DOI:
10.1039/D4QO01122H
(Research Article)
Org. Chem. Front., 2024,
11, 4869-4873
Total synthesis of (–)-deglycocadambine†
Received
20th June 2024
, Accepted 9th July 2024
First published on 10th July 2024
Abstract
The first total synthesis of the monoterpene indole alkaloid (–)-deglycocadambine is achieved in 12 steps with (+)-genipin as the chiral starting material. The reported synthetic approach is characterized by an orchestrated cascade annulation between tryptamine and the highly functionalized dialdehyde precursor, rapidly introducing the unique 6/5/6/7/6-fused pentacyclic skeleton and the ketone functional group at C19 in a convergent manner. The successful implementation of transannular oxidative cyclization at C3 for bridged oxazolidine formation in the late-stage synthetic campaign ensured the final total synthesis of this molecule.
Introduction
(–)-Deglycocadambine (Fig. 1) is a member of the monoterpene indole alkaloids, a large family of metabolites that have attracted much attention from both synthetic and pharmaceutical chemists due to their high structural diversity and significant biological activities.1 First isolated from the twigs and leaves of Emmenopterys henryi by Song and Zhao in 2013,2 (–)-deglycocadambine has a characteristic 6/5/6/5/6/6 hexacyclic ring system, with its 6/5/6/7 tetracyclic nucleus containing a unique hydropyrido[1,2-a]azepine subunit, which can also be found in (–)-rubenine, (–)-kopsiyunnanine K, (–)-voacacines A and B and other cadambine-type alkaloids, as shown in Fig. 1.3 Although preliminary studies revealed no promising activity, scarcity in nature precludes its systematic biological evaluation.2 To the best of our knowledge, no synthetic route has been reported thus far for this molecule. Of note, in 1991, Brown et al. reported an elegant biologically inspired synthesis of (–)-cadambine from (–)-secologanin,4,5 wherein a well-designed chemo- and regioselective reaction between tryptamine and a highly functionalized epoxide was conducted to efficiently introduce the seven-membered azepine ring of (–)-cadambine. Theoretically, hydrolysis of (–)-cadambine should give the desired aglycone (–)-deglycocadambine. Accordingly, the exploration of a general synthetic route for the total synthesis of (–)-deglycocadambine is required to pursue the novel molecular architecture and investigate the potential molecular functionality. Based on a rationally designed two-fold annulation sequence for the rapid installation of the 6/5/6/7/6-fused pentacyclic core, we report our synthetic efforts toward (–)-deglycocadambine.
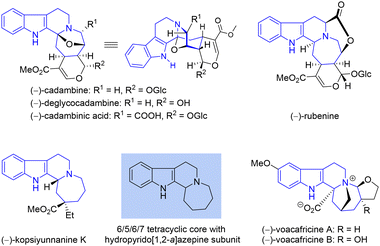 |
| Fig. 1 Representative indole alkaloids with hydropyrido[1,2-a]azepine subunits embedded in the 6/5/6/7-fused tetracyclic core. | |
Retrosynthetically, as shown in Scheme 1, (–)-deglycocadambine could be properly obtained from the pentacyclic precursor A by the formation of the bridged oxazolidine ring via the orchestrated transannular oxidative cyclization.6 The stereospecific hydroxyl group at C19 of A could theoretically be introduced via the diastereoselective reduction of the corresponding ketone in synthon B. The critical hydropyrido[1,2-a]azepine subunit embedded in B could be tentatively assembled by a rationally designed twofold annulation reaction between tryptamine and the highly functionalized dialdehyde C,7 the latter of which could be formally derived from the commercially available (+)-genipin by means of continuous functional group transformations.
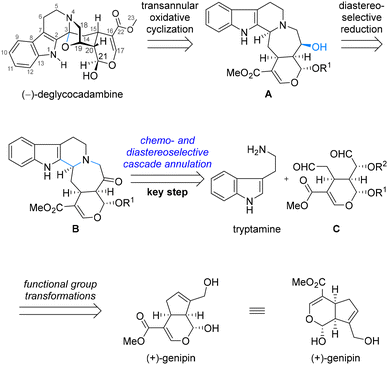 |
| Scheme 1 Retrosynthetic analysis of (−)-deglycocadambine. | |
Results and discussion
Initially, to access fragment 8 (Scheme 2), we selected commercially available iridoid (+)-genipin8 (∼$5 per g) from the chiral pool to start our synthetic campaign. Protection of both hydroxyl groups as TBS ethers was conducted in the presence of TBSCl and AgNO3, giving 1 in 92% yield. The following chemoselective dihydroxylation/oxidative cleavage cascade reaction of the isolated alkene in the five-membered ring was performed, affording compound 2 in 95% yield, with the left-hand alkene embedded in the conjugated system remaining intact. After the two-step operation involving the chemoselective protection of the aldehyde in 2 as the acetal and the diastereoselective reduction of the ketone in 3, alcohol 4 was produced as the major epimer (dr ∼ 10
:
1) in an overall yield of 81%. Notably, for the reductive transformation from 3 to 4, the observed stereochemistry at C19 could be explained by the Felkin–Anh model,9 wherein the Si face attack was preferred. With 4 in hand, esterification of the secondary alcohol in the presence of Ac2O gave, after chemoselective deprotection of the right-hand TBS ether, the primary alcohol 6. Finally, oxidation of the hydroxyl group in 6 using Dess–Martin periodinane followed by chemoselective hydrolysis of the ethylene glycol acetal in 7 delivered the dialdehyde 8 without incident.
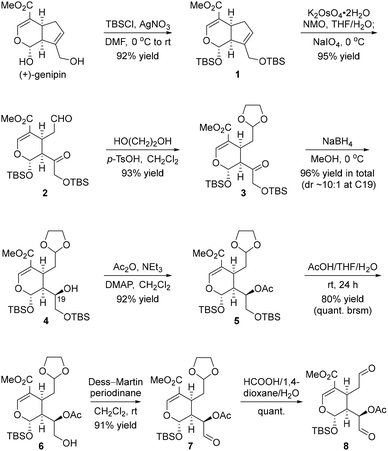 |
| Scheme 2 Synthesis of dialdehyde 8. | |
For the key cascade annulation of dialdehyde 8 and tryptamine, the pentacyclic ketone 11 was obtained as the sole identified product in 41% yield when the reaction mixture was exposed to TFA in CH2Cl2.10,11 In this process, as shown in Scheme 3, it was postulated that tryptamine firstly condensed chemoselectively with the sterically more accessible aldehyde 8 to form inter-1, followed by the second condensation, delivering inter-2-1. Inter-2-1 might isomerize to inter-2-2 and further to inter-2-3. Among these isomers existing in dynamic equilibration, inter-2-3 might be the most structurally favored for further aromatic substitution. The resulting pentacyclic compound 9 was further in situ hydrolyzed to give enol 10, which was spontaneously isomerized to the more stable ketone 11. The stereochemical outcome at C3 in 11 might be attributed to the much easier accessibility of the Re face of the iminium ion in inter-2-3 as molecular modelling of inter-2-3 revealed that the Si face attack presumably led to severe steric repulsion between the indole ring and H-15. Strategically, by using this elaborated twofold annulation protocol, we not only incorporated the synthetically challenging hydropyrido[1,2-a]azepine substructure but also finished the installation of ketone at C19 for further selective transformation at the same time. It should be noted that this is the first example for this cascade annulation wherein a complex and asymmetric dialdehyde was used to react with tryptamine.7,12
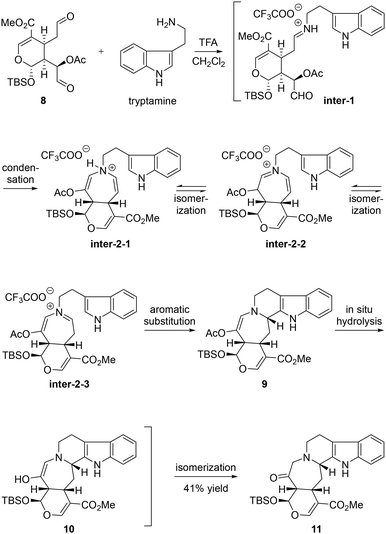 |
| Scheme 3 Synthesis of pentacyclic ketone 11. | |
With 11 in hand, as shown in Scheme 4, a chemo- and diastereoselective reduction of a ketone using L-selectride as the reducing reagent gave alcohol 12 in 84% yield. The I2-mediated transannular oxidative cyclization of the tertiary benzylic amine occurred smoothly to furnish compound 13, with the last bridged oxazolidine ring characterized by the N,O-substituted quaternary stereocenter at C3 assembled efficiently.6 When subjected to HCl in THF at elevated temperature, the TBS ether of 13 was directly deprotected to provide (–)-deglycocadambine, the absolute stereochemistry of which was unambiguously confirmed by X-ray crystallographic analysis.13 The 1H and 13C NMR spectroscopic data of the synthetic sample are in accordance with the reported ones.2,11
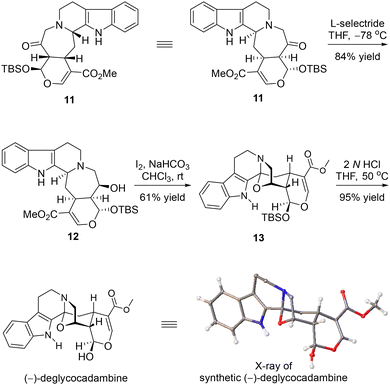 |
| Scheme 4 Late-stage synthesis of (–)-deglycocadambine. | |
Conclusions
In conclusion, a convergent synthetic strategy has been developed based on the ingenious use of a twofold annulation reaction between the dialdehyde and tryptamine to rapidly install the highly functionalized 6/5/6/7/6-fused pentacyclic ring system and a late-stage transannular oxidative cyclization to directly incorporate the bridged oxazolidine ring for the total synthesis of (–)-deglycocadambine, which was obtained in an overall yield of 9.5% over a longest linear sequence of 12 steps from (+)-genipin. Strategically, the bond-forming logic demonstrated in our protocol for installing the multicyclic ring system characterized by a unique hydropyrido[1,2-a]azepine subunit of (–)-deglycocadambine should enlighten the upcoming efforts towards chemical synthesis of other structurally related monoterpene indole alkaloids in our laboratory.
Author contributions
F.-X. W. and Y. R. C. directed the project. F.-X. W. and Y. R. C. conceived the synthetic route. F.-X. W., Y.-T. C. and H. L. conducted the synthetic work. F.-X. W., Y. R. C., Y.-T. C and H. L. analyzed the results. F.-X. W. and Y. R. C. wrote the manuscript. All authors commented on this manuscript and gave approval to the final version of this manuscript.
Data availability
Electronic supplementary information (ESI) available: Experimental procedures, NMR spectra, IR, and HRMS data. CCDC 2358655. For ESI and crystallographic data in CIF or other electronic format see https://doi.org/10.1039/D4QO01122H.
Conflicts of interest
There are no conflicts to declare.
Acknowledgements
We are grateful for financial support from the National Natural Science Foundation of China (22201050, 21772029, 21801051, 21807091, 21961006, 22071036, and 22061007); the Natural Science Foundation of Guangxi Zhuang Autonomous Region (2024GXNSFAA010091 and 2023GXNSFBA026329); the Singapore National Research Foundation under its Competitive Research Program (NRF-CRP22-2019-0002); the Ministry of Education, Singapore, under its MOE AcRF Tier 1 Award (RG7/20 and RG5/19), MOE AcRF Tier 2 (MOE2019-T2-2-117), Nanyang Technological University; the Frontiers Science Center for Asymmetric Synthesis and Medicinal Molecules, Department of Education, Guizhou Province [Qianjiaohe KY number (2020)004]; the 10 Talent Plan (Shicengci) of Guizhou Province ([2016]5649); the Science and Technology Department of Guizhou Province ([2018]2802 and [2019]1020); the State Key Laboratory for Chemistry and Molecular Engineering of Medicinal Resources (CMEMR2022-A01); Guangxi Normal University; the Program of Introducing Talents of Discipline to Universities of China (111 Program, D20023) at Guizhou University; and Guizhou University.
References
- For recent reviews on chemistry and biology of monoterpene indole alkaloids, see:
(a) X.-Y. Liu and Y. Qin, Indole Alkaloid Synthesis Facilitated by Photoredox Catalytic Radical Cascade Reactions, Acc. Chem. Res., 2019, 52, 1877–1891 CrossRef CAS PubMed;
(b) H. Takayama, Total Syntheses of Lycopodium and Monoterpenoid Indole Alkaloids Based on Biosynthesis-Inspired Strategies, Chem. Pharm. Bull., 2020, 68, 103–116 CrossRef CAS PubMed;
(c) A. E. Mohammed, Z. H. Abdul-Hameed, M. O. Alotaibi, N. O. Bawakid, T. R. Sobahi, A. Abdel-Lateff and W. M. Alarif, Chemical Diversity and Bioactivities of Monoterpene Indole Alkaloids (MIAs) from Six Apocynaceae Genera, Molecules, 2021, 26, 488 CrossRef CAS PubMed;
(d) X.-Y. Liu and Y. Qin, Recent advances in the total synthesis of monoterpenoid indole alkaloids enabled by asymmetric catalysis, Green Synth. Catal., 2022, 3, 25–39 CrossRef CAS;
(e) L. Wang, S. Chen, X. Gao, X. Liang, W. Lv, D. Zhang and X. Jin, Recent progress in chemistry and bioactivity of monoterpenoid indole alkaloids from the genus gelsemium: a comprehensive review, J. Enzyme Inhib. Med. Chem., 2023, 38, 2155639 CrossRef PubMed.
- For the isolation of (–)-deglycocadambine, see: X.-D. Wu, L. Wang, J. He, X.-Y. Li, L.-B. Dong, X. Gong, X. Gao, L.-D. Song, Y. Li, L.-Y. Peng and Q.-S. Zhao, Two New Indole Alkaloids from Emmenopterys henryi, Helv. Chim. Acta, 2013, 96, 2207–2213 CrossRef CAS.
- Efficient synthetic methods for the rapid installation of this 6/5/6/7 tetracyclic nucleus characterized by a unique hydropyrido[1,2-a]azepine subunit in the synthesis of indole natural products and the analogues are very limited. For one example, see: N. M. Vlaskina, K. F. Suzdalev, M. N. Babakova, V. V. Mezheritskii and V. G. Kartsev, Use of the tert-amino effect in the synthesis of spirocyclic fused α-carbolines, Russ. Chem. Bull., 2006, 55, 384–386 CrossRef CAS.
- For the only report on the chemical synthesis of (–)-cadambine, see: R. T. Brown, D. M. Duckworth and C. A. M. Santos, Biogenetically patterned synthesis of cadambine, Tetrahedron Lett., 1991, 32, 1987–1990 CrossRef CAS.
- Although (–)-secologanin is commercially available, it is priced high. The cost of a 5 mg-scale of (–)-secologanin ranges from $88 to 438 worldwide, which severely hampered its synthetic application as a chiral starting material. Synthetically, 10 steps were needed to access (–)-secologanin. For the only report on the chemical synthesis of enantiopure (–)-secologanin, see: K. Rakumitsu, J. Sakamoto and H. Ishikawa, Total Syntheses of (–)-Secologanin, (–)-5-Carboxystrictosidine, and (–)-Rubenine, Chem. – Eur. J., 2019, 25, 8996–9000 CrossRef CAS.
- For selected examples on the oxidative cyclization to form the N,O-ketal at the benzylic position in the total synthesis of indole alkaloids, see:
(a) I. Moldvai, C. Szantay Jr. and C. Szantay, Synthesis of Vinca Alkaloids and Related Compounds. Part 97. Epimerization with the Aid of Iodine, Heterocycles, 2001, 55, 2147–2155 CrossRef CAS;
(b) L. Li, P. Aibibula, Q. Jia and Y. Jia, Total Syntheses of Naucleamides A−C and E, Geissoschizine, Geissoschizol, (E)-Isositsirikine, and 16-epi-(E)-Isositsirikine, Org. Lett., 2017, 19, 2642–2645 CrossRef CAS PubMed. For selected examples on a similar transformation in the total synthesis of isoquinoline alkaloids, see:
(c) Q. Tan, Z. Yang, D. Jiang, Y. Cheng, J. Yang, S. Xi and M. Zhang, Copper-Catalyzed Aerobic Oxidative Cyclization Cascade to Construct Bridged Skeletons: Total Syntheses of (–)-Suaveoline, Angew. Chem., Int. Ed., 2019, 58, 6420–6424 CrossRef CAS;
(d) B. M. Trost, C.-I. J. Huang and Z. Jiao, Enantioselective Divergent Syntheses of (+)-Bulleyanaline and Related Isoquinoline Alkaloids from the Genus Corydalis, J. Am. Chem. Soc., 2019, 141, 16085–16092 CrossRef CAS PubMed.
- For the pioneering work on the condensation of glutaraldehyde with tryptamine, see: G. W. Gribble, A Convenient Synthesis of 1,2,3,4,6,7,12,12b-Octahydroindolo[2,3-a]quinolizine, J. Org. Chem., 1972, 37, 1833–1835 CrossRef CAS.
- For selected examples on the total synthesis of natural products with (+)-genipin as the chiral starting material, see:
(a) W. Zhang, M. Ding, J. Li, Z. Guo, M. Lu, Y. Chen, L. Liu, Y.-H. Shen and A. Li, Total Synthesis of Hybridaphniphylline B, J. Am. Chem. Soc., 2018, 140, 4227–4231 CrossRef CAS PubMed;
(b) Y. Gao, Y. Wei and D. Ma, Synthetic Studies toward Plumisclerin A, Org. Lett., 2019, 21, 1384–1387 CrossRef CAS PubMed.
- For one review on the Felkin–Anh model, see: A. Mengel and O. Reiser, Around and beyond Cram's Rule, Chem. Rev., 1999, 99, 1191–1223 CrossRef CAS.
- The absolute configuration at C3 in compounds 11 and 12 was determined by comprehensive 1D and 2D NMR spectroscopic analysis of compound 12, wherein H-3 showed strong NOE signals with H-19 and H-20, respectively.
- For details, see the ESI.†.
- For selected recent examples on the total synthesis of natural products using twofold annulation of tryptamine and the analogues, see:
(a) E. R. Miller, M. T. Hovey and K. A. Scheidt, A Concise, Enantioselective Approach for the Synthesis of Yohimbine Alkaloids, J. Am. Chem. Soc., 2020, 142, 2187–2192 CrossRef CAS;
(b) S.-H. Wang, R.-Q. Si, Q.-B. Zhuang, X. Guo, T. Ke, X.-M. Zhang, F.-M. Zhang and Y.-Q. Tu, Collective Total Synthesis of Aspidofractinine Alkaloids through the Development of a Bischler-Napieralski/Semipinacol Rearrangement Reaction, Angew. Chem., Int. Ed., 2020, 59, 21954–21958 CrossRef CAS;
(c) L. Yang, S. Huang, R. Huang, A. Hou, S. Zhang, H. Su, X. Ding, B. Lin, M. Cheng and Y. Liu, Total Syntheses of Aspidospermidine, N-Methylaspidospermidine, N-Acetylaspidospermidine, and Aspidospermine via a Tandem Cyclization of Tryptamine-Ynamide, Org. Lett., 2021, 23, 6471–6476 CrossRef CAS PubMed;
(d) A. Nakayama, T. Nakamura, T. Zaima, S. Fujimoto, S. Karanjit and K. Namba, Concise Total Synthesis of Tronocarpine, Angew. Chem., Int. Ed., 2021, 60, 635–639 CrossRef CAS PubMed;
(e) L.-P. Zhao, P.-J. Li, L. Wang and Y. Tang, Allenamide-Initiated Cascade [2 + 2 + 2] Annulation Enabling the Divergent Total Synthesis of (–)-Deoxoapodine, (–)-Kopsifoline D and (±)-Melotenine A, Angew. Chem., Int. Ed., 2022, 61, e202207360 CrossRef CAS;
(f) D. L. Cain, N. A. Anderson, D. B. Cordes, A. M. Z. Slawin and A. J. B. Watson, Total Synthesis of (±)-Aspidospermidine, (±)-Aspidofractinine, (±)-Limaspermidine, and (±)-Vincadifformine via a Cascade and Common Intermediate Strategy, J. Org. Chem., 2022, 87, 15559–15563 CrossRef CAS PubMed;
(g) Y.-J. Cheng, L.-P. Zhao, L. Wang and Y. Tang, Catalytic [2 + 2 + 2] Tandem Cyclization with Alkyl Substituted Methylene Malonate Enabling Concise Total Synthesis of Four Malagasy Alkaloids, CCS Chem., 2023, 5, 124–132 CrossRef CAS;
(h) Y. Wu, M. Wang, M. Xu and S. Liu, Rapid Total Synthesis of Iheyamine A via a Pictet−Spengler/Redox-Mediated Cyclization Cascade, J. Org. Chem., 2023, 88, 4814–4817 CrossRef CAS PubMed;
(i) M. Tang, H. Lu and L. Zu, Collective total synthesis of stereoisomeric yohimbine alkaloids, Nat. Commun., 2024, 15, 941 CrossRef CAS.
- CCDC 2358655† for (–)-deglycocadambine contains the supplementary crystallographic data for this paper.
Footnotes |
† Electronic supplementary information (ESI) available: Experimental procedures, NMR spectra, IR, and HRMS data. CCDC 2358655. For ESI and crystallographic data in CIF or other electronic format see DOI: https://doi.org/10.1039/d4qo01122h |
‡ These authors contributed equally to this work. |
|
This journal is © the Partner Organisations 2024 |
Click here to see how this site uses Cookies. View our privacy policy here.