DOI:
10.1039/D3QO01588B
(Research Article)
Org. Chem. Front., 2024,
11, 171-175
Metal-free hydrophosphorodithiolation of alkynes with P4S10 and alcohols leading to vinyl phosphorodithioates†
Received
27th September 2023
, Accepted 14th November 2023
First published on 23rd November 2023
Abstract
A simple and metal-free strategy has been developed for the construction of vinyl phosphorodithioates via direct hydrophosphorodithiolation of alkynes with P4S10 and alcohols. This three-component reaction could be conducted under mild conditions to access a variety of vinyl phosphorodithioates with Z-isomers as the major products without the use of any metal reagents, ligands, or additives. Preliminary mechanistic studies suggested that a radical process was involved in this reaction procedure.
Introduction
Phosphorodithioates are frequently found in a broad range of agrochemicals, functional materials, and pharmaceuticals.1–3 In particular, vinyl phosphorodithioates have attracted great attention from chemists due to their exceptional insecticidal activity and relatively low mammalian toxicity.4 Consequently, a number of methods have been developed to construct vinyl phosphorodithioates.5–9 The traditional method for the synthesis of vinyl phosphorodithioates involves the reactions of Grignard reagents or vinyl lithium reagents with disulfides of O,O-dialkyl phosphorodithioic acids (Scheme 1a).5 This strategy would produce stoichiometric quantities of metal waste accompanied by the use of excessive amounts of metal reagents and suffers from poor functional group compatibility. During the past several years, some alternative methods have been explored,6–8 for example, the nucleophilic substitution of specific chloro carbonyl compounds with potassium O,O-dialkyl phosphorodithioate (Scheme 1b)7 and copper-catalyzed phosphorothiolation of alkenyliodonium salts with potassium O,O-dialkyl phosphorodithioates (Scheme 1c).8 In 2021, Wu and co-workers reported an efficient copper-catalyzed thioamination of maleimides with diethylphosphorodithioate and amines to construct a series of β-amino vinyl phosphorodithioates (Scheme 1d).9 Despite some obvious advances in these methods, the utilization of metal catalysts and not easily available starting materials still stimulated chemists to explore a simple, efficient, and especially metal-free strategy to construct vinyl phosphorodithioates.
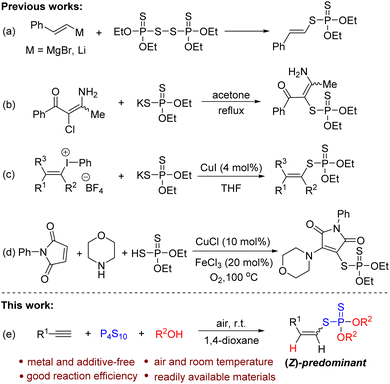 |
| Scheme 1 The synthetic strategies for the construction of vinyl phosphorodithioates. | |
Alkynes are easily available and versatile building blocks that have been widely utilized in various synthetic transformations.10 From the atom-economy and synthetic efficiency points of view, the direct functionalization of alkynes is one of the most attractive strategies to access vinyl compounds.11 On the other hand, as a simple and commercially available thionation reagent, P4S10 has attracted great interest from chemists for the construction of sulfur-containing compounds in synthetic chemistry.12 Very recently, Zhou and Lu described an elegant method for the synthesis of allyl dialkylphosphorodithioates through ring-opening of spirovinylcyclopropyl oxindoles with P4S10 and alcohols.13 With our continued interest in the development of new reactions to access sulfur-containing compounds,14 herein, we wish to present a simple and efficient multi-component reaction strategy for the construction of vinyl phosphorodithioates via metal-free hydrophosphorodithiolation of alkynes with P4S10 and alcohols (Scheme 1e). The present reaction could be carried out at room temperature to afford a series of vinyl phosphorodithioates with Z-isomers as the major products without requiring any metal reagents, ligands, or additives.
Results and discussion
Initially, phenylacetylene (1a), P4S10 (2), and EtOH (3a) were chosen as model substrates to test the optimized reaction conditions in our investigation. When oxidants such as TBHP and Na2S2O8 were used in this model reaction system at room temperature, the corresponding vinyl phosphorodithioate 4a (Z-isomer predominant) could be obtained in 84% and 81% yields, respectively (Table 1, entries 1 and 2). The addition of metal catalysts such as CuCl and FeCl3 would completely inhibit this hydrophosphorodithiolation of alkyne and the desired product 4a was not detected (Table 1, entries 3 and 4). Both reactions only generated a large amount of disulfide of O,O-diethyl phosphorodithioic acid. Only a trace amount of the product was observed when molecular iodine was added to this reaction system (Table 1, entry 5). To our delight, the model reaction could give the product 4a in 84% yield under air in the absence of any additives (Table 1, entry 6). The mixed solvent of EtOH with other organic solvents was investigated to improve the reaction efficiency. The product 4a was generally obtained in good yields when EtOH/DCE, EtOH/EtOAc, EtOH/toluene, EtOH/DME, EtOH/CH3CN, and EtOH/1,4-dioxane were used as the reaction medium (Table 1, entries 7–12). No transformation was observed when DMSO or DMF was used as the co-solvent (Table 1, entries 13 and 14). Further optimization showed that the yield of 4a could be increased to 88% when the mixed solvent of EtOH/1,4-dioxane (1/3) was employed (Table 1, entry 16). Finally, the increase of reaction temperature did not promote this reaction, and thus room temperature is still the best choice (Table 1, entry 18).
Table 1 Optimization of reaction conditionsa

|
Entry |
Additive |
Solvent |
4a yield (%) (Z/E)b |
Reaction conditions: 1a (0.2 mmol), 2 (0.2 mmol), 3a (0.2–2 mL), additive (10–100 mol%), solvent (2 mL), air, r.t., 6 h.
Isolated yields based on 1a; Z/E ratios in parentheses were determined by 1H NMR.
60 °C.
|
1 |
TBHP (100 mol%) |
EtOH |
84% (77 : 23) |
2 |
Na2S2O8 (100 mol%) |
EtOH |
81% (72 : 28) |
3 |
CuCl2 (10 mol%) |
EtOH |
0 |
4 |
FeCl3 (10 mol%) |
EtOH |
0 |
5 |
I2 (10 mol%) |
EtOH |
Trace |
6 |
— |
EtOH |
84% (80 : 20) |
7 |
— |
EtOH/DCE (1/4) |
69% (72 : 28) |
8 |
— |
EtOH/EtOAc (1/4) |
70% (74 : 26) |
9 |
— |
EtOH/toluene (1/4) |
81% (77 : 23) |
10 |
— |
EtOH/DME (1/4) |
73% (71 : 29) |
11 |
— |
EtOH/CH3CN (1/4) |
70% (77 : 23) |
12 |
— |
EtOH/1,4-dioxane (1/4) |
85% (81 : 19) |
13 |
— |
EtOH/DMSO (1/4) |
0 |
14 |
— |
EtOH/DMF (1/4) |
0 |
15 |
— |
EtOH/1,4-dioxane (1/1) |
74% (80 : 20) |
16
|
—
|
EtOH/1,4-dioxane (1/3)
|
88% (83 : 17)
|
17 |
— |
EtOH/1,4-dioxane (1/10) |
78% (80 : 20) |
18 |
— |
EtOH/1,4-dioxane (1/3) |
73%(76 : 24)c |
With the standard reaction conditions in hand, we moved on to investigate the scope of this hydrophosphorodithiolation of alkynes with P4S10 and alcohols (Table 2). Generally, this reaction covers a range of alkynes with various substituted groups including both electron-donating and electron-withdrawing groups at the aromatic ring, and the desired products 4a–4n (Z-isomer predominant) could be efficiently obtained in moderate to good yields. It was found that the steric hindrance has no obvious effect on the reaction efficiency. More sterically demanding aromatic alkynes such as 2-chlorophenylacetylene and 2-fluorophenylacetylene were well compatible with this reaction process to afford the desired products 4d and 4i in good yields. Notably, functional groups such as fluoro, chloro, bromo, acetyl, and nitro groups were tolerated in this procedure and thus amenable to further structural modification. 1,3-Diethynylbenzene could also be used in this reaction system to afford the dihydrophosphorodithiolation product 4o in 48% yield. The reactions of 1-ethynylnaphthalene and heterocyclic alkynes such as 2-ethynylthiophene and 3-ethynylthiophene proceeded well to yield the corresponding products 4p–4r in good yields. Additionally, ethyl propiolate was also a suitable substrate to give the corresponding product 4s in 98% yield. Unfortunately, when aliphatic alkynes such as hex-1-yne and but-3-ynylbenzene were investigated in the present reaction system, the desired product was not detected. Finally, the scope of alcohols was tested under the optimized conditions. Both chain alcohols such as methanol, n-butanol, and iso-propanol and cyclic alcohols such as cyclohexanol could all react smoothly to provide the desired products 4t–4w in moderate to good yields. Phenylmethanol was also tolerated in this procedure to afford the product 4x in 75% yield.
Table 2 Substrate scopea,b
Reaction conditions: 1 (0.2 mmol), 2 (0.2 mmol), 3 (0.5 mL), 1,4-dioxane (1.5 mL), air, r.t., 6 h.
Isolated yields based on 1; Z/E ratios in parentheses were determined by 1H NMR.
|
|
Furthermore, to demonstrate the synthetic feasibility of this method, a gram-scale reaction was investigated. When the model reaction of phenylacetylene, P4S10, and EtOH was carried out on a 5 mmol scale, the corresponding product 4a was still obtained in 84% yield (1.21 g). This result showed that the present reaction could be utilized as an efficient and practical protocol to construct vinyl phosphorodithioates.
To probe the possible reaction mechanism, several control experiments were conducted and the results are shown in Scheme 2. First, when the model reaction was conducted in the absence of phenylacetylene 1a, O,O-diethyl S-hydrogen phosphorodithioate 5a was isolated in 86% yield (Scheme 2a). Furthermore, the desired product 4a could be obtained in 85% yield when phenylacetylene 1a reacted separately with O,O-diethyl S-hydrogen phosphorodithioate 5a under standard conditions, indicating that 5a might be a key intermediate in this reaction system (Scheme 2b). Subsequently, the model reaction was completely suppressed when TEMPO was added in the model reaction system (Scheme 2c). Furthermore, when the reaction of phenylacetylene (1a) with O,O-diethyl S-hydrogen phosphorodithioate 5a was carried out in the presence of TEMPO, the corresponding disulfide of O,O-diethyl phosphorodithioate (5a′) was obtained in 58% yield and product 4a was not detected (Scheme 2d). This result indicated that the addition of S-hydrogen phosphorodithioate 5a to phenylacetylene 1a might involve a radical pathway. Next, no transformation was observed when the model reaction or the reaction of phenylacetylene 1a with intermediate 5a was conducted under N2, which suggested that dioxygen in air was essential for promoting this hydrophosphorodithiolation of alkynes (Scheme 2e and f). Moreover, when EtOD was used as the substrate and solvent, the deuterated-product D-4a was readily obtained in 84% yield with 90% D incorporation (Scheme 2g). This result indicated that the vinyl hydrogen atom adjacent to the benzene ring of the product came from EtOH.
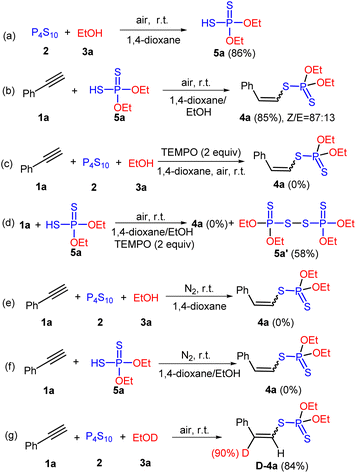 |
| Scheme 2 Control experiments. | |
Based on the above experiments and related reports,12,15,16 we proposed a possible reaction mechanism as described in Scheme 3. First, the interaction of P4S10 with EtOH formed the dialkylphosphorodithioate 5. Subsequently, the oxidation of dialkylphosphorodithioate 5 by air (O2) provided thiyl radical 6. Then, the addition of radical 6 to alkyne 1 gave alkenyl radical 7, which abstracted a hydrogen atom from 5 to form the product 4 with the release of radical intermediate 6. The formation of Z-predominant vinyl phosphorodithioate might be caused by the fact that the abstraction of a hydrogen atom from 5 by radical intermediate 7 (A) is more sterically favored than that by radical intermediate 7 (B).16
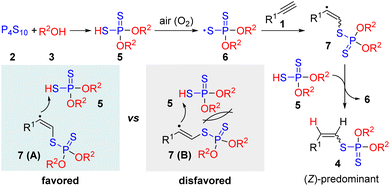 |
| Scheme 3 Possible reaction mechanism. | |
Conclusions
In summary, we have developed a facile and metal-free hydrophosphorodithiolation of alkynes with P4S10 and alcohols leading to vinyl phosphorodithioates under mild conditions. A variety of alkynes and alcohols were capable of the conversion to afford the corresponding vinyl phosphorodithioates with Z-isomers as major products in good yields. This chemistry highlights the advantages of simple operation, metal-free conditions, and good functional group tolerance. The present reaction matches the idea of green chemistry and is expected to spur more interest in developing new P4S10-participating reactions in synthetic chemistry.
Conflicts of interest
There are no conflicts to declare.
Acknowledgements
This work was supported by the Program of Science and Technology International Cooperation Project of Qinghai Province (2022-HZ-813) and the National Natural Science Foundation of China (22101237).
References
-
(a) F. A. F. Ali, A. C. Chukwudebe and T. R. Fukuto, Toxicological Properties of O,S,S-trialkyl phosphorodithioates, J. Agric. Food Chem., 1986, 34, 45–48 CrossRef CAS;
(b) W. S. Marshall and M. H. Caruthers, Phosphorodithioate DNA as A Potential Therapeutic Drug, Science, 1993, 259, 1564–1570 CrossRef CAS PubMed;
(c) W. T. Wiesler and M. H. Caruthers, Synthesis of Phosphorodithioate DNA via Sulfur-Linked, Base-Labile Protecting Groups, J. Org. Chem., 1996, 61, 4272–4281 CrossRef CAS PubMed;
(d) W. Dbkowski, I. Tworowska, W. Dbkowski and M. Michalska, Highly Efficient Synthesis of Phosphorodithioates Derived from 3-Thiothymidine by Anhydro-ring Opening of 2,3-Anhydro-5-O-tritylthymidine with O,O-disubstituted Phosphorodithioic Acids, Chem. Commun., 1998, 427–428 RSC;
(e) O. J. Plante and P. H. Seeberger, Anomeric Phosphorodithioates as Novel Glycosylating Agents, J. Org. Chem., 1998, 63, 9150–9151 CrossRef CAS;
(f) T. S. Kumar, T. Yang, S. Mishra, C. Cronin, S. Chakraborty, J.-B. Shen, B. T. Liang and K. A. Jacobson, 5-Phosphate and 5-Phosphonate Ester Derivatives of (N)-Methanocarba Adenosine with in Vivo Cardioprotective Activity, J. Med. Chem., 2013, 56, 902–914 CrossRef CAS PubMed.
-
(a) T. B. Gaines, Acute toxicity of Pesticides, Toxicol. Appl. Pharmacol., 1969, 14, 515–534 CrossRef CAS PubMed;
(b) R. E. Menzer, Z. M. Iqbal and G. R. Boyd, Metabolism of O-ethyl S,S-dipropyl phosphorodithioate (Mocap) in Bean and Corn Plants, J. Agric. Food Chem., 1971, 19, 351–356 CrossRef CAS PubMed;
(c) D. L. Bull, C. J. Whitten and G. W. Ivie, Fate of O-ethyl O-[4-(methylthio)phenyl] S-propyl Phosphorodithioate (BAY NTN 9306) in Cotton Plants and Soil, J. Agric. Food Chem., 1976, 24, 601–605 CrossRef CAS PubMed;
(d) Y. Z. Piao, Y. J. Kim, Y. A. Kim, H.-S. Lee, B. D. Hammock and Y. T. Lee, Development of ELISAs for the Class-Specific Determination of Organophosphorus Pesticides, J. Agric. Food Chem., 2009, 57, 10004–10013 CrossRef CAS PubMed.
-
(a) O. BrÜmmer, P. Wentworth Jr., D. R. Weiner and K. D. Janda, Phosphorodithioates Synthesis and Evaluation of New Haptens for the Generation of Antibody Acyl Transferases, Tetrahedron Lett., 1999, 40, 7307–7310 CrossRef;
(b) C.-M. Park, Y. Zhao, Z. Zhu, A. Pacheco, B. Peng, N. O. Devarie-Baez, P. Bagdon, H. Zhang and M. Xian, Synthesis and Evaluation of Phosphorodithioate-Based Hydrogen Sulfide Donors, Mol. BioSyst., 2013, 9, 2430–2434 RSC.
-
(a) G. A. Johnson, J. H. Fletcher, K. G. Nolan and J. T. Cassaday, Decreased Toxicity and Cholinesterase Inhibition in a New Series of Dithiophosphates, J. Econ. Enfomol., 1952, 45, 279 CrossRef CAS;
(b)
D. E. H. Frear, Chemistry of Pesticides, Van Nostrand Co., New York, 3rd edn, 1955, pp. 86–89 Search PubMed;
(c) W. H. Earned and J. E. Casida, Dioxathion Metabolites, Photoproducts, and Oxidative Degradation Products, J. Agric. Food Chem., 1976, 24, 689–699 CrossRef PubMed.
-
(a) B. Miller, A new synthesis of phosphorodithioate esters, J. Am. Chem. Soc., 1960, 82, 6205 CrossRef CAS;
(b) B. Miller, Reactions of phosphorothionyl disulphides with organometallic reagents, Tetrahedron, 1964, 20, 2069–2078 CrossRef CAS.
-
(a) V. Dodin-Carnot, B. Stephan, M. Curci, J. L. Mieloszynski and D. Paquer, Reactivity of dithiophosphoric acid towards sulfurated monosubstituted alkynes in presence of transition-metal-catalyst, Phosphorus, Sulfur Silicon Relat. Elem., 1996, 117, 225–230 CrossRef CAS;
(b) V. Dodin-Camot, M. Curci, J. C. Wilhelm, J. L. Mieloszynski and D. Paquer, Reactivite del’ acide surdes alcynes vrais sulfures o,o’-diisopropyldithiophosphoriq, Phosphorus, Sulfur Silicon Relat. Elem., 1995, 107, 219–226 CrossRef.
-
(a) V. A. Kozlov, S. G. Churusova, S. V. Yarovenko, O. A. Kononova, V. V. Negrebetskii and A. F. Grapov, Organo-phosphorus compounds, isocyclic O,N,S derivatives, Zh. Obshch. Khim., 1994, 64, 1439 CAS.
-
(a) J. Yan and Z.-C. Chen, Hypervalent Iodine in synthesis: An Efficient Route for The Synthesis of S-Vinyl O, O-Dialkylphosphorodithioates, Synth. Commun., 1999, 29, 3275–3282 CrossRef CAS;
(b) P. Zhang and Z.-C. Chen, Hypervalent Iodine in Synthesis: Synthesis and Reactivity of New Functionalised Alkenyliodonium Salts, J. Chem. Res., 2003, 570–571 CrossRef CAS.
- Y. Xu, J. Gao, C. Wang, Y. Ma, J. Zhou and G. Wu, Copper-catalyzed thioamination of maleimides with diethylphosphorodithioate and amines, Org. Chem. Front., 2021, 8, 3457–3462 RSC.
-
(a) G. Fang and X. Bi, Silver-catalysed reactions of alkynes: recent advances, Chem. Soc. Rev., 2015, 44, 8124–8173 RSC;
(b) Q. Liu, Y. Lv, R. Liu, X. Zhao, J. Wang and W. Wei, Catalyst- and additive-free selective sulfonylation/cyclization of 1,6- enynes with arylazo sulfones leading to sulfonylated g-butyrolactams, Chin. Chem. Lett., 2021, 32, 136–139 CrossRef CAS;
(c) W.-L. Lei, B. Yang, Q.-B. Zhang, P.-F. Yuan, L.-Z. Wu and Q. Liu, Visible-light-enabled aerobic synthesis of benzoin bis-ethers from alkynes and alcohols, Green Chem., 2018, 20, 5479–5483 RSC;
(d) D. Yang, Q. Yan, E. Zhu, J. Lv and W.-M. He, Carbon-sulfur bond formation via photochemical strategies: An efficient method for the synthesis of sulfur-containing compounds, Chin. Chem. Lett., 2022, 33, 1798–1816 CrossRef CAS.
-
(a) F. Zhao, X. Jia, Pi. Li, J. Zhao, Y. Zhou, J. Wang and H. Liu, Catalytic and catalyst-free diboration of alkynes, Org. Chem. Front., 2017, 4, 2235–2255 RSC;
(b) G. Wu, U. Chakraborty and A. Wangelin, Regiocontrol in the cobalt-catalyzed hydrosilylation of alkynes, Chem. Commun., 2018, 54, 12322–12325 RSC;
(c) S. Ghosh, D. Lai and A. Hajra, Trifunctionalization of alkenes and alkynes, Org. Biomol. Chem., 2020, 18, 7948–7976 RSC;
(d) S. Mandal, P. K. Verma and K. Geetharani, Lewis acid catalysis: regioselective hydroboration of alkynes and alkenes promoted by scandium triflate, Chem. Commun., 2018, 54, 13690–13693 RSC;
(e) J. Jiang, Z. Wang and W.-M. He, Electrosynthesis of 1-indanones, Chin. Chem. Lett., 2021, 32, 1591–1592 CrossRef CAS;
(f) A. N. V. Satyanarayana, N. Mukherjee and T. Chatterjee, 100% atom-economical and highly regio- and stereoselective iodosulfenylation of alkynes: a reagentless and sustainable approach to access (E)-β-iodoalkenyl sulfides and (Z)-tamoxifen, Green Chem., 2023, 25, 779–788 RSC.
-
(a) T. Ozturk, E. Ertas and O. Mert, A Berzelius Reagent, Phosphorus Decasulfide (P4S10), in Organic Syntheses, Chem. Rev., 2010, 110, 3419–3478 CrossRef CAS PubMed;
(b) B. Kaboudin, V. Yarahmadi, J.-Y. Kato and T. Yokomatsu, A simple and novel method for the direct conversion of carboxylic acids into thioamides, RSC Adv., 2013, 3, 6435–6441 RSC;
(c) S. K. Bur, 1,3-Sulfur Shifts: Mechanism and Synthetic Utility, Top. Curr. Chem., 2007, 274, 125–171 CrossRef CAS;
(d) A. Sudalai, S. Kanagasabapathy and B. C. Benicewicz, Phosphorus Pentasulfide: A Mild and Versatile Catalyst/Reagent for the Preparation of Dithiocarboxylic Esters, Org. Lett., 2000, 2, 3213–3216 CrossRef CAS PubMed;
(e) C. K. Maurya, A. Mazumder and P. K. Gupta, Phosphorus pentasulfide mediated conversion of organic thiocyanates to thiols, Beilstein J. Org. Chem., 2017, 13, 1184–1188 CrossRef CAS PubMed.
- J. Wang, F. Han, S. Hao, Y.-J. Tang, C. Xiong, L. Xiong, X. Li, J. Lu and Q. Zhou, Metal-Free Regioselective Hydrophosphorodithioation of Spirovinylcyclopropyl Oxindoles: Rapid Access to Allyl Dialkylphosphorodithioates, J. Org. Chem., 2022, 87, 12844–12853 CrossRef CAS PubMed.
-
(a) Q. Liu, L. Wang, H. Yue, J.-S. Li, Z. Luo and W. Wei, Catalyst-free visible-light-initiated oxidative coupling of aryldiazo sulfones with thiols leading to unsymmetrical sulfoxides in air, Green Chem., 2019, 21, 1609–1613 RSC;
(b) Z. Wang, Q. Liu, R. Liu, Z. Ji, Y. Li, X. Zhao and W. Wei, Visible-light-initiated 4CzIPN catalyzed multi-component tandem reactions to assemble sulfonated quinoxalin-2(1H)-ones, Chin. Chem. Lett., 2022, 33, 1479–1482 CrossRef CAS;
(c) Y. Lv, H. Cui, N. Meng, H. Yue and W. Wei, Recent advances in the application of sulfinic acids for the construction of sulfur-containing compounds, Chin. Chem. Lett., 2022, 33, 97–114 CrossRef CAS;
(d) D. Yi, L. He, Z. Qi, Z. Zhang, M. Li, J. Lu, J. Wei, X. Du, Q. Fu and S. Wei, Copper-Catalyzed Aerobic Oxidative Cleavage of Unstrained Carbon-Carbon Bonds of 1,1-Disubstituted Alkenes with Sulfonyl Hydrazides, Chin. J. Chem., 2021, 39, 859–865 CrossRef CAS;
(e) Z. Wang, N. Meng, Y. Lv, W. Wei, H. Yue and G. Zhong, Photocatalyst-free visible-light-me diate d three-component reaction of α-diazoesters, cyclic ethers and NaSCN to access organic thiocyanates, Chin. Chem. Lett., 2023, 34, 107599 CrossRef CAS.
- X. Zhou, R. Li and Z. Sun, Green Synthesis of α-Keton Thiol Ester via Photocatalytic Oxidative Radical Addition of Thioic Acid to Alkene, Org. Lett., 2023, 25, 4173–4176 CrossRef CAS PubMed.
- W. Li and M. Shi, Addition of diphenylphosphinodithioic acid and thioacetic acid with vinylidenecyclopropanes: reversed regioselectivities, Tetrahedron, 2009, 65, 6815–6821 CrossRef CAS.
|
This journal is © the Partner Organisations 2024 |