DOI:
10.1039/D3RA08160E
(Review Article)
RSC Adv., 2024,
14, 8481-8501
Decarboxylative 1,3-dipolar cycloadditions of L-proline
Received
29th November 2023
, Accepted 2nd February 2024
First published on 13th March 2024
Abstract
1,3-Dipolar cycloaddition is one of the important chemical reactions between a 1,3-dipole and a dipolarophile to construct a five-membered heterocyclic compound. As an available α-amino acid reactant, L-proline is extensively used in 1,3-dipolar cycloaddition reactions. A diverse spectrum of bioactive spiro and fused N-heterocycles is obtained through this synthetic approach. In this review, we have described the use of L-proline in the synthesis of various spiro- and fused heterocyclic scaffolds.
1. Introduction
1,3-Dipolar cycloaddition (1,3-DC) reaction is a well-known fundamental strategy for the regio- and stereoselective construction of five-membered spiro and fused heterocyclic compounds.1–5 This synthetic route is considered a powerful tool for the synthesis of many natural products and pharmaceutical molecules containing a pyrrolidine moiety.6–8 Some of these structures are shown in Scheme 1. 1,3-DC exhibits notable advantages over traditional methods, enabling the construction of complex molecular structures from simple starting materials in a one-pot synthesis without separating intermediates.
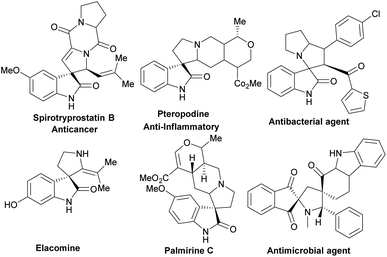 |
| Scheme 1 Some of bioactive spiro-heterocyclic molecules constructing from amino acids. | |
Azomethine ylide intermediates generated in situ from the decarboxylative condensation of amino acids with isatins/aldehydes act as 1,3-dipoles to react with various dipolarophiles via 1,3-DC reaction.9–12 Azomethine ylides consist of two sp2 carbon atoms and one nitrogen atom with a HOMO orbital that directly interacts with a LUMO orbital of a dipolarophile. These intermediates are the most efficient synthons in the construction of spiro-heterocycles, such as pyrrolizines, pyrrolizidines, and pyrazolidines. Among these spiro-heterocycles, spiro-oxindoles show a broad spectrum of biological activities, including anticancer,13,14 antiviral,15,16 antibacterial,17,18 antimalarial,19 anti-tubercular,20 and anti-inflammatory.21
L-Proline is a natural α-amino acid with exceptional conformational rigidity. Due to the presence of a carboxylic acid and an amine, proline is considered a multifunctional amino acid in organic transformations. It can act as a chiral organocatalyst for catalyzing asymmetric catalysis systems, especially aldol condensation, as a chiral bidentate ligand for activating metal complexes, or as a nucleophilic reagent in organic syntheses.22–24 In addition, the availability, cheapness, stability, and non-toxicity of proline make it an attractive molecule for chemists. This privileged α-amino acid is widely used in the multi-component 1,3-DC reactions towards the synthesis of spiro and fused N-heterocyclic motifs.
There are several review articles on the use of amino acids as reactants in organic transformations.25–29 In this review, we have described decarboxylative 1,3-DC reactions of L-proline as a versatile α-amino acid over the last decade.
2. 1,3-Dipolar cycloadditions for synthesis of spiro-heterocycles
2.1. 1,3-Dipolar cycloadditions of proline and isatins with dipolarophiles
2.1.1. 1,3-Dipolar cycloadditions with diones as dipolarophiles. In 2013, Ouyang and co-workers introduced a method for 1,3-DC of isatin 2, α-amino acid 1, and 5-benzylideneimidazolidine-2,4-dione 3 (Scheme 2).30 The reaction was carried out via the formation of azomethine ylide B from the decarboxylative condensation of isatin 2 and L-proline 1, followed by the endo cycloaddition with the dipolarophile 3. A series of regio-, and diastereoselective spiro-pyrrolidines 4 were produced in a multi-component procedure. Moreover, the authors reported the construction of spiro-thiopyrrolidine using L-thioproline as an amino acid reactant.
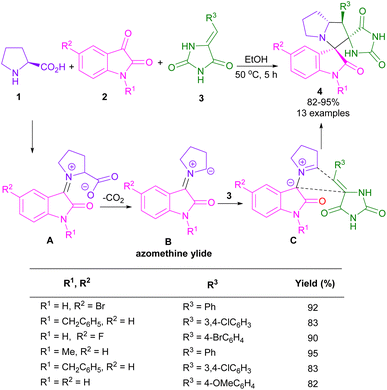 |
| Scheme 2 Reaction of isatin and α-amino acid with 5-benzylideneimidazolidine-2,4-dione. | |
In 2014, an elegant strategy for the synthesis of spiro-oxindolopyrrolizine, or pyrrolo[1,2-c]thiazole fused coumarins 8 was disclosed by Kumar et al. (Scheme 3).31 In this method, the preparation of products was investigated under heating and ultrasound conditions. At first, 2,2-dimethyl-1,3-dioxane-4,6-dione 5, and salicylaldehydes 6 were treated in H2O as a green solvent under ultrasonic irradiation to afford coumarin 3-carboxylic acid A via the aldol condensation and intramolecular cyclization. Afterward, isatins 2, and cyclic α-amino acids 1, or 7 were added to the mixture and stirred for another 1 h. The spiro intermediate B was formed through the condensation of isatin and amino acid. Decarboxylation of B, followed by 1,3-DC with A led to the final product 8. A radical pathway was involved in the decarboxylation step in the presence of ultrasonic irradiation, whereas ionic intermediates were formed under heating conditions. The products were obtained in higher yields in the presence of ultrasound irradiation.
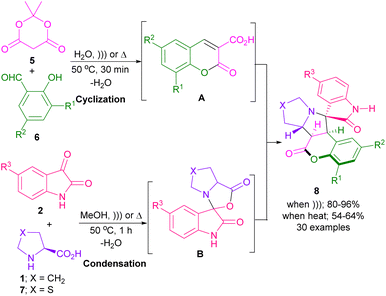 |
| Scheme 3 Synthesis of oxindole-pyrrolizine or pyrrolo[1,2-c]thiazole fused coumarin hybrid heterocycles. | |
In 2015, the Liu32 and Shanmugam33 research teams in two distinct works reported the synthesis of spiro-oxindolopyrrolizines from isatin, L-proline, and different dipolarophiles. Also, Kumar and co-workers were able to make dispiro-oxindolopyrrolizine-thiazolidine-2,4-dione hybrids 10 from the treatment of isatin 2, L-proline 1 and (Z)-5-arylidene-3-(2-cyclopropyl-1-(2-fluorophenyl)-2-oxoethyl)-thiazolidine-2,4-diones 9 (Scheme 4).34 In this work, an unusual regioisomer was synthesized through a 1,3-DC reaction. It was found that 1,3-dipole A passes through uncommon transition state C compared to transition state B. This may be due to the lower free energy activation in terms of the lack of electrostatic repulsion between the carbonyls of the dipole and the dipolarophile in TS C, which favored this pathway (Scheme 5). A variant of the same transformation was developed to make spiro-pyrrolizidines 13, 14, 15 from isatin 2, L-proline 1, and (E)-3-arylidene-1-phenyl-pyrrolidine-2,5-diones 12 (Scheme 6).35 In this 1,3-DC reaction, three stereoisomers were detected in different ratios. Mechanistic studies showed that products 13, or 15 are formed depending on the formation of ylides A or B. As shown in section II, Scheme 7, the iminium cation can provide either 13, or 15 using retro-mannich. Also, retro-1,3-DC can afford 13 from A, or B with alkene 12. Whereas, product 14 was obtained from (3 + 2)-annulation. Also, again (Z)-4-arylidene-1-phenylpyrazolidine-3,5-diones were used as a dipolarophile for the condensation reaction with azomethine ylide generated form isatin and L-proline to produce spiro-oxindolopyrrolizidines.36
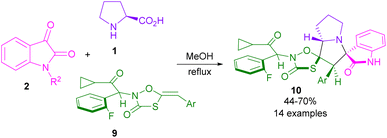 |
| Scheme 4 Reaction of isatin, L-proline and (Z)-5-arylidene-3-(2-cyclopropyl-1-(2-fluorophenyl)-2-oxoethyl)-thiazolidine-2,4-diones. | |
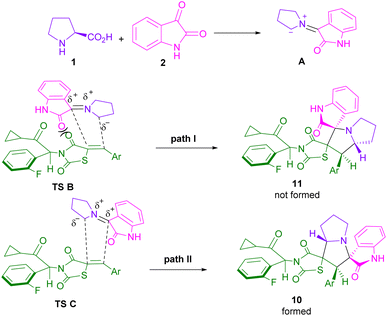 |
| Scheme 5 Two possible pathways for reaction of isatin, L-proline and (Z)-5-arylidene-3-(2-cyclopropyl-1-(2-fluorophenyl)-2-oxoethyl)-thiazolidine-2,4-diones. | |
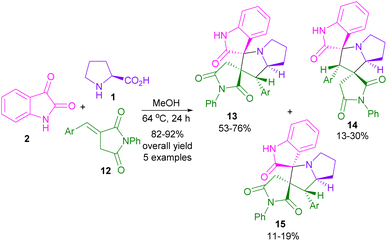 |
| Scheme 6 Reaction of isatin, L-proline and (E)-3-arylidene-1-phenyl-pyrrolidine-2,5-diones. | |
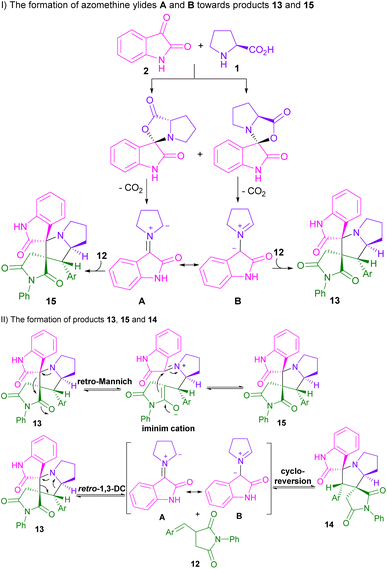 |
| Scheme 7 Plausible mechanism for reaction of isatin, L-proline and (E)-3-arylidene-1-phenyl-pyrrolidine-2,5-diones. | |
In 2021, Bekhradnia and Akhavan described (3 + 2)-cyclization of azomethine ylides with 5-arylidenthiazolidine-2,4-diones 17 as a dipolarophile (Scheme 8).37 To achieve better results, they used an unexampled MnCoCuFe2O4@L-proline magnetic nanorod in this cycloaddition reaction. One-pot synthesis of spiro-pyrrolidine/pyrrolizidine/pyrrolothiazolidine scaffolds 18, 19, or 20, tolerance of heteroaryl substituents in dipolarophile, the use of recyclable nanocatalyst, and high regio-, and diastereoselectivities of the products were among the advantages of this methodology. Similar to Bekhradnia's reaction, El-Tahawy and co-workers employed 3-arylidene-1-methyl-pyrrolidine-2,5-diones as a dipolarophile in the reaction with isatins and L-proline to form spiro-oxindolopyrrolizidines.38 In this method, the cyclization reactions were performed in MeOH as the solvent under reflux conditions. They could also report anti-microbial and anti-coagulant properties of these spiro products in the range of moderate to strong activity.
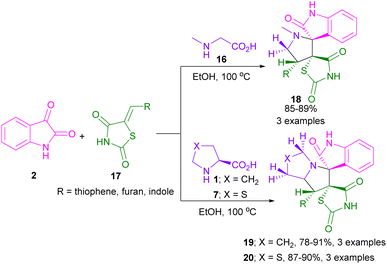 |
| Scheme 8 Reaction of isatin, amino acids and 5-arylidenthiazolidine-2,4-diones. | |
In 2021, the Barakat39 and Hügel40 research groups reported the reactions of isatins, α-amino acids and various dipolarophiles. A series of spiro-oxindolopyrrolizidines were synthesized by Barakat's work, which exhibited potent anticancer activity. In Hügel's report, the resulting spiro products were evaluated as anti-amyloidogenic agents. At the same time, by using azomethine ylides in the reaction with enediones 21, Korotaev et al. could provide spiro-oxindolopyrrolizidine derivatives 22, 23 (Scheme 9).41 Afterward, the treatment of the tetracyclic products with arylhydrazine hydrochloride reagents 24 led to 5-substituted 1,3-diaryl-1H-pyrazole skeletons 25 in moderate to excellent yields.
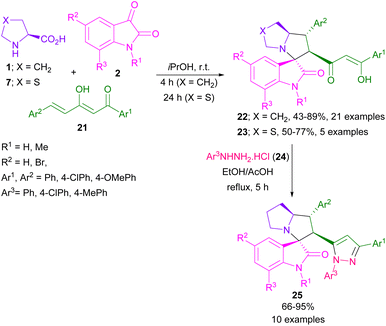 |
| Scheme 9 Reaction of isatins, amino acids and enediones. | |
2.1.2. 1,3-Dipolar cycloadditions with alkynes an allenes as dipolarophiles. In 2013, Pal et al. found that copper salts can catalyze the 1,3-DC of isatins 2, L-proline 1, and terminal alkynes 26 (Scheme 10).42 In this context, CuI, CuBr, or CuCl served as a catalyst, and products were obtained in 92, 78, and 75% yields. Due to the higher efficiency of CuI, the reaction was carried out in the presence of CuI as an optimal catalyst and bioactive spiro-pyrrolidine oxindoles were isolated in high yields. Considering the mechanism in Scheme 11, the imine intermediate D was formed from the reaction of zwitterionic L-proline A and isatin 2 under copper catalysis. Next, the dipolar azomethine ylide F was obtained by the CO2 liberation, which was cyclized with the dipolarophile terminal alkyne 26 in the presence of Cu(I) to provide product 27.
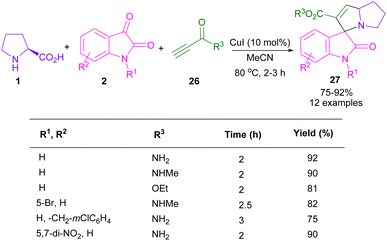 |
| Scheme 10 Reaction of isatin, L-proline and terminal alkynes. | |
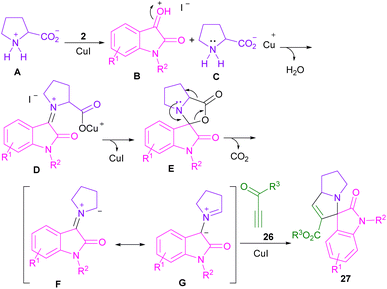 |
| Scheme 11 Possible mechanism for reaction of isatin, L-proline and terminal alkynes. | |
In 2017, the Meshram laboratory synthesized a new family of spiro-oxindole compounds and investigated their cytotoxic activity (Scheme 12).43 For this purpose, the reaction of isatin 2, amino acids 1, 28, but-2-ynedioates 29, and phenacyl bromide 30 was conducted with the assistance of MW power. To make oxindoles 31, isatins 2, L-proline 1, or 4-hydroxy proline 28 and but-2-ynedioates 29 were treated in H2O as a green solvent for 10 min under MW irradiation. Upon the addition of phenacyl bromide 30 in the reaction mixture, including isatin, L-proline and but-2-ynedioate, spiro products 32 were obtained at 100 °C after 15 min. The mechanism involved the initial condensation of α-amino acid 1 with isatin 2 to form azomethine intermediate B along with the CO2 extrusion. Later, 1,3-DC of B with but-2-ynedioates 29 afforded product 31. In another transformation, spiro compound 31 further reacted with phenacyl bromide 29 to render spiro product 32 (Scheme 13). Spiro-oxindolopyrrolizidines 35, 36 were also achieved in the Chen reaction (Scheme 14).44 In this work, α, γ-dialkylallenoate esters 33 were reported as dipolarophiles in the reaction with azomethine ylides and led to the endo-selective assembly of polycyclic spiro-oxindolopyrrolidines 35, 36. Two favorable transition states were proposed for the endo-cycloaddition reaction.
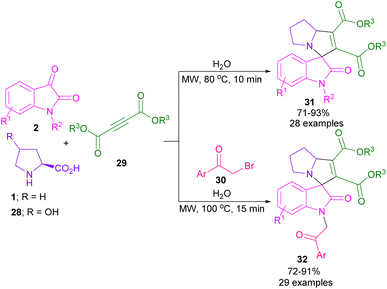 |
| Scheme 12 Reaction of isatin, amino acids, but-2-ynedioates and phenacyl bromide. | |
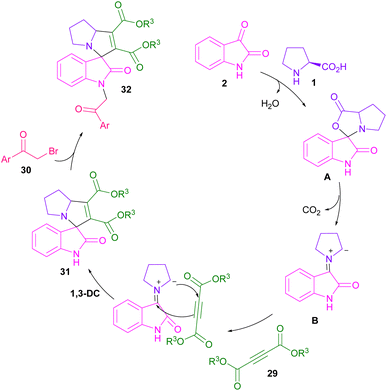 |
| Scheme 13 Possible mechanism for reaction of isatin, amino acids, but-2-ynedioates and phenacyl bromide. | |
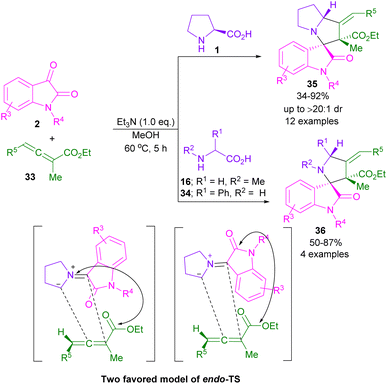 |
| Scheme 14 Reaction of isatin, amino acids, and α,γ-dialkylallenoate. | |
2.1.3. 1,3-Dipolar cycloadditions with indolines and indole as dipolarophiles. In 2020, Siitonen's group was able to present the total synthesis of a 3,3′-spiropyrrolidine oxindole alkaloid called isatin digotindoline C 39 via two steps of dehydrogenation and (3 + 2)-annulation (Scheme 15).45 For this purpose, isatin 2 and L-proline 1 were considered to produce azomethine ylide, and α,β-unsaturated methyleneindoline 37 was used as a dipolarophile for the cyclization with azomethine ylide. In the next step, anti-diastereomer of bisoxindole 39 was obtained in a ratio of 64% relative to other cis-diastereomer (36%). Also, 2-oxoindolino-3-ylidene acetophenones 40 were reported by Youseftabar-Miri et al. as dipolarophiles in the cycloaddition with isatin 2 and L-proline 1 (Scheme 16).46 Using DFT computations, the researchers demonstrated that the major product is kinetically favored.
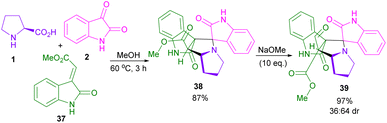 |
| Scheme 15 Reaction of isatin, L-proline and α,β-unsaturated methyleneindoline. | |
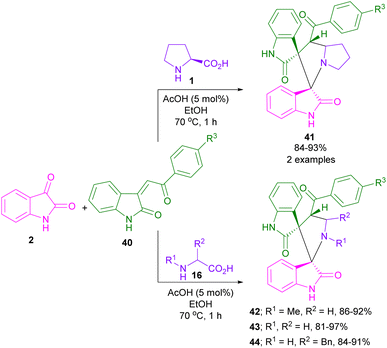 |
| Scheme 16 Reaction of isatins, amino acids and 2-oxoindolino-3-ylidene acetophenones. | |
2.1.4. 1,3-Dipolar cycloadditions with chalcones as dipolarophiles. In 2014, Lalitha and Revathy reported a three-component transformation, including isatin 2/acenaphthenequinone 49, amino acids and chalcones 45 to obtain spiro-oxindolopyrrolizidines 46–48 and spiro-oxindolothiapyrrolizidines 50, 51 (Scheme 17).47 L-Proline 1, L-thiaproline 7, and sarcosine 16 were used as amino acid precursors. D-Proline, a non-natural isomer of L-proline, did not give the desired product, because of the loss of the stereocentre in proline during the azomethine ylide formation. Acenaphthenequinone 49 resulted in higher yields in shorter reaction times compared to isatins. In a similar method by Jadidi and co-workers, a series of spiro-oxindolopyrrolizidines were synthesized starting from isatin, L-proline, and 3-(2-alkenoyl)-1,3-oxazolidin-2-ones as a dipolarophile.48 In their reaction performance, they used Cu(OTf)2 as a catalyst in combination with a chiral dinitrogen ligand. The employment of O-acryloylacridinediones as dipolarophiles in the reaction with azomethine ylides generated in situ from isatin and L-proline, led to spiro-pyrrolidine/pyrrolizidine scaffolds via (3 + 2)-annulation.49 In another work, Lipson et al. could synthesize spiro-oxindolopyrrolidines and pyrrolizidines from isatin, α-amino acids, such as L-proline, and sarcosine with asymmetric dipolarophiles, including acrylamides, and aroyl acrylic acids.50 Highly reactive aroyl acrylic acids lowered the reaction time (10–15 min).
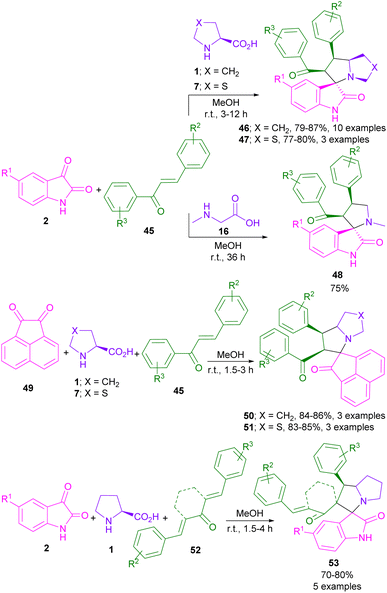 |
| Scheme 17 Reaction of amino acids, isatins, and chalcones. | |
Regio-, chemo-, and stereoselective synthesis of spiro-oxindolopyrrolizines 56 and spiro-oxindolopyrrolidines 57 was achieved in Shanmugam's work in 2015 (Scheme 18).51 In this context, amino acids, such as L-proline 1 and phenyl alanine 55 were smoothly reacted with isatin 2 and α-aroylidineketene dithioacetals 54 via (3 + 2)-cycloaddition strategy. The utility of this transformation was demonstrated by the gram-scale synthesis of the spiro-oxindole product in excellent yield (3.18 g, 99%) as well as the preparation of spiro-benzimidazole via the treatment of spiro-oxindolopyrrolizine with ortho-phenylenediamine. In the same year, Zhou et al. achieved chiral spiro-oxindolopyrrolizine from the reaction of isatin, L-proline and functionalized oxindole.52
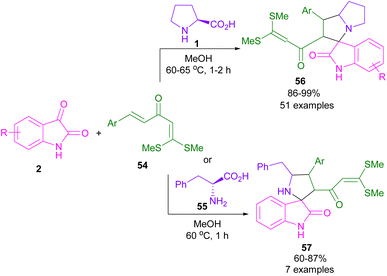 |
| Scheme 18 Reaction of isatin, L-proline/phenyl alanine and α-aroylidineketene dithioacetals. | |
In 2017, a sonication technique was used to synthesize furanyl spiro-oxindolopyrrolizidines and spiro-quinoxalinopyrrolizidines (Scheme 19).53 Isatin 2, L-proline 1, and various chalcones containing heteroaryl moieties 58 were applied as starting materials. Products were made by using three methods including ultrasonic, heating and fusion. A comparison between heating, and fusion conditions with ultrasonic conditions showed that not only the desired products were obtained in a much shorter reaction time, but also in an obviously higher chemical yield.
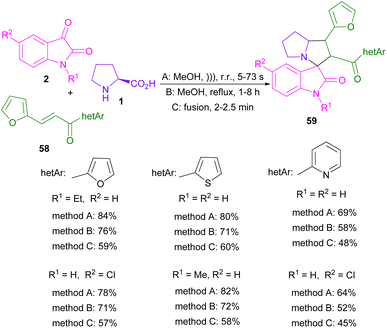 |
| Scheme 19 Reaction of isatin, L-proline, and chalcone. | |
In 2017, a strategy for the synthesis of piperazinyl-quinolinyl dispiro heterocycles was described by Gengan and co-workers (Scheme 20).54 In this regard, they performed the reaction of isatin 2 with L-proline 1/L-thioproline 7/sarcosine 16, and chalcone 60 to obtain spiro products 61, 62, 65. Spiro compounds 63, 64 and 66 were isolated when acenaphthenequinone was used instead of isatin. The reactions were set up in a MW irradiation reflux system. Examining the orbital interaction between the carbonyl group of 60 and azomethine ylide showed the endo-selective formation of regioisomer A, not exo-regioisomer B. In addition, molecular docking the fluorescence quench titration techniques were performed to investigate the binding ability of the obtained product with human serum albumin (HSA). L-Proline and L-thioproline together with isatin were employed in the synthesis of 3,3′-pyrrolidinyl-dispirooxindoles.55 In 2021, Zhu,56 Siva,57 and Velmathi58 reported in separate works, the cyclization of azomethine ylides derived from isatin and α-amino acids with (E)-3-cinnamoyl-2H-chromen-2-ones, chalcones and benzodioxole chalcones as dipolarophiles.
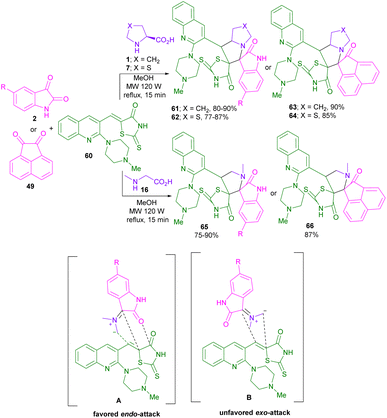 |
| Scheme 20 Reaction of isatin, amino acids, and α,γ-dialkylallenoate. | |
In 2018, the Barakat research group developed a three-component reaction, containing isatin 2, L-proline 1, and 3-acyl indoles 67 (Scheme 21).59 They synthesized a variety of novel spiro-oxindole derivatives 68 and investigated their anticancer properties against three cancer cell types (colorectal, hepatocellular carcinoma, and prostate). The protocol proceeded through azomethine ylide generation from isatin and L-proline, and 1,3-DC with the activated alkene 67. The spiro-oxindole compounds showed good cytotoxic activity and selectivity compared to cisplatin. Another synthesis of spiro-oxindoles from isatin, L-proline and 5-arylidene-2-thioxothiazolidin-4-one as a dipolarophile was presented by this group in the same year.60 DFT calculations, molecular docking and antimicrobial properties of the resulting products were also reported in this work.
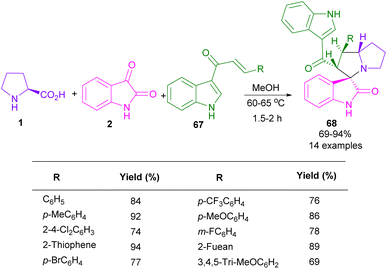 |
| Scheme 21 Reaction of isatin, L-proline, and 3-substituted indoles. | |
In 2019, Nagarapu et al. explored a new dipolarophile for the reaction with isatin 2 and L-proline 1 (Scheme 22).61 They conducted cycloaddition of azomethine ylide with (E)-3-(9-chloro-2,3-dimethyl-6,7-dihydro-5H-benzo[7]annulen-8-yl)-1-phenylprop-2-en-1-ones 69 to furnish the corresponding spiro-oxindole derivatives 70. They also studied anti-proliferative activities of the obtained products against five human cancer cell lines.
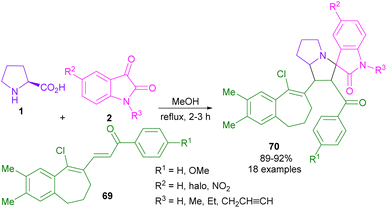 |
| Scheme 22 Reaction of isatin, L-proline, and substituted alkenes. | |
Deepthi and his team in 2020, developed a (3 + 2)-annulation approach for the synthesis of spiro-oxindolopyrrolidines in a deep eutectic solvent called acetylcholine iodide-ethylene glycol (ACI/EG) (Scheme 23).62 In this regard, isatin, amino acids, together with thiazolo[3,2-b]indole 71 were utilized in ACI/EG solvent to provide spiro-pyrrolidine oxindoles containing four stereocenters in high to excellent yields. Various amino acids, such as L-proline 1, L-thioproline 7, sarcosine 16, L-phenyl alanine 55, and L-tryptophan 72 were feasible in this method. All products were observed in the form of cis diastereoisomer.
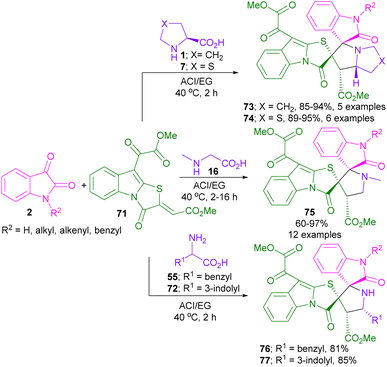 |
| Scheme 23 Reaction of isatin, amino acids and thiazolo[3,2-b]indole. | |
In 2022, the synthesis of spiro-oxindolopyrrolidines containing indole and pyridine rings 79–81 was presented by the Maheswarai library (Scheme 24).63 The reaction proceeded through the formation of azomethine ylides from α-amino acids and isatins, followed by (3 + 2)-cycloaddition with (E)-2-(1H-indole-3-carbonyl)-3-(pyridin-3-yl)acrylonitrile 78. Additionally, anticancer activity, and cytotoxic activity, as well as molecular docking of the obtained products were studied in this work.
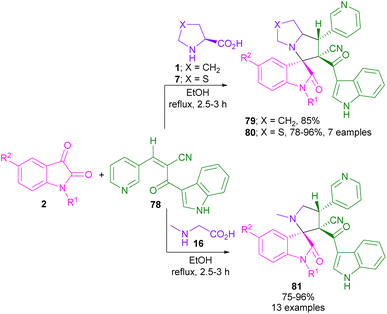 |
| Scheme 24 Reaction of isatins, amino acids and (E)-2-(1H-indole-3-carbonyl)-3-(pyridin-3-yl)acrylonitrile. | |
The Lamsabhi research team introduced the synthesis of a new class of spiro-oxindolopyrrolizidines and pyrrolidines through a four-component (3 + 2)-cyclization reaction (Scheme 25).64 In this regard, they treated isatins 2, and L-proline 1/L-valine 83 with p-substituted 1,3-bis(arylidene)tetral-2-ones 82 in EtOH as a solvent. According to the results of DFT calculations, the authors realized that due to the higher reactivity of the double bond at the 3-position relative to the double bond at the 1-position in enone 82, the reaction proceeded with high chemoselectivity and two products 84 and 85 were detected with high diastereomeric excess. It was said that the unsymmetrical reaction was carried out through the formation of two W-shaped and S-shaped azomethine ylide intermediates.
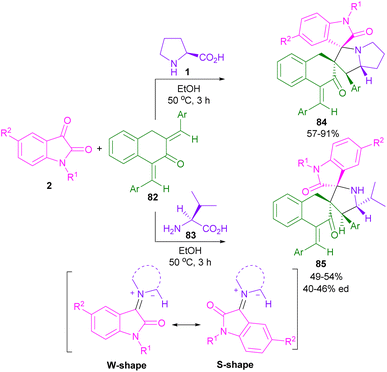 |
| Scheme 25 Reaction of isatins, amino acids and p-substituted 1,3-bis(arylidene)tetral-2-ones. | |
2.1.5. 1,3-Dipolar cycloadditions with quinolines, and quinazolines as dipolarophiles. In 2014, Maurya and Kamal et al. performed a multi-component reaction of isatins 2, L-proline 1 and 2-styrylquinazolin-4(3H)-ones 86 to make spiropyrrolidine-quinazolinones 87 (Scheme 26).65 The reaction was carried out through the initial interaction between isatin and amino acid towards azomethine ylides, which then underwent stereoselective 1,3-DC with alkene 86. The protocol takes advantage of high atom-economic, good product yield, and broad substrate tolerance. A variant of the same method was reported by Perumal et al., involving 1,3-DC of azomethine ylides with alkyl indeno[2,1-b]quinoxalin-11-ylidene acetate 88 access to dispiropyrrolidine and dispiro-pyrrolizidine oxindoles.66 Sarcosine 16, L-proline 1, and L-thioproline 7 can be included in this transformation. 1,3-DC of isatin, amino acids, and quinolinyl dipolarophiles led to novel quinolinyl dispiro heterocyclic compounds 89, 90 (Scheme 27).67 Amino acids participated in the formation of azomethine ylide intermediates with isatins, which then underwent cycloaddition with alkene 88.
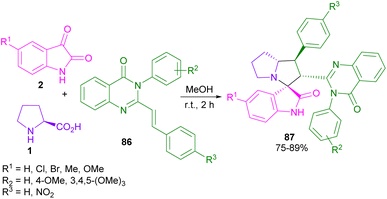 |
| Scheme 26 1,3-DC reactions of azomethine ylides and 2-styrylquinazolin-4(3H)-ones. | |
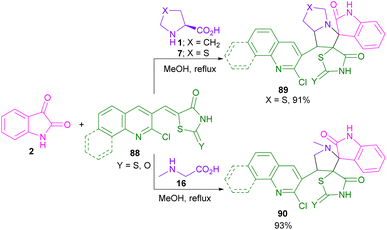 |
| Scheme 27 Cycloaddition of isatin, amino acids and quinolinyl dipolarophiles. | |
In 2019, for the synthesis of benzotriazoloquinolinyl spiro-oxindolopyrrolizidines 92, Basavoju and co-workers used the ultrasound method in the reaction of isatins 2, and L-proline 1 with 3-(2-(1H-benzo[d][1,2,3]triazol-1-yl)quinolin-3-yl)-1-phenylprop-2-en-1-one 91 (Scheme 28).68 Screening of organic solvents, such as MeOH, EtOH, 1,4-dioxxane, and MeCN led to products in moderate yields under conventional conditions. While, the use of ionic liquids, like [Bmim]BF4 and [Bmim]HSO4 as green solvents could increase the reaction rate and product yield. Consequently, the best result was obtained in the presence of [Bmim]BF4 and ultrasonic. Lastly, they evaluated the antimycobacterial and anti-proliferative activities of some of the obtained products.
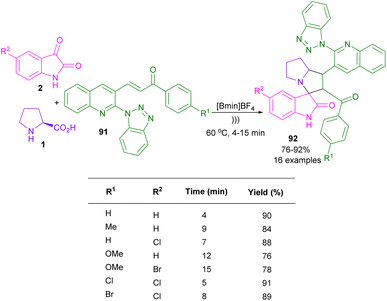 |
| Scheme 28 Reaction of isatin, L-proline and quinoline dipolarophile. | |
2.1.6. 1,3-Dipolar cycloadditions with cyclopropenes and azirines as dipolarophiles. In 2017, the synthesis of unprecedented 3-spiro[cyclopropa[a]pyrrolizine]oxindoles and 3-spiro[3-azabicyclo[3.1.0]hexane]oxindoles from (3 + 2)-cycloaddition of substituted isatins, α-amino acids and cyclopropenes 93 was presented in the Boitsov work (Scheme 29).69 Various amino acids, including L-proline 1, sarcosine 16, L-4-thiazolidine carboxylic acid 7, DL-pipecolinic acid 95, and dipeptide Gly-Gly 97, as well as benzylamine 96 as an amine precursor were participated in this transformation. Higher yields were observed when L-proline, or dipeptide Gly-Gly 97 were used to generate azomethine ylides. However, in all cases, high diasteroeselectivity were evident in products. Additionally, the anticancer activities of the resulting products were evaluated in this study. Sosnovskikh et al. utilized 3,3,3-trihalogen-1-nitropropenes as the activated alkene to react with azomethine ylides derived from isatin and L-proline.70 The cycloaddition led to a series of spiro-oxindoles comprising trihalomethyl and nitro functional groups.
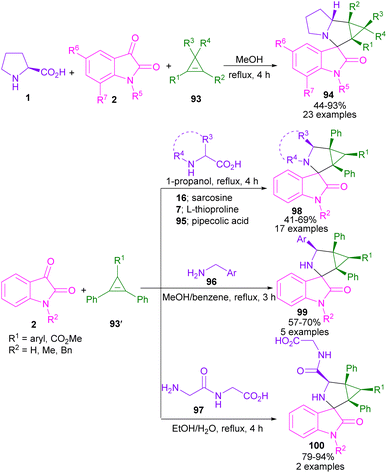 |
| Scheme 29 Reaction of isatins, α-amino acids and cyclopropenes. | |
In 2019, Han,71 Pavlovska,72 Srinivasan,73 and Deepthi74 research teams reported the synthesis of spiro-oxindolopyrrolizines staring from isatin, L-proline and different dipolarophiles. For example, Kanizsai and co-workers used 2H-azirines 101 with isatin 1 and α-amino acids 1, 102 to make 1,3-diazaspiro[bicyclo[3.1.0]hexane]oxindoles 103, 104 (Scheme 30).75 Various amino acids, such as D-2-phenylglycines bearing electron-donating, or electron-withdrawing groups, S-benzylcysteine, tryptophan, serine, glutamine, and aliphatic norleucine were compatible in this methodology. However, L-proline worked well in iPrOH as the optimal solvent at ambient temperature. The authors proposed a possible mechanism, involving the generation of azomethine ylide A from 1 and 2. Regioselective 1,3-DC of A with 2H-azirine 101 can occur through endo-TS, or exo-TS. However, no exocyclic product was detected, possibly due to the steric repulsion between the methyl group in azirine and the phenyl ring in oxindole. In the case of endo-cyclic compounds, the S-shaped conformation of A was sterically more favorable than the U-shaped conformation, so this conformer underwent endo-selective 1,3-DC to yield diastereomer 103 (Scheme 31).
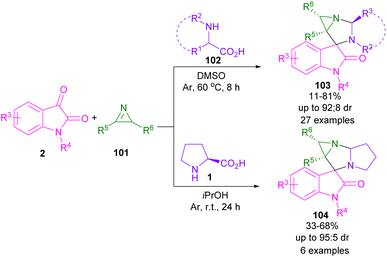 |
| Scheme 30 Reaction isatins, amino acids, and 2H-azirines. | |
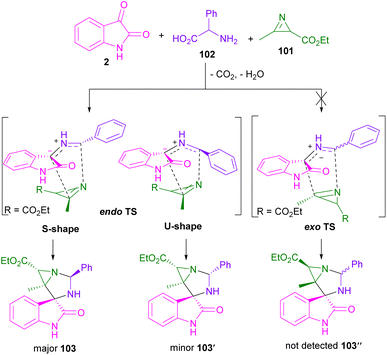 |
| Scheme 31 Possible mechanism for reaction isatins, amino acids, and 2H-azirines. | |
2.1.7. 1,3-Dipolar cycloadditions with ketones as dipolarophiles. In 2013, Raghunathan and co-workers reported a protocol for the construction of naphthyl dispiro-pyrrolidine/pyrrolizidine 106, 107 through 1,3-DC of isatin, or N-substituted isatin 2, sarcosine 16/L-proline 1, and naphthylidene tetralone 105 (Scheme 32).76 The reaction proceeded through the formation of azomethine ylides, which reacted with naphthylidene tetralone 105 as dipolarophiles. It was reported that anthrylidene tetralone was not feasible in this three-component reaction. Using the same method, Raghunathan et al. could prepare spiro-pyrrolidine/spiro-pyrrolizidine/spiro-thiazolidine-grafted macrocycles starting from isatin, or acenaphthenequinone and amino acids. They also achieved spiropyrrolidine-oxindole grafted 3-nitrochromanes by using 3-nitrochromenes instead of acenaphthenequinone.77,78 Several amino acids, such as L-proline, sarcosine, and thiazolidine-4-carboxylic acid could be incorporated in this synthetic method.
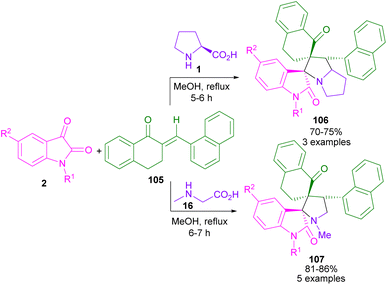 |
| Scheme 32 Reaction of isatins, and sarcosine/L-proline with naphthylidene tetralone. | |
Spiropyrrolidine and pyrrolizine derivatives were obtained from a multi-component reaction between 4-piperidone hydrochloride monohydrate 108, aryl aldehyde 18, isatin 2 and amino acids (Scheme 33).79 Sarcosine 16, L-proline 1, and phenylglycine 110 were used as the α-amino acid reactants. At the first step, 3,5-bis[(E)-arylmethyl idene]tetrahydro-4(1H)-pyridinones 109 were constructed from the reaction of 4-piperidone hydrochloride monohydrate 108 with 2.0 equivalent of aryl aldehyde 18. Then, the treatment of isatin 2 with α-amino acids 16, 110, or 1 led to N-methyl spiro-pyrrolidines 111, N-α-phenyl spiropyrrolidines 112, or spiro-pyrrolizines 113, respectively. In addition, the evaluation of anti-proliferative activities of the resulting products was also studied in this work.
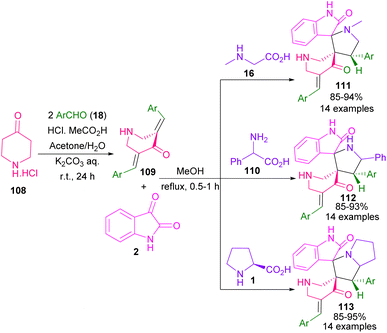 |
| Scheme 33 Synthesis of spiropyrrolidine and pyrrolizine derivatives. | |
In 2020, Khan et al. used Cu(OAc)2 and TEMPO to catalyze the dehydrogenation of alkylated ketones to act as an activated alkene in 1,3-DC with azomethine ylides (Scheme 34).80 In this method, L-proline and sarcosine were used as the α-amino acid components. Various alkylated ketones 114, quinolinyl-alkylated ketones 115 and 116 were well tolerated in this cycloaddition transformation. The reaction proceeded through a radical pathway in the dehydrogenation of alkylated ketone, followed by an ionic pathway in (3 + 2)-annulation with azomethine ylide. Finally, antioxidant, and anti-diabetic activities of the spiro products were investigated in this work. In another report by the Khan team, they again employed Cu(OAc)2/TEMPO as an efficient catalytic system for the dehydrogenative Friedlander annulation of secondary alcohols, followed by C(sp3)-H functionalization by primary alcohols, and subsequent regioselective 1,3-DC with azomethine ylids (Scheme 35).81 Considering the mechanism in Scheme 36, from the interaction of active TEMPO with Cu(II) ions, TEMPOH and Cu(I) species were generated, which transferred an electron to 121. Ketone A which formed through this process, reacted with alkyl acetoacetate 123 to produce methyl quinoline B after the dehydration in a deep eutectic solvent (DES). In this procedure, DMU-tartaric acid was used as a green DES solvent. Next, C was reacted with aldehyde to obtain 2-strylqunolines D. At this time, the hydrogenation of D by Cu(l) ions could provide product 125. On the other hand, azomethine ylide G was formed from the condensation of isatin 2 with L-proline 1. Finally, product 6 was obtained by cycloaddition of G with D.
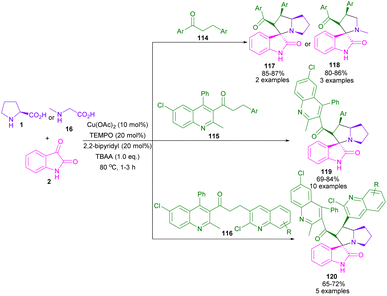 |
| Scheme 34 Cu-catalyzed reaction of isatin, amino acids and alkylated ketones. | |
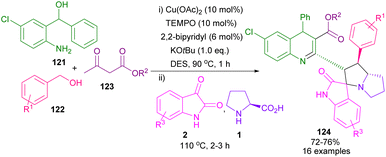 |
| Scheme 35 Reaction of secondary, and primary alcohols with isatin, and L-proline. | |
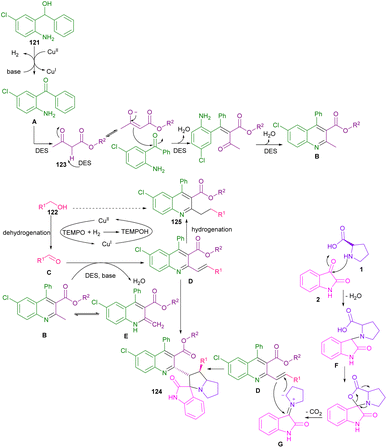 |
| Scheme 36 Tentative mechanism for reaction of secondary, and primary alcohols with isatin, and L-proline. | |
2.1.8. 1,3-Dipolar cycloadditions with azolidines and oxazoles as dipolarophiles. In 2016, the Menéndez research group made 2-pyrrolin-5-ones 125 from primary amines, 1,3-dicarbonyl compounds, and 2-bromoesters and successfully applied them in (3 + 2)-cyclization reaction with isatin 2 and α-amino acids, such as L-proline 1, and sarcosine 16 (Scheme 37).82 A series of spiro-pyrrolidines 126, 127 were synthesized in acceptable yields under MW irradiation at 100 °C for 1 h. It should be noted that sarcosine as an amino acid component is a better reactant due to the less steric hindrance in comparison with L-proline. However, in both resulting products, only the endo structure was constructed during the regioselective mechanism.
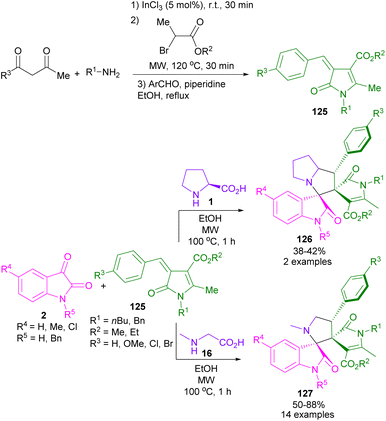 |
| Scheme 37 Reaction of isatins, amino acids and 2-pyrrolin-5-ones. | |
In 2018, Chowhan and co-workers found that Graphene oxide (GO) can catalyze the cycloaddition between azomethine ylides generated from indeno quinoxalinone (11H-indeno[1,2-b]quinoxalin-2-one) 129 and L-proline 1, with 3-methyl-4-nitro-5-alkenylisoxazole 128 as a dipolarophile (Scheme 38).83 Spiro-oxindoles 131 were also obtained from isatin 2, L-proline 1, and substrate 128 in the presence of the GO catalyst. The protocol has the advantages of low catalyst loading with recyclability, high product yields, broad functional group tolerance, the performance of the reaction at room temperature in a short time, and the gram-scale synthesis of the spiro products.
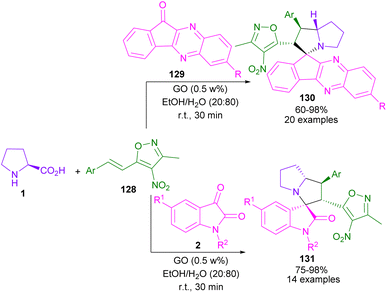 |
| Scheme 38 GO-catalyzed reaction of azomethine ylide with dipolarophiles. | |
In 2021, a one-pot three-component synthesis of aza-spirocyclic pyrazolones was performed by Tiwari and his team (Scheme 39).84 In this method, several amino acids, including L-proline 1, thiazolidine-4-carboxylic acid 7, thiazolidine-2-carboxylic acid 132, and pipecolic acid 95 were smoothly reacted with aryl aldehydes 18 to form azomethine ylide intermediates for further annulation with alkylidenes pyrazolones 133. The evaluation of azetidine-2-carboxylic acid and 1,2,3,4-tetrahydroisoquinoline-3-carboxylic acid as an amino acid precursor was not effective. In the case of aldehydes, electron-rich and electron-poor aldehydes, heteroaromatic aldehydes as well as polycyclic aldehydes were well tolerated in this transformation. A good result was also obtained in the gram-scale synthesis of the spiro product (4.25 g, 86% yield).
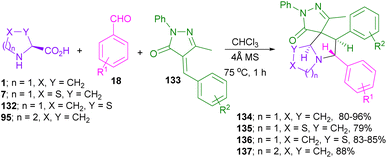 |
| Scheme 39 Reaction of aryl aldehydes, amino acids and unsaturated alkylidenes. | |
2.1.9. 1,3-Dipolar cycloadditions with chromenes and naphthalenes as dipolarophiles. In 2013, Reddy and Yuvaraj demonstrated that 1,3-DC of isatin, and amino acids (sarcosine, L-proline, and DL-pipecolinic acid) with Baylis–Hillman adducts 138 can lead to spiro-pyrrolidine oxindoles 139, 140 (Scheme 40).85 Two regioisomers were made from 1,3-DC of dipolarophile 138 with azomethine ylide A with the isomer preference in path I. This phenomenon was due to the energetically favored interaction between the HOMO orbital of a dipole with the LUMO orbital of a dipolarophile compared to the HOMO orbital of a dipolarophile and the LUMO orbital of a dipole. The use of dimethyl 2-(alkyl-, or arylthio)fumarates as a dipolarophile in the reaction with L-proline, and isatin led to the stereo-, and regioselective synthesis of spiro-oxindolopyrrolizidine, or pyrrolidine derivatives.86 Whereas, dimethyl 2-(aryloxy)fumarates as a dipolarophile resulted in a mixture of regioisomers. The reaction was carried out in EtOH, or MeCN as a solvent in a short reaction time (<5 min).
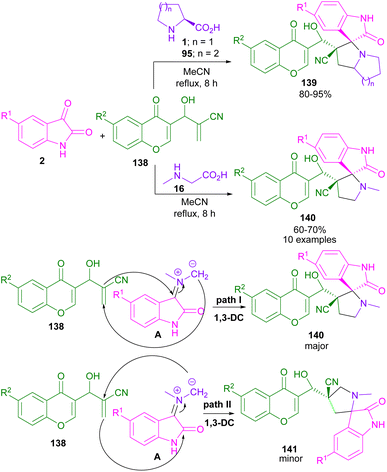 |
| Scheme 40 Reaction of isatin, amino acids and with Baylis–Hillman adduct. | |
In 2023, a microwave-promoted (3 + 2)cycloaddition of azomethine ylide with 1,4-dihydro-1,4-epoxynaphthalene 142 was reported by Zhao et al. (Scheme 41).87 Novel oxygen-bridged spiro-oxindole compounds were constructed in a very short time under MW irradiation. In addition to L-proline, a large spectrum of amino acids, including L-alanine, L-phenylalanine, 4-iodo-L-phenylalanine, L-homophenylalanine, L-tyrosine, L-methionine, L-lysine, L-leucine, and L-thiproline were well tolerated in this green synthetic methodology. L-Isoleucine, and trans-4-cyclohexyl-L-proline resulted in two diastereomers. While L-serine, L-piperidine-2-carboxylic acid, and peptide L-Ala-L-Ala-OH were not workable.
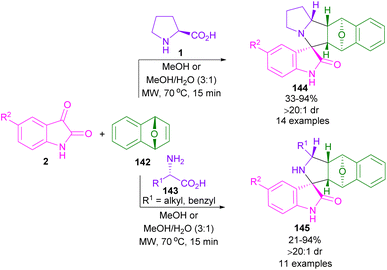 |
| Scheme 41 Reaction of isatin, amino acids and 1,4-dihydro-1,4-epoxynaphthalene. | |
2.1.10. 1,3-Dipolar cycloadditions with S/Se/P/EWG-substituted alkenes as dipolarophiles. In 2015, the Wu team explained a procedure for the assembly of a new library of spiro-indolopyrrolizine, spiro-indoloindolizine, and spiro-indolopyrrolidine gem-bisphosphonates (Scheme 42).88 In this regard, they treated isatin with amino acids, such as L-proline 1, or pipecolic acid 95 to generate azomethine ylides in the presence of montmorllonite catalyst for further reaction with tetraethyl vinylidenebis(phosphonate) 146. According to the mechanism in Scheme 43, azomethine ylide E was formed through the release of H2O and CO2. Due to the stability of the negative and positive charges by the carbonyl unit and the electron lone pair of the nitrogen atom, E is the most stable resonance form that can participate in 1,3-DC with alkene 146 to yield product 147.
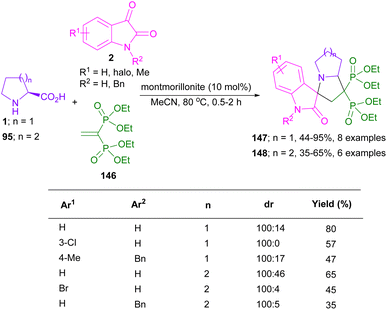 |
| Scheme 42 Reaction of isatins, tetraethyl vinylidenebis(phosphonate), and amino acids. | |
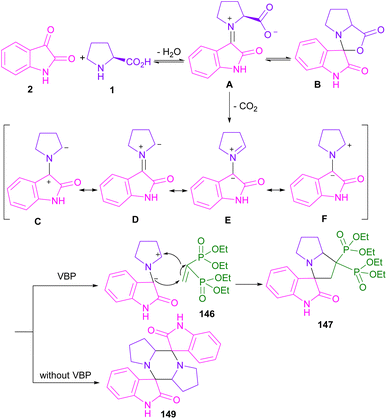 |
| Scheme 43 Plausible mechanism for reaction of isatins, tetraethyl vinylidenebis(phosphonate), and amino acids. | |
Vinyl selenones 150 served as an efficient dipolarophile in (3 + 2)-cycloaddition with azomethine ylide to synthesize a wide variety of spiro-oxindolopyrrolizine derivatives 152–155 (Scheme 44).89 The selenonyl group was able to activate the alkene and released as a leaving group in the form of benzeneseleninic acid. Several amino acids, such as L-proline 1, L-thioproline 7, trans-4-hydroxy-L-proline 151 and sarcosine 16 worked well in the condensation with isatins. In a variant of the same method, phenyl vinyl sulfones 156 were applied in 1,3-DC with isatins and amino acids to give spiro-oxindole-based phenylsulfones 157–159 (Scheme 45).90 L-Proline 1, syn-4-hydroxy-L-proline 151, and L-thioproline 7 as amino acids were participated in the reaction with substituted isatins to produce azomethine ylides. The final spiro products exhibited antiviral activity against SARS-CoV-2.
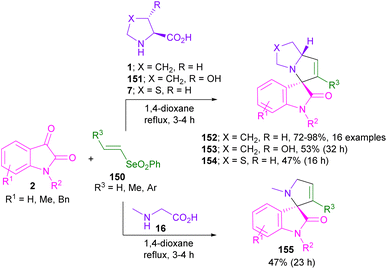 |
| Scheme 44 Reaction of isatin, amino acids and vinyl selenones. | |
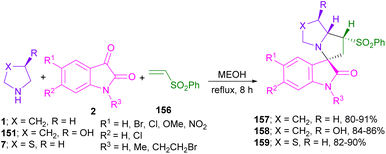 |
| Scheme 45 Reaction of isatin, amino acids and phenyl vinyl sulfones. | |
In 2022, conjugated arylcyanosulfones 160 were applied by Chen and Wang et al. for a 1,3-DC reaction with isatin and L-proline (Scheme 46).91 Various 3,3′-pyrrolidinyl-spiro-oxindole structures were obtained depending on the steric effect between electron-withdrawing groups (EWG) and substituents (R3) of the electrophilic substrates 160 in the formation of the final product. Therefore, the presence of p-toluenesulfonyl (Ts) as the EWG led to the formation of diastereomer 161 and 162 in the presence of L-proline, or L-thioproline. The replacement of Ts with CO2Et gave diastereomer 163, or 164. The spiro products 165 were isolated when pipecolic acid was reacted with N-methyl isatins 2 and alkenes 160 bearing CO2Et as the EWG. In contrast, the reaction was not significantly affected by the electronic effects of the functional groups on the substrates.
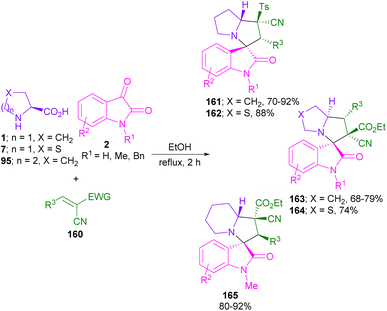 |
| Scheme 46 Reaction of isatins, amino acids and conjugated arylcyanosulfones. | |
2.2. 1,3-Dipolar cycloadditions of proline and aldehydes with dipolarophiles
2.2.1. 1,3-Dipolar cycloadditions with diones as dipolarophiles. In 2016, Yang and co-workers found that the microwave can accelerate the multi-component reaction of L-proline, aryl/heteroaryl aldehydes, and 1,3-diketones (Scheme 47).92 Two different dipolarophiles, such as 4-hydroxycoumarins 166 and 2-arylmethylene-indene-1,3-diones 167 were employed to make pyrrolizidines and pyrrolizinones. It should be noted that the performance of the multi-component reaction in the presence of 1,3-indanedione 166 using thermal conditions resulted in lower yields of products at longer reaction times (15% of 169, 15% of 170 after 1 h). This transformation was accomplished via in situ generation of azomethine ylide from L-proline and aryl aldehyde, followed by (3 + 2)-cycloaddition in the presence of 167, or Michael addition using 166 as a dipolarophile.
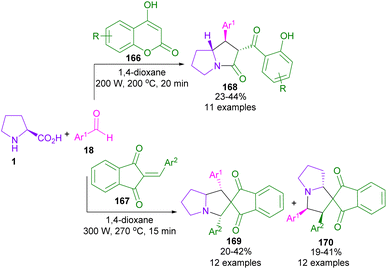 |
| Scheme 47 Reaction of L-proline, aryl aldehydes, and 1,3-diketones. | |
In 2016, the Askri and Kumar research groups used isatin and L-proline to synthesize spiro-oxindolopyrrolizidine derivatives through the reactions of azomethine ylides with (E,E)-1,3-bis(arylidene)indan-2-one,93 or acrylonitrile/methyl acrylate94 as a dipolarophile. Meantime, Khurana et al. presented a one-pot five-component reaction for the assembly of triazolyl spiro-cyclic oxindole frameworks (Scheme 48).95 In their protocol, isatin 2, L-proline 1, aryl azides 172, aryl aldehydes 18, and acetylacetone 171 were used as starting reactants and the reaction was carried out in the presence of DBU as a catalyst. The authors suggested a tentative mechanism for this five-component reaction, involving the formation of triazole A via the interaction of acetylacetone and azide in the presence of a base. Then, A was subjected to the aldol condensation in the presence of aryl aldehyde to form chalcone B. At the same time, the condensation of isatin with L-proline, followed by the decarboxylation yielded D, which then underwent (3 + 2)-cycloaddition with B through two possible pathways. In path I, the intermediate was stabilized by a secondary orbital interaction between the double bond of the triazole ring and the carbonyl group of isatin in TS, which afforded product 173. While, in path II, there was no such stabilization and product 174 was not observed in the reaction (Scheme 49).
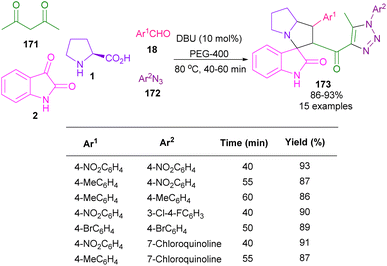 |
| Scheme 48 Multi-component reaction of isatin, L-proline, aryl azides, aryl aldehydes, and acetylacetone. | |
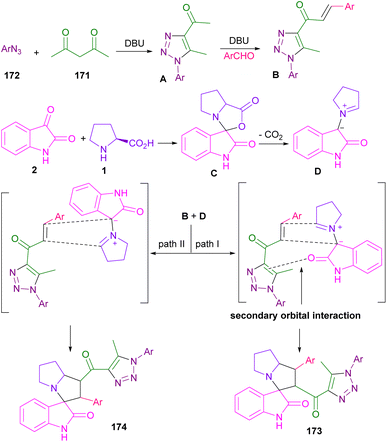 |
| Scheme 49 Tentative mechanism for reaction of isatin, L-proline, aryl azides, aryl aldehydes, and acetylacetone. | |
An elegant multi-component strategy, including dione-ninhydrin 175, ortho-phenylenediamine 177, L-proline 1/sarcosine 16, aryl aldehyde 18 and 3-cyanoacetyl indole 176 for the synthesis of spiro-pyrrolidines and spiro-pyrrolizidines 178 was disclosed by Zhu and co-workers in 2018 (Scheme 50).96 They treated these five reactants in EtOH as a benign solvent under reflux conditions to achieve polycyclic spiro compounds in a one-pot fashion. The reaction started with the condensation of ninhydrin 175 with diamine 177 to give indenoquinoxaline-11-one A, which could react with amino acid 1 to obtain azomethine ylide B. Meantime, Knoevenagel reaction between indole 176 and aldehyde 18 gave adduct C, which underwent 1,3-DC with B to deliver product 178 (Scheme 51). Another use of dione-ninhydrin and isatin in the generation of azomethine ylides from amino acids was observed in the Koodlur report.97 In this work, 1,3-DC was carried out in the presence of isatin/dione-ninhydrin, amino acids (L-proline, L-thioproline, or sarcosine), and ethynyl azaindole as a dipolarophile.
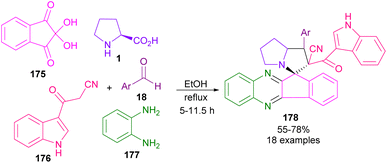 |
| Scheme 50 Reaction of ninhydrin, 1,2-phenylenediamine, amino acids, 3-cyanoacetyl indoles and aryl aldehydes. | |
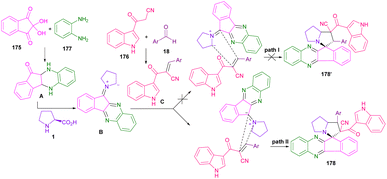 |
| Scheme 51 Proposed mechanism for reaction of ninhydrin, 1,2-phenylenediamine, amino acids, 3-cyanoacetyl indoles and aryl aldehydes. | |
2.2.2. 1,3-Dipolar cycloadditions with indolines as dipolarophiles. In 2018, Mukhopadhyay and Basu found that spiro-oxindolopyrrolizidines 179 could be prepared from the 1,3-DC of azomethine ylides with (E)-3-(2-oxo-2-arylethylidene)indolin-2-ones 40 as dipolarophiles (Scheme 52).98 For the synthesis of dipoarophiles, they first treated isatin and internal alkynes in the presence of FeCl3 as a catalyst. In the next stage, the cycloaddition reaction was conducted in the presence of L-proline, aryl aldehydes, and activated alkene 40 in DMF as the optimal solvent. The reaction efficiency was moderate in the EtOH, MeCN, and MeNO2 and very low in toluene and THF as solvents.
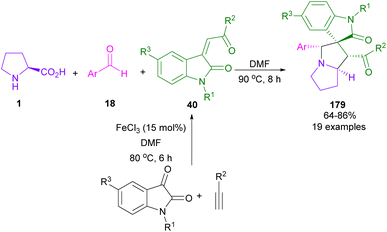 |
| Scheme 52 Reaction of isatin, L-proline, and (E)-3-(2-oxo-2-arylethylidene)indolin-2-ones. | |
2.2.3. 1,3-Dipolar cycloadditions with chalcones as dipolarophiles. In 2015, Banerji and Gayen were able to synthesize a new class of spiro-pyrrolizidine compound 181 by the azomethine ylide formation from aryl aldehydes 18 and L-proline 1 (Scheme 53).99 This intermediate then reacted with diaryl cyclohexanone under regio-, and stereoselective cyclization. It was seen that one of the double bonds in the dipolarophile remain unaffected. A year later, Banerji et al. used aryl aldehydes 18 to form azomethine ylides from L-proline 1 (Scheme 54).100 In the next phase, they treated chalcone 45 with these imine intermediates to make tri-substituted pyrrolizidines and di-substituted oxazolidines. Pyrrolizidines 182 were obtained as the main products from (3 + 2)-cycloaddition of azomethine ylide with chalcone, while oxazolidines 183 were identified as by-products because of cycloaddition of azomethine ylides with the second molecule of aryl aldehyde. DFT calculations revealed an exo-TS as the favored TS in 1,3-DC of azomethine ylide to chalcone.
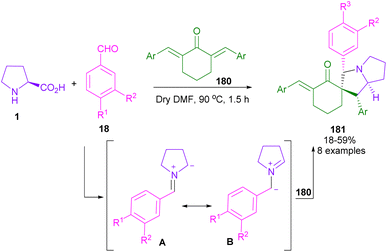 |
| Scheme 53 Cycloaddition of isatin, amino acids and dibenzylidene cyclohexanone. | |
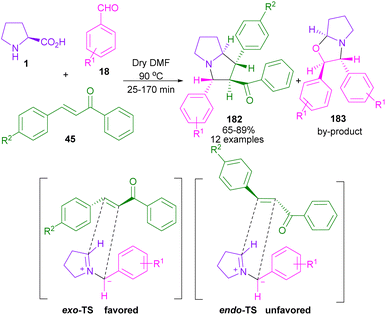 |
| Scheme 54 Reaction of arylaldehydes, L-proline and chalcones. | |
3. 1,3-Dipolar cycloadditions for synthesis of fused-oxindoles
3.1. 1,3-Dipolar cycloadditions of proline and isatins with dipolarophiles
In 2020, Reddy and co-workers described a microwave-assisted 1,3-DC of azomethine ylide with Baylis–Hillman adducts 184 (Scheme 55).101 In this strategy, a new class of fused 2-quinolinones 185 were constructed from isatins 2, α-amino acids 1, or 95, and the activated alkenes 184 under MW irradiation. CuI could catalyze the condensation of isatin and amino acid to azomethine ylide. Comparison of conventional and MW methods showed that the reaction in an oil bath takes 12 h, while 10 min in the presence of MW is sufficient to complete the reaction.
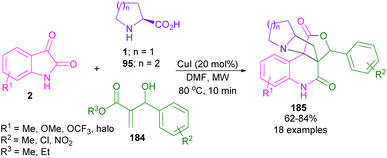 |
| Scheme 55 Reaction of isatin, amino acids and Baylis–Hillman adducts. | |
3.2. 1,3-Dipolar cycloadditions of proline and aldehydes with dipolarophiles
In 2013, Perumal et al. developed a three-segment approach, including aryl aldehydes, 3-phenyl-5-isoxazolone, and secondary amino acids (Scheme 56).102 When L-proline 1 reacted with aryl aldehyde 18 and 3-phenyl-5-isoxazolone 186, pyrrolizidinones 187 were obtained, while pyrrolidinone scaffolds 188 were isolated using sarcosine 16 as an amino acid reactant. In another work, the reaction of amino acids 1, or 95, aldehydes 18, or 190 and 2-substituted coumarins 189 resulted in the formation of 1-benzopyrano[3,4-c]pyrrolidine frameworks 191, 192 through the 1,3-DC process (Scheme 57).103 Various benzopyranopyrrolidines were prepared from the addition of anti-ylide of A to coumarin.
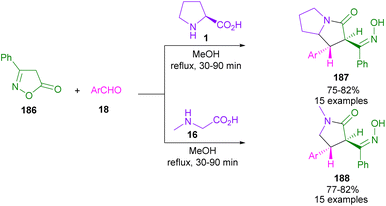 |
| Scheme 56 Reaction of aryl aldehydes, 3-phenyl-5-isoxazolone and secondary amino acids. | |
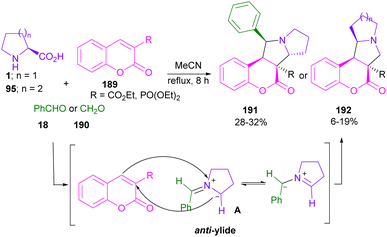 |
| Scheme 57 Reaction of amino acids, aldehydes and coumarins. | |
In 2014, Yang and co-workers developed a microwave-promoted reaction of L-proline with aryl aldehydes, and 1,3-diketones, such as 4-hydroxycoumarin and pyran (Scheme 58).104 Interestingly, when 2.0 equivalents of aryl aldehyde 18 were used, a series of highly functionalized pyrano[2,3-b]pyrroles 194, 195 were constructed, while the stereoselective formation of pyrrolizinones 196 was achieved in the presence of 4.0 equivalents of thiophene, or furan carbaldehyde 18 under MW conditions. Not only proline 1, but also pipecolic acid 95 was included in this α,β-difunctionlization reaction. The researchers showed that azomethine ylide can be produced by MW-promoted decarboxylative coupling of L-proline and aryl aldehyde. This intermediate then underwent proton-mediated isomerization to enamine C, or D. Depending on the concentration of aryl aldehyde, enamine can give two different products. Enamine D was subjected to the attack of A, followed by the cyclization to afford pyrano[2,3-b]pyrrole 194. While the condensation of enamine D with aryl aldehyde and subsequent conjugate addition with A delivered pyrrolizinone 196 (Scheme 59).
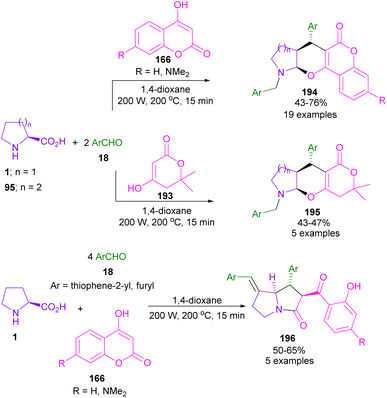 |
| Scheme 58 Microwave-promoted reaction of amino acids, aldehydes, and 1,3-diketones. | |
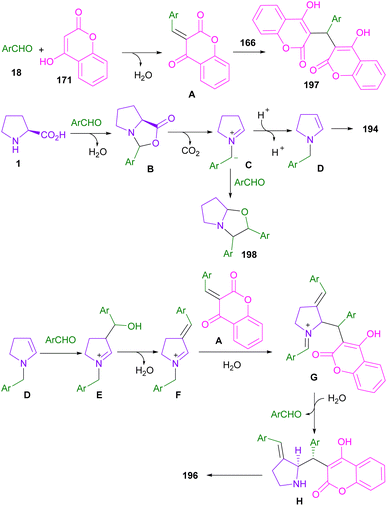 |
| Scheme 59 Proposed mechanism for MW-promoted reaction of amino acids, aldehydes, and 1,3-diketones. | |
In 2016, Higashi and Arimitsu et al. reported the synthesis of trifluoromethylated pyrrolizidines 200 from L-proline 1, aryl aldehydes 18, and β-trifluoromethyl acrylamide 199 (Scheme 60).105 With the assistance of DFT calculations, the researchers were able to identify the reason for excellent diastereoselectivity (>20
:
1) and moderate regioselectivity (up to 5.9
:
1) observed in products. The use of L-proline and aldehydes in the synthesis of bicyclic hexahydropyrrolo[1,2-c]oxazol-1-ylphosphonates starting from acylphosphonates was also reported in Subasi's report.106
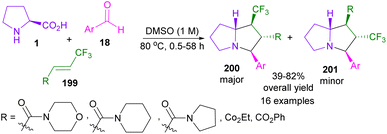 |
| Scheme 60 Reaction of L-proline, aldehydes, and β-trifluoromethyl acrylamide. | |
In 2018, the Wei laboratory succeeded in synthesizing multi-functionalized pyrrolizidines 204 through the 1,3-DC between aryl aldehydes 18, L-proline 1, and acetone 203 (Scheme 61).107 In addition to L-proline 1, (2S,4R)-4-OTBS-proline 202 was amenable to this transformation. The reaction involved the formation of enamine A from oxazolidinone. The aldol addition and the aldol condensation can both occur to render B and D, respectively. The aldol condensation pathway was promoted by MgSO4. In the next step, the iminium salt E was produced from the condensation of L-proline and α,β-unsaturated ketone D. The decarboxylation of E resulted in azomethine ylide F, followed by 1,3-DC with D to yield the endo-product 204, or 205 (Scheme 62).
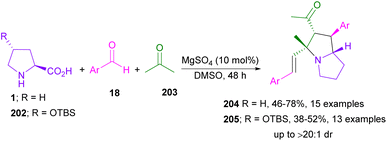 |
| Scheme 61 Reaction of amino acids, aryl aldehydes and ketone. | |
 |
| Scheme 62 Possible mechanism for reaction of amino acids, aryl aldehydes and ketone. | |
A year later, in another work, Yoo et al. used L-proline 1 and aryl aldehydes 18 for the generation of azomethine ylides A, or A′ for further reaction with a series of N-aromatic zwitterions 206 via (3 + 2)-annulation reaction (Scheme 63).108 Polycyclic fused pyrrolizidines 207 were constructed through the formation of azomethine ylide A′ against A, followed by intramolecular cyclization with 206 to obtain intermediate B. It was obvious that the electron deficiency of aryl aldehyde (Ar = 4-NO2C6H4) causes stability in intermediate A′.
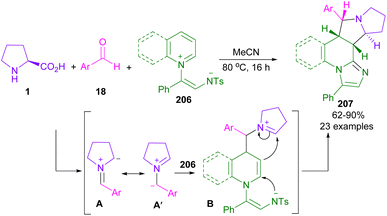 |
| Scheme 63 Reaction of N-aromatic zwitterions, aldehydes, and amino acids. | |
In 2023, Nyerges and co-workers developed an approach for the preparation of unprecedented pyrrolo[3,4-c]quinolinone derivatives from azomethine ylides (Scheme 64).109 In this procedure, L-proline 1, or sarcosine 16 were treated with aryl aldehyde 18, or formaldehyde 190 to produce azomethine ylide. Then, this dipole intermediate was added to 3-nitro-2(1H)-quinolinone 209 to give an anti-endo isomer of 3a-nitro-4-oxo-1,2,3,3a,5,9b-hexahydropyrrolo[3,4-c]quinoline-4-ones 210, and 211 in a regio-, and stereoselective manner as the only cycloadduct. However, aryl aldehyde with a large ortho-substituent near the reaction centre showed a direct effect on the stereochemistry of the cycloaddition, where a syn-endo isomer was also observed as the other diastereomer.
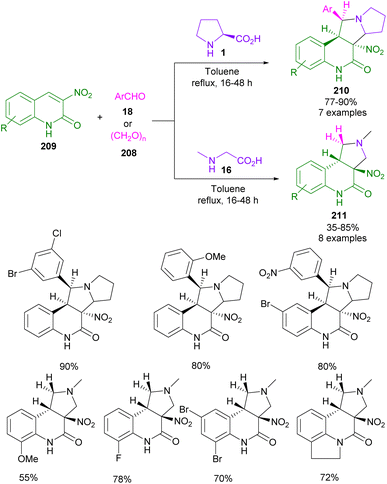 |
| Scheme 64 Reaction of isatin, amino acids and 3-nitro-2(1H)-quinolinone. | |
4. Conclusions
The remarkable features of L-proline have made this α-amino acid an appropriate candidate for 1,3-dipolar cycloaddition reactions. Due to the presence of carboxylic acid and amine functional groups, L-proline can easily react with 1,2-dicarbonyl compounds to produce azomethine ylide for further reaction with various organic dipolarophiles. In this context, we have covered important L-proline incorporations in 1,3-DC reactions. Most of these reactions are carried out under mild conditions without the need for catalysts, oxidants, or additives. Methanol and ethanol are commonly used organic solvents in these 1,3-DC reactions. In most reports, products are obtained in high yields with good regio-, and diastereoselectivity. Despite great advances in the azomethine ylide cycloadditions, various functionalized dipolarophiles for the preparation of enantioenriched bioactive spiro-oxindole scaffolds remain unexplored. Moreover, the combination of radical approaches, such as visible-light and electrochemistry with azomethine ylide reactions may lead to better results in the reaction rate and efficiency.
Conflicts of interest
There are no conflicts to declare.
Acknowledgements
The acknowledgements come at the end of an article after the conclusions and before the notes and references.
Notes and references
- S. Karlsson and H.-E. Högberg, Org. Prep. Proced. Int., 2001, 33, 103–172 CrossRef CAS.
- M. Kissane and A. R. Maguire, Chem. Soc. Rev., 2010, 39, 845–883 RSC.
- T. Hashimoto and K. Maruoka, Chem. Rev., 2015, 115, 5366–5412 CrossRef CAS PubMed.
- L. Maiuolo, V. Algieri, F. Olivito and A. De Nino, Catalysts, 2020, 10, 65 CrossRef.
- M. Breugst and H. U. Reissig, Angew. Chem., Int. Ed., 2020, 59, 12293–12307 CrossRef CAS PubMed.
- H. A. Döndas, M. de Gracia Retamosa and J. M. Sansano, Synthesis, 2017, 49, 2819–2851 CrossRef.
- R. Narayan, M. Potowski, Z.-J. Jia, A. P. Antonchick and H. Waldmann, Acc. Chem. Res., 2014, 47, 1296–1310 CrossRef CAS PubMed.
- G. Patel, A. R. Patel, S. Kheti, P. K. Sao, G. Rathore and S. Banerjee, Curr. Organocatal., 2023, 10, 180–208 CrossRef CAS.
- J. Adrio and J. C. Carretero, Chem. Commun., 2014, 50, 12434–12446 RSC.
- S. S. Panda, M. N. Aziz, J. Stawinski and A. S. Girgis, Molecules, 2023, 28, 668 CrossRef CAS PubMed.
- T. Das, R. Jana, S. Dubey, A. Pal, S. Roy, S. Sasmal and A. Tamrakar, New J. Chem., 2023, 47, 8997–9034 RSC.
- S. V. Kumar and P. J. Guiry, Chem.–An Euro. J., 2023, 29, e202300296 CrossRef CAS PubMed.
- S. Puri, I. Ahmad, H. Patel, K. Kumar and K. Juvale, Toxicol. in Vitro, 2023, 86, 105517 CrossRef CAS PubMed.
- D. Yan, J. Xu, X. Wang, J. Zhang, G. Zhao, Y. Lin and X. Tan, Int. J. Mol. Sci., 2022, 23, 4668 CrossRef CAS PubMed.
- S. A. El-Kalyoubi, A. Ragab, O. A. Abu Ali, Y. A. Ammar, M. G. Seadawy, A. Ahmed and E. A. Fayed, Pharmaceut., 2022, 15, 376 CrossRef CAS PubMed.
- N. Ye, H. Chen, E. A. Wold, P.-Y. Shi and J. Zhou, ACS Infect. Dis., 2016, 2, 382–392 CrossRef CAS PubMed.
- S. Askri, A. Dbeibia, C. Mchiri, S. Boudriga, M. Knorr, E. Roulland, O. Laprévote, N. Saffon-Merceron and R. Gharbi, Appl. Sci., 2021, 12, 360 CrossRef.
- N. Arumugam, K. I. Al-Shemaimari, M. Altaf, K. Ponmurugan, D. Premnath, S. Djearamane, L. S. Wong and S. Kayarohanam, J. King Saud Univ. Sci., 2023, 102996 Search PubMed.
- B. K. Yeung, B. Zou, M. Rottmann, S. B. Lakshminarayana, S. H. Ang, S. Y. Leong, J. Tan, J. Wong, S. Keller-Maerki and C. Fischli, J. Med. Chem., 2010, 53, 5155–5164 CrossRef CAS PubMed.
- P. Prasanna, K. Balamurugan, S. Perumal, P. Yogeeswari and D. Sriram, Eur. J. Med. Chem., 2010, 45, 5653–5661 CrossRef CAS PubMed.
- M. Shahidul Islam, A. Mohammed Al-Majid, E. Nageh Sholkamy, S. Yousuf, M. Ayaz, A. Nawaz, A. Wadood, A. U. Rehman, V. Prakash Verma and A. Motiur Rahman, ChemSelect, 2022, 7, e202203047 CAS.
- L. Szabados and A. Savouré, Trends Plant Sci., 2010, 15, 89–97 CrossRef CAS PubMed.
- B. List, Tetrahedron, 2002, 58, 5573–5590 CrossRef CAS.
- N. Suzuki, D. Mizuno, A. M. Guidote, S. Koyama, Y. Masuyama and M. Rikukawa, Lett. Org. Chem., 2020, 17, 717–725 CrossRef CAS.
- M. Rahman, A. Mukherjee, I. S. Kovalev, D. S. Kopchuk, G. V. Zyryanov, M. V. Tsurkan, A. Majee, B. C. Ranu, V. N. Charushin and O. N. Chupakhin, Adv. Synth. Catal., 2019, 361, 2161–2214 CrossRef CAS.
- B. Bdiri, B.-J. Zhao and Z.-M. Zhou, Tetrahedron Asymmetry, 2017, 28, 876–899 CrossRef CAS.
- X. Zhang, X. Ma and W. Zhang, Beilstein J. Org. Chem., 2023, 19, 1677–1693 CrossRef CAS PubMed.
- S. Mondal and S. Chowdhury, Adv. Synth. Catal., 2018, 360, 1884–1912 CrossRef CAS.
- T. Shao, X. Ban and Z. Jiang, Chem. Rec., 2023, 23, e202300122 CrossRef CAS PubMed.
- J. He, G. Ouyang, Z. Yuan, R. Tong, J. Shi and L. Ouyang, Molecules, 2013, 18, 5142–5154 CrossRef CAS PubMed.
- S. Kanchithalaivan, R. V. Sumesh and R. R. Kumar, ACS Comb. Sci., 2014, 16, 566–572 CrossRef CAS PubMed.
- H.-J. Wang, B.-W. Pan, W.-H. Zhang, C. Yang, X.-L. Liu, Z. Zhao, T.-T. Feng, Y. Zhou and W.-C. Yuan, Tetrahedron, 2015, 71, 8131–8139 CrossRef CAS.
- S. Gouthaman, S. Periyaraja and P. Shanmugam, Tetrahedron Lett., 2015, 56, 5920–5923 CrossRef CAS.
- S. Ponnuchamy, R. V. Sumesh and R. R. Kumar, Tetrahedron Lett., 2015, 56, 4374–4376 CrossRef CAS.
- S. Haddad, S. Boudriga, F. Porzio, A. Soldera, M. Askri, M. Knorr, Y. Rousselin, M. M. Kubicki, C. Golz and C. Strohmann, J. Org. Chem., 2015, 80, 9064–9075 CrossRef CAS PubMed.
- E. M. Hussein, S. A. Ahmed, N. E. Guesmi and K. S. Khairou, J. Chem. Res., 2017, 41, 346–351 CrossRef.
- M. Akhavan and A. Bekhradnia, RSC Adv., 2021, 11, 14755–14768 RSC.
- S. Askri, H. Edziri, M. B. Hamouda, C. Mchiri, R. Gharbi, H. H. Abd El-Gawad and M. M. El-Tahawy, J. Mol. Struct., 2022, 1250, 131688 CrossRef CAS.
- G. Lotfy, Y. M. A. Aziz, M. M. Said, H. El Sayed, H. El Sayed, M. M. Abu-Serie, M. Teleb, A. Dömling and A. Barakat, Bioorg. Chem., 2021, 117, 105427 CrossRef CAS PubMed.
- N. H. de Silva, S. Pyreddy, E. W. Blanch, H. M. Hügel and S. Maniam, Bioorg. Chem., 2021, 114, 105128 CrossRef CAS PubMed.
- V. Y. Korotaev, N. S. Zimnitskiy, A. D. Denikaev, A. Y. Barkov, I. B. Kutyashev and V. Y. Sosnovskikh, Chem. Heterocycl. Compd., 2021, 57, 81–91 CrossRef CAS.
- S. N. Singh, S. Regati, A. K. Paul, M. Layek, S. Jayaprakash, K. V. Reddy, G. S. Deora, S. Mukherjee and M. Pal, Tetrahedron Lett., 2013, 54, 5448–5452 CrossRef CAS.
- P. R. Mali, P. K. Shirsat, N. Khomane, L. Nayak, J. B. Nanubolu and H. Meshram, ACS Comb. Sci., 2017, 19, 633–639 CrossRef CAS PubMed.
- Y. Wang and Y. Chen, Tetrahedron Lett., 2017, 58, 1545–1547 CrossRef CAS.
- J. H. Siitonen, S. Lira, M. Yousufuddin and L. Kürti, Org. Biomol. Chem., 2020, 18, 2051–2053 RSC.
- F. Mataji, L. Youseftabar-Miri, M. J. Javan, E. Rajabbeigi and S. Hallajian, J. Mol. Struct., 2022, 1270, 133891 CrossRef CAS.
- K. Revathy and A. Lalitha, RSC Adv., 2014, 4, 279–285 RSC.
- F. Salahi, M. J. Taghizadeh, H. Arvinnezhad, M. Moemeni, K. Jadidi and B. Notash, Tetrahedron Lett., 2014, 55, 1515–1518 CrossRef CAS.
- R. Rajesh, M. Suresh, R. Selvam and R. Raghunathan, Tetrahedron Lett., 2014, 55, 4047–4053 CrossRef CAS.
- T. L. Pavlovskaya, F. G. Yaremenko, V. V. Lipson, S. V. Shishkina, O. V. Shishkin, V. I. Musatov and A. S. Karpenko, Beilstein J. Org. Chem., 2014, 10, 117–126 CrossRef PubMed.
- P. Dhanalakshmi, S. S. Babu, S. Thimmarayaperumal and S. Shanmugam, RSC Adv., 2015, 5, 33705–33719 RSC.
- Z. Dong, C. Yan, Y. Gao, C. Dong, G. Qiu and H. B. Zhou, Adv. Synth. Catal., 2015, 357, 2132–2142 CrossRef CAS.
- V. B. Nishtala, J. B. Nanubolu and S. Basavoju, Res. Chem. Intermed., 2017, 43, 1365–1381 CrossRef CAS.
- A. Murugesan, R. M. Gengan, R. Rajamanikandan and M. Ilanchelian, J. Mol. Struct., 2017, 1149, 439–451 CrossRef CAS.
- B. Lin, W.-H. Zhang, D.-D. Wang, Y. Gong, Q.-D. Wei, X.-L. Liu, T.-T. Feng, Y. Zhou and W.-C. Yuan, Tetrahedron, 2017, 73, 5176–5188 CrossRef CAS.
- P. Gong, Y. Ma, X. Wang, L. Yu and S. Zhu, Tetrahedron, 2021, 91, 132221 CrossRef CAS.
- C. Chithiraikumar, K. V. Ponmuthu, M. Harikrishnan, N. Malini, M. Sepperumal and A. Siva, Res. Chem. Intermed., 2021, 47, 895–909 CrossRef CAS.
- N. Nivetha, R. M. Martiz, S. M. Patil, R. Ramu, S. Sreenivasa and S. Velmathi, RSC Adv., 2022, 12, 24192–24207 RSC.
- A. Barakat, M. S. Islam, H. M. Ghawas, A. M. Al-Majid, F. F. El-Senduny, F. A. Badria, Y. A. M. Elshaier and H. A. Ghabbour, RSC Adv., 2018, 8, 14335–14346 RSC.
- A. Barakat, S. M. Soliman, A. M. Al-Majid, M. Ali, M. S. Islam, Y. A. Elshaier and H. A. Ghabbour, J. Mol. Struct., 2018, 1152, 101–114 CrossRef CAS.
- S. Kasaboina, R. Bollu, V. Ramineni, P. M. Gomedhika, K. Korra, S. R. Basaboina, U. D. Holagunda, L. Nagarapu, N. Dumala and P. Grover, J. Mol. Struct., 2019, 1180, 355–362 CrossRef CAS.
- V. Sathi, N. V. Thomas and A. Deepthi, Org. Biomol. Chem., 2020, 18, 7822–7826 RSC.
- S. Mayakrishnan, D. Kathirvelan, Y. Arun, K. Saranraj, C. Balachandran, S. Aoki, P. Yuvaraj and N. U. Maheswarai, New J. Chem., 2022, 46, 10089–10106 RSC.
- H. Gazzeh, F. Rouatbi, S. Chniti, M. Askri, M. Knorr, C. Strohmann, C. Golz and A. M. Lamsabhi, New J. Chem., 2022, 46, 19198–19212 RSC.
- R. A. Maurya, R. Nayak, C. N. Reddy, J. S. Kapure, J. B. Nanubolu, K. K. Singarapu and M. Ajitha, RSC Adv., 2014, 4, 32303–32311 RSC.
- S. Lanka, S. Thennarasu and P. T. Perumal, RSC Adv., 2014, 4, 2263–2266 RSC.
- G. S. Kumar, R. Satheeshkumar, W. Kaminsky, J. Platts and K. J. R. Prasad, Tetrahedron Lett., 2014, 55, 5475–5480 CrossRef.
- V. Pogaku, V. S. Krishna, C. Balachandran, K. Rangan, D. Sriram, S. Aoki and S. Basavoju, New J. Chem., 2019, 43, 17511–17520 RSC.
- A. S. Filatov, N. A. Knyazev, A. P. Molchanov, T. L. Panikorovsky, R. R. Kostikov, A. G. Larina, V. M. Boitsov and A. V. Stepakov, J. Org. Chem., 2017, 82, 959–975 CrossRef CAS PubMed.
- A. Y. Barkov, N. S. Zimnitskiy, V. Y. Korotaev, I. B. Kutyashev, V. S. Moshkin and V. Y. Sosnovskikh, Tetrahedron, 2016, 72, 6825–6836 CrossRef CAS.
- M. Zhu, Y. Han, C. Liu, W. Ma and C.-G. Yan, Chin. Chem. Lett., 2020, 31, 1554–1557 CrossRef CAS.
- T. L. Pavlovska, V. V. Lipson, S. V. Shishkina, V. I. Musatov, A. V. Borisov and A. V. Mazepa, Chem. Heterocycl. Compd., 2019, 55, 679–683 CrossRef CAS.
- V. Nookaapparao Gorli and R. Srinivasan, Synth. Commun., 2020, 50, 516–525 CrossRef CAS.
- S. Vidya, K. Priya, D. Velayudhan Jayasree, A. Deepthi and P. G. Biju, Synth. Commun., 2019, 49, 1592–1602 CrossRef CAS.
- A. Angyal, A. Demjén, V. Harmat, J. N. Wölfling, L. G. Puskás and I. Kanizsai, J. Org. Chem., 2019, 84, 4273–4281 CrossRef CAS PubMed.
- P. Saravanan, S. Pushparaj and R. Raghunathan, Tetrahedron Lett., 2013, 54, 3449–3452 CrossRef CAS.
- S. Purushothaman, R. Prasanna and R. Raghunathan, Tetrahedron, 2013, 69, 9742–9750 CrossRef CAS.
- J. N. S. Rao and R. Raghunathan, Tetrahedron Lett., 2013, 54, 6568–6573 CrossRef.
- A. I. Almansour, R. S. Kumar, F. Beevi, A. N. Shirazi, H. Osman, R. Ismail, T. S. Choon, B. Sullivan, K. McCaffrey and A. Nahhas, Molecules, 2014, 19, 10033–10055 CrossRef PubMed.
- C. Teja, S. N. Babu, A. Noor, J. A. Daniel, S. A. Devi and F. R. N. Khan, RSC Adv., 2020, 10, 12262–12271 RSC.
- D. Pavithra, K. R. Ethiraj and F. R. Nawaz Khan, Eur. J. Org Chem., 2020, 2020, 7035–7050 CrossRef CAS.
- Á. Cores, V. Estévez, M. Villacampa and J. C. Menéndez, RSC Adv., 2016, 6, 39433–39443 RSC.
- M. S. Reddy, N. S. Kumar and L. R. Chowhan, RSC Adv., 2018, 8, 35587–35593 RSC.
- A. Awasthi, P. Yadav and D. K. Tiwari, New J. Chem., 2021, 45, 2374–2383 RSC.
- P. Yuvaraj and B. S. Reddy, Tetrahedron Lett., 2013, 54, 821–827 CrossRef CAS.
- Y. Sarrafi, M. Sadatshahabi, M. Hamzehloueian, K. Alimohammadi and M. Tajbakhsh, Synthesis, 2013, 45, 2294–2304 CrossRef CAS.
- Y. Shi, H. Zhao and Y. Zhao, Molecules, 2023, 28, 3508 CrossRef CAS PubMed.
- G. Li, M. Wu, F. Liu and J. Jiang, Synthesis, 2015, 3783–3796 CAS.
- M. Palomba, E. De Monte, A. Mambrini, L. Bagnoli, C. Santi and F. Marini, Org. Biomol. Chem., 2021, 19, 667–676 RSC.
- A. Barakat, A. Mostafa, M. Ali, A. M. Al-Majid, L. R. Domingo, O. Kutkat, Y. Moatasim, K. Zia, Z. Ul-Haq and Y. A. Elshaier, Int. J. Mol. Sci., 2022, 23, 11861 CrossRef CAS PubMed.
- K. K. Wang, Y. L. Li, R. X. Chen, A. L. Sun, Z. Y. Wang, Y. C. Zhao, M. Y. Wang and S. Sheng, Adv. Synth. Catal., 2022, 364, 2047–2052 CrossRef CAS.
- K. B. Manjappa, Y.-T. Peng, W.-F. Jhang and D.-Y. Yang, Tetrahedron, 2016, 72, 853–861 CrossRef CAS.
- F. Rouatbi, M. Askri, F. Nana, G. Kirsch, D. Sriram and P. Yogeeswari, Tetrahedron Lett., 2016, 57, 163–167 CrossRef CAS.
- K. N. Tiwari, T. P. Pandurang, S. Pant and R. Kumar, Tetrahedron Lett., 2016, 57, 2286–2289 CrossRef CAS.
- S. Kumari, H. Singh and J. M. Khurana, Tetrahedron Lett., 2016, 57, 3081–3085 CrossRef CAS.
- R. Wen, L. Cen, Y. Ma, J. Wang and S. Zhu, Tetrahedron Lett., 2018, 59, 1686–1690 CrossRef CAS.
- M. Narayanarao, L. Koodlur, S. Gopal, S. Y. Reddy and S. Kamila, Synth. Commun., 2018, 48, 2441–2451 CrossRef CAS.
- S. Basu and C. Mukhopadhyay, Eur. J. Org Chem., 2018, 2018, 1496–1506 CrossRef CAS.
- B. Gayen and A. Banerji, J. Heterocycl. Chem., 2015, 52, 919–925 CrossRef CAS.
- B. Gayen, A. Banerji and K. Dhara, Synth. Commun., 2016, 46, 293–308 CrossRef CAS.
- N. R. Keesari, S. Mudavath, K. R. MV, B. Sridhar and B. Subba Reddy, Synth. Commun., 2020, 50, 973–979 CrossRef CAS.
- N. V. Lakshmi and P. T. Perumal, Tetrahedron Lett., 2013, 54, 1817–1820 CrossRef CAS.
- V. S. Moshkin, V. Y. Sosnovskikh and G.-V. Roeschenthaler, Tetrahedron, 2013, 69, 5884–5892 CrossRef CAS.
- K. B. Manjappa, W.-F. Jhang, S.-Y. Huang and D.-Y. Yang, Org. Lett., 2014, 16, 5690–5693 CrossRef CAS PubMed.
- Y. Toma, M. Kunigami, K.-j. Watanabe, M. Higashi and S. Arimitsu, J. Fluorine Chem., 2016, 189, 22–32 CrossRef CAS.
- N. T. Subasi, H. Yalcinkaya and A. S. Demir, Tetrahedron, 2017, 73, 4329–4334 CrossRef CAS.
- Z.-Y. Mao, Y.-W. Liu, P. Han, H.-Q. Dong, C.-M. Si, B.-G. Wei and G.-Q. Lin, Org. Lett., 2018, 20, 1090–1093 CrossRef CAS PubMed.
- S. Samala, C. E. Song and E. J. Yoo, Org. Biomol. Chem., 2019, 17, 1773–1777 RSC.
- M. Molnár, T. John, A. Dancsó and M. Nyerges, Tetrahedron, 2023, 131, 133225 CrossRef.
|
This journal is © The Royal Society of Chemistry 2024 |