DOI:
10.1039/D3RA07270C
(Review Article)
RSC Adv., 2024,
14, 2929-2946
Update on novel synthetic approaches towards the construction of carbazole nuclei: a review
Received
25th October 2023
, Accepted 10th January 2024
First published on 18th January 2024
Abstract
The carbazole scaffold is a significant entity in organic compounds due to its variety of biological and synthetic applications. Traditionally, carbazole skeletons have been synthesized either via the Grabe–Ullman method, Clemo–Perkin method or Tauber method. With the passage of time, these methods have been modified and explored to accomplish the synthesis of target compounds. These methods include hydroarylations, C–H activations, annulations and cyclization reactions mediated by a variety of catalysts to construct carbazole-based compounds. This brief review article intends to provide recent updates on important methodological developments reported for the synthesis of carbazole nuclei covering 2019–2023.
Introduction
The chemistry of heterocycles is a fundamental part of organic compounds.1 Drug design and development is responsible for a wide variety of heterocyclic compounds that have been discovered and exploited in the area of drug synthesis,2 synthetic polymers,3 fluorescent probes,4 agrochemicals,5 veterinary products,6 dyes7 etc. A large number of heterocyclic compounds occur naturally having algal, fungal, or plant sources of origin, whose synthesis can be carried out via a number of strategies.8,9 These heterocycles may possess oxygen, nitrogen, or sulfur in their cyclic structure.10,11 The presence of a heteroatom influences a substantial change in the properties of the respective compounds making them biologically active12 and medicinally important.13 The heterocycles containing one or more nitrogen in their structures have a significant place in the synthetic field.14 Some of the nitrogen-containing drugs are presented in Fig. 1 which include lisinopril15 (anti-hypertensive) 1, cephalexin16 2 and (+)-penicillin V potassium salt17 4 (anti-biotic), enalapril18 (treat heart failure and diabetes) 3, Atorvastatin19 (decrease cholesterol level) 5 and Sumatriptan20 (treat migraine) 6.
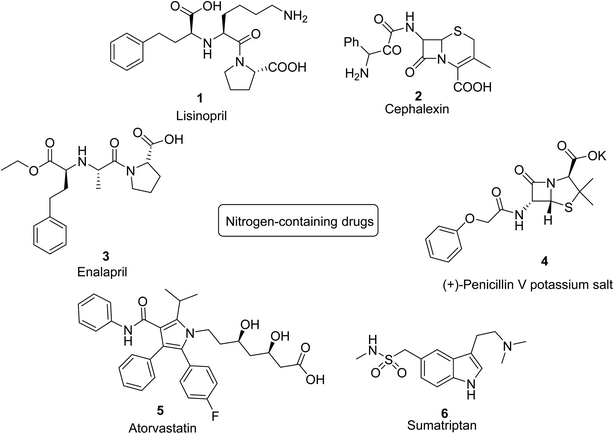 |
| Fig. 1 N-Heterocyclic drugs and their structures. | |
Carbazole is an important part of significant nitrogen-containing heterocycles. It consists of a tricyclic core with two fused benzene rings on either side of the pyrrole ring (Fig. 2).21 This heterocyclic moiety accounts for a number of industrial, and biological properties. Carbazoles belong to the class of indoles and represent privileged scaffolds in biological and non-biological systems by exhibiting a wide spectrum of applications in different fields. It exhibits a number of biological activities22,23 i.e., anti-microbial,24 anti-cancerous,25 anti-inflammatory,26 anti-viral,27 neuroprotective28 and anti-oxidative.29 Among industrial applications, carbazole scaffold has found potential applications in fluorescent probes,30 bioimaging devices,31 organic semiconductors32 and polymers33,34 etc.
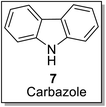 |
| Fig. 2 The structure of [9H-carbazole]. | |
Carbazole embodies a vast variety of plant-based natural compounds, harbored with profound pharmaceutical properties.35,36 It is widespread in a number of naturally occurring compounds such as alkaloids.37 The Rutaceae family is found to be the source of carbazoles among many other naturally occurring sources.38 Cancer has been the leading cause of death among mankind. The discovery of multiple etiologies along with their treatments goes side by side. Various naturally occurring compounds have been found active against cancer-causing agents i.e., oncogens. The carbazole derivatives have emerged to find anti-cancerous activities against such oncogens, i.e., ellipticine 11,39 a promising anti-tumor drug. Carvedilol 13,40 a vasodilator and effective anti-oxidant has been found to be operative in the treatment of heart failure. Along with the compounds as mentioned above, the structures of some of the bioactive carbazoles38–43 are presented as follows (Fig. 3).
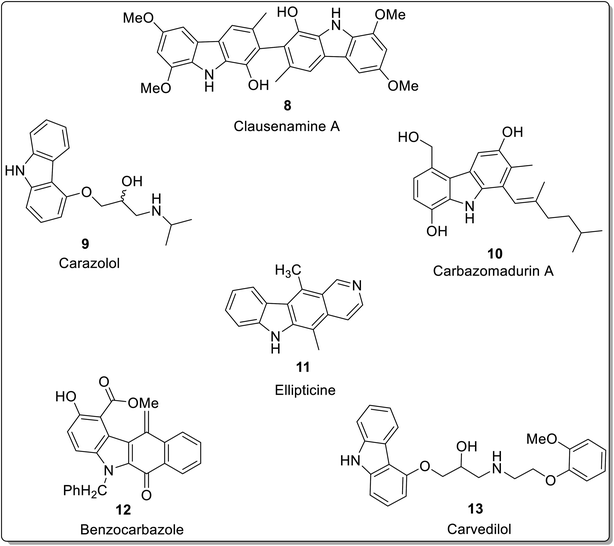 |
| Fig. 3 Structures of carbazole containing natural compounds. | |
A lot of research has been conducted in the past for the synthesis of N-heterocycles44,45 including carbazoles.46–48 The synthesis of carbazole scaffold has been achieved via alternative strategies.49–54 These strategies involved electrocyclic reactions, cycloaddition reactions, annulations and insertion reactions. This review article covers the latest updates on the methodological development of carbazole skeleton reported since 2019. The division of content has been focused on the process involved in the construction of carbazole nuclei. The synthesis of carbazole skeleton has been achieved via a number of strategies which have been explained under specific classifications as follows.
Synthesis of carbazole nucleus
Hydroarylation reactions for the synthesis of carbazoles
Transition metals are widely employed as catalysts in the field of organic synthesis.55 Hydroarylation reactions are considered efficient owing to economically appealing, mild conditions and high-bond forming efficacy.56 Palladium-catalyzed hydroarylation for carbazole synthesis was proposed by Martin et al. in 2021, for which Pd(PPh3)2Cl2 was employed as a catalyst with CuI acting as a co-catalyst and triethylamine as a solvent at 40 °C to accomplish the target compounds.57 A chemo- and regioselective synthesis of 2- iodo-1-aryl-9H-carbazoles was achieved by employing different substrates leading towards a diverse series of three types of products. The Pd-catalysis was performed by reaction of aryl iodides 15, 2-iodo thiophenes 18 and 3-iodo-indole carbaldehydes 21 with substituted iodo alkynols 14 to furnish respective carbazoles 16, 19 and 22 in moderate yields (Scheme 1). The feasible mechanistic pathway for this reaction included the synthesis of Sonogashira adduct A from alkynols 14 and aryl iodide under palladium catalysis. In the next step, palladium salt coordinated with alkyne moiety to form complex B which underwent 5-endo-dig carbopalladation to generate pallada-spirocyclopentene intermediate C. Next, iodotetrahidrocarbazolium specie D was produced via 1,2-alkyl migration which was proceeded by 1,4-iodonium migration to give 2-iodo 1-aryl-4,9-dihydro-3H-carbazol-4-ols E. In the final step, 2-iodocarbazoles were produced after dehydration (Scheme 2).57 The key attributes of this reaction are the selectivity induced by Pd-catalyst and the functionalization of the aryl iodide group by employing the Suzuki reaction to furnish a variety of compounds. In the subsequent year, Jatoth et al. accomplished the synthesis of N-heterocycles fused with carbazole by employing TFA-mediated, metal-free, green, one-pot hydroarylation protocol.58 The reaction of 3-ethynyl-2-chloro substituted quinoxalin 27 and 5-ethynyl-4-chloro substituted pyrimidines 25 with indole derivatives 24 in the presence of trifluoroacetic acid at room temperature for 60 min furnished N-heterocycle fused substituted carbazoles 26 and 28 in 65–68 and 63–71% respective yields comprising of twelve and two examples each. This methodology features short reaction time, wide substrate scope, facile conditions and good yields of products (Scheme 3).
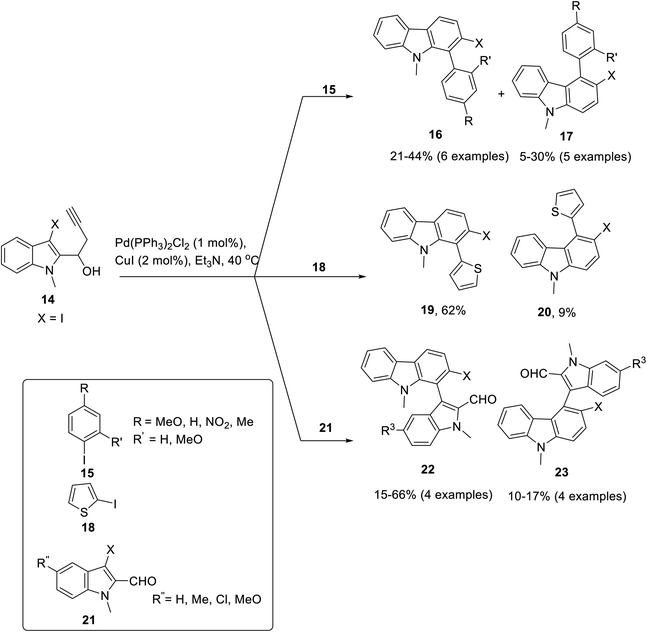 |
| Scheme 1 Pd-catalyzed hydroarylation for synthesis of 2-iodo-1-aryl-9H-carbazoles. | |
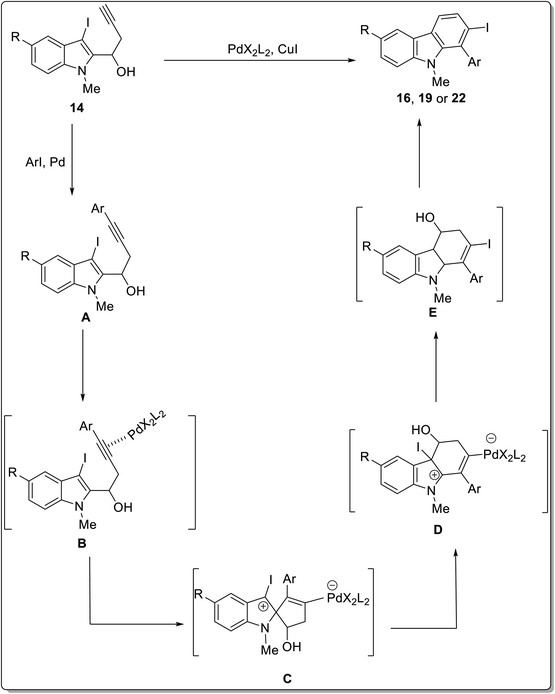 |
| Scheme 2 Mechanistic details for the synthesis of iodocarbazoles 16, 19 and 22.57 | |
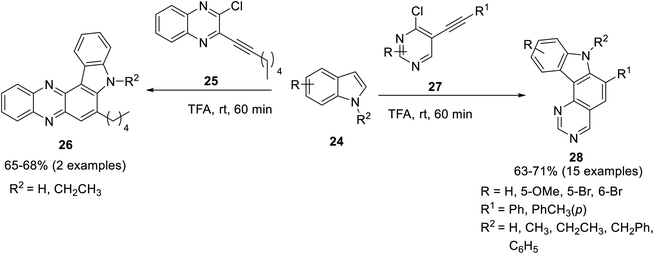 |
| Scheme 3 One-pot hydroarylation for carbazole synthesis. | |
Annulation reactions for the synthesis of carbazoles
Annulation reactions are widely employed for the synthesis of functionalized carbazoles. These include [4 + 2] annulation, benzannulation, cascade annulation etc.59,60 Among these, the [4 + 2] annulation is deliberated to be the most productive protocol for indole-to-carbazole synthesis. El-Harairy et al. in 2019 employed a [4 + 2] annulation protocol based on a Brønsted acid having imidazolium-based ionic liquid with sulfone moiety in butyl acetate to achieve the task.61 The efficiency of this catalytic system was checked by employing it for the conversion reactions of different indoles. For [4 + 2] annulation, 2 molecules of N-methylindole 30 were made to react with 2,5-dihydro-2,5-dimethoxyfuran 29 in the presence of butyl acetate as a solvent and 10 mol% of ionic liquid 31 as a ligand to afford 70–92% of target compounds 32. Some important features of this methodology included the reusability and effectiveness of ionic liquid in obtaining better selectivity and yields (Scheme 4). The imidazolium-based ionic liquid breaks the stereotype of using toxic and hazardous solvents for the synthesis of organic compounds and introduces a more selective, high-yielding and recoverable green catalytic solvent system with butyl acetate. Another Brønsted acid-catalyzed pinacol rearrangement for the synthesis of functionalized carbazoles from α-(3-indolyl) ketones via cascade annulation (one-pot) was reported by Kundu et al. in the similar year.62 The targeted compounds were obtained by reacting 2-alkenyl indole 33 and substituted aldehydes 34 in the presence of 20 mol% of para-toluenesulfonic acid (PTSA·H2O) as a catalyzing agent in toluene as a solvent at 120 °C leading towards the synthesis of functionalized carbazoles 35. The functionalized carbazoles 35 could further N-methylated in the presence of methyl iodide and dimethyl sulfoxide providing carbazole derivatives 35a in 44–67% yields (Scheme 5). The mechanistic details for the synthesis functionalized carbazole 35 involved the synthesis of indolyl diol A which underwent pinacol-type rearrangement to give α-(3-indolyl) ketone B. Next, desired carbazole 35 was synthesized via tandem cyclization and aromatization of subsequent intermediates (Scheme 6).62 The catalyst employed for this methodology is commercially available and inexpensive making it a feasible and convenient methodology.
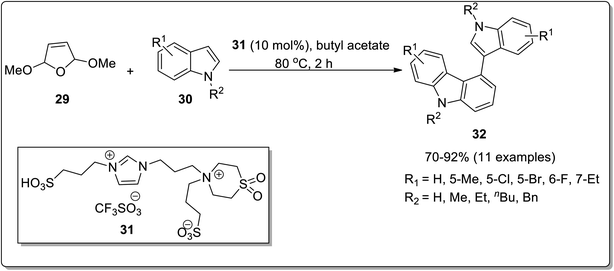 |
| Scheme 4 [4 + 2] Annulation for the synthesis of carbazole. | |
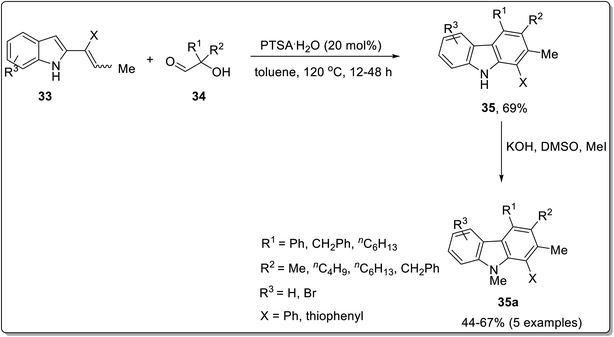 |
| Scheme 5 One-pot cascade annulation reaction for synthesis of carbazole nucleus. | |
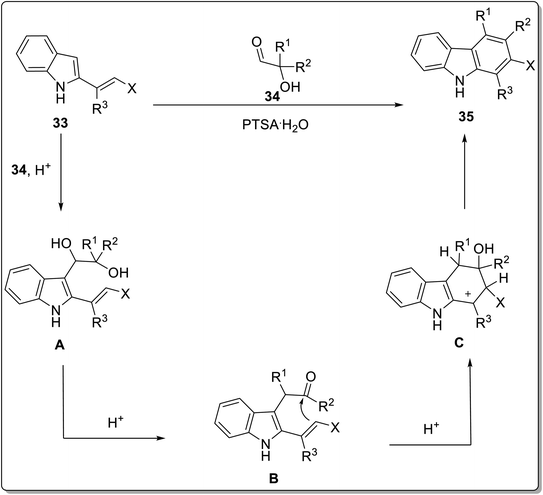 |
| Scheme 6 Mechanistic details for the synthesis of functionalized carbazole 35.62 | |
The benzannulation reactions are used widely to access functionalized and naturally occurring carbazoles. The Scheme 7 presents the synthesis of 4-hydroxy carbazoles 38 from nitroindoles 36a via a metal-free benzannulation with alkylidene azalactones 37, reported by Cao et al. in 2021.63 The N-Ts-3-nitroindole 36a on conjugate vinylogous addition to azalactone 37 in the presence of 2 equivalents of base and 1
:
2 solvent ratio of THF/hexane at 40 °C furnished 4-hydroxy carbazole derivatives 38 in 20–92% yield. The mechanistic details implied the synthesis of dienolate A via deprotonation of azalactone 37 by K2CO3 which gave nitronate intermediate B by reacting with 3-nitroindole 36a. Next, indoline-fused compound C was produced from intermediate B via intramolecular cyclization. The compound C eliminated a molecule of nitrous acid to generate indole-fused compound D which produced potassium carbazol-4-olate E followed by acidification to give carbazole derivative 38 (Scheme 8).63 The azalactone tolerated a wide variety of substrates possessing facile and scalable methodology. Karan et al. employed a triple cascade one pot benzannulation protocol and provided a back-to-front approach for synthesizing carbazole nuclei 40 from indole 2-carboxaldehyde 36b and substituted boronic acids 39.64 The one-pot procedure consisted of allylation followed by E-2 elimination mediated with triethylamine and 6π-electro cyclization to furnish carbazoles 40 in 49–74% yield range (Scheme 7). The mechanistic details for the synthesis of carbazole 40 inferred the reaction between indole 2-carboxaldehyde 36b and substituted boronic acids 39 to give allylic alcohol A. The next step involved mesylation of allylic alcohol A along with triethyl amine assisted elimination to give conjugated alkene C. In the final step, carbazoles 40 are produced via 6π-electrocyclization and aromatization of alkene C (Scheme 9).64 The developed procedure provided a scalable pathway for the synthesis of carbazole-based natural products i.e., glycozolinol and glycozoline. Another one-pot, metal-free and base-catalyzed synthesis was reported by Singh et al. for the synthesis of carbazole-based natural products from carbazole 41.65 The synthesized natural products are calothrixin B 41a, staurosporine 41b and carbazomycin A 41c (Fig. 4).
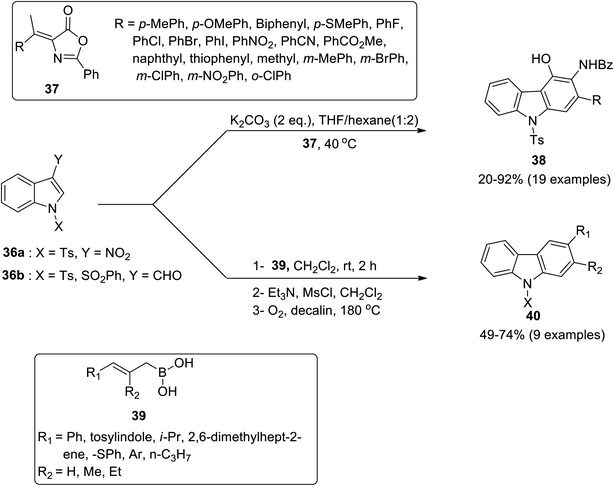 |
| Scheme 7 Synthesis of 4-hydroxy carbazole 38 and substituted carbazoles 40. | |
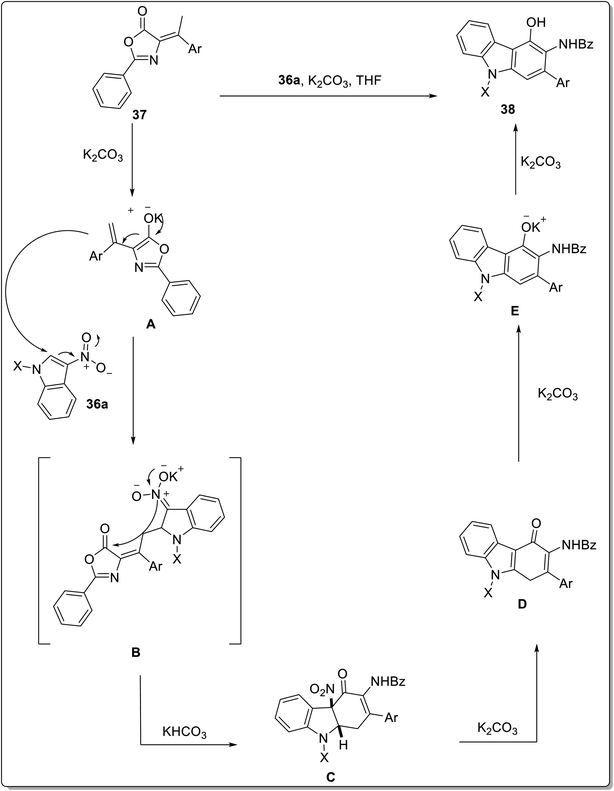 |
| Scheme 8 Mechanistic details for the synthesis of carbazole derivative 38.63 | |
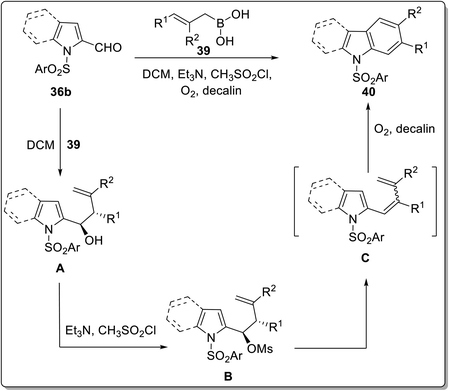 |
| Scheme 9 Mechanistic details for the synthesis of carbazole derivative 40.64 | |
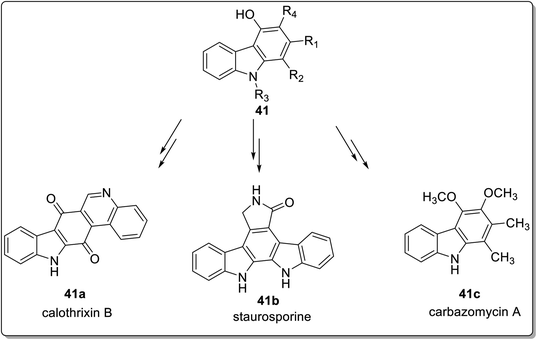 |
| Fig. 4 Structure of carbazole derivative 41 and natural products (calothrixin B 41a, staurosporine 41b and carbazomycin A 41c) obtained by it. | |
Intramolecular reactions are regioselective, efficient and can be highly versatile. The synthesis of carbazoles has been achieved by various intramolecular cyclization strategies, which might involve serendipitous discovery sometimes.66 Kumar et al. reported the synthesis of tetracyclic carbazoles via an intramolecular benzannulation of alkyne-tethered indoles catalyzed with rhodium acetate in the presence of an oxidant and solvent.67 The reaction conditions were optimized by screening different catalysts and solvents by altering their concentration. The intramolecular benzannulation of ethyl 3-(indol-3-yl)acrylate tethered with alkyne 42 was performed by employing 5 mol% of rhodium acetate, 2 mol% of copper bromide in dimethyl acetate to obtain the annulated product 43. This methodology has been reported to be atom-economical, feasible and substrate-scalable (Scheme 10). The plausible mechanism for the synthesis of carbazole derivative 43 involved the oxidative addition of Rh(III) to bromocompound 42a to give intermediate A. Next, rhodacycle B was produced by a concerted metalation deprotonation step followed by 6-membered rhodacycle intermediate C synthesis via an internal alkyne insertion. In the final step, the target compound was synthesized by reductive elimination of intermediate C (Scheme 11).67 The exploitation of stable bisalkynol 44, obtained from N,N-bis(2- bromoallyl)amines, for a gold-catalyzed back-to-front approach to access carbazoles 45 via a double benzannulation strategy was proposed by Muñoz-Torres et al. in 2021.68 The reaction proceeded in the presence of 5 mol% of NaAuCl4·2H2O in DCM which induced double benzannulation to give substituted carbazoles in 60–85% yields (Scheme 12). This useful methodology allows the regioselective synthesis of functionalized carbazoles from readily available starting materials.
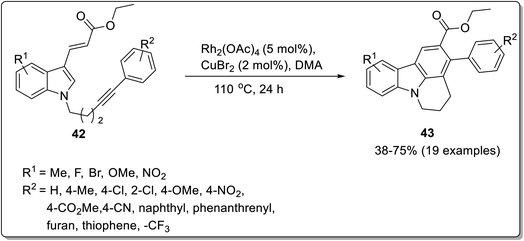 |
| Scheme 10 Rhodium catalyzed intramolecular benzannulation for synthesis of carbazole. | |
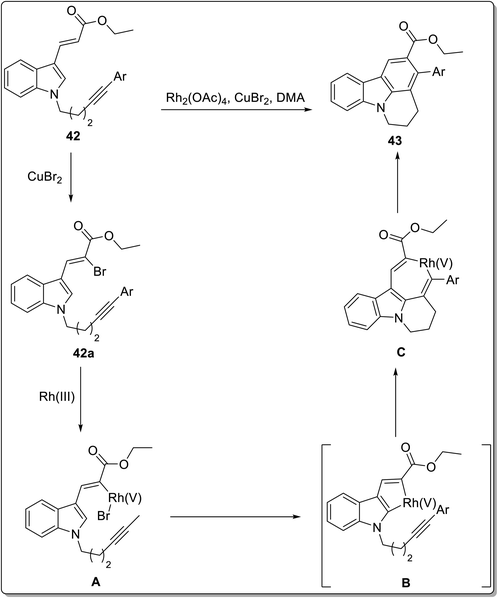 |
| Scheme 11 Mechanistic details for the synthesis of carbazole derivative 43.67 | |
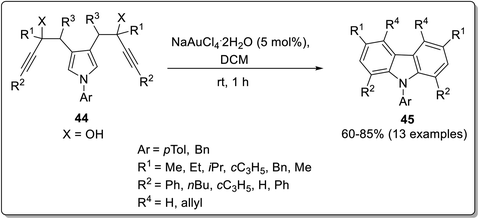 |
| Scheme 12 Gold-catalyzed double benzannulation for synthesis of carbazole 45. | |
The carbazole and its derivatives have been constructed by various strategies.69 Despite the difficulty of synthesizing N–H carbazole from N–H indole, a three-component annulation reaction for the carbazole development has been reported by Huang et al. in 2021.70 It involved the reaction between an indole 46, bromoacetaldehyde dimethyl acetal 47 and 1,3-dicarbonyl compound 48 to furnish carbazole-2-carboxylates 49. The synthesized carbazole compounds were further substituted by hydrazine and amide to afford biologically active compounds, which were screened and evaluated against different cell lines (Scheme 13).
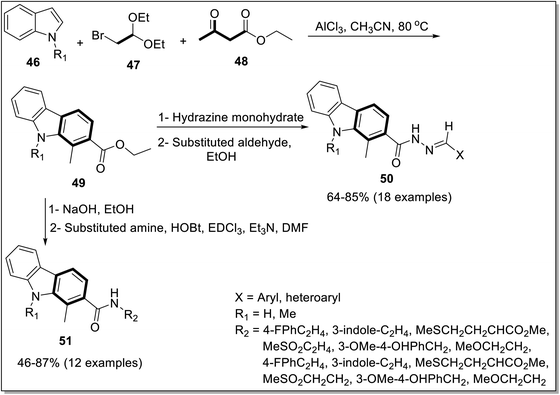 |
| Scheme 13 AlCl3-mediated annulation for the synthesis of carbazoles 49 and its derivatives. | |
C–H activation for the synthesis of carbazoles
The carbazole nucleus can be constructed via C–C, C–N and C–H activation. Among these, C–H activation approach catalyzed with metal salts is considered to be an efficient and mild approach. In that respect, C–H activation via intermolecular and intramolecular reactions has been included in this review. Khan et al. reported the synthesis of functionalized carbazoles by employing Pd-catalyzed intramolecular C–H activation in 2019.71 This approach consisted of N-arylation by Chan-Lam coupling of boronic acids 53 and o-iodoanilines 52 in the presence of 10 mol% of Cu(OAc)2, 1.2 equivalents of DBU as a base and decanoic acid as a solvent to give 2-iodo-N-phenyl aniline 54. The second step involved 5 mol% of palladium catalyst and 6 equivalents of DBU-mediated C–H activation of 2-iodo-N-phenyl aniline 54 in toluene under a nitrogen atmosphere to furnish carbazoles 55a in the 41–93% yield range. Alam et al. proposed a similar methodology with modified conditions including less catalyst loading of DBU(2 equiv.) in DMF and alteration with microwave irradiation for the synthesis of substituted carbazoles 55b in 20–95% yields.72 The Pd-catalyzed strategy was also amenable for the synthesis of 1-hydroxy carbazoles under aerobic conditions (Scheme 14). The plausible mechanism proposed for the synthesis of carbazoles 55a and 55b took place in four steps which involved the synthesis of pallado σ-complex A by in situ generated Pd(0) species via oxidative addition. Next, σ-bond metathesis gave intermediate B constituting C–H activation step followed by DBU-assisted deprotonation to furnish 6-membered palladacycle C. The final step consisted of reductive elimination to generate carbazole 55a and 55b (Scheme 15). Microwave irradiation led to the synthesis of products in better yields with less amount of base and solvent in a short reaction time.
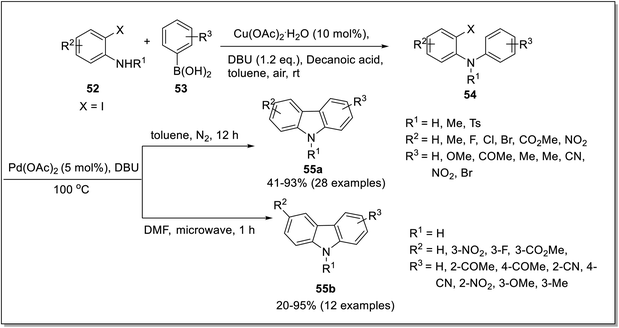 |
| Scheme 14 Pd-catalyzed and microwave-assisted synthesis of carbazole via C–H activation. | |
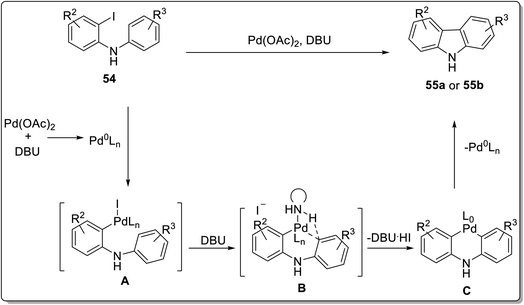 |
| Scheme 15 Mechanistic details for the synthesis of carbazoles 55a and 55b.71,72 | |
Another class of carbazole i.e., indolocarbazole has found significant value in the organic field.73 Intramolecular C–H activation methodology to access carbazole skeleton has also been reported by Youn et al. (2019)74 and Martinez-Lara et al. (2021).75 The palladium-catalyzed C–H activation, reported by Youn et al. exploited N-Ts-2-aminobiaryl derivatives 56 as starting compounds. Hydroxy carbazole 58 was furnished in the presence of 5 mol% Pd(OAc)2, bathocuproine 57 as a ligand, sodium acetate as an additive and oxygen as an oxidant (Scheme 16). The methodology acquired by Martinez-Lara et al. explored the synthesis of indolocarbazoles via gold and molybdenum catalyzed reaction.47 The synthesis commenced with the construction of alpha-indol-3-yl alkyl propargylic alcohol 60 from bis-indolyl ketone 59 by Pd-catalysis. The indolo (2,3-c) carbazoles 61 were afforded by regioselective gold-catalyzed cyclization causing 1,2-alkyl migration followed by dioxomolybdenum-mediated Cadogan reductive cyclization reaction to furnish 64–85% yields (Scheme 17). The gold catalysis can also induce 1,2-alkenyl migration which will provide indolo (3,2-α) carbazoles in the presence of toluene as a solvent making it a regioselective catalyst.
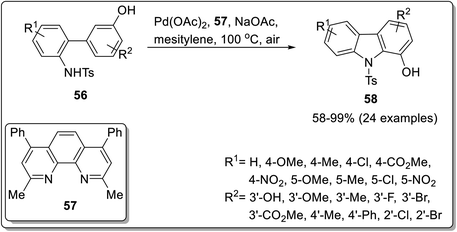 |
| Scheme 16 Pd-catalyzed C–H amidation for synthesis of 1-hydroxy carbazoles. | |
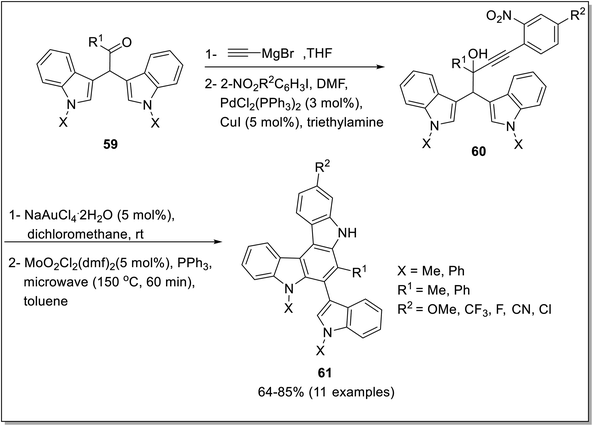 |
| Scheme 17 Synthesis of carbazole via Au- and Mo-catalysis. | |
Min et al. reported an intermolecular rhodium catalyzed C–H activation protocol for the synthesis of C2-formylated nucleus of carbazole.76 The tandem cross-coupling reaction between indolyl nitrones 62 and 2-methylidene cyclic carbonates 63 was proceeded in the presence of 5 mol% of rhodium catalyst, a combination of CuF2·MgSO4 as an additive and toluene as solvent at 120 °C for 12 h to give target compounds 64 in 10–71% yields (Scheme 18). The mechanism of reaction involves C–H activation of rhodium catalyst as the first step followed by migratory insertion of cyclic carbonates. The next step involved β-O-elimination for the removal of carbon dioxide and exo [3 + 2] cycloaddition reaction and oxidation to give bridged heterocycle. Further, aromatization and formylation provided targeted carbazole. The proposed mechanism implied the synthesis of intermediate A via C–H activation step between [Rh(III)Cp*(MeCN)3(SbF6)2] and indolyl nitrone 62. Next, cyclic carbonate 63 and intermediate A coordinated to give intermediate B which underwent migratory insertion to give eight-membered O–Rh(III)–C complex C. The complex C generated intermediate D by β-O-elimination. Further, bridged heterocycle E was afforded via exotype [3 + 2] cycloaddition step. The aromatization of bridged heterocycle E was succeeded by aerobic oxidation to furnish C2-formylated carbazole 64 (Scheme 19).76
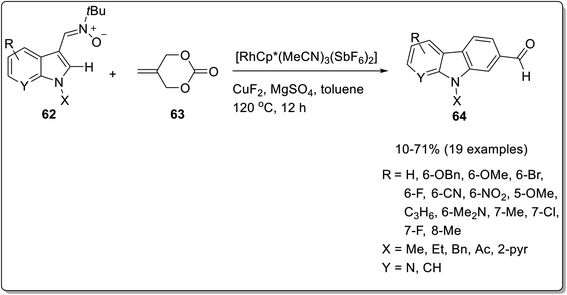 |
| Scheme 18 Rh-catalyzed synthesis of carbazoles. | |
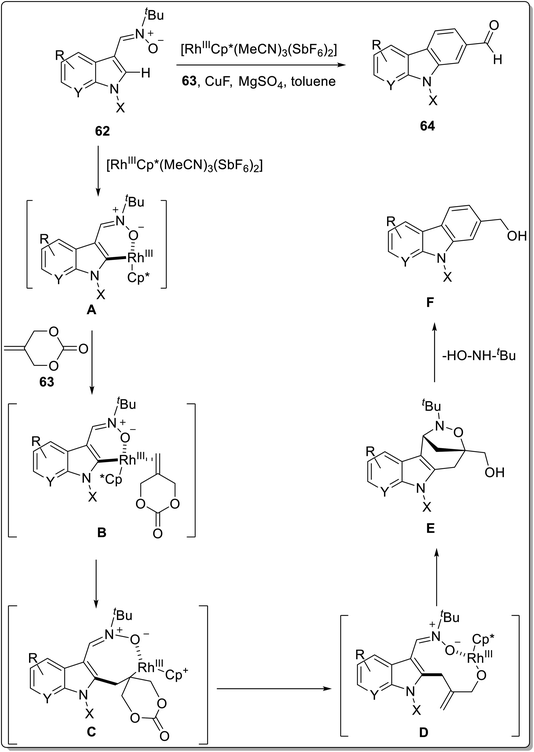 |
| Scheme 19 Mechanistic details for the synthesis of carbazoles 64.76 | |
Synthesis of carbazole via carboamination
2-Alkynyl anilines have been utilized for the synthesis of indoles via annulation reaction catalyzed by transition metals.77 But these reactions have certain drawbacks, including low substrate scalability and high temperature requirements.78 Thus, to overcome these shortcomings, Tambe et al. reported an alternative methodology, namely, trans carboamination for synthesizing functionalized indoles catalyzed by nickel complex and applied it to synthesize carbazoles.79 The method consisted of propynyl anilino acrylate 65 undergoing carboamination in the presence of Ni(COD)2 as a catalyst, PyPhos as ligand and CH3CN as a solvent in the reaction mixture to afford functionalized indoles 66 which were cyclized in the presence of pyrrolidine and DMF to furnish carbazoles 68 with a yield range of 48–75% (Scheme 20). The salient features of this methodology included efficient synthetic access to multifunctionalized indoles which could be converted to important N-heterocycles via one step process.
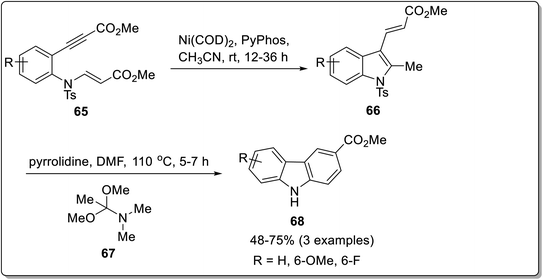 |
| Scheme 20 Ni-catalyzed carboamination for the synthesis of carbazoles derivatives. | |
Synthesis of carbazoles via [3 + 2] cycloaddition
In 2021, Zhang et al. aimed at the construction of carbazole skeleton 71 by [3 + 2] cycloaddition reaction between azobenzenes 69 and arynes 70 catalyzed by sunlight.80 The cycloaddition was performed in the presence of CsF in CH3CN mediated by sunlight (Scheme 21). The important features of this reaction include good compatibility of functional groups and catalyst-free conditions. The mechanism consisted of the synthesis of benzyne 70a by treating compound 70 with CsF. Next, intermediate A was synthesized by interaction of benzyne 70a and azobenzene 69. The intermediate A underwent rotation mediated by sunlight to produce intermediate B followed by intramolecular cyclization to furnish five-membered intermediate C. The final step involved aromatization facilitated by water molecules to generate target compound 71 (Scheme 22).
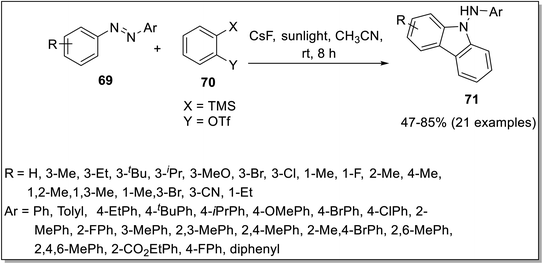 |
| Scheme 21 Sunlight-mediated [3 + 2] cycloaddition for carbazole synthesis. | |
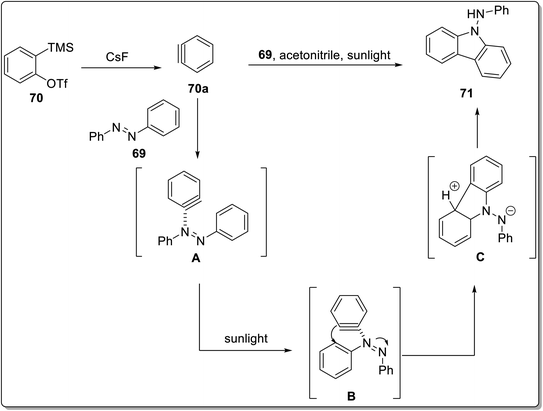 |
| Scheme 22 Mechanistic details for the synthesis of carbazoles 71.80 | |
Conclusion
To conclude the updates provided in the article, the carbazole skeleton has been reported to be constructed via allylation, annulation, C–H activation, transition-metal catalyzed as well as under metal-free and sunlight mediated methodologies. These involve either oxidative cyclization of biphenyls with an ortho nitrogen, metal-catalyzed indole cyclization or a step-by-step synthesis of carbazole starting from phenyl systems. These strategies have been applied to wide substrate scope which includes pyrimidine derivatives, bisalkynyls, substituted biaryls, indolyl nitrones, alkyne anilinoacrylates, and azobenzenes. The employment of these methods has aided in the synthesis of carbazole-based significant natural products and derivatives employed in pharmacological and industrial areas. Additionally, there are certain drawbacks in the reported methodologies as well which include the use of expensive catalysts, harsh chemicals, toxic solvents and extensive energy resources. These can be overcome by employing cost-effective resources and reagents to reduce the harmful impacts. To add further, there's still room left for improvement to this significant research area for introducing more environment-friendly and benign methods to carry out the required synthesis.
Conflicts of interest
There are no conflicts to declare.
Acknowledgements
A. Irfan extends his appreciation to the Deanship of Scientific Research at King Khalid University for funding through large group Research Project under grant number RGP2/276/44.
References
- A. Al-Mulla, A Review: Biological Importance of Heterocyclic Compounds, Pharma Chem., 2017, 9, 141–147 CAS.
- I. Shahzadi, A. F. Zahoor, A. Rasul, N. Rasool, Z. Raza, S. Faisal, S. Faisal, B. Parveen, S. Kamal, M. Z. Rehman and F. M. Zahid, Synthesis, Anticancer, and Computational Studies of 1, 3, 4-Oxadiazole-Purine Derivatives, J. Heterocycl. Chem., 2020, 57, 2782–2794 CrossRef CAS.
- M. E. Cinar and T. Ozturk, Thienothiophenes, Dithienothiophenes, and Thienoacenes: Syntheses, Oligomers, Polymers, and Properties, Chem. Rev., 2015, 115, 3036–3140 CrossRef CAS PubMed.
- M. S. T. Goncalves, Fluorescent Labeling of Biomolecules with Organic Probes, Chem. Rev., 2009, 109(1), 190–212 CrossRef CAS PubMed.
- Y. Ogawa, E. Tokunaga, O. Kobayashi, K. Hirai and N. Shibata, Current Contributions of Organofluorine Compounds to the Agrochemical Industry, iScience, 2020, 23, 101467 CrossRef CAS PubMed.
- E. Kabir and M. Uzzaman, A Review on Biological and Medicinal Impact of Heterocyclic Compounds, Results Chem., 2022, 4, 100606 CrossRef CAS.
- M. C. Ríos, N. F. Bravo, C. C. Sánches and J. Portilla, Chemosensors Based On N-Heterocyclic Dyes: Advances in Sensing Highly Toxic Ions such as CN− and Hg2+, RSC Adv., 2021, 11, 34206–34234 RSC.
- S. Tabassum, A. F. Zahoor, S. Ahmad, R. Noreen, S. G. Khan and H. Ahmad, Cross-Coupling Reactions Towards the Synthesis of Natural Products, Mol. Diversity, 2021, 1–43 Search PubMed.
- A. F. Zahoor, M. Yousaf, R. Siddique, S. Ahmad, S. A. R. Naqvi and S. M. A. Rizvi, Synthetic Strategies Toward The Synthesis of Enoxacin-, Levofloxacin-, and Gatifloxacin-Based Compounds: A Review, Synth. Commun., 2017, 47(11), 1021–1039 CrossRef CAS.
- R. Akhtar, A. F. Zahoor, N. Rasool, M. Ahmad and K. G. Ali, Recent Trends in the Chemistry of Sandmeyer Reaction: a review, Mol. Diversity, 2022, 26(3), 1837–1873 CrossRef CAS PubMed.
- S. Ahmad, A. F. Zahoor, S. A. R. Naqvi and M. Akash, Recent Trends in Ring Opening of Epoxides with Sulfur Nucleophiles, Mol. Diversity, 2018, 22(1), 191–205 Search PubMed.
- S. Faiz, A. F. Zahoor, M. Ajmal, S. Kamal, S. Ahmad, A. M. Abdelgawad and M. E. Elnaggar, Design, Synthesis, Antimicrobial Evaluation, and Laccase Catalysis Effect of Novel Benzofuran–Oxadiazole and Benzofuran–Triazole Hybrids, J. Heterocycl. Chem., 2019, 56(10), 2839–2852 Search PubMed.
- A. De, S. Sarkar and A. Majee, Recent Advances on Heterocyclic Compounds with Antiviral Properties, Chem. Heterocycl. Compd., 2021, 57, 410–416 Search PubMed.
- I. Shahzadi, A. F. Zahoor, A. Rasul, A. Mansha, S. Ahmad and Z. Raza, Synthesis, Hemolytic Studies, and In Silico Modeling of Novel Acefylline-1,2,4-Triazole Hybrids as Potential Anti-cancer Agents against MCF-7 and A549, ACS Omega, 2021, 6(18), 11943–11953 CrossRef CAS PubMed.
- M. T. Wu, A. W. Douglas, D. L. Ondeyka, L. G. Payne, T. J. Ikeler, H. Joshua and A. A. Patchett, Synthesis of N2-[(S)-1-Carboxy-3-phenylpropyl]-L-lysyl-L-proline (Lisinopril), J. Pharm. Sci., 1985, 74, 352–354 CrossRef CAS PubMed.
- C. W. Ryan, R. L. Simon and E. M. Van Heyningen, Chemistry of cephalosporin antibiotics. III. Deacetoxycephalosporins. Synthesis of Cephalexin and Some Analogs, J. Med. Chem., 1969, 12, 310–313 CrossRef CAS PubMed.
- R. Vardanyan and V. Hruby, Chapter 22: Antihypertensive Drugs, Synthesis of Essential Drugs, Elsevier, 2006, pp. 295–310 Search PubMed.
- P. J. Fischer and C. R. Ganellin, Analogue-Based Drug Discovery, John Wiley & Sons, 2006, p. 490 Search PubMed.
- M. Muller, Angew. Chem., Int. Ed., 2005, 44, 362–365 CrossRef PubMed.
- S. J. Hopkins, Drugs Today, 1992, 28, 155 CrossRef.
- F. F. Zhang, L. L. Gan and C. H. Zhou, Synthesis, Antibacterial and Antifungal Activities of some Carbazole Derivatives, Bioorg. Med. Chem. Lett., 2010, 20, 1881–1884 CrossRef CAS PubMed.
- H. J. Knolker and K. R. Reddy, Isolation and Synthesis of Biologically Active Carbazole Alkaloids, Chem. Rev., 2002, 102, 4303–4428 CrossRef PubMed.
- A. Głuszyńska, Biological Potential of Carbazole Derivatives, Eur. J. Med. Chem., 2015, 94, 405–426 Search PubMed.
- W. Gu and S. Wang, Synthesis and Antimicrobial Activities of Novel 1H-Dibenzo[A,C]Carbazoles from Dehydroabietic Acid, Eur. J. Med. Chem., 2010, 45, 4692–4696 CrossRef CAS PubMed.
- F. Song, D. Liu, X. Huo and D. Qiu, The Anticancer Activity of Carbazole Alkaloids, Arch. Pharm., 2022, 355, 2100277 Search PubMed.
- Y. Nalli, V. Khajuria, S. Gupta, P. Arora, S. Riyaz-Ul-Hassan, Z. Ahmed and A. Ali, Four New Carbazole Alkaloids from Murraya Koenigii that Display Anti-Inflammatory and Anti-Microbial Activities, Org. Biomol. Chem., 2016, 14, 3322–3332 Search PubMed.
- M. R. TePaske, J. B. Gloer, D. T. Wicklow and P. F. Dowd, Tubingensin A: An Antiviral Carbazole Alkaloid From the Sclerotia of Aspergillus tubingensis, J. Org. Chem., 1989, 54, 4743–4746 CrossRef CAS.
- D. Zhu, M. Chen, M. Li, B. Luo, Y. Zhao, P. Huang, F. Xue, S. Rapposelli, R. Pi and S. Wen, Discovery of Novel N-Substituted Carbazoles As Neuroprotective Agents With Potent Anti-Oxidative Activity, Eur. J. Med. Chem., 2013, 68, 81–88 CrossRef CAS PubMed.
- Y. Tachibana, H. Kikuzaki, N. H. Lajis and N. Nakatani, Antioxidative Activity of Carbazoles from Murraya koenigii Leaves, J. Agric. Food Chem., 2001, 49, 5589–5594 CrossRef CAS PubMed.
- W. D. Wang, Y. Hu, Q. Li and S. L. Hu, A Carbazole-Based Turn-On Fluorescent Probe For The Detection Of Hydrazine In Aqueous Solution, Inorg. Chim. Acta, 2018, 477, 206–211 CrossRef CAS.
- J. Yin, Y. Ma, G. Li, M. Peng and W. Lin, A Versatile Small-Molecule Fluorescence Scaffold: Carbazole Derivatives For Bioimaging, Coord. Chem. Rev., 2020, 412, 213257 CrossRef CAS.
- C. M. Hendrich, V. D. Hannibal, L. Eberle, L. E. Hertwig, U. Zschieschang, F. Rominger, M. Rudolph, H. Klauk and A. S. K. Hashmi, Gold-Catalyzed Synthesis of π-Extended Carbazole-Based Systems and their Application as Organic Semiconductors, Adv. Synth. Catal., 2021, 363, 1401–1407 CrossRef CAS.
- J. V. Grazulevicius, P. Strohriegl, J. Pielichowski and K. Pielichowski, Carbazole-Containing Polymers: Synthesis, Properties And Applications, Prog. Polym. Sci., 2003, 28, 1297–1353 CrossRef CAS.
- W. J. Fan, B. Sun, J. Ma, X. Li, H. Tan and L. Xu, Coordination-Driven Self-Assembly of Carbazole-Based Metallodendrimers with Generation-Dependent Aggregation-Induced Emission Behavior, Chem.–Eur. J., 2015, 21, 12947–12959 CrossRef CAS PubMed.
- T. A. Choi, R. Czerwonka, W. Fröhner, M. P. Krahl, K. R. Reddy, S. G. Franzblau and H. J. Knölker, Synthesis and Activity of Carbazole Derivatives Against Mycobacterium Tuberculosis, ChemMedChem, 2006, 1, 812–815 Search PubMed.
- K. Dhara, T. Mandal, J. Das and J. Dash, Synthesis of Carbazole Alkaloids by Ring-Closing Metathesis and Ring Rearrangement–Aromatization, Angew. Chem., Int. Ed., 2015, 54, 15831–15835 CrossRef CAS PubMed.
- R. Hesse, K. K. Gruner, O. Kataeva, A. W. Schmidt and H. J. Knölker, Efficient Construction of Pyrano [3, 2-a] carbazoles: Application to a Biomimetic Total Synthesis of Cyclized Monoterpenoid Pyrano [3,2-a] carbazole Alkaloids, Chem.–Eur. J., 2013, 19, 14098–14111 Search PubMed.
- E. Yamuna and K. Prabakaran, Recent Trends and Latest Innovations in Life Sciences, 2022, 80–88 Search PubMed.
- N. C. Garbett and D. E. Graves, Extending Nature’s Leads: The Anticancer Agent Ellipticine, Anticancer Agents Med. Chem., 2004, 4, 149–172 CrossRef CAS PubMed.
- W. M. Book, Carvedilol: A Nonselective β Blocking Agent With Antioxidant Properties, CHF, 2007, 8, 173–190 Search PubMed.
- A. Zhang and G. Lin, The First Synthesis Of Clausenamine-A And Cytotoxic Activities Of Three Biscarbazole Analogues Against Cancer Cells, Bioorg. Med. Chem. Lett., 2000, 10, 1021–1023 CrossRef CAS PubMed.
- A. Mejean, J. L. Guillaume and A. D. Strosberg, Carazolol: A Potent, Selective β3-Adrenoceptor Agonist, Eur. J. Pharmacol., 1995, 291, 359–366 CrossRef CAS PubMed.
- Y. Hieda, T. Choshi, S. Kishida, H. Fujioka and S. Hibino, A Novel Total Synthesis Of The Bioactive Poly-Substituted Carbazole Alkaloid Carbazomadurin A, Tetrahedron Lett., 2010, 51, 3593–3596 CrossRef CAS.
- A. J. Rago and G. Dong, Synthesis Of Indoles, Indolines, And Carbazoles Via Palladium-Catalyzed C–H Activation, Green Synth. Catal., 2021, 2, 216–227 Search PubMed.
- M. S. Christodoulou, E. M. Beccalli, F. Foschi and S. Giofrè, Pd-Catalyzed Domino Reactions Involving Alkenes To Access Substituted Indole Derivatives, Synthesis, 2020, 52, A–AD Search PubMed.
- P. H. Li, H. Jiang, W. J. Zhang, Y. L. Li, M. C. Zhao, W. Zhou, L. Y. Zhang, Y. D. Tang, C. Z. Dong, Z. S. Huang and H. X. Chen, Synthesis of Carbazole Derivatives Containing Chalcone Analogs as Non-Intercalative Topoisomerase II Catalytic Inhibitors and Apoptosis Inducers, Eur. J. Med. Chem., 2018, 145, 498–510 CrossRef CAS PubMed.
- M. Bashir, A. Bano, A. S. Ijaz and B. A. Chaudhary, Recent Developments And Biological Activities of N-Substituted Carbazole Derivatives: A Review, Molecules, 2015, 20, 13496–13517 CrossRef CAS PubMed.
- J. Roy, A. K. Jana and D. Mal, Recent Trends In The Synthesis Of Carbazoles: An Update, Tetrahedron, 2012, 68, 6099–6121 CrossRef CAS.
- S. Maiti and P. Mal, Dehydrogenative Aromatic Ring Fusion For Carbazole Synthesis via C–C/C–N Bond Formation And Alkyl Migration, Org. Lett., 2017, 19, 2454–2457 Search PubMed.
- Y. Men, Z. Hu, J. Dong, X. Xu and B. Tang, Formal [1 + 2 + 3] Annulation: Domino Access To Carbazoles And Indolocarbazole Alkaloids, Org. Lett., 2018, 20, 5348–5352 Search PubMed.
- S. Ramesh and R. Nagarajan, Efficient One-Pot Multicomponent Synthesis Of (Carbazolylamino) Furan-2 (5H)-One And Carbazolyltetrahydropyrimidine Derivatives, Synthesis, 2011, 2011, 3307–3317 CrossRef.
- T. Nishiyama, T. Choshi, K. Kitano and S. Hibino, New Synthesis of Carbazole-1, 4-Quinone Using a Tandem Ring-Closing Metathesis and Dehydrogenation Reaction Under Oxygen Atmosphere, and Its Application to the Synthesis of Murrayaquinone A, Tetrahedron Lett., 2011, 52, 3876–3878 CrossRef CAS.
- T. N. Poudel and Y. R. Lee, Construction of Highly Functionalized Carbazoles via Condensation of an Enolate to a Nitro Group, Chem. Sci., 2015, 6, 7028–7033 RSC.
- Y. Qiu, J. Zhou, C. Fu and S. Ma, A General Diversified Synthesis of Carbazoles and the First Synthesis of Karapinchamine A, Chem.–Eur. J., 2014, 20, 14589–14593 CrossRef CAS PubMed.
- S. Noreen, A. F. Zahoor, S. Ahmad, I. Shahzadi, A. Irfan and S. Faiz, Novel Chiral Ligands for Palladium-Catalyzed Asymmetric Allylic Alkylation/Asymmetric Tsuji-Trost Reaction: A Review, Curr. Org. Chem., 2019, 23(11), 1168–1213 CrossRef CAS.
- J. M. Yang, M. L. Yao, J. C. Li, J. K. Liu and B. Wu, Access to Azepino-Annulated Benzo[c]carbazoles Enabled by Gold-Catalyzed Hydroarylation of Alkynylindoles and Subsequent Oxidative Cyclization, Org. Lett., 2022, 24, 6505–6509 CrossRef CAS PubMed.
- I. Martín, C. Aragoncillo and P. Almendros, Palladium-Catalyzed Hydroarylation of Homopropargyl Iodoindoles with Concurrent Alkyl and Iodonium Migrations, Adv. Synth. Catal., 2021, 363, 1449–1456 CrossRef.
- R. Jatoth, P. K. Naikawadi, B. Bhaskar, K. Gugulothu, P. Edukondalu and K. S. Kumar, Metal-Free TFA-Promoted Regioselective (Hetero)Arylation: Synthesis of (Hetero)Aryl Substituted and Carbazole/Oxepine Fused N-Heterocycles, Adv. Synth. Catal., 2022, 364, 1271–1276 CrossRef CAS.
- A. Banerjee, S. Sahu and M. S. Maji, Benzannulation of 2-Alkenylindoles using Aldehydes by Sequential Triple-Relay Catalysis: A Route to Carbazoles and Carbazole Alkaloids, Adv. Synth. Catal., 2017, 359, 1860–1866 CrossRef CAS.
- T. Wang and T. R. Hoye, Hexadehydro-Diels–Alder (HDDA)-Enabled Carbazolyne Chemistry: Single Step, De Novo Construction of the Pyranocarbazole Core of Alkaloids of the Murraya Koenigii (Curry Tree) Family, J. Am. Chem. Soc., 2016, 138, 13870–13873 CrossRef CAS PubMed.
- A. El-Harairy, Y. M. Yue, W. Fan, F. Popowycz, Y. Queneau, M. Li and M. Gu, Novel Non-toxic and Non-hazardous Solvent Systems for the Chemistry of Indoles: Use of a Sulfone-containing Brønsted Acid Ionic Liquid Catalyst in Butyl Acetate, ChemCatChem, 2019, 11, 4403–4410 Search PubMed.
- S. Kundu, A. Banerjee and M. S. Maji, Brønsted Acid-Catalyzed Tandem Pinacol-Type Rearrangement for the Synthesis of α-(3-Indolyl) Ketones by Using α-Hydroxy Aldehydes, J. Org. Chem., 2019, 84, 16003–16012 CrossRef CAS PubMed.
- D. Cao, G. Chen, D. Chen, Z. Xia, Z. Li, Y. Wang, D. Xu and J. Yang, Synthesis of 4-Hydroxycarbazole Derivatives by Benzannulation of 3-Nitroindoles with Alkylidene Azlactones, ACS Omega, 2021, 6, 16969–16979 CrossRef CAS PubMed.
- G. Karan, S. Sahu and M. S. Maji, A One-Pot ‘‘Back-to-Front’’ Approach for the Synthesis of Benzene Ring Substituted Indoles Using Allyl Boronic Acids, Chem. Commun., 2021, 57, 5274–5277 RSC.
- S. Singh, R. Samineni, S. Pabbaraja and G. Mehta, A General Carbazole Synthesis via Stitching of Indole–Ynones with Nitromethanes: Application to Total Synthesis of Carbazomycin A, Calothrixin B, and Staurosporinone, Org. Lett., 2019, 21, 3372–3376 CrossRef CAS PubMed.
- M. Faltracco, S. Ortega-Rosales, E. Janssen, R. C. Cioc, C. M. Vande Velde and E. Ruijter, Synthesis of Carbazoles by a Diverted Bischler–Napieralski Cascade Reaction, Org. Lett., 2021, 23, 3100–3104 CrossRef CAS PubMed.
- Y. B. C. Kumar, P. Samatha, P. S. Mainkar and S. Adepu, Rhodium Catalyzed Intramolecular Benzannulation for the Formation of Tetracyclic Carbazoles, Org. Biomol. Chem., 2022, 20, 9117 RSC.
- M. A. Muñoz-Torres, F. Martínez-Lara, M. Solas, S. Suarez-Pantiga and R. Sanz, “Back-to-Front” Indole and Carbazole Synthesis from N,N-Bis-(2- bromoallyl)amines by Combining Carbolithiation Reactions with Gold-Catalysis, Adv. Synth. Catal., 2022, 364, 3716–3724 Search PubMed.
- E. Yamuna, M. Zeller and K. J. R. Prasad, Microwave Assisted Synthesis of Indolo [2,3-b] Dibenzo [b,g][1, 8] Naphthyridines, Tetrahedron Lett., 2012, 53, 1514–1517 CrossRef CAS.
- W. Huang, Z. Gao, Z. Zhang, W. Fang, Z. Wang, Z. Wan, L. Shi, K. Wang and S. Ke, Selective and Effective Anticancer Agents: Synthesis, Biological Evaluation and Structure–Activity Relationships of Novel Carbazole Derivatives, Bioorg. Chem., 2021, 113, 104991 Search PubMed.
- A. Khan, R. Karim, H. Dhimane and S. Alam, Mild and Efficient Synthesis of Functionalized Carbazoles via a DBU-Assisted Sequence Involving Cu- and Pd-Catalyzed Coupling Reactions, ChemistrySelect, 2019, 4, 6598–6605 CrossRef CAS.
- S. Alam, R. Karim, A. Khan, A.
R. Mallick, N. Sepay and S. Ghosh, Microwave-Assisted Synthesis of Functionalized Carbazoles via Palladium-Catalyzed Aryl C–H Activation and Study of Their Interactions with Calf-Thymus DNA, Synth. Commun., 2022, 52(18), 1834–1855 CrossRef CAS.
- R. A. Irgashev, N. A. Kazin, G. A. Kim, G. L. Rusinov and V. N. Charushin, A New Synthetic Approach to Fused Nine-Ring Systems of the Indolo [3, 2-b] Carbazole Family Through Double Pd-Catalyzed Intramolecular C–H Arylation, RSC Adv., 2016, 6, 70106–70116 Search PubMed.
- S. W. Youn, Y. H. Kim and Y. H. Jo, Palladium-Catalyzed Regioselective Synthesis of 1- Hydroxycarbazoles Under Aerobic Conditions, Adv. Synth. Catal., 2019, 361, 462–468 Search PubMed.
- F. Martínez-Lara, A. Suárez, S. Suárez-Pantiga, M. José Tapia and R. Sanz, Straight Access to Highly Fluorescent Angular Indolocarbazoles via Merging Au- and Mo-Catalysis, Org. Chem. Front., 2020, 7, 1869–1877 RSC.
- S. Min, T. Kim, T. Jeong, J. Yang, Y. Oh, K. Moon, A. Rakshit and I. S. Kim, Synthesis of 2-Formyl Carbazoles via Tandem Reaction of Indolyl Nitrones with 2-Methylidene Cyclic Carbonate, Org. Lett., 2023, 25, 4298–4302 CrossRef CAS PubMed.
- R. Akhtar and A. F. Zahoor, Transition Metal Catalyzed Glaser and Glaser-Hay Coupling Reactions: Scope, Classical/Green Methodologies and Synthetic Applications, Synth. Commun., 2020, 50(22), 3337–3368 CrossRef CAS.
- A. Fürstner and P. W. Davies, Heterocycles by PtCl2-Catalyzed Intramolecular Carboalkoxylation or Carboamination of Alkynes, J. Am. Chem. Soc., 2005, 127, 15024–15025 CrossRef PubMed.
- S. D. Tambe, N. Iqbal and E. J. Cho, Nickel-Catalyzed trans-Carboamination across Internal Alkynes to Access Multifunctionalized Indoles, Org. Lett., 2020, 22, 1–5 CrossRef PubMed.
- W. Zhang, J. Bu, L. Wang, P. Li and H. Li, Sunlight-Mediated [3 + 2] Cycloaddition of Azobenzenes with Arynes: An Approach Toward the Carbazole Skeleton, Org. Chem. Front., 2021, 8, 5045–5051 RSC.
|
This journal is © The Royal Society of Chemistry 2024 |
Click here to see how this site uses Cookies. View our privacy policy here.