. Chem., 2023, 21, 8426–8462 RSC;
(e) D. Mu, X. Wang, G. Chen and G. He, J. Org. Chem., 2017, 82, 4497–4503 CrossRef CAS PubMed;
(f) X. Xiao, G. Jia, F. Liu, G. Ou and Y. Xie, J. Org. Chem., 2018, 83, 13811–13820 CrossRef CAS;
(g) Y. Du, C. Zhou, W. To, H. Wang and C. M. Che, Chem. Sci., 2020, 11, 4680–4686 RSC.
-
(a) T. Kawano, K. Hirano, T. Satoh and M. Miura, J. Am. Chem. Soc., 2010, 132, 6900–6901 CrossRef CAS;
(b) E. Kianmehr, Y. A. Lomedasht, N. Faghih and K. M. Khan, J. Org. Chem., 2016, 81, 6087–6092 CrossRef CAS;
(c) Z. Yin, Z. Wang and X. Wu, Org. Biomol. Chem., 2018, 16, 2643–2646 RSC.
-
(a) A. K. Ghosh, M. Brindisi and A. Sarkar, ChemMedChem, 2018, 13, 2351–2373 CrossRef CAS;
(b) K. Yoshimura, K. Okano, R. Ishikawa, H. Yamamoto, M. Sumimoto and K. Hori, J. Phys. Org. Chem., 2012, 25, 394–399 CrossRef CAS;
(c) A. K. Ghosh, A. Sarkar and M. Brindisia, Org. Biomol. Chem., 2018, 16, 2006–2027 RSC;
(d) L. Song, Y. Meng, T. Zhao, L. Liu, X. Pan, B. Huang, H. Yao, R. Lin and R. Tong, Green Chem., 2024, 26, 428–438 RSC;
(e) H. Peng, Z. Yang, S. Huang and T. Liu, Chin. J. Org. Chem., 2010, 28, 1223–1228 CrossRef CAS;
(f) K. Lin and H. Lu, Org. Lett., 2023, 25, 4534–4539 CrossRef CAS PubMed;
(g) T. Kalita, D. Dev, S. Mondal, R. S. Giri and B. Mandal, Asian J. Org. Chem., 2021, 10, 1523–1529 CrossRef CAS.
-
(a) S. K. Sinha, P. Ghosh, S. Jain, S. Maiti, S. A. Al-Thabati, A. A. Alshehri, M. Mokhtar and D. Maiti, Chem. Soc. Rev., 2023, 52, 7461–7503 RSC;
(b) K. Seth, Org. Chem. Front., 2022, 9, 3102–3141 RSC;
(c) R. Li and X. Yang, Dalton Trans., 2021, 50, 12888–12895 RSC;
(d) C. Chen, C. Shi, Y. Yang and B. Zhou, Chem. Sci., 2020, 11, 12124–12129 RSC;
(e) S. Devkota, S. Kim, S. Y. Yoo, S. Mohandoss, M. H. Baik and Y. R. Lee, Chem. Sci., 2021, 12, 11427–11437 RSC;
(f) Y. Park, S. Jee, J. G. Kim and S. Chang, Org. Process Res. Dev., 2015, 19, 1024–1029 CrossRef CAS;
(g) Y. Park, K. T. Park, J. G. Kim and S. Chang, J. Am. Chem. Soc., 2015, 137, 4534–4542 CrossRef CAS;
(h) Q. Xing, C. Chan, Y. W. Yeung and W. Yu, J. Am. Chem. Soc., 2019, 141, 3849–3853 CrossRef CAS PubMed.
|
This journal is © The Royal Society of Chemistry 2024 |
Click here to see how this site uses Cookies. View our privacy policy here.