DOI:
10.1039/D3QO01109G
(Research Article)
Org. Chem. Front., 2024,
11, 12-20
A simple method for N-arylation of secondary amides/amines through a NaH-initiated aryne generation strategy†
Received
19th July 2023
, Accepted 2nd October 2023
First published on 3rd October 2023
Abstract
A very simple and practical method has been uncovered for N-arylation of many sets of secondary amides/amines. The reaction could be easily conducted in a vial without the use of strictly anhydrous reagents and/or an inert atmosphere. This transition metal-free coupling reaction was based on the use of our previously reported novel aryne generation system: a combination of o-diiodoarene and sodium hydride. In our protocol, sodium hydride acts as an iodophile by forming a neighboring-group-assisted transition state with o-diiodoarene, which greatly enhances the metal–halogen exchange process. o-Diiodoarene has two roles in the reaction: (i) as an aryne precursor to produce an aryne at near room temperature and (ii) as an electrophilic iodine donor to support the generation of the final o-iodoaryl product. The generality of the method is demonstrated by 99 examples containing almost all kinds of amides and amines.
Introduction
The formation of the C(aryl)–N bond is of great importance because the resulting arylamides/arylamines are ubiquitous in natural products, pharmaceuticals, agrochemicals and materials and also widely used as building blocks in organic synthesis.1 Since the discovery of the Ullmann–Goldberg reaction2 for assembling arylamines at high temperatures, many TM (transition metal)-catalyzed strategies have been developed to avoid the use of harsh reaction conditions and to achieve a broad substrate scope, represented by the Buchwald–Hartwig reaction,3 the Chan–Lam coupling,4 the ligand-accelerated Ullmann reaction,5 and nickel-catalyzed amination.6 On the other hand, TM-free methods have flourished recently due to the advantages of milder reaction conditions, no ligand requirement, and no transition metal residue in products. These methods include SNAr reactions of fluoroarenes,7 the use of diaryliodonium salts as substrates for direct N-arylation,8 electrochemical9 and photocatalyzed C–H amidation,10 and other kinds of TM-free protocols.11 Despite several benefits of current reports, the development of new protocols is still highly desirable from the viewpoints of generality, simple operation, cost-efficiency and sustainable development.
Aryne chemistry has proven to be a very efficient tool for rapid build-up of aromatic structures.12 Among them, the representative reactions are those of nitrogen nucleophiles with arynes. A lot of examples of normal N-arylation of various N-nucleophiles have been documented in the literature (Scheme 1a, left).13 On the other hand, for some secondary amides, aryne insertion into an amide bond to produce the rearrangement products can also occur (Scheme 1a, right).14 However, the conversion of challenging secondary acyclic amides8a,15 into o-iodoaryl tertiary amides is still a formidable and fascinating task in aryne chemistry.
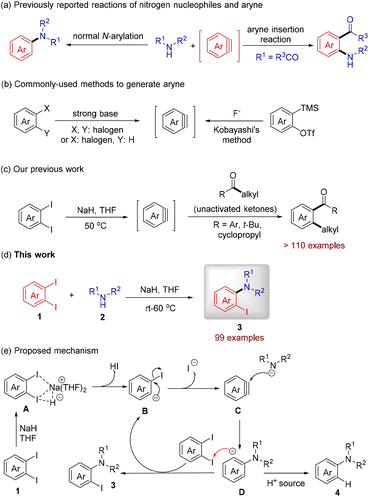 |
| Scheme 1 Methods to generate arynes and reactions with amides/amines. | |
The aryne generation process is the central topic of aryne chemistry. Traditionally, cheap and readily available haloarenes or o-dihaloarenes have been employed as aryne precursors (Scheme 1b, left),12a–c but the use of strong bases such as NaNH2, LDA, LiTMP or BuLi in these methods reduces the functional group compatibility. Alternatively, Kobayashi's o-silylaryl triflate/fluoride salt protocol could generate arynes in a mild and controlled manner and thus was widely applied in organic synthesis (Scheme 1b, right).12d However, the synthetic route to obtain o-silylaryl triflate is tedious,16 which restricts its practical application, particularly in an industrial setting.
In the exploration of new reactivities of sodium hydride by Chiba17 and our groups,18 very recently, we have shown that due to the low solubility and specific activity of this reagent, the treatment of o-diiodoarenes with NaH in THF could lead to the controlled generation of aryne species. The active aryne intermediate then participated in the C–C σ-bond insertion reaction of unactivated ketones (Scheme 1c),18f a kind of transformation that has never been achieved previously. DFT calculations have identified an unprecedented neighboring-group-assisted metal-halogen exchange transition state, in which the complexation network A has a low activation free energy (8.1 kcal mol−1) (Scheme 1e) that facilitates the formation of an aryne at near room temperature.18f The new activation mode has been transformed into a unique power for developing new reactions. It was found that compared with the established aryne chemistry, our protocol frequently gave rise to structurally novel products. Herein, to demonstrate the robustness of the o-diiodoarene/NaH combination, we disclose the N-arylation reactions for several sets of secondary amides/amines. The unexpected o-iodoaryl products are synthetically very useful materials19 but not easy to prepare by known methods20 (Scheme 1d).
We suggested that the o-iodoaryl structure 3 could be assembled by quenching anion D with the electrophilic iodine of another o-diiodoarene (Scheme 1e), a mechanism similar to that described by Schlosser,21a Leroux,21b–e and Hamura and Suzuki21fet al. This aryne-involved reaction mechanism implies that the remaining iodine in product 3 will not further react with other nucleophiles, which is in sharp contrast to that observed in TM-catalyzed coupling reactions.22 Notably, other documented methods to synthesize o-iodoaryl compounds usually require additional stoichiometric amounts of the iodine reagent.23 Finally, it should be noted that we also observed a small amount of the iodine-free byproduct 4 in some cases, and this might be produced from anion D by abstracting a proton from the surroundings (Scheme 1e).
Results and discussion
Our study began by evaluating the model reaction of the secondary acyclic amide 1a with o-diiodobenzene 2a (Table 1). After extensive experiments, it was found that the treatment of 2a (2.5 equiv.) with NaH (2.5 equiv.) in THF (0.25 M) at 30 °C could efficiently generate benzyne, which then led to N-arylation of 1a (0.6 mmol) and afforded o-iodoaryl product 3a in 90% yield (entry 1). It should be noted that the iodine-free product 4a was not observed under the reaction conditions. Significantly, the reaction could be easily conducted in a vial (5 mL) without the use of strictly anhydrous reagents and/or an inert atmosphere. The very simple operation constitutes a great advantage of our approach over conventional protocols. In the exploration of a base, NaH was identified as the optimal one for the process, and other commonly used hydrides, whether it was the more active KH or the less active LiH and CaH2, proved to be inefficient for the transformation (entries 2 and 3). Next, several benzyne precursors were tested (entries 4–7). Consistent with our previous research and DFT calculations,18f the iodine atom in o-dihalobenzenes was identified to be key to the success of the reaction. Therefore, precursors 2b–2d could deliver the desired product (entries 4–6), while o-dibromobenzene 2e failed to react with NaH and remained intact even after a long reaction time (entry 7). Remarkably, no bromide 3a′ or chloride 3a′′ was observed when 2b or 2c was used as the precursor, which could support the proposed mechanism (Scheme 1e). In the optimization of the solvent, DMA and DME also performed well, giving 3a in good yields (entries 8 and 9). Subsequently, the loadings of NaH and o-diiodobenzene were evaluated. Reducing the dosage of NaH from 2.5 equiv. to 2.0 equiv. slightly reduced the yield to 88% (entry 10). On the other hand, increasing NaH to 3.0 equiv. led to the appearance of a very small amount of 4a (entry 11). An effort to save 2a resulted in a decrease in the reaction yield as well as the generation of 4a (entry 12), proving, to some degree, the role of o-diiodoarene as an electrophilic iodine donor in the formation of the o-iodoaryl product 3 (Scheme 1e). We have noticed that to trigger the reaction, the suitable temperature range is from 30 °C to 50 °C, which is crucial for the generation of the aryne. As evidence, similar results were obtained when performing the reaction at 30 °C and 50 °C (entry 1 and entry 13), whereas no reaction occurred at 0 °C even after 24 h (entry 14). Finally, a gram-scale synthesis was conducted (entry 15) under similar reaction conditions to entry 1 at 40 °C instead of 30 °C. To our delight, a higher yield was obtained (92%).
Table 1 Optimization of the reaction conditionsa
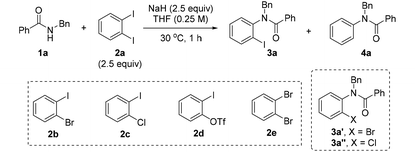
|
Entry |
Deviation from the standard conditions |
Yield of 3a b (%) |
Yield of 4a b (%) |
Reaction conditions for entry 1: To NaH (60% in oil, 60 mg, 1.5 mmol, 2.5 equiv.) in a vial was added a solution of amide 1a (0.6 mmol, 1.0 equiv.) in THF (2.0 mL). After stirring at room temperature for 3 min, 2a (1.5 mmol, 2.5 equiv.) in THF (0.4 mL) was added. The mixture was warmed to 30 °C and stirred for 1 h.
Isolated yield.
|
1 |
None |
90 |
0 |
2 |
KH instead of NaH |
11 |
2 |
3 |
LiH or CaH2, 50 °C instead of NaH, 30 °C |
<5 |
0 |
4 |
2b (3.0 equiv.), 15 h instead of 2a (2.5 equiv.), 1 h |
74 |
0 |
5 |
2c (3.0 equiv.), 15 h instead of 2a (2.5 equiv.), 1 h |
8 |
4 |
6 |
2d (3.0 equiv.), 0.5 h instead of 2a (2.5 equiv.), 1 h |
67 |
7 |
7 |
2e (3.0 equiv.), 24 h instead of 2a (2.5 equiv.), 1 h |
0 |
0 |
8 |
DMA instead of THF |
70 |
8 |
9 |
DME instead of THF |
68 |
2 |
10 |
2.0 equiv. of NaH was used |
88 |
0 |
11 |
3.0 equiv. of NaH was used |
85 |
4 |
12 |
2.0 equiv. of 2a was used |
77 |
7 |
13 |
50 °C, 1 h instead of 30 °C, 1 h |
88 |
0 |
14 |
0 °C, 24 h instead of 30 °C, 1 h |
0 |
0 |
15 |
One-gram scale, 40 °C, 1 h |
92 |
0 |
With the optimized conditions in hand, we evaluated the generality of the reaction. As shown in Scheme 2a, first, a variety of substituted N-methylbenzamides 1b–1t were subjected to the standard reaction conditions. To our delight, both electron-donating groups (–Me, –vinyl, –OBn, –OMe, and –NMe2) and electron-withdrawing groups (–F, –Cl, –Br, –I, –OCF3, –CF3, and –NO2) at the para-, meta- or ortho-position of the benzamide were tolerated, affording 3b–3t in moderate to high yields (33–97%). In addition, N-methyl-2-naphthamide 1u and heteroaromatic N-ethyl-amides 1v–1x were all competent substrates for the reaction, successfully providing the target molecules 3u–x. Remarkably, all kinds of halogens (especially iodine) posed no problem for the process, thus leaving ample room for further functionalization of the products (3h–3j, 3m, 3p, 3q and 3s). The protocol was also applicable to sensitive acrylamide substrates, as exemplified by the smooth transformation of secondary amides 1y–1aa into the corresponding products 3y–3aa in good yields. It is noteworthy that no cyclized product was detected in these examples, which indicates that the radical pathway should be ruled out for the reaction. Next, sterically hindered α,α-disubstituted aliphatic amides 1ab–1ad were tested, and they worked equally efficiently to give 3ab–3ad in impressive yields. Significantly, the fluorine atom at the α-position of the amide in 3ac survived the alkaline environment caused by NaH.
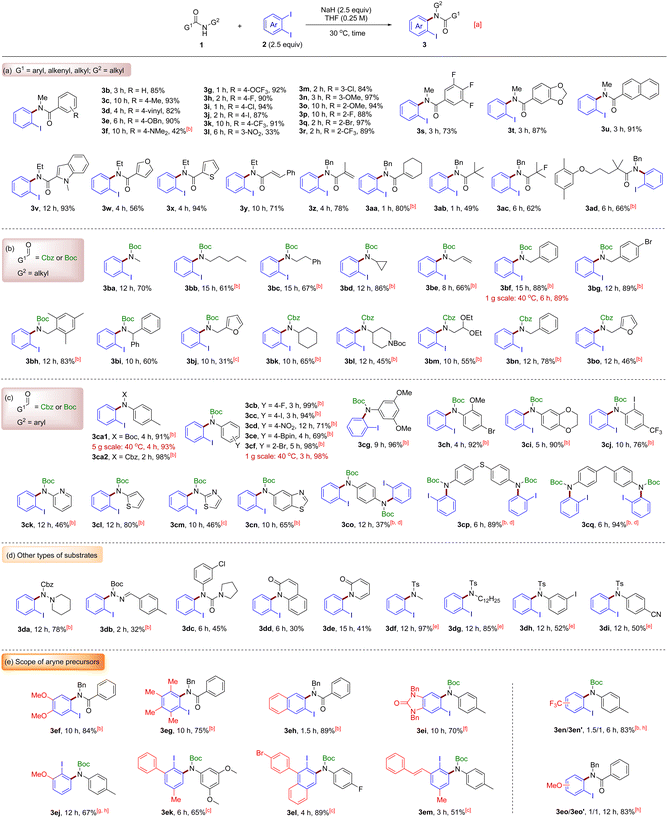 |
| Scheme 2 Substrate scope of amides and aryne precursors. a To NaH (60% in oil, 60 mg, 1.5 mmol, 2.5 equiv.) in a vial was added a solution of amides 1 (0.6 mmol, 1.0 equiv.) in THF (2.0 mL). After stirring at room temperature for 1–3 min, aryne precursor 2 (1.5 mmol, 2.5 equiv.) in THF (0.4 mL) was added. The mixture was warmed to 30 °C and stirred for the indicated time. b Performed at 40 °C. c Performed at 50 °C. d The dosage of 2 and NaH was doubled. e THF : DMA = 5 : 1 or 1 : 1 was used as the solvent. f Performed at 45 °C and 1.5 equiv. of 2i was used. g Performed at 60 °C. h Commercially available 1-bromo-2-iodo-substrates were used as the aryne precursors, see the ESI† for details. | |
Boc (t-butyloxycarbonyl) and Cbz (benzyloxycarbonyl) are both excellent protecting groups because they can be easily and selectively removed when required. Therefore, a series of Boc- or Cbz-protected primary alkyl amines were subsequently probed (Scheme 2b). Some representative cases, such as methylamine (the starting material to prepare substrate 1ba; the following directly abbreviated as 1bb–1bm), pentylamine (1bb), phenylethylamine (1bc), cyclopropylamine (1bd), allylamine (1be), benzylamines (1bf–1bi and 1bn), furan-2-ylmethanamine (1bj and 1bo), cyclohexylamine (1bk), 4-amino-1-Boc-piperidine (1bl) and 2,2-diethoxyethylamine (1bm), after being masked with Boc or Cbz, all acted as good nucleophiles in the reaction with benzyne. The coupling products 3ba–3bo could serve as valuable building blocks for constructing nitrogen-containing heterocycles and drugs. More importantly, deprotection is convenient for these compounds, thereby creating a practical means for the synthesis of diverse secondary aromatic amines.
To our delight, we found that the strategy could also be applied to Boc- or Cbz-protected primary arylamines 1ca–1cq, as listed in Scheme 2c. Generally, the N-arylation of these substrates proceeded well to furnish the protected diarylamines 3ca–3cq in good to excellent yields. Notably, the synthetically versatile –Bpin group is compatible with the reaction conditions, offering opportunities for extensive derivatization of compound 3ce. Apart from substituted anilines 1ca–1cj, the reactions of heteroaromatic amines 1ck–1cn took place smoothly as well to generate the desired products 3ca–3cn with high efficiency. Furthermore, substrates 1co–1cq possessing two reactive sites were double arylated without any problem, affording the interesting structures 3co–3cq that could potentially be used for the synthesis of functional materials.
Other successful N-nucleophiles include hydrazine derivatives (3da and 3db), urea (3dc), lactams (3dd and 3de) and even sulfonamides (3df–3di) (Scheme 2d). They demonstrate that the o-diiodobenzene/NaH system is universal for forging the C(aryl)–N bond, thereby providing a powerful tool for arylation of a wide range of secondary amides/sulfonamides.
The generality of the method was further illustrated by varying the aryne precursors (Scheme 2e). Although o-diiodobenzene 2a is commercially available and not expensive, other substituted o-diiodoarenes need to be prepared by ourselves. Fortunately, these o-diiodo compounds are easier to synthesize (see the ESI†) compared with Kobayashi's aryne precursors, which once again demonstrates the practical value of our method. For symmetrical precursors 2f–2i, the corresponding arynes were mildly generated under the standard conditions and then reacted with amides to afford the desired products 3ef–3ei. Of particular note is the imidazolinone moiety in 3ei. To our knowledge, this pharmaceutically interesting structure has been introduced into aryne precursors for the first time. In the case of 3-substituted unsymmetrical aryne precursors, whether the substituents are methoxy (2j), phenyl (2k and 2l) or styryl (2m) groups, the reactions proceeded smoothly and led to the formation of densely functionalized compounds 3ej–3em with exclusive regioselectivity control, with Boc-protected aniline moieties at the meta-position of the substituents. In contrast, the aryne precursors with trifluoromethyl or methoxy groups at the C4 position gave rise to 3en/3en′ or 3eo/3eo′ as a mixture of regioisomers.
Having evaluated more than 80 amide substrates, we then turned our attention to investigating the equally important secondary amines and N-heterocycles as reactants, aiming to establish a mild and concise route to access tertiary amines (Scheme 3). The reaction conditions were slightly modified by employing 2.0 equiv. of o-diiodoarenes and 3.0 equiv. of NaH in a mixture of solvents THF and DMA (4
:
1) at rt (see the ESI†). As shown, first, some typical aliphatic amines, including dibenzylamine (5a), diallylamine (5b) and 2-picolylmethylamine (5c), were smoothly converted into o-iodoaniline derivatives 6a–6c. Then, two aromatic amines, N-methylaniline (5d) and diphenylamine (5e), also participated in the reaction well to provide the desired compounds 6d (57% yield) and 6e (72% yield). Moreover, a gram-scale synthesis of triarylamine 6e was performed and the product was obtained in 57% yield. Subsequently, a wide spectrum of N-heterocycles were subjected to the standard reaction conditions. Encouragingly, positive results were obtained for them (6f–6n), further indicating the broad applicability of this aryne system. Finally, our attempts to expand the scope of aryne precursors were also successful, delivering products 6o–6q in good yields. It should be noted that for the existing approaches, high temperatures and transition metals are often necessary to achieve this type of coupling reaction.24 In this context, our protocol provides a better alternative due to the avoidance of harsh reaction conditions and metal contamination.
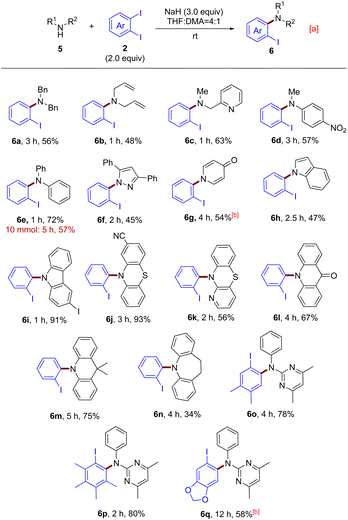 |
| Scheme 3 Substrate scope of amines. a A solution of NaH (1.8 mmol, 3.0 equiv.) and 5 (0.6 mmol, 1.0 equiv.) in THF + DMA (1.0 + 0.5 mL) was stirred for 5 min before 2 (1.2 mmol, 2.0 equiv.) in 1.0 mL THF was added, and the reaction was stirred at rt for the indicated time. b Performed at 40 °C instead of rt. | |
To prove the practicality of this methodology, we used these conveniently obtained products for further synthetic elaboration (Scheme 4). It is well known that iodine is versatile as a handle for extensive functionalizations. In this manner, combining with various amino moieties at the ortho-position, a great variety of pharmaceutically useful molecules could be readily prepared, especially nitrogen-containing heterocycles. First, to meet the derivatization requirement, scale-up reactions of 3bf, 3ca1 and 3cf were conducted (Scheme 2), with yields similar to the yields of the small-scale process, followed by deprotection of the Boc group to afford o-iodoanilines 7, 10 and 15. With these materials in hands, a series of copper or palladium-catalyzed coupling or cyclization reactions were carried out to obtain the important heterocycles 8, 9, 11, 14 and 16. An InCl3-mediated radical process involving 10 and an acrylate afforded compound 12 in a moderate yield. Furthermore, the reaction of 10 and K2S in DMSO at a high temperature without the use of a catalyst gave benzothiazole-2-thione 13 in 75% yield.
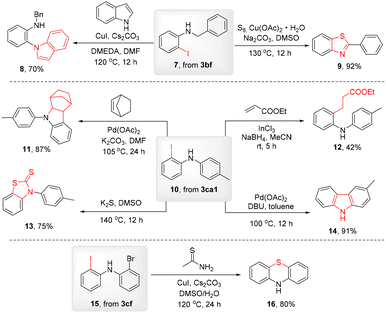 |
| Scheme 4 Elaboration of the products. | |
Conclusions
In summary, we have developed a very simple and practical method for N-arylation of almost all kinds of nitrogen-centered nucleophiles, including various secondary amides, Boc- or Cbz-protected primary alkyl and aryl amines, hydrazine derivatives, urea, lactams, sulfonamides, and many kinds of secondary amines and N-heterocycles. This mechanistically novel protocol is based on the use of o-diiodoarene/NaH as a cheap and convenient combination for aryne generation, which has been previously reported by us to conduct aryne insertion into the C–C σ-bond of unactivated ketones and herein to achieve the mild and efficient preparation of a large number of o-iodoaryl amide/amine products. After further derivatizations, this protocol could provide concise and general routes to access various pharmaceutically interesting molecules.
Conflicts of interest
There are no conflicts to declare.
Acknowledgements
This work was supported by the National Natural Science Foundation of China (22271206 and 22071053), East China University of Science and Technology, the ‘111’ Project and PAPD (A Project Funded by the Priority Academic Program Development of Jiangsu Higher Education Institutions).
References
-
(a) Z.-T. Li, J.-L. Hou and C. Li, Peptide mimics by linear arylamides: A structural and functional diversity test, Acc. Chem. Res., 2008, 41, 1343–1353 CrossRef CAS;
(b) C. Torborg and M. Beller, Recent applications of palladium-catalyzed coupling reactions in the pharmaceutical, agrochemical, and fine chemical industries, Adv. Synth. Catal., 2009, 351, 3027–3043 CrossRef CAS;
(c) C. L. Allen and J. M. Williams, Metal-catalysed approaches to amide bond formation, Chem. Soc. Rev., 2011, 40, 3405–3415 RSC;
(d) A. W. Schmidt, K. R. Reddy and H. J. Knőlker, Occurrence, biogenesis, and synthesis of biologically active carbazole alkaloids, Chem. Rev., 2012, 112, 3193–3328 CrossRef CAS PubMed;
(e) D. W. Zhang, X. Zhao, J. L. Hou and Z. T. Li, Aromatic amide foldamers: Structures, properties, and functions, Chem. Rev., 2012, 112, 5271–5316 CrossRef CAS;
(f) M. E. O'Reilly and A. S. Veige, Trianionic pincer and pincer-type metal complexes and catalysts, Chem. Soc. Rev., 2014, 43, 6325–6369 RSC;
(g) Y. Lin, Z. Li, H. Ma, Y. Wang, X. Wang, S. Song, L. Zhao, S. Wu, S. Tian, C. Fu, L. Luo, F. Zhu, S. He, J. Zheng and X. Zhang, Design, synthesis, and characterization of novel CXCR4 antagonists featuring cyclic amines, ChemMedChem, 2020, 15, 1150–1162 CrossRef CAS PubMed;
(h) R. M. de Figueiredo, J. S. Suppo and J. M. Campagne, Nonclassical routes for amide bond formation, Chem. Rev., 2016, 116, 12029–12122 CrossRef CAS;
(i) P. Ruiz-Castillo and S. L. Buchwald, Applications of palladium-catalyzed C-N cross-coupling reactions, Chem. Rev., 2016, 116, 12564–12649 CrossRef CAS;
(j) J. Wang, K. Liu, L. Ma and X. Zhan, Triarylamine: Versatile platform for organic, dye-sensitized, and perovskite solar cells, Chem. Rev., 2016, 116, 14675–14725 CrossRef CAS PubMed;
(k) X. Ji and Z. Li, Medicinal chemistry strategies toward host targeting antiviral agents, Med. Res. Rev., 2020, 40, 1519–1557 CrossRef CAS PubMed.
- I. Goldberg, Phenylation with presence of copper as catalyst, Ber. Dtsch. Chem. Ges., 1906, 39, 1691–1692 CrossRef.
- P. A. Forero-Cortes and A. M. Haydl, The 25th anniversary of the Buchwald-Hartwig amination: Development, applications, and outlook, Org. Process Res. Dev., 2019, 23, 1478–1483 CrossRef CAS.
- M. J. West, J. W. B. Fyfe, J. C. Vantourout and A. J. B. Watson, Mechanistic development and recent applications of the Chan-Lam amination, Chem. Rev., 2019, 119, 12491–12523 CrossRef CAS PubMed.
-
(a) G. Evano, N. Blanchard and M. Toumi, Copper-mediated coupling reactions and their applications in natural products and designed biomolecules synthesis, Chem. Rev., 2008, 108, 3054–3131 CrossRef CAS;
(b) F. Monnier and M. Taillefer, Catalytic C-C, C-N, and C-O ullmann-type coupling reactions, Angew. Chem., Int. Ed., 2009, 48, 6954–6971 CrossRef CAS;
(c) D. S. Surry and S. L. Buchwald, Dialkylbiaryl phosphines in Pd-catalyzed amination: A user's guide, Chem. Sci., 2011, 2, 27–50 RSC;
(d) C. Sambiagio, S. P. Marsden, A. J. Blacker and P. C. McGowan, Copper catalysed ullmann type chemistry: From mechanistic aspects to modern development, Chem. Soc. Rev., 2014, 43, 3525–3550 RSC.
-
(a) J. P. Wolfe and S. L. Buchwald, Nickel-catalyzed amination of aryl chlorides, J. Am. Chem. Soc., 1997, 119, 6054–6058 CrossRef CAS;
(b) N. H. Park, G. Teverovskiy and S. L. Buchwald, Development of an air-stable nickel precatalyst for the amination of aryl chlorides, sulfamates, mesylates, and triflates, Org. Lett., 2014, 16, 220–223 CrossRef CAS;
(c) S. Ge, R. A. Green and J. F. Hartwig, Controlling first-row catalysts: Amination of aryl and heteroaryl chlorides and bromides with primary aliphatic
amines catalyzed by a binap-ligated single-component Ni(0) complex, J. Am. Chem. Soc., 2014, 136, 1617–1627 CrossRef CAS;
(d) E. B. Corcoran, M. T. Pirnot, S. Lin, S. D. Dreher, D. A. DiRocco, I. W. Davies, S. L. Buchwald and D. W. C. MacMillan, Aryl amination using ligand-free Ni(ii) salts and photoredox catalysis, Science, 2016, 353, 279–283 CrossRef CAS;
(e) C. Li, Y. Kawamata, H. Nakamura, J. C. Vantourout, Z. Liu, Q. Hou, D. Bao, J. T. Starr, J. Chen, M. Yan and P. S. Baran, Electrochemically enabled, nickel-catalyzed amination, Angew. Chem., Int. Ed., 2017, 56, 13088–13093 CrossRef CAS;
(f) C. H. Lim, M. Kudisch, B. Liu and G. M. Miyake, C-N cross-coupling via photoexcitation of nickel-amine complexes, J. Am. Chem. Soc., 2018, 140, 7667–7673 CrossRef CAS PubMed;
(g) Y. Y. Liu, D. Liang, L. Q. Lu and W. J. Xiao, Practical heterogeneous photoredox/nickel dual catalysis for C-N and C-O coupling reactions, Chem. Commun., 2019, 55, 4853–4856 RSC;
(h) S. Gisbertz, S. Reischauer and B. Pieber, Overcoming limitations in dual photoredox/nickel-catalyzed C-N cross-couplings due to catalyst deactivation, Nat. Catal., 2020, 3, 611–620 CrossRef CAS;
(i) N. A. Till, L. Tian, Z. Dong, G. D. Scholes and D. W. C. MacMillan, Mechanistic analysis of metallaphotoredox C-N coupling: Photocatalysis initiates and perpetuates Ni(i)/Ni(iii) coupling activity, J. Am. Chem. Soc., 2020, 142, 15830–15841 CrossRef CAS.
-
(a) S. Duan, S. Venkatraman, X. Hong, K. Huang, L. Ulysse, B. I. Mobele, A. Smith, L. Lawless, A. Locke and R. Garigipati, Process development and scale-up of an Hsp90 inhibitor, Org. Process Res. Dev., 2012, 16, 1787–1793 CrossRef CAS;
(b) L. Wang, E. Ji, N. Liu and B. Dai, Site-selective N-arylation of carbazoles with halogenated fluorobenzenes, Synthesis, 2016, 737–750 CAS;
(c) C. B. Jacobsen, M. Meldal and F. Diness, Mechanism and scope of base-controlled catalyst-free N-arylation of amines with unactivated fluorobenzenes, Chem. – Eur. J., 2017, 23, 846–851 CrossRef.
-
(a) F. Tinnis, E. Stridfeldt, H. Lundberg, H. Adolfsson and B. Olofsson, Metal-free N-arylation of secondary amides at room temperature, Org. Lett., 2015, 17, 2688–2691 CrossRef CAS;
(b) A. H. Sandtorv and D. R. Stuart, Metal-free synthesis of aryl amines: Beyond nucleophilic aromatic substitution, Angew. Chem., Int. Ed., 2016, 55, 15812–15815 CrossRef CAS;
(c) N. Purkait, G. Kervefors, E. Linde and B. Olofsson, Regiospecific N-arylation of aliphatic amines under mild and metal-free reaction conditions, Angew. Chem., Int. Ed., 2018, 57, 11427–11431 CrossRef CAS;
(d) H. Chen, L. Wang and J. Han, Deacetylative aryl migration of diaryliodonium salts with C(sp2)-N bond formation toward ortho-iodo N-aryl sulfonamides, Org. Lett., 2020, 22, 3581–3585 CrossRef CAS;
(e) E. Linde, D. Bulfield, G. Kervefors, N. Purkait and B. Olofsson, Diarylation of N- and O-nucleophiles through a metal-free cascade reaction, Chem., 2022, 8, 850–865 CrossRef CAS.
- M. Puthanveedu, V. Khamraev, L. Brieger, C. Strohmann and A. P. Antonchick, Electrochemical dehydrogenative C(sp2)-H amination, Chem. – Eur. J., 2021, 27, 8008–8012 CrossRef CAS PubMed.
-
(a) N. A. Romero, K. A. Margrey, N. E. Tay and D. A. Nicewicz, Site-selective arene C-H amination via photoredox catalysis, Science, 2015, 349, 1326–1330 CrossRef CAS PubMed;
(b) L.-H. Li, Y. Wei and M. Shi, N-hydroxyphthalimide imidate esters as amidyl radical precursors in the visible light photocatalyzed C-H amidation of heteroarenes, Org. Chem. Front., 2021, 8, 1935–1940 RSC.
-
(a) A. P. Antonchick, R. Samanta, K. Kulikov and J. Lategahn, Organocatalytic, oxidative, intramolecular C-H bond amination and metal-free cross-amination of unactivated arenes at ambient temperature, Angew. Chem., Int. Ed., 2011, 50, 8605–8608 CrossRef CAS PubMed;
(b) S. H. Cho, J. Yoon and S. Chang, Intramolecular oxidative C-N bond formation for the synthesis of carbazoles: Comparison of reactivity between the copper-catalyzed and metal-free conditions, J. Am. Chem. Soc., 2011, 133, 5996–6005 CrossRef CAS PubMed;
(c) C. L. Sun and Z. J. Shi, Transition-metal-free coupling reactions, Chem. Rev., 2014, 114, 9219–9280 CrossRef CAS PubMed;
(d) P. Yang, X. Wang, L. Wang, J. He, Q. Zhang and D. Li, Oxidative cross-dehydrogenative coupling between iodoarenes and acylanilides for C-N bond formation under metal-free conditions, Org. Chem. Front., 2021, 8, 2556–2562 RSC;
(e) S. Sil, A. S. Bhaskaran, S. Chakraborty, B. Singh, R. Kuniyil and S. K. Mandal, Reduced-phenalenyl-based molecule as a super electron donor for radical-mediated C-N coupling catalysis at room temperature, J. Am. Chem. Soc., 2022, 144, 22611–22262 CrossRef CAS PubMed.
-
(a) P. M. Tadross and B. M. Stoltz, A comprehensive history of arynes in natural product total synthesis, Chem. Rev., 2012, 112, 3550–3577 CrossRef CAS PubMed;
(b) J. Shi, Y. Li and Y. Li, Aryne multifunctionalization with benzdiyne and benztriyne equivalents, Chem. Soc. Rev., 2017, 46, 1707–1719 RSC;
(c) H. Takikawa, A. Nishii, T. Sakai and K. Suzuki, Aryne-based strategy in the total synthesis of naturally occurring polycyclic compounds, Chem. Soc. Rev., 2018, 47, 8030–8056 RSC;
(d) J. Shi, L. Li and Y. Li,
O-silylaryl triflates: A journey of kobayashi aryne precursors, Chem. Rev., 2021, 121, 3892–4044 CrossRef CAS.
-
(a) Z. Liu and R. C. Larock, Facile N-arylation of amines and sulfonamides, Org. Lett., 2003, 5, 4673–4675 CrossRef CAS PubMed;
(b) Z. Liu and R. C. Larock, Facile N-arylation of amines and sulfonamides and O-arylation of phenols and arenecarboxylic acids, J. Org. Chem., 2006, 71, 3198–3209 CrossRef CAS;
(c) J. C. Haber, M. A. Lynch, S. L. Spring, A. D. Pechulis, J. Raker and Y. Wang, A facile N-arylation of acetanilides with arynes, Tetrahedron Lett., 2011, 52, 5847–5850 CrossRef CAS;
(d) M. Wang and Z. Huang, Transition metal-free N-arylation of secondary amides through iodonium salts as aryne precursors, Org. Biomol. Chem., 2016, 14, 10185–10188 RSC;
(e) L. Zhang, Y. Geng and Z. Jin, Transition-metal-free synthesis of N-aryl hydroxamic acids via insertion of arynes, J. Org. Chem., 2016, 81, 3542–3552 CrossRef CAS PubMed.
-
(a) H. Yoshida, E. Shirakawa, Y. Honda and T. Hiyama, Addition of ureas to arynes: Straightforward synthesis of benzodiazepine and benzodiazocine derivatives, Angew. Chem., Int. Ed., 2002, 41, 3247–3249 CrossRef CAS;
(b) Z. Liu and R. C. Larock, Intermolecular C-N addition of amides and S-N addition of sulfinamides to arynes, J. Am. Chem. Soc., 2005, 127, 13112–13113 CrossRef CAS PubMed;
(c) D. G. Pintori and M. F. Greaney, Insertion of benzene rings into the amide bond: One-step synthesis of acridines and acridones from aryl amides, Org. Lett., 2010, 12, 168–171 CrossRef CAS;
(d) Y. Fang, D. C. Rogness, R. C. Larock and F. Shi, Formation of acridones by ethylene extrusion in the reaction of arynes with beta-lactams and dihydroquinolinones, J. Org. Chem., 2012, 77, 6262–6270 CrossRef CAS PubMed;
(e) J. Kim and B. M. Stoltz, A C-N insertion of β-lactam to benzyne: Unusual formation of acridone, Tetrahedron Lett., 2012, 53, 4994–4996 CrossRef CAS;
(f) N. Saito, K.-i. Nakamura, S. Shibano, S. Ide, M. Minami and Y. Sato, Addition of cyclic ureas and 1-methyl-2-oxazolidone to pyridynes: A new approach to pyridodiazepines, pyridodiazocines, and pyridooxazepines, Org. Lett., 2013, 15, 386–389 CrossRef CAS;
(g) M. Mesgar and O. Daugulis, Silylaryl halides can replace triflates as aryne precursors, Org. Lett., 2016, 18, 3910–3913 CrossRef CAS;
(h) M. Asamdi and K. H. Chikhalia, Aryne insertion into σ bonds, Asian J. Org. Chem., 2017, 6, 1331–1348 CrossRef CAS;
(i) A. Shamsabadi and V. Chudasama, A facile route to 1H- and 2H-indazoles from readily accessible acyl hydrazides by exploiting a novel aryne-based molecular rearrangement, Chem. Commun., 2018, 54, 11180–11183 RSC;
(j) A. Nilova, P. A. Sibbald, E. J. Valente, G. A. Gonzalez-Montiel, H. C. Richardson, K. S. Brown, P. H.-Y. Cheong and D. R. Stuart, Regioselective synthesis of 1,2,3,4-tetrasubstituted arenes by vicinal functionalization of arynes derived from aryl(mes)iodonium salts, Chem. – Eur. J., 2021, 27, 7168–7175 CrossRef CAS.
- J. D. Hicks, A. M. Hyde, A. M. Cuezva and S. L. Buchwald, Pd-catalyzed N-arylation of secondary acyclic amides: Catalyst development, scope, and computational study, J. Am. Chem. Soc., 2009, 131, 16720–16734 CrossRef CAS PubMed.
-
(a) D. Pena, A. Cobas, D. Perez and E. Guitian, An efficient procedure for the synthesis of ortho-trialkylsilylaryl triflates: Easy access to precursors of functionalized arynes, Synthesis, 2002, 1454–1458 CAS;
(b) D. J. Atkinson, J. Sperry and M. A. Brimble, Improved synthesis of the benzyne precursor 2-(trimethylsilyl)phenyl trifluoromethanesulfonate, Synthesis, 2010, 911–913 CAS.
-
(a) D. Y. Ong, C. Tejo, K. Xu, H. Hirao and S. Chiba, Hydrodehalogenation of haloarenes by a sodium hydride-iodide composite, Angew. Chem., Int. Ed., 2017, 56, 1840–1844 CrossRef CAS PubMed;
(b) Y. Huang, G. H. Chan and S. Chiba, Amide-directed C-H sodiation by a sodium hydride/iodide composite, Angew. Chem., Int. Ed., 2017, 56, 6544–6547 CrossRef CAS;
(c) D. Y. Ong, Z. Yen, A. Yoshii, J. R. Imbernon, R. Takita and S. Chiba, Controlled reduction of carboxamides to alcohols or amines by zinc hydrides, Angew. Chem., Int. Ed., 2019, 58, 4992–4997 CrossRef CAS;
(d) D. Y. Ong, J. H. Pang and S. Chiba, Synthetic organic reactions mediated by sodium hydride, J. Synth. Org. Chem., Jpn., 2019, 77, 1060–1069 CrossRef CAS;
(e) B. Wang, D. Y. Ong, Y. Li, J. H. Pang, K. Watanabe, R. Takita and S. Chiba, Stereo-controlled anti-hydromagnesiation of aryl alkynes by magnesium hydrides, Chem. Sci., 2020, 11, 5267–5272 RSC.
-
(a) Y. Mao, Y. Liu, Y. Hu, L. Wang, S. Zhang and W. Wang, Pd-catalyzed debenzylation and deallylation of ethers and esters with sodium hydride, ACS Catal., 2018, 8, 3016–3020 CrossRef CAS;
(b) Y. Liu, Y. Mao, Y. Hu, J. Gui, L. Wang, W. Wang and S. Zhang, The employment of sodium hydride as a michael donor in palladium-catalyzed reductions of α, β-unsaturated carbonyl compounds, Adv. Synth. Catal., 2019, 361, 1554–1558 CrossRef CAS;
(c) W. Sun, X. Chen, Y. Hu, H. Geng, Y. Jiang, Y. Zhou, W. Zhu, M. Hu, H. Hu, X. Wang, X. Wang, S. Zhang and Y. Hu, A NaH-promoted N-detosylation reaction of diverse p-toluenesulfonamides, Tetrahedron Lett., 2020, 61, 152442 CrossRef CAS;
(d) J. Gui, X. Cai, L. Chen, Y. Zhou, W. Zhu, Y. Jiang, M. Hu, X. Chen, Y. Hu and S. Zhang, Facile and practical hydrodehalogenations of organic halides enabled by calcium hydride and palladium chloride, Org. Chem. Front., 2021, 8, 4685–4692 RSC;
(e) F. Luo, X. Chen, J. Yu, Y. Yin, X. Hu, Y. Hu, X. Liu, X. Chen, S. Zhang and Y. Hu, Palladium-catalyzed transfer hydrogenation and acetylation of N-Heteroarenes with sodium hydride as the reductant, Synthesis, 2023, 1451–1459 CrossRef;
(f) F. Luo, C. Li, P. Ji, Y. Zhou, J. Gui, L. Chen, Y. Yin, X. Zhang, Y. Hu, X. Chen, X. Liu, X. Chen, Z. Yu, W. Wang and S. Zhang, Direct aryne insertion into C-C σ-bond of unactivated ketones enabled by NaH-mediated aryne chemistry, Chem., 2023, 9, 2620–2636 CrossRef CAS.
-
(a) D. S. Roman, Y. Takahashi and A. B. Charette, Potassium tert-butoxide promoted intramolecular arylation via a radical pathway, Org. Lett., 2011, 13, 3242–3245 CrossRef CAS;
(b) Y. Yang, X. Zhang, W. Zeng, H. Huang and Y. Liang, Copper catalyzed three-component synthesis of benzothiazolones from o-iodoanilines, DMF, and potassium sulfide, RSC Adv., 2014, 4, 6090–6093 RSC;
(c) P. Dang, W. Zeng and Y. Liang, Copper-catalyzed three-component synthesis of benzothiazolethiones from o-iodoanilines, isocyanide, and potassium sulfide, Org. Lett., 2015, 17, 34–37 CrossRef CAS PubMed;
(d) W. Dong, Y. Liu, B. Hu, K. Ren, Y. Li, X. Xie, Y. Jiang and Z. Zhang, Visible light induced radical cyclization of o-iodophenylacrylamides: A concise synthesis of indolin-2-one, Chem. Commun., 2015, 51, 4587–4590 RSC;
(e) S. B. Nallapati, R. Adepu, M. A. Ashfaq, B. Y. Sreenivas, K. Mukkanti and M. Pal, A Pd-catalyzed direct entry to 11-substituted 6H-isoindolo[2,1-a]indol-6-one derivatives as potential anticancer agents, RSC Adv., 2015, 5, 88686–88691 RSC;
(f) X. Li, B. Zhou, R.-Z. Yang, F.-M. Yang, R.-X. Liang, R.-R. Liu and Y.-X. Jia, Palladium-catalyzed enantioselective intramolecular dearomative heck reaction, J. Am. Chem. Soc., 2018, 140, 13945–13951 CrossRef CAS PubMed;
(g) Z. M. Zhang, B. Xu, L. Wu, L. Zhou, D. Ji, Y. Liu, Z. Li and J. Zhang, Palladium/xuphos-catalyzed enantioselective carboiodination of olefin-tethered aryl iodides, J. Am. Chem. Soc., 2019, 141, 8110–8115 CrossRef CAS PubMed;
(h) X. Abel-Snape, A. Whyte and M. Lautens, Synthesis of aminated phenanthridinones via palladium/norbornene catalysis, Org. Lett., 2020, 22, 7920–7925 CrossRef CAS PubMed;
(i) A. R. Flynn, K. A. McDaniel, M. E. Hughes, D. B. Vogt and N. T. Jui, Hydroarylation of arenes via reductive radical-polar crossover, J. Am. Chem. Soc., 2020, 142, 9163–9168 CrossRef CAS;
(j) P. Yang, C. Zheng, Y. H. Nie and S. L. You, Palladium-catalyzed dearomative 1,4-difunctionalization of naphthalenes, Chem. Sci., 2020, 11, 6830–6835 RSC;
(k) W. Chen, X. Tu, M. Xu, Y. Chu and Y. Zhu, Copper(I) iodide catalyzed tandem reactions of N-propargyl-N,-(2-iodoaryl)amides with sodium azide: An efficient synthesis of [1,2,3]triazolo[1,5-a]quinoxalines, Synlett, 2021, 32, 805–809 CrossRef CAS;
(l) Y. Zuo, X. He, Q. Tang, W. Hu, T. Zhou, W. Hu and Y. Shang, Palladium-catalyzed 5-exo-dig cyclization cascade, sequential amination/etherification for stereoselective construction of 3-methyleneindolinones, Adv. Synth. Catal., 2021, 363, 2117–2123 CrossRef CAS;
(m) L. Li, X. L. Liu, Z. Qi, A. H. Yang, A. J. Ma and J. B. Peng, Palladium-catalyzed carbonylative sonogashira/annulation reaction: Synthesis of indolo[1,2-b]isoquinolines, Org. Lett., 2022, 24, 1201–1206 CrossRef CAS PubMed.
-
(a) U. K. Sharma, N. Sharma, Y. Kumar, B. K. Singh and E. V. Van der Eycken, Domino carbopalladation/C-H functionalization sequence: An expedient synthesis of bis-heteroaryls through transient alkyl/vinyl-palladium species capture, Chem. – Eur. J., 2016, 22, 481–485 CrossRef CAS PubMed;
(b) P. P. Sharp, J. M. Garnier, T. Hatfaludi, Z. Xu, D. Segal, K. E. Jarman, H. Jousset, A. Garnham, J. T. Feutrill, A. Cuzzupe, P. Hall, S. Taylor, C. R. Walkley, D. Tyler, M. A. Dawson, P. Czabotar, A. F. Wilks, S. Glaser, D. C. S. Huang and C. J. Burns, Design, synthesis, and biological activity of 1,2,3-triazolobenzodiazepine bet bromodomain inhibitors, ACS Med. Chem. Lett., 2017, 8, 1298–1303 CrossRef CAS PubMed.
-
(a) F. Leroux and M. Schlosser, The “aryne” route to biaryls featuring uncommon substituent patterns, Angew. Chem., Int. Ed., 2002, 41, 4272–4274 CrossRef CAS;
(b) F. R. Leroux, L. Bonnafoux, C. Heiss, F. Colobert and D. A. Lanfranchi, A practical transition metal-free aryl-aryl coupling method: Arynes as key intermediates, Adv. Synth. Catal., 2007, 349, 2705–2713 CrossRef CAS;
(c) L. Bonnafoux, F. Colobert and F. R. Leroux, 2,2′,6-tribromobiphenyl via transition-metal-free aryne coupling: A valuable tool in the synthesis of biphenyls, Synlett, 2010, 2953–2955 CAS;
(d) V. Diemer, M. Begaud, F. R. Leroux and F. Colobert, Regioselectivity in the aryne cross-coupling of aryllithiums with functionalized 1,2-dibromobenzenes, Eur. J. Org. Chem., 2011, 341–354 CrossRef CAS;
(e) A. Berthelot-Brehier, A. Panossian, F. Colobert and F. R. Leroux, Atroposelective synthesis of axially chiral p,s-lig ands based on arynes, Org. Chem. Front., 2015, 2, 634–644 RSC;
(f) T. Hamura, Y. Chuda, Y. Nakatsuji and K. Suzuki, Catalytic generation of arynes and trapping by nucleophilic addition and iodination, Angew. Chem., Int. Ed., 2012, 51, 3368–3372 CrossRef CAS.
-
(a) Y. Liu, H. Wang, X. Cao, Z. Fang and J.-P. Wan, Copper-catalyzed selective single arylsulfanylation of aryl diiodides with aryl thiols, Synthesis, 2013, 2977–2982 CAS;
(b) L. Yadav, M. K. Tiwari, B. R. K. Shyamlal and S. Chaudhary, Organocatalyst in direct C(sp2)-H arylation of unactivated arenes: [1-(2-hydroxyethyl)-piperazine]-catalyzed inter-/intra-molecular C-H bond activation, J. Org. Chem., 2020, 85, 8121–8141 CrossRef CAS PubMed;
(c) A. O. Alsahli and A. N. Cammidge, Synthesis and mesophase properties of triphenylene dimers linked through linear and bent alkyne-aryl-alkyne bridges, Eur. J. Org. Chem., 2022, e202200990 CrossRef CAS.
-
(a) S. Tripathy, R. LeBlanc and T. Durst, Formation of 2-substituted iodobenzenes from iodobenzene via benzyne and ate complex intermediates, Org. Lett., 1999, 1, 1973–1975 CrossRef CAS;
(b) W. Lin, I. Sapountzis and P. Knochel, Preparation of functionalized aryl magnesium reagents by the addition of magnesium aryl thiolates and amides to arynes, Angew. Chem., Int. Ed., 2005, 44, 4258–4261 CrossRef CAS;
(c) Y. Zeng, L. Zhang, Y. Zhao, C. Ni, J. Zhao and J. Hu, Silver-mediated trifluoromethylation-iodination of arynes, J. Am. Chem. Soc., 2013, 135, 2955–2958 CrossRef CAS;
(d) T. Truong, M. Mesgar, K. K. Le and O. Daugulis, General method for functionalized polyaryl synthesis via aryne intermediates, J. Am. Chem. Soc., 2014, 136, 8568–8576 CrossRef CAS PubMed;
(e) Y. Zeng, G. Li and J. Hu, Diphenyliodonium-catalyzed fluorination of arynes: Synthesis of ortho-fluoroiodoarenes, Angew. Chem., Int. Ed., 2015, 54, 10773–10777 CrossRef CAS;
(f) M. Zhou, C. Ni, Y. Zeng and J. Hu, Trifluoromethyl benzoate: A versatile trifluoromethoxylation reagent, J. Am. Chem. Soc., 2018, 140, 6801–6805 CrossRef CAS;
(g) S. J. Li, L. Han and S. K. Tian, 1,2-aminohalogenation of arynes with amines and organohalides, Chem. Commun., 2019, 55, 11255–11258 RSC;
(h) W. Cao, S. L. Niu, L. Shuai and Q. Xiao, Copper-catalysed three-component carboiodination of arynes: Expeditious synthesis of o-alkynyl aryl iodides, Chem. Commun., 2020, 56, 972–975 RSC;
(i) S. Cho and Q. Wang, 1,2-difunctionalization of aryl triflates: A direct and modular access to diversely functionalized anilines, Org. Lett., 2020, 22, 1670–1674 CrossRef CAS;
(j) Y. Huang, Y. Hu, Y. Han, Y. Ou, Y. Huo, X. Li and Q. Chen, Direct synthesis of ortho-halogenated arylphosphonates via a three-component reaction involving arynes, J. Org. Chem., 2021, 86, 7010–7018 CrossRef CAS PubMed.
-
(a) A. J. Paine, Mechanisms and models for copper mediated nucleophilic aromatic substitution. 2. Single catalytic species from three different oxidation states of copper in an ullmann synthesis of triarylamines, J. Am. Chem. Soc., 1987, 109, 1496–1502 CrossRef CAS;
(b) M. Ito, R. Kawasaki, K. S. Kanyiva and T. Shibata, Construction of a polycyclic conjugated system containing a dibenzazepine moiety by cationic gold(I)-catalyzed cycloisomerization, Eur. J. Org. Chem., 2016, 5234–5237 CrossRef CAS;
(c) M. Lu, O. Allemann, J. Xu, A. Linden, K. K. Baldridge and J. S. Siegel, Peraryl-x-onium ions of nitrogen and oxygen, Org. Chem. Front., 2019, 6, 2640–2646 RSC.
|
This journal is © the Partner Organisations 2024 |
Click here to see how this site uses Cookies. View our privacy policy here.