DOI:
10.1039/D3QO01736B
(Research Article)
Org. Chem. Front., 2024,
11, 2720-2726
Convenient syntheses of trans-resveratrol 3-O and 4′-O-β-D-glucuronides and a study of their aqueous stability†
Received
23rd October 2023
, Accepted 22nd November 2023
First published on 22nd November 2023
Abstract
The two isomeric monoglucuronides of the stilbene triol derivative, resveratrol, are thought to have potentially valuable biological activities, including possible antiproliferative properties, in common with resveratrol itself. In connection with a wider interest in naturally occurring phenolic glucuronides, we sought access to both of these conjugates from readily available resveratrol triesters. Selective monodeacylation using either chemical or enzymatic hydrolyses afforded the required diesters: subsequent glucuronidation using the trichloroacetimidate procedure, then mild hydrolysis, afforded the desired products. We also discovered a very mild, effective method for the anomeric deacetylation of the readily available methyl glucuronate β-tetraacetate using N-Me piperazine en route to the imidate. This reagent appears to have wider value in the deprotection of relatively activated acetates. We further studied the stability of the glucuronides in aqueous solution, and found remarkable differences in their properties, especially in the ready E/Z isomerisation of the 3-glucuronide.
Introduction
While it is still commonly believed that glucuronides of xenobiotics and endogenous materials are mere biological waste byproducts, an increasing body of evidence has found that many glucuronides have biological activity per se.1 A well-known example is morphine-6-glucuronide (M6G) 1, which has some advantages over morphine itself as an analgesic: better tolerance, especially reduced respiratory depression, and longer duration of action.2–4 We have recently shown that p-cresyl glucuronide 2, an end product of Tyr metabolism in the gut, is significantly less toxic in human kidney cells than the parent phenol and may indeed possess cytoprotective properties.5,6
As part of our continuing programme, we sought to study the behaviour of other glucuronides of endogenous and dietary substances: the isomeric 3-
O and 4′-
O-β-
D-glucuronides of the stilbenetriol, resveratrol
3 were of particular interest. Resveratrol, present in various plants, nuts and red wine skins, has been associated with diverse biological activities and health benefits,
7–9 generally thought to be associated with its radical scavenging properties. It occurs naturally mainly as the
trans (
E) isomer shown, but the
cis (
Z) isomer
3′ is always present in lesser amounts.
10 Interest in
3 was greatly spurred by the discovery of its cancer chemopreventive effect:
8b more recently, the relative effects of
3 and its glucuronide metabolites in lowering hepatic cholesterol have been studied.
11
Clearly, efficient syntheses of the two glucuronides
4 and
5 will depend on selective preparations of suitable di-protected intermediates. Other preparations of
4 and
5 have proceeded
via ab initio synthesis of
3 from benzenoid precursors,
12 or the use of regiospecifically silylated derivatives.
13,14 Also Koenigs–Knorr glucuronidation of
3 itself, followed by deprotection and preparative HPLC of the resulting mixture of isomers has been used.
14 The enzymology and uridine diphosphate glucuronosyltransferase (UGT) specificity in the human glucuronidation of both
3 and
3′, including gender differences, have been studied.
15,16
We considered that resveratrol triacetate
6, commercially available or readily prepared from
3 in essentially quantitative yield,
17 should be a convenient precursor. Indeed, others have described selective monodeacylation of
6 using chemical and enzymatic methods,
18,19 with conversion of the 3,4′-diacetate into
4,
18 and we aimed to build on these results.
Regarding the conjugation step, our recent synthesis5 of p-cresyl glucuronide 2 was a good example of successful glucuronidation of a phenol of low acidity with the anomeric tetraacetate 7. However, the success of this straightforward method appears critically dependent on phenolic pKa: any substrate more acidic than phenol itself (pKa = 10.0) cannot be readily glucuronidated in this way, and we verified that this was true with resveratrol diacetates once available. A recent thorough study20 gave a value of pKa1 = 9.2 for 3, with the 4′-OH being the most acidic. Resorcinol (1,3-dihydroxybenzene) also has an experimental pKa1 of 9.2
21 (the authors determined a theoretical value of 9.07) and the values for monoacylated derivatives are expected to be lower. We therefore selected the trichloroacetimidate method22 and found a valuable new method for efficient anomeric deacylation of 7 under mild conditions, giving 8 which after conversion to 9 (Scheme 1) was conjugated with the appropriate diesters of 3 followed by deprotection of the products to afford the desired monoglucuronides.
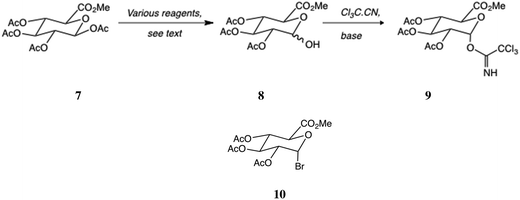 |
| Scheme 1 Carbohydrate intermediates in the glucuronic acid series. | |
Results and discussion
Carbohydrate intermediates
Several reagents have been used for the conversion of tetraester 7 into hemiacetal 8 (Scheme 1), including ammonium acetate,23 ammonium carbonate,24 hydrazine25,26 (with or without buffering), morpholine,27 benzylamine28 and a tin-based reagent.29 In addition the longer route via heavy metal-catalysed hydrolysis of the bromosugar 10 derived from 7 is still used.1 We felt that there was still room for improvement in view of the toxicity of some of the reagents used and the inhomogeneity of the ammonium salts. As a result, we screened a number of amine reagents, aiming for homogeneous conditions and the avoidance of metal reagents as a minimum.
We were pleased to find that N-methylpiperazine, pKa1 = 9.14,30 (and a very weakly basic pKa2 of 4.73) performed extremely well in this reaction, Scheme 1. The best conditions called for 3 eq. of the amine buffered with equimolar acetic acid in acetonitrile: after 24 h at 20 °C, reaction was complete, and after neutralization the hemiacetal 8 was extracted in 88% yield, pure enough for immediate progression. It was converted to the trichloroacetimidate 9 as described previously.31
Resveratrol diesters: chemical hydrolysis
We studied both selective chemical and enzymic hydrolyses to convert triacetate 6 into the 3,4′-and 3,5-diesters (Scheme 2). Reaction of 6 with NH4OAc has been used for synthesis of the 3,4′-diester 11 in 41% yield,18 but the elaborate procedure called for two crystallizations in addition to chromatography. The authors reported other possible conditions: catalytic K2CO3/EtOH, catalytic HCl/EtOH, catalytic DBU/EtOH/CH2Cl2 and catalytic Ph3P/EtOH at reflux. Using their optimum procedure, viz. NH4OAc in MeOH/THF, we could only obtain 21% of 11, together with the 3,5-diester and mixed monoesters. Here too, however, N-Me piperazine buffered with AcOH was a superior alternative and allowed 11 to be isolated in 36% yield by direct chromatography, in sufficient amount to obtain useful quantities of the 3-glucuronide.
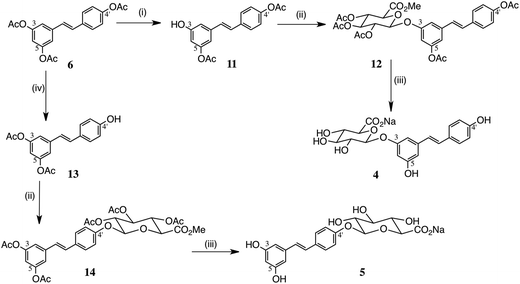 |
| Scheme 2 Synthesis of resveratrol monoglucuronides. Reagents: (i) N-Me piperazine, AcOH, MeCN, 20 °C; (ii) 9, BF3·OEt2, CH2Cl2, −20 °C; (iii) Na2CO3, aq. MeOH, 0 °C to 20 °C, then IR-120 (H+) to pH 6.2, or see text; (iv) Cal-B, n-BuOH, TBME, 45 °C. | |
We also studied resveratrol tri-isobutyrate 15, Scheme 3, hoping that the additional bulk of the esters might allow a more controlled, selective hydrolysis. Compound 15 was obtained by acylation of 3 with isobutyryl chloride in essentially quantitative yield. However, there was only a slight improvement in the mono-deacylation step. The resulting 3,4′-diisobutyrate 16, however, gave a better yield of 17 in the glucuronidation step.
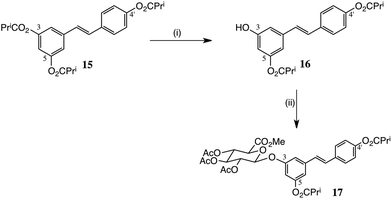 |
| Scheme 3 Isobutyryl intermediates. Reagents: as Scheme 2. | |
Resveratrol diesters: enzymic hydrolysis
Nicolosi et al. described the preparation of a number of resveratrol esters using enzymatic transesterification:19 in particular, the 3,5-diester 13, ideal for the synthesis of the 4′-glucuronide, was obtained in high yield by treatment of 6 with Burkholderia cepacia and n-butanol. We did not have access to this particular enzyme, but we studied immobilized Candida enzymes, CAL A and CAL B, which are known to be valuable in transesterification.32 While CAL A gave a virtually equal mixture of the 3,5- and 3,4′-diesters in moderate yield in a slow reaction (ca. 50% conversion after 72 h), CAL B showed high selectivity for removal of the 4′-acetate in just 1 h at 45 °C. In this way, following removal of the enzyme and chromatography, or direct crystallisation, the 3,5-diester 13 was obtained in 75% yield.
Glucuronidation
Both diesters 11 and 13 were successfully glucuronidated using trichloroacetimidate 9, affording 12 and 14 in 53% and 56% yields respectively, employing BF3·OEt2 as the Lewis acid catalyst. Similarly, the 3, 4′-diisobutyrate 16 gave the related conjugate 17 in 66% yield. Neither diester gave any useful product on Lewis acid-catalysed reaction with the β-tetraacetate 7, using TMSOTf or BF3·OEt2.
Hydrolysis was performed using the mild conditions of Na2CO3 in aq. methanol, and the products 4 and 5 were finally isolated as their Na salts.31 Alternatively, in this series, somewhat purer products were obtained by first isolating the free glucuronic acid, following acidification to pH 3 using IR-120 (H+) resin. The sodium salts were then obtained by adding an equimolar amount of aq. NaHCO3. The NMR spectra (see ESI†) were consistent with published data; Na salts of glucuronic acids show an upfield shift of the H(5) proton, typically by about 0.1 ppm, compared to the free acid forms.
Aqueous stability of the glucuronides
When the 1H NMR of the 3-glucuronide 4 was recorded in D2O, we found that small extra signals (sc. <5%) were visible in the aryl region after leaving the solution at 20 °C for several hours on the bench. The NMR spectrum showed two AB quartets which can be assigned to pairs of olefinic Hs. The multiplets have been simulated (see ESI†) to obtain the chemical shifts and coupling constants (δ 7.11, 6.93 and 6.61, 6.46) with J = 16.5 Hz and 12.1 Hz respectively An nOe is observed between the resonances of the AB quartet around 6.5 ppm but is not observed between the resonances of the AB quartet around 7 ppm consistent with the assignment of these resonances to the olefinic protons of the Z and E isomers of 4 respectively.
Transformation of the 4(E) to the 4(Z) isomer occurs on exposure of the solution in the NMR tube to light but is reversed on storage in the dark. Thus, after one week in dull ambient light, the spectrum showed the presence of two compounds in a roughly 2
:
1 ratio. On returning the NMR tube to the refrigerator, after ca. 7 days the 1H NMR spectrum revealed the sample was >90% E, (Fig. 1a) and after a further 7 days in the dark of the NMR magnet the sample was 96% E, (Fig. 1b) i.e. the Z to E isomerization is slow and happens in the dark. On exposing the sample to bright sunlight for ca. 8 h the NMR spectrum revealed the sample had converted to ca. 90% Z together with a small amount of decomposition (Fig. 1c). Finally, after seven days in the NMR magnet conversion back to the E isomer, which was now present as ca. 45% of the sample, had occurred (Fig. 1d).
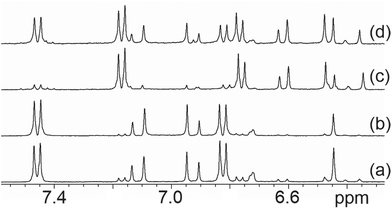 |
| Fig. 1 Time course of the E to Z isomerization of 4: 1H NMR of the aryl region: (a) after ca. 1 week in the dark E 90%, Z 10%; (b) after 2 weeks E 96%, Z 4%; (c) after ca. 8 h bright sunlight E 11%, Z 89%; (d) after further 5 days in dark E 45%, Z 55%. | |
While such isomerization is well known for 3 and 3′ themselves,10b employing a 450 W medium pressure mercury lamp, it has not been reported for the glucuronides, and the conditions are remarkably mild. In the ESI† we present a full assignment of the 1H and 13C spectra of both isomers, with HSQC, HMBC and nOe difference analyses.
In contrast, under identical conditions the isomeric 4′-glucuronide
5 showed essentially no
E/
Z isomerisation after 120 h. Instead, complete H/D exchange of the aryl Hs of the resorcinol ring was observed (see the spectrum in ESI
†) after just 24 h, giving the tri-deuterio species
5-d3.
33 While this is in a sense not surprising, as H/D exchange of aryl protons is quite facile in phenols, it is clear that exchange under these very mild conditions requires the free resorcinol group. The 3-glucuronide
4, where only free
monophenols are present, showed no observable H/D exchange under these conditions.
These results may be rationalized by postulating that the free 4-phenol is necessary for facile E/Z isomerisation: see Scheme 4. Ionisation of this phenol, followed by one-electron oxidation (or direct air/light-catalysed H abstraction from starting material) generates a phenoxy radical that is directly conjugated to the double bond, allowing an isomeric C radical to form, which is disposed to revert to the isomeric phenoxy radical. This mechanism is clearly inaccessible to the 4′-glucuronide 5.
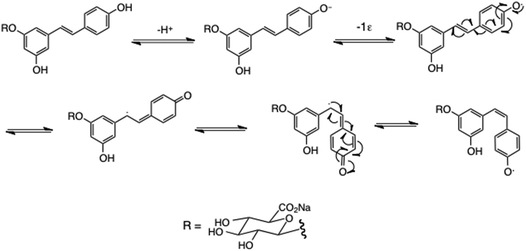 |
| Scheme 4 Proposed mechanism for the E/Z isomerisation of glucuronide 4. | |
E/Z isomerisation of resveratrol diesters
We studied the pair of diesters 13 and 16 to look for similar behaviour, in particular to investigate whether the position of the free phenol was important for the rate of E/Z isomerisation as observed with 4 and 5. These compounds are water-insoluble, but d3-acetonitrile was a satisfactory solvent for both. When these solutions were stored at 20 °C in the dark, no significant E/Z isomerisation was observed for either isomer after 12 h, but in diffused sunlight over a further 12 h both underwent isomerisation, with the 3,5-diester 13 reacting at least 5× faster; see the spectra in the ESI.† While these further experiments have introduced new variables (substituent groups, solvent), the faster isomerisation of 13, which contains a 4′-OH group, is consistent with the behaviour of 4 and 5. We shall report our findings on E/Z isomerisation of a series of resveratrol derivatives elsewhere.
Conclusions
Convenient syntheses of both isomeric resveratrol monoglucuronides have been achieved from the readily available triacetate, using selective chemical and enzymatic deprotections to access the necessary diesters. The glucuronidation step was performed in good yields for both isomers using the trichloroacetimidate method, and mild final deprotection was carried out using aqueous Na2CO3 solution with methanol. In the course of these studies, we discovered that N-methylpiperazine was a highly suitable reagent for both selective deacetylation of the anomeric glucuronate perester and cleavage of a single ester from resveratrol triacetate.
The final glucuronides, as their Na salts in D2O, showed very different properties, namely ready E/Z alkene isomerisation in the case of 4 and H/D exchange in the case of 5, mutually exclusive. We shall report the results of biological investigations of the products in future publications.
Author contributions
MF: investigation, formal analysis, experimental write-up. AG: investigation, formal analysis, experimental write-up. EY: project administration, supervision, editing of MS. KB: investigation, formal analysis. JI: NMR experimental design and NMR methodology, formal analysis, editing of MS. AS: conceptualization, supervision, formal analysis, experimental verification, MS write-up.
Conflicts of interest
We have no conflicts of interest to declare.
Acknowledgements
We are grateful to the EPSRC for funding (summer internship to A. G.). M. K. F. was a final year M. Chem. project student at the University of Liverpool, 2021–2022.
References
- A. V. Stachulski and X. L. Meng, Glucuronides from metabolites to medicines: a survey of the in vivo generation, chemical synthesis and properties of glucuronides, Nat. Prod. Rep., 2013, 30, 806–848 RSC
.
-
(a) R. J. Osborne, S. P. Joel, N. S. Nixon and M. L. Slevin, The Analgesic Activity of Morphine-6-glucuronide, Lancet, 1988, 331, 828 CrossRef PubMed
;
(b) R. J. Osborne, P. I. Thompson, S. P. Joel, D. Trew, N. Patel and M. Slevin, The Analgesic Activity of Morphine-6-glucuronide, Br. J. Clin. Pharmacol., 1992, 34, 130–138 CrossRef CAS PubMed
.
- R. T. Brown, N. E. Carter, F. Scheinmann and N. J. Turner, Synthesis of morphine-6-glucuronide via a highly selective enzyme catalysed hydrolysis reaction, Tetrahedron Lett., 1995, 36, 1117–1120 CrossRef CAS
.
- A. V. Stachulski, F. Scheinmann, J. R. Ferguson, J. L. Law, K. W. Lumbard, P. Hopkins, N. Patel, S. Clarke, A. Gloyne and S. P. Joel, Structure-Activity Relationships of Some Opiate Glycosides, Bioorg. Med. Chem. Lett., 2003, 13, 1207–1214 CrossRef CAS PubMed
.
- J. A. London, E. C. S. Wang, I. L. Barsukov, E. A. Yates and A. V. Stachulski, Synthesis and toxicity profile in 293 human embryonic kidney cells of the β-D-glucuronide derivatives of ortho-, meta- and para-cresol, Carbohydr. Res., 2021, 499, 108225 CrossRef CAS PubMed
.
- A. V. Stachulski, T. B.-A. Knausenberger, S. N. Shah, L. Hoyles and S. McArthur, The host/gut microbial co-metabolite of aromatic amino acids, p-cresol glucuronide, promotes blood–brain barrier integrity in, vivo, Tissue Barriers, 2023, 11, e2073175 CrossRef PubMed
.
-
(a)
C. Wilson, XPharm: The Comprehensive Pharmacology Reference, 2010, pp. 1–9 Search PubMed
;
(b) T. Rezanka, I. Kolouchova, L. Gharwalova and K. Sigler, Metabolic Screening of Wine (Grapevine) resveratrol, Stud. Nat. Prod. Chem., 2018, 59, 1–30 CrossRef CAS
.
-
(a) J. A. Baur and D. A. Sinclair, Therapeutic Potential of Resveratrol: The in Vivo Evidence, Nat. Rev. Drug Discovery, 2006, 5, 493–506 CrossRef CAS PubMed
;
(b) M. S. Jang, E. N. Cai, G. O. Udeani, K. V. Slowing, C. F. Thomas, C. W. W. Beecher, H. H. S. Fong, N. R. Farnsworth, A. D. Kinghorn, R. G. Mehta, R. C. Moon and J. M. Pezzuto, Cancer chemopreventive activity of resveratrol, a natural product derived from grapes, Science, 1997, 275, 218–220 CrossRef CAS PubMed
.
- C. Iuga, J. R. Alvarez-Idaboy and N. Russo, Antioxidant activity of trans-resveratrol towards hydroxyl and hydroperoxyl radicals: a quantum chemical and computational kinetics study, J. Org. Chem., 2012, 77, 3868–3877 CrossRef CAS PubMed
.
-
(a) N. Y. Anisimova, M. V. Kiselevsky, A. V. Sosnov, S. V. Sadovnikov, I. N. Stankov and A. A. Gakh, Trans, cis, and dihydro-resveratrol: a comparative study, Chem. Cent. J., 2011, 5, 88 CrossRef CAS PubMed
. These authors point out that dihydro-3 is also present in vivo;
(b) R. A. Rodriguez, I. R. Lahoz, O. N. Faza, M. M. Cid and C. S. Lopez, Theoretical and experimental exploration of the photochemistry of resveratrol: beyond the simple double bond isomerization, Org. Biomol. Chem., 2012, 10, 9175–9182 RSC
.
- D. Shao, Y. Wang, Q. Huang, J. Shi, H. Yang, Z. Pan, M. Jin, H. Zhao and X. Xu, Cholesterol-Lowering Effects and Mechanisms in view of Bile Acid Pathway of Resveratrol and Resveratrol Glucuronides, J. Food Sci., 2016, 81, H2841–H2848 CrossRef CAS PubMed
.
- D. Learmonth, A Concise Synthesis of the 3-O-β-D- and 4′-O-β-D-Glucuronide Conjugates of trans-Resveratrol, Bioconjugate Chem., 2003, 14, 262–267 CrossRef CAS PubMed
.
- R. Lucas, D. Alcantara and J. C. Morales, A concise synthesis of glucuronide metabolites of urolithin-B, resveratrol and hydroxytyrosol, Carbohydr. Res., 2009, 344, 1340–1346 CrossRef CAS PubMed
.
- L.-X. Wang, A. Hereida, H. Song, Z. Zhang, B. Yu, C. Davis and R. Redfield, Resveratrol glucuronides as the metabolites of resveratrol in humans: Characterisation, synthesis and anti-HIV activity, J. Pharm. Sci., 2004, 93, 2448–2457 CrossRef CAS PubMed
.
- V. Aumont, S. Krisa, E. Battaglia, P. Netter, T. Richard, J.-M. Merillon, J. Magdalou and N. Sabolovic, Regioselective and stereospecific glucuronidation of trans- and cis-resveratrol, Arch. Biochem. Biophys., 2001, 393, 281–289 CrossRef CAS PubMed
.
- R. W. Dellinger, A. M. G. Garcia and F. L. Meyskens Jr., Differences in the glucuronidation of resveratrol and pterostilbene: altered enzyme stereospecificity and potential gender differences, Drug Metab. Pharmacokinet., 2014, 29, 112–119 CrossRef CAS PubMed
.
-
(a) G. S. Jayatilake, B. J. Baker and J. B. McClintock, Isolation and Identification of a Stilbene Derivative from the Antarctic Sponge Kirkpatrickia variolosa, J. Nat. Prod., 1995, 58, 1958–1960 CrossRef CAS
;
(b) B. Ruan, X. Huang, H. Ding, C. Xu, H. Ge, H. Zhu and R. Tan, Synthesis and cytotoxic evaluation of a series of resveratrol derivatives, Chem. Biodiversity, 2006, 3, 975–981 CrossRef CAS PubMed
.
- C. S. Jungong and A. V. Novikov, Practical Preparation of Resveratrol 3-O-β-D-glucuronide, Synth. Commun., 2012, 42, 3589–3597 CrossRef CAS PubMed
.
- G. Nicolosi, C. Spatafora and C. Tringali, Chemo-enzymatic preparation of resveratrol derivatives, J. Mol. Catal. B: Enzym., 2002, 16, 223–229 CrossRef CAS
.
-
(a) L. Zimanyi, S. Thekkan, B. Eckert, A. R. Condren, O. Dmitrenko, L. R. Kuhn, I. V. Alabugin and J. Saltiel, Determination of the pKa Values of trans-Resveratrol, a Triphenolic Stilbene, by Singular Value Decomposition. Comparison with Theory, J. Phys. Chem., 2020, 124, 6294–6302 CrossRef CAS PubMed
;
(b) A valuable broad study of the pKas of many phenols, comparing theoretical and experimental values, was given by M D. Liptak, K. C. Gross, P. G. Seybold, S. Feldgus and G. C. Shields, Absolute pKa determinations for Substituted Phenols, J. Am. Chem. Soc., 2002, 124, 6421–6427 CrossRef PubMed
.
- S. E. Blanco, M. C. Almandoz and F. H. Ferretti, Determination of the overlapping pKa values of resorcinol using UV-visible spectroscopy, Spectrochim. Acta, Part A, 2005, 61, 93–102 CrossRef CAS PubMed
.
-
(a) R. R. Schmidt, New Methods for the Synthesis of Glycosides and Oligosaccharides-Are There Alternatives to the Konigs-Knorr Method? [New Synthetic Methods (56)], Angew. Chem., Int. Ed. Engl., 1986, 25, 212–235 CrossRef
;
(b) A. Nudelman, J. Herzig, H. E. Gottlieb, E. Keinan and J. Sterling, Selective deacetylation of anomeric sugar acetates with tin alkoxides, Carbohydr. Res., 1987, 162, 145–152 CrossRef CAS
, as applied to the glucuronic acid series.
-
(a) Y. J. Lu, Y. Liu, M. Prashad and W.-C. Shieh, Synthesis of vildagliptin-β-O-glucuronide, Adv. Chem. Eng. Sci., 2012, 2, 379–383 CrossRef CAS
;
(b) S. Chittaboina, B. Hodges and Q. Wang, A Facile Route for the Regioselective Deacetylation of Peracetylated Carbohydrates at Anomeric Position, Lett. Org. Chem., 2006, 3, 35–38 CrossRef CAS
.
-
(a) B. Wang, S. van Herck, Y. Chen, X. Bai, Z. Zhong, K. Keswarte, B. N. Lambrecht, N. N. Sanders, S. Lienenklaus, H. W. Scheeren, S. A. David, F. Kiessling, T. Lammers, B. G. de Geest and Y. Shi, Potent and Prolonged Innate Immune Activation by Enzyme-Responsive Imidazoquinoline TLR7/8 Agonist Prodrug Vesicles, J. Am. Chem. Soc., 2020, 142, 12133–12139 CrossRef CAS PubMed
;
(b) Compound 8 was originally
described by: G. N. Bollenback, J. W. Long, D. G. Benjamin and J. A. Lindquist, The Synthesis of Aryl-D-glucopyranosiduronic Acids, J. Am. Chem. Soc., 1955, 78, 3310–3315 CrossRef
.
- L. Kerins, S. Byrne, A. Gabba and P. V. Murphy, Anomeric Preferences for Glucuronic and Galacturonic Acid and Derivatives and Influence of Electron-Withdrawing Substituents, J. Org. Chem., 2018, 83, 7714–7729 CrossRef CAS PubMed
.
- D. S.-E. K. Teki, A. Bil, V. Moreau, V. Chagnault, B. Fante, A. Adjou and J. Kovensky, Synthesis of multivalent S-glycoside analogues of a heparan sulfate sequence, Org. Chem. Front., 2019, 6, 2718–2725 RSC
.
-
M. Eloranta, L. Hirvelae, L. Kangas, P. Koskimies, R. Lammintausta and M. Unkila, WO 2014207309, 2014
.
- Y. Chen, Y. Li, H. Yu, G. Sugiarto, V. Thon, J. Hwang, L. Ding, L. Hie and X. Chen, Tailored Design and Synthesis of Heparan Sulfate Oligosaccharide Analogues Using Sequential One-Pot Multienzyme Systems, Angew. Chem., Int. Ed., 2013, 52, 11852–11856 CrossRef CAS PubMed
.
-
(a) R. Nakajima, M. Ono, S. Aiso and H. Akita, Synthesis of Methyl 1-O-(4-hydroxymethamphetaminyl)-α-D-glucopyranouronate, Chem. Pharm. Bull., 2005, 53, 684–687 CrossRef CAS PubMed
;
(b) J. A. Hayes, K. S. Eccles, S. E. Lawrence and H. E. Moynihan, Preparation and characterisation of solid state forms of paracetamol-O-glucuronide, Carbohydr. Res., 2012, 349, 108–112 CrossRef CAS PubMed
;
(c) M. M. Cavalluzzi, G. Lentini, A. Lovece, C. Bruno, A. Catalano, A. Carocci and C. Franchini, First synthesis and full characterisation of mexiletene N-carbonyloxy β-D-glucuronide, Tetrahedron Lett., 2010, 51, 5265–5268 CrossRef CAS
.
- N-Me, piperazine pKas: 9.14, 4.73. F. Khalili, A. Henni and A. L. L. East, pKa Values of Some Piperazines at (298, 303, 313, and 323) K, J. Chem. Eng. Data, 1990, 35, 276–277 CrossRef
.
-
(a) R. T. Brown, F. Scheinmann and A. V. Stachulski, Intermediates for Glucuronide Synthesis: 7-Hydroxycoumarin Glucuronide, J. Chem. Res., Synop., 1997, 370–371 RSC
;
(b) B. Fischer, A. Nudelman, M. Ruse, J. Herzig, H. E. Gottlieb and E. Keinan, A Novel Method for Stereoselective Glucuronidation, J. Org. Chem., 1984, 49, 4988–4993 CrossRef CAS
.
- O. Kirk and M. W. Christensen, Lipases from Candida antarctica: Unique biocatalysts from a unique origin, Org. Process Res. Dev., 2002, 6, 446–451 CrossRef CAS
.
- The D2O solution of 5 was evaporated, then co-evaporated quickly with two small portions of H2O to exchange any O-deuterio species, for mass spectrometric analysis. This product showed [M + 3]+ as required.
Footnotes |
† Electronic supplementary information (ESI) available: Details of preparation and characterisation of all compounds described, including photocopy 1H and 13C NMR spectra; detailed assignment and 1H/13C correlation of the E/Z isomers of 4. See DOI: https://doi.org/10.1039/d3qo01736b |
‡ Current address: GSK Medicines Research Centre, Gunnels Wood Road, Stevenage, SG1 2NY, UK. |
§ Current address: School of Chemistry, Cardiff University, Main Building, Park Place, Cardiff, CF10 3AT, UK. |
|
This journal is © the Partner Organisations 2024 |
Click here to see how this site uses Cookies. View our privacy policy here.