DOI:
10.1039/D4PM00079J
(Paper)
RSC Pharm., 2024,
1, 775-785
Effect of leucine as an aerosolization enhancer on the bioactivity of spray dried viral-vectored vaccines for inhalation
Received
16th March 2024
, Accepted 22nd June 2024
First published on 2nd July 2024
Abstract
Aerosolization enhancers, like L-leucine, can improve deep-lung deposition of inhalable dry powders although the implications of their use have not yet been evaluated for sensitive biologics like viral vectored vaccines. This study investigates the effect of L-leucine concentrations (0–50 wt%) as an added component to the viral-encapsulating matrix comprised of mannitol and dextran, on aerosolization relative to bioactivity of spray dried human serotype 5 adenovirus. Modelling the intended purpose of inhalation, the aerodynamic properties (fine particle fraction and mass median aerodynamic diameter) of the powders were analyzed using a Next Generation Impactor. Overall, increasing the L-leucine concentration in the spray dried formulations improved the fine particle fraction (>40%) and reduced the aerodynamic diameter (<5 μm). However, bioactivity was negatively affected by the presence of L-leucine in the formulation and that demanded deeper investigation. The root cause for the declining bioactivity was finally attributed to aggregation of the adenovirus induced by L-leucine in the feed solution prior to spray drying, which was determined using a qViro-X particle counter. The intent of this study was to emphasize that advantages and disadvantages will exist with additives like an aerosolization enhancer, for this relatively new class of vaccines.
1. Introduction
Pulmonary delivery of dry powder vaccines via inhalation is known to confer more effective protection than parenteral delivery against respiratory infections like tuberculosis.1–4 It has been further demonstrated that delivery of vaccine to the lower respiratory airways (deep lung) induces an enhanced immunogenic response.5–8 The aerodynamic size for successfully delivering aerosol to the lower respiratory tract should ideally be less than 10 μm. For aerosol deposition in the oropharynx, larynx and proximal airways, aerosol size between 2–5 μm is ideal for deposition in the central airways, and 0.5–2 μm for the peripheral airways.9–13 Spray drying is potentially employed to produce powders suitable for inhalation, offering the ability to engineer the morphological and physicochemical properties of the particles.14–18
Although dry powder viral vaccines are gaining acceptance as a viable technology to tackle cold chain complications, there remain barriers before their use for pulmonary delivery will be accepted for public use. Two major hurdles to clinical adoption of inhalable vaccine powders include inconsistent aerosolization and low potency. One of the commonly used approaches employed to achieve consistent aerosolization of dry powders is addition of dispersibility enhancers, like L-leucine, to a formulation.19–25L-Leucine as an excipient has demonstrated its potential to improve the aerosolization of dry powders containing small molecules like respiratory drugs, antibiotics, siRNA, and monoclonal antibodies (mAb).10,20,26–32
Unlike smaller molecules, biologics have complex large molecular structures consisting of more potential sites for degradation and are highly prone to degradation.33,34 Biologics like non-enveloped adenoviruses, are particularly sensitive to various stresses, such as shear stress, air–liquid interfaces, and heat during continuous dry powder preparation methods, which can be damaging despite their economic advantages.35,36 The effect of process related stresses on biological activity of such biologics have been studied previously, however, the biological implications of L-leucine on active biologics like viral vectored vaccines (human adenovirus type 5 in this work) remain unexplored. L-Leucine displayed no nature to stabilize the viral vector37 as a spray-dried encapsulating matrix but the potential of this aid to improve the aerosolization properties of this new class of vaccines requires that its advantages and disadvantages are thoroughly studied. Therefore, exploring L-leucine with adenovirus formulations presents a unique study since this information is presently missing from the scientific literature.
In this study, various concentrations of L-leucine were examined to understand how this amino acid was affecting the adenovirus relative to its expected improvement to aerosolizing our spray dried powder for pulmonary delivery. For its importance in vaccination against pulmonary infectious diseases, human type 5 adenovirus (AdHu5) was used as the viral vector encapsulated in these inhalable particles under study. The mannitol/dextran (3
:
1 weight ratio) formulation was chosen as the control due to its well-studied ability to efficiently encapsulate AdHu5 during spray drying.37–40 Mannitol/dextran (hereafter abbreviated as MD) formulation retains high viral activity, offers exceptional thermostability of AdHu5 for a prolonged period of storage at room temperature, and is intended for inhalation delivery.37,38,41
2. Materials and methods
2.1. Materials and adenoviral vector
Dextran (40 kDa), D-mannitol, and L-leucine were purchased as USP grade from Sigma-Aldrich (Ontario, Canada). Milli-Q water with a resistivity of 18.2 MΩ cm was obtained from a Barnstead GenPure Pro water purification system (Thermo Fisher Scientific, Waltham, MA). The viral vector AdHu5-GFP (human type 5 adenovirus expressing green fluorescent protein) was produced in the vector facility of McMaster Immunology Research Centre (MIRC) as described previously.42 Lung epithelial (A549) cells (CCL-185, American Type Culture Collection (ATCC), Manassas, VA, United States) were used for in vitro viral activity testing of the viral vector. A549 cells were grown in Alpha Minimum Essential Medium (α-MEM) prepared in house according to the protocol by the supplier (Life Technologies, Ontario, Canada) and supplemented with 10% fetal bovine serum and 1% streptomycin/penicillin (Invitrogen; Ontario, Canada).
2.2. Preparation of spray dried powders
A 1% (w/v) aqueous solution of mannitol/dextran (MD, 75%/25% (weight basis)) was prepared as the control formulation. L-Leucine (hereafter referred to as leucine) containing MD formulations were prepared using three different concentrations of leucine: 15% (w/w), 30% (w/w), and 50% (w/w) of the solids content in the control formulation. The four formulations compared in the study are labelled as MD, 15%LL-MD, 30%LL-MD, and 50% LL-MD. For spray drying AdHu5 containing formulations, a 10 μL dosage of AdHu5 stock solution at 1010 pfu (plaque forming units) was added in a 10 mL feedstock solution, making a final viral titer of 107 pfu.
The prepared formulations were spray dried using a B-290 mini spray dryer (Büchi, Switzerland) equipped with a high-performance cyclone and 0.7 mm nozzle. All samples were spray dried at a single set of parameters based on a previous study to produce suitably sized particles for aerosolization with acceptable AdHu5 losses.43 These parameters were: spray gas flow rate of 439.11 L h−1, inlet temperature of 120 °C, feed solution rate of 188.5 mL h−1, and an outlet temperature ranging from 60–62 °C. To allow meaningful comparisons between spray dried samples, these parameters were used without optimization per formulation, resulting in higher activity losses for the adenovirus than normally acceptable (generally less than 0.5 log titer loss). Spray dried samples were stored in sealable polyethylene bags with indicating silica gel desiccant and were tested on the same day as they were spray dried (usually within 2–3 hours).
2.3. Powder particle characterization
Most of the characterization of powders, except the powders prepared for in vitro activity testing, was conducted on spray dried powders without AdHu5-GFP to avoid biosafety concerns. We expect the placebo powders and AdHu5-containing powders to be identical in morphology and particle properties due to the tiny fraction (<1/10
000th by liquid volume) of viral vector present compared to the excipients, as demonstrated in a previous study.37
2.3.1. Geometric particle size distribution.
Laser diffraction using a HELOS sensor with the R2 lens (0.25/0.45–87.5 μm) (Sympatec GmbH, Clausthal-Zellerfeld, Germany) was used to measure the geometric particle size of the spray dried samples. The powder was dispersed using a dry powder inhalation (DPI) device, ICOone®, kindly donated by Iconovo, Sweden. ICOone® is a commercial ultralow-cost single-dose inhaler, moulded in one piece44 with Al-foil intended to protect the formulation in the dosing chamber, though no foil was used in this study. With the addition of a protective foil, the inhaler becomes suitable for the administration of inhaled therapeutics, including vaccines. The sample powders were dispersed into the Sympatec from an ICOone® with a pressure difference of 4 kPa. Measurements of geometric particle size distribution were done in triplicates. The span value was calculated using eqn (1), where D10 represents the diameter greater than 10% of measured particles, D50 represents the median diameter, and D90 represents the diameter greater than 90% of measured particles. | 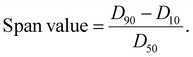 | (1) |
2.3.2. Particle morphology (scanning electron microscopy).
Particle morphology was analyzed using a scanning electron microscope (SEM) (JEOL-JSM 7000F; JEOL Ltd, Japan). Powder samples were mounted on double-sided tape attached to an aluminum stub, and excess powder was dusted off. A Polaron E5100 sputter coater (Polaron Equipment Ltd, Watford, Hertfordshire) was used to coat the mounted powder samples with a 24 nm layer of gold. Images were taken at an electron accelerating voltage of 5 kV and a working distance of approximately 17 mm.
2.3.3. X-ray photoelectron spectroscopy (XPS) for surface composition analysis.
Spray dried samples were analyzed for surface elemental composition analysis using a Quantera II model X-ray photoelectron spectrometer manufactured by Physical Electronics Inc. A K-alpha monochromatic X-ray source (1486.6 eV) was used, and spectra of elements (carbon, oxygen, and nitrogen) were collected at 55 eV pass energy with 0.1 eV energy increments. XPS spectra were analyzed using Multipak software, version 9 (PHI Sales, Laval, Quebec). Gaussian–Lorentzian peak deconvolution and the Shirley background reduction method were facilitated for spectra analysis.
2.3.4. Thermogravimetric analysis (TGA) for moisture content analysis.
The residual moisture content of the samples was determined using TGA/DSC 3 + instrument (Mettler Toledo; Columbus, OH). Spray dried samples (5–10 mg) without viral vector were heated in aluminum crucibles at a rate of 5 °C per minute up to 150 °C under argon gas. Stable mass loss up to 150 °C was monitored, and residual moisture content was calculated using the Stare software (Mettler Toledo; Columbus, OH).
2.4.
In vitro aerosol performance using next generation impactor
Aerosol performance of the spray dried powders was analyzed in vitro using a Next Generation Impactor (NGI) (MSP Corporation Model 170, Copley Scientific; Nottingham, UK) with the standard right-angle stainless-steel inlet. Filter papers were sized to fit the NGI stages, weighed and placed on each stage before actuation. Five ICOone inhalers were weighed, loaded with 10 ± 2 mg of powder in each, and actuated in succession at an inspiratory flow rate of 60 Lpm over 4 s to achieve 4 L of air withdrawal with a 4 kPa pressure drop (USP <601>).45 Post actuation, the inhalers and all the filters (on each stage) were weighed using a microbalance. The instrument was set up, and mass median aerodynamic diameter (MMAD), fine particle fraction (FPF), and available dose (AD, sometimes called emitted dose) were obtained based on gravimetric analysis of powder deposited at each stage, as described elsewhere.41
The cut-off diameters of each stage of the NGI were recalculated for the 60 Lpm sampling air flowrate,45 and the MMAD of the powder was derived as the particle size that crossed past 50% of cumulative mass fraction from the plot of stage cut-off diameters vs. cumulative mass fraction. The FPF was obtained by interpolating the plot of stage cut-off diameter vs. cumulative fraction of powder stages. FPF was expressed as the percentage of powder mass below the aerodynamic diameter of 5 μm and was expressed relative to the available dose (FPF/AD). AD was determined as the percent of total loaded mass exiting the ICOone inhaler post-actuation. The MMAD, FPF, and AD were expressed as the mean of triplicate impactor runs (n = 3) and error intervals are the standard error.
2.5.
In vitro viral activity testing
2.5.1. Culturing A549 cells.
Lung epithelial cells (A549) were cultured in T150 culture flasks using Minimum Essential Medium Eagle (α-MEM) after revival from liquid nitrogen storage. Controlled environmental conditions were used to culture the cells at 37.0 °C and 5% CO2 in a water-jacketed CO2 incubator (Forma Series II, Thermo Scientific Corporation; Waltham, MA). For in vitro activity testing, the cells were split and plated in a 96-well plate.
2.5.2. Viral infectivity of spray dried formulations.
To minimize the potential activity loss due to storage at room temperature, samples containing viral vectors were stored on ice before spray drying and were assessed on the same day as they were spray dried. The spray dried samples were reconstituted in cell culture media to attain solids concentration matching the initial solids concentration before spray drying. These reconstituted powders (100 μL) were used to transfect A549 cells, cultured for 24 hours in a flat-bottom 96-well plate starting at a concentration of 4 × 104 cells per well. The transfected cells were prepared for flow cytometry (as described previously)35 after keeping the infected cells overnight. Flow cytometry was done using a MACSQuant Analyzer 10 (Miltenyi Biotec; Bergisch Gladbach, Germany), and the data obtained were processed in FlowJo software (Tree Star; Ashland, OR) as described elsewhere.35 The obtained GFP expressing cells were compared against a standard curve for the modality of infection (0.1 to 100) vs. percentage of GFP expressing cells. The titer of the spray dried viral vector was thus calculated and used for obtaining the titer log loss before and after spray drying by eqn (2). |  | (2) |
2.6. Viral aggregation measurements (using nanoparticle counter)
Viral aggregation of AdHu5 in the liquid formulation before spray drying was analyzed by the qViro-X nanoparticle counter, using a protocol described previously.35 A single nanopore comprising of a thermoplastic polyurethane membrane (NP150) was used, for which the analysis range of particle sizes varied from 70–420 nm (Izon Science; Christchurch, New Zealand). To increase the conductivity of samples in the range required for the qViro-X instrument and to meet the recommended particle concentration (5 × 109 particles per mL), samples were diluted by a factor of 10 in electrolyte buffer (10 mM HEPES, 150 mM NaCl, and 4% by weight sucrose) before testing.
Three liquid formulations were tested: (1) positive control composed of the viral vector stock suspension (without excipients) diluted in the electrolyte buffer (1
:
10), (2) 1%MD solution containing AdHu5 diluted in formulation buffer to attain a final 1
:
10 viral vector stock suspension to liquid ratio, and (3) highest leucine concentration containing liquid formulation (1.5% leucine-MD solution) with AdHu5 and diluted in formulation buffer to achieve a 1
:
10 viral vector stock suspension to liquid ratio. All experiments were run at a voltage of 0.46 volt applied across the pore, and a pressure of 0.6 kPa applied to the fluid cell to increase the rate of particles passing through the pore. The recording of the passage of particles through the membrane were limited by the q-Viro-X control software and measured until either 500 events had been recorded or 10 min had elapsed. CPC100 qNano carboxylated polystyrene calibration beads with the mean particle size of 115 nm (Izon Science; Christchurch, New Zealand) were used to calibrate the relationship between particle size and blockade event magnitude.
3. Results and discussion
3.1. Effect of leucine concentrations on powder/particle characteristics
To display the effect of leucine on bioactivity relative to the improved aerosolization properties that it offers, various concentrations were examined in the spray dried formulation with the viral vector. Solid-state properties of spray dried powders containing these differing concentrations of leucine were analyzed and are discussed in this section. Powder yield was calculated as the percentage of dry powder collected after spray drying relative to the initial mass of solids in the spray drying formulation. The yield of spray dried samples in this study varied from 60% to 83% (±5%) and increased with increasing leucine concentration in the liquid feed.
3.1.1. Geometric particle size distribution.
Particle size is crucial in governing the effective deposition of powders in the lungs, indicated in the clinical effectiveness of an inhaled product.46,47 The mean geometric particle size (D50) for our spray dried samples ranged from 5.7 μm to 9.0 μm, as seen in Fig. 1. The particle size (D50) for the 15% LL-MD sample was similar to the control. As the leucine concentration further increased in the samples (referring to 30% LL-MD and 50% LL-MD), the particle size also increased. In the control, dextran is expected to precipitate faster, enriching the surface of drying particles due to its lower solubility in water (30 mg mL−1 for dextran vs. 216 mg mL−1 for mannitol). With additions of leucine, its notably high surface activity coupled with low aqueous solubility leads to its precipitation at the surface of drying droplets faster than other components,12 causing larger but more often, hollow, particles as a result.
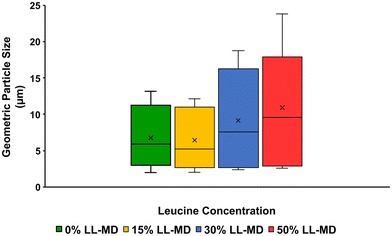 |
| Fig. 1 Geometric particle size distribution of spray dried samples containing various leucine concentrations ranging from 0% to 50% in a mannitol/dextran blend. The distribution spans from D10 to D90 for each represented sample. The line in the middle of the size distribution boxes represents the D50 of the particles for each sample, while the cross marks represent the mean of the distribution. | |
The span values of these spray dried powders, indicating the breadth of the size distribution, were 1.6, 1.6, 2.0 and 2.1 for 0%LL-MD, 15% LL-MD, 30% LL-MD, and 50% LL-MD, respectively. From the small span values and the monomodal nature for the size distributions, it is anticipated that the bulk of particles of a sample will display near-consistent properties in their aerosolization and deposition when inhaled, based on the findings of others studying particles of similar attributes.25
3.1.2. Surface texture of particles with leucine addition (particle morphology).
The result of increasing leucine concentration on particle morphology of the spray dried powders was characterized by SEM (Fig. 2). The control MD particles with no leucine appeared smooth, spherical and with no noticeable wrinkling at the surface (Fig. 2A), as has been seen previously.37,40 The 15% LL-MD sample showed some wrinkled particles, although the majority appeared close to the control in morphology with smooth and spherical shapes (Fig. 2B). Compared to smooth particles, wrinkled particles were more uniformly distributed in the 15% LL-MD sample (Fig. 2B). Comparatively, the 30% LL-MD particles appeared more wrinkled (or roughened) than 15%LL-MD. Small, and generally smooth, particles were seen to be settled in the pockets formed by the larger corrugated particles (Fig. 2C), which could pose a negative impact on the overall aerodynamics of the 30% LL-MD powder sample due to a greater overall number of large sized particles. The 50% LL-MD particles appeared to have deep fractures in the surface, with the larger particles being hollow with a thin labile shell, as evident by the broken particles in Fig. 2D.
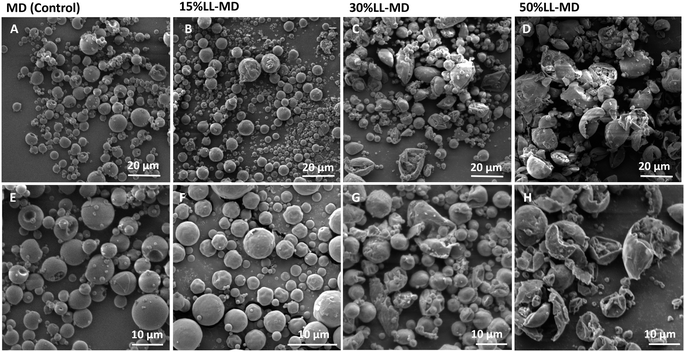 |
| Fig. 2 Scanning electron microscopy images of spray dried samples of MD control and leucine containing samples. (A and E). Mannitol/dextran (control) particles, (B and F). 15% leucine in MD containing particles, (C and G). 15% leucine in MD containing particles, and (D and H). 15% leucine in MD containing particles. The images A to D are magnified at 2000× at a scale of 20 micrometers, while images E, G, H at 4000×, and the image F at 6500× at a scale of 10 micrometers. | |
At 15% concentration, leucine has little influence on particle morphology; this may be the reason that this concentration of leucine showed no improvement in the aerosolization of spray dried MD powders in an earlier study examining multiple amino acid additives.41 At higher concentrations, leucine appears to have a dominating influence on the surface of our particles, as evident by its crystalline nature now causing shrinkage of this outer shell and the appearance of wrinkling.12,19,48 The wider particle size distributions for 30% and 50% LL-MD particles reported in the previous figure (Fig. 1) was due a higher D50 and D90 resulting from the entrapment/bridging of smaller particles inside the crevices of larger wrinkled particles (seen in Fig. 2C). We are seeing a trade-off in properties resulting from the higher leucine concentration in the otherwise well-established MD matrix,37,38,49 with the resulting larger particles being generally undesired for therapeutics needing deep-lung deposition versus the increased wrinkling which favors improved aerosolization.14,21,23,24,50
3.1.3. Surface enrichment of leucine on surface.
To quantify enrichment of leucine at the surface of the increasingly wrinkled powders, particles were analyzed using X-ray photoelectron spectroscopy (XPS). Since leucine is the only compound in the formulation containing nitrogen, it was chosen as the distinguishing element among the different formulations to ascertain a trend for surface enrichments. The mass of nitrogen from XPS was expected to be a direct indication of enrichment of leucine on the surface. Fig. 3 shows the mass fraction (%) of elements by XPS for the surface of our spray dried powders. The MD control showed no nitrogen content, as expected, and a relatively high oxygen content compared to the other samples.
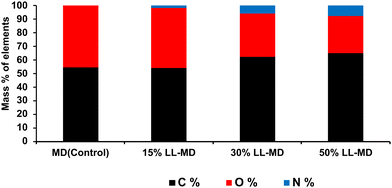 |
| Fig. 3 Elemental surface composition represented as mass percentage of individual elements (carbon C12, oxygen O16, and nitrogen N14) of dried particles analyzed using XPS. | |
For the other samples, the measured mass fraction of nitrogen atoms on the surface of particles was 1.5%, 5.8%, and 7.8% for the 15%LL-MD, 30%LL-MD, and 50%LL-MD, respectively. The N concentration on the particle surface increased almost linearly (R2 = 0.95) as a function of leucine concentration in the formulation, and by 50% leucine addition, the surface was mostly but not completely comprised of leucine (pure, 11.9% nitrogen molar mass fraction); exclusive surface enrichment by leucine tends to be inhibited by polysaccharides like dextran.19 It is therefore satisfactory to conclude that the wrinkled morphology was directly related to leucine enrichment on the surface. XPS is sensitive to a depth of 10 nm from the outer surface of particles (low standard errors ranging from ±0.1 to 1%)51 and therefore, the measured nitrogen composition for 50% LL-MD was likely affected by the inclusion of broken particles. Since a low nitrogen content (<10%) was expected for the bulk of a particle's interior due leucine enrichment at the surface, the nitrogen content for 50% LL-MD was lower than expected.
3.1.4. Moisture content in spray dried powders.
Residual moisture content was found to decrease (but not to a significant degree) with increasing leucine concentration (Table 1). The residual moisture content ranged from 1.5% for both control and 15%LL-MD samples, to 1.1% for the highest leucine concentration (50%LL-MD). Since residual moisture is a known plasticizer for a formulation,37 the lack of change in residual moisture with leucine content indicated that the original thermal-stabilizing nature of the MD formulation immobilizing the viral vector was not likely impacted in this study.
Table 1 Aerosol properties (MMAD, FPF/AD, and AD) of spray dried MD powders containing different leucine concentrations
Sample name |
Residual moisture content (%) |
MMAD (μm) |
FPF/AD (%) |
AD (%) |
The standard deviation for moisture content (%) was ±0.2%. |
MD (Control) |
1.51 |
5.7 ± 0.6 |
24 ± 2 |
91 ± 1 |
15% LL-MD |
1.54 |
4.4 ± 0.8 |
29 ± 4 |
92 ± 2 |
30%LL-MD |
1.36 |
4.4 ± 0.4 |
30 ± 4 |
83 ± 2 |
50%-LL-MD |
1.06 |
4.2 ± 0.1 |
42 ± 5 |
80 ± 3 |
3.2. Effect of leucine concentration on aerosolization
Aerosolization performance (quantified by MMAD, FPF, and AD) of our spray dried samples was characterized using a Next Generation impactor (NGI). Table 1 shows the available dose (AD) values, which ranged from 80% to 91%, indicating that the chosen inhaler (ICOone®) was highly effective in delivering the powders. The AD for the control was comparable to 15%LL-MD; however, the AD decreased with further increase in leucine concentration (30%LL-MD and 50%LL-MD). The lower available dose for these higher leucine concentrations was attributed to the observed loss of fine powder sticking to the inhaler wall, the connector between the inhaler, and the stainless-steel inlet, during the measurement.
For effective delivery of dry powders, the deposition of powder particles in the respiratory tract must be significant47,52 and is governed by powder characteristics like particle size and shape, and density, which are all reflected in the aerodynamic diameter (MMAD). An important descriptor of the deposition site for powders in the respiratory airways is given by MMAD. For delivery and deposition of dry powder aerosols to the peripheral airways and deep lungs, an optimal MMAD in the range between 2–3 μm is expected.10,53Table 1 shows the MMAD values, which were found to decrease with leucine compared to the control. However, these MMAD values are similar, and all cases were considered adequate for lung deposition at the upper airways and bronchiolar regions (1–5 μm).10,18 Furthermore, the MMAD values for the samples with 30% or 50% leucine were low compared to their corresponding mean geometric particle sizes (Fig. 1), which is attributed to these spray dried particles having lower densities due to the hollowness seen in the SEM images (Fig. 2) as well as by others.54
While MMAD implies how deeply into the lungs that a particle may travel, another relevant descriptor for researchers describing the potential for powder aerosolization is FPF, which broadly forecasts the amount of inhalable powder. FPF is defined as the percentage of particles below 5 μm but on account of mass losses between the inhaler and NGI. Our measured FPF value were normalized based on AD to make a true comparison on the influence of leucine concentration on particle flow. Table 1 shows FPF normalized by the available dose (FPF/AD, %) where a higher value is desired. The aerosolization of powders containing 15% leucine and 30% leucine was not significantly different from the control (24% FPF/AD). Only at the highest leucine concentration used (50%LL-MD sample) was the FPF/AD notably higher (42%). It appears that only by excessive roughening of these particles was aerosolization enhanced relative to the control, though it was noted that our samples showed a range of FPF values comparable to the FPF (lung dose) of various dry powders from some commercially available DPI devices;55,56 and compared to other studies aerosolization studies for spray dried powders containing leucine.57 We believe the improved FPF (>40%) of the 50%LL-MD sample was primarily attributed to reduced particle aggregation by the weaker interparticle forces between wrinkled particles compared to spherical particles.22,50,58 In addition, with a higher FPF, the powder contains higher amounts of respirable particles allowing greater lung deposition due to less dependency on inhaler flow.
While FPF describes the general potential of a powder for inhalation, the more important consideration for a dry powder viral vaccine is appropriate deposition at the targeted areas of a lung, which can be inferred from the mass fraction deposited on each stage of the NGI. The mass percentages of powder on each stage are shown in Fig. 4, indicating that anticipated deposition of particles in the central and peripheral airways (stage 2 to stage 6) will be similar for all formulations except 50% LL-MD, which showed a significantly higher cumulative mass percentage deposited on stages 2 and 3 (p < 0.05).
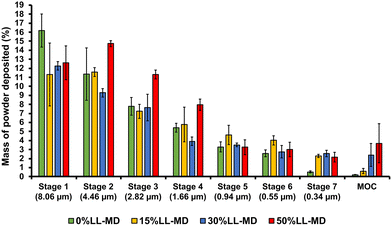 |
| Fig. 4 The mass percentage of powder deposited on each stage of the next generation impactor normalized by total mass deposited in the impactor for each measurement. Four spray dried samples containing various leucine concentrations were compared for distribution of powder on each stage of the impactor, error bars represent the standard error. (Abbreviation: MOC – micro-orifice collector). | |
The deposition of the vaccine in the upper respiratory airways (modelled by impactor stages 1, 2, and 3) is desired for some diseases, like influenza; however, for a TB vaccine seeking deep-lung deposition, these results with 50% LL-MD should be less favorable to inducing a high mucosal immunogenic response.5,7,8 For maximum immunogenic response upon inhalation, any of our formulations would be acceptable, though the three containing leucine were better for reaching the furthest areas, as noted by their higher mass fractions on the lower stages (stage 5 to 7).
3.3. Viral activity of AdHu5 in spray dried powders
The retained potency of the viral vector after spray drying and storage as a powder is paramount for this emerging class of thermally stable vaccines, before even beginning to contemplate new delivery modes like inhalation. The in vitro activity of the dry powder viral vector vaccines was estimated by the ability to infect lung cells viably.38 The titer log loss in viral activity in spray dried powders with increasing concentration of leucine is shown in Fig. 5. The activity losses were notably higher than normal since the spray drying conditions were selected to produce particles in the respirable size for all formulations without optimization for each chemistry. For reference, we considered activity losses between 0.5–1.0 log loss as ideal, while 1–2 log loss was considered acceptable since our experiences have shown that the trends seen remain similar to those optimized as ideal powders, whereas greater than 2 log loss was considered unacceptable.37,38,41
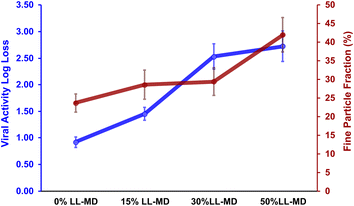 |
| Fig. 5 Log loss of viral activity of AdHu5 after infecting A549 cells with spray dried samples containing various leucine concentrations ranging from 0% to 50% leucine with mannitol dextran. | |
As seen in Fig. 5, while the fine particle fraction increased, the viral activity log loss also increased with increasing leucine concentration (R2 = 0.94, linearized fit). The activity loss for the control (0.9 log loss) was in the ideal range whereas the activity losses with 15% LL-MD were acceptable but still reflected a notable decline in activity (1.4 log loss). The reported losses with 30% LL-MD and 50% LL-MD (2.5 log loss and 2.7 log loss, respectively) indicated that they would have poorer effectiveness as compared to the control. Leucine tends to crystallize and could increase the chances of interior void formation in spray dried particles that introduce the virus to hidden, undesirable air interfaces.59,60 As a result, predicting the effect of crystallization on the small amount of viral particles present in the produced powders is not straightforward, especially since we do not believe that these crystals are inherently damaging to the virus directly. We have not completely ruled out the possibility that the activity losses observed in this study could be partially due to leucine crystallization. However, this does not affect the primary objective of this study, which was to suggest caution in the use of this well known aerosolization enhancer (leucine) in the presence of a biologic like the adenovirus.
The correlation of activity loss with leucine concentration was strongly evident in this analysis but also for the same formulations when tested with the lung cells without spray drying first. Even prior to spray drying, increasing the concentration of leucine produced a decline in viral activity; this decline was thus attributed to an interaction with the virus since leucine itself showed no cytotoxicity towards the cells (data not shown). Multiple potential degradation sites exist for large complex molecules, like biologics, rendering them prone to loss of therapeutic efficacy as their structures change.34 The most common mechanism for degradation and loss of virus activity is molecular aggregation, making it the primary focus of our subsequent testing to explain the decline of viral activity with additions of leucine.33,34
3.4. Effect of leucine concentrations on viral aggregation
It was hypothesized that viral aggregates were being formed in the feed solution prior to spray drying due to presence of leucine and this led to the increased activity losses found by in vitro testing. The presence of viral aggregates in formulations cause damage to the viral capsid fibers during atomization, as suggested in a previous study.35 Attempting to validate this hypothesis of viral aggregation prior to spray drying, we looked for evidence of aggregated AdHu5 in the liquid formulation with the highest leucine concentration (50%LL-MD) and compared our findings with the control liquid formulation (MD only), and a positive control (containing only PBS and stock virus with no spray drying excipients). Particle size analysis was obtained for these samples using the qViro-X technique, with distributions shown in Fig. 6A. The positive control contained particles with a mean diameter of 90 ± 10 nm, consistent with the range of adenovirus particle sizes reported previously35,61–63 and matched the near non-existence of aggregates with previous findings.64 The mean particle diameter of the virus in the presence of the excipients of mannitol and dextran was slightly higher, 95 ± 10 nm and significantly larger (p < 0.05, t-test) for 50%LL-MD, 100 ± 20 nm. These mean values appear similar because they are swayed by the large number of non-aggregated viruses that exist in all samples, but the appearance of peaks at large diameters is clear for formulations with leucine. The cut-off particle size to consider something an aggregate was taken to be 129 nm, which is the smallest adenovirus dimer size, calculated theoretically, Fig. 6B.35 Inclusion of leucine can be seen to lead to significant aggregation, with many more peaks above 129 nm seen in the size distribution, as high as 300 nm (Fig. 6A).
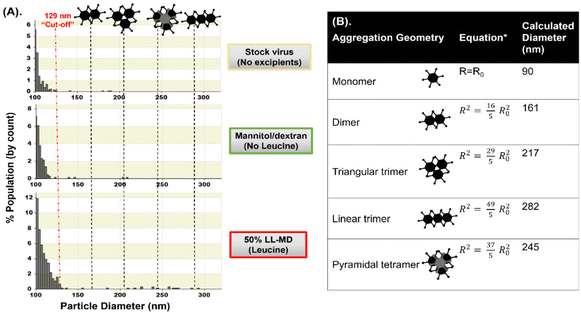 |
| Fig. 6 (A) Percentage particle count based on particle diameter comparing the aggregation (determined by the particle size) in various leucine concentrations containing liquid formulations. (B) Estimated particle size diameters for various viral aggregate geometries. (* Diameter was calculated based on the equations from ref. 35 and 65). | |
The geometries depicted in Fig. 6B are model estimates intended to give context to the aggregation results, calculated based on the work of McEvoy et al.;65 however, the actual aggregates will vary in size due to adenovirus size variability (ranging between 72 nm–105 nm), and these geometries do not describe all potential geometries present in the suspension. The shoulders seen in the particle size distribution presented in Fig. 6A were thought to be composed of dimers of adenovirus particles smaller than 90 nm in diameter or dimers of damaged particles without fiber proteins. Although the exact mechanism of activity loss due to the aggregation is not known, enhanced virus aggregation in the presence of increasing amount of leucine aligns with the decline in activity prior to spray drying. In addition to the elevated stresses associated with ionic strength and pH produced by high concentrations of leucine in the feed solution as causes for aggregation,66 the feasible electrostatic disruption of the capsid leading to denaturation could be a potential contributor. We believe that while there are multiple factors affecting the virus activity during spray drying, molecular aggregation is likely the dominant cause.
4. Conclusions
In this study, we examined the implications of leucine on the activity of large complex biologic, adenovirus, when added as an aerosolization enhancer for the formulation development of inhalable dry powder viral vaccines. Increased leucine concentration in the formulation enhanced the aerosolization performance relative to our control mannitol/dextran formulation, allowing us to achieve final powders with >40% FPF and low aerodynamic diameter of 4.2 μm. It is noteworthy that while aerosolization of spay dried adenovirus powders was enhanced, a noticeable decline in in vitro viral activity was observed with increasing leucine that could not be attributed to cytotoxicity. The dominant cause for this phenomenon was ultimately attributed to induced aggregation in the feed solution before spray drying, though other stress factors have not been ruled out until the exact mechanism for deactivation is found. Without ruling out factors like internal voids on activity loss, this work highlights one important factor to be considered by formulators – aggregation in presence of leucine. Despite the potential loss in activity, we conclude that use of leucine as aerosolization enhancer is worthwhile in preparing our dry powder vaccine for inhalation, with increased loading of the viral dosage being suggested to compensate for the loss in effective dose.
Data availability
Data for this article, including particle size, XPS, residual moisture content, aerosolization performance, viral activity and viral aggregation data are available at Open Science Framework (OSF) at https://osf.io/gdynu/?view_only=4f61db0293334b7e929c7264745c933d.
Author contributions
Varsha Singh: conceptualization, methodology, investigation, formal analysis, visualization, validation, writing – original draft preparation; Myrna Dolovich: resources, supervision, writing – reviewing and editing; Zhou Xing: resources, supervision, writing – reviewing and editing; Emily D. Cranston: resources, funding acquisition, conceptualization, supervision, writing – reviewing and editing; Michael R. Thompson: resources, funding acquisition, conceptualization, supervision, writing – reviewing and editing.
Conflicts of interest
The authors declare no conflict of interest.
Acknowledgements
The authors want to thank Xueya Feng for AdHu5 vector preparation, Dr Mayo James for TGA analysis, and Rod G. Rhem for his help in experiments related to the aerosol performance of the powders. We are grateful to Iconovo, Sweden, for donating the IcoOne inhalers. The authors also acknowledge Stephanie E. Barrett from Merck & Co. for her valuable advice. We acknowledge that XPS measurements were conducted at the Biointerfaces Institute of McMaster University, Canada, by Dr Zeynel Bayindir. We recognize his contribution to the data analysis and interpretation. The authors would also like to thank the Canadian Centre for Electron Microscopy (CCEM) at the Faculty of Health Sciences for the use of their electron microscopy facilities. This study is supported by funds from the Canadian Institutes of Health Research and the Natural Sciences and Engineering Research Council of Canada.
References
- F. A. W. Verreck, E. Z. Tchilian, R. A. W. Vervenne, C. C. Sombroek, I. Kondova, O. A. Eissen, V. Sommandas, N. M. van der Werff, E. Verschoor, G. Braskamp, J. Bakker, J. A. M. Langermans, P. J. Heidt, T. H. M. Ottenhoff, K. W. van Kralingen, A. W. Thomas, P. C. L. Beverley and C. H. M. Kocken, Variable BCG Efficacy in Rhesus Populations: Pulmonary BCG Provides Protection Where Standard Intra-Dermal Vaccination Fails, Tuberculosis, 2017, 104, 46–57, DOI:10.1016/J.TUBE.2017.02.003.
- N. Aguilo, S. Alvarez-Arguedas, S. Uranga, D. Marinova, M. Monzón, J. Badiola and C. Martin, Pulmonary but Not Subcutaneous Delivery of BCG Vaccine Confers Protection to Tuberculosis-Susceptible Mice by an Interleukin 17-Dependent Mechanism, J. Infect. Dis., 2016, 213(5), 831–839, DOI:10.1093/infdis/jiv503.
- S. Afkhami, Y. Yao and Z. Xing, Methods and Clinical Development of Adenovirus-Vectored Vaccines against Mucosal Pathogens, Mol. Ther.–Methods Clin. Dev., 2016, 3, 16030, DOI:10.1038/mtm.2016.30.
- M. Jeyanathan, Z. Shao, X. Yu, R. Harkness, R. Jiang, J. Li, Z. Xing and T. Zhu, AdHu5Ag85A Respiratory Mucosal Boost Immunization Enhances Protection against Pulmonary Tuberculosis in BCG-Primed Non-Human Primates, PLoS One, 2015, 10(8), e0135009, DOI:10.1371/JOURNAL.PONE.0135009.
- V. Jeyananthan, S. Afkhami, M. R. D’Agostino, A. Zganiacz, X. Feng, M. S. Miller, M. Jeyanathan, M. R. Thompson and Z. Xing, Differential Biodistribution of Adenoviral-Vectored Vaccine Following Intranasal and Endotracheal Deliveries Leads to Different Immune Outcomes, Front. Immunol., 2022, 13, 860399, DOI:10.3389/fimmu.2022.860399.
- R. L. De Swart, R. D. De Vries, L. J. Rennick, G. Van Amerongen, S. McQuaid, R. J. Verburgh, S. Yüksel, A. De Jong, K. Lemon, D. T. Nguyen, M. Ludlow, A. D. M. E. Osterhaus and W. P. Duprex, Needle-Free Delivery of Measles Virus Vaccine to the Lower Respiratory Tract of Non-Human Primates Elicits Optimal Immunity and Protection, npj Vaccines, 2017, 2(1), 1–11, DOI:10.1038/s41541-017-0022-8.
- A. Minne, J. Louahed, S. Mehauden, B. Baras, J. C. Renauld and R. Vanbever, The Delivery Site of a Monovalent Influenza Vaccine within the Respiratory Tract Impacts on the Immune Response, Immunology, 2007, 122(3), 316, DOI:10.1111/J.1365-2567.2007.02641.X.
- J. Todoroff, B. Ucakar, M. Inglese, S. Vandermarliere, C. Fillee, J. C. Renauld, K. Huygen and R. Vanbever, Targeting the Deep Lungs, Poloxamer 407 and a CpG Oligonucleotide Optimize Immune Responses to Mycobacterium Tuberculosis Antigen 85A Following Pulmonary Delivery, Eur. J. Pharm. Biopharm., 2013, 84(1), 40–48, DOI:10.1016/J.EJPB.2012.11.020.
- T. Sou, E. N. Meeusen, M. de Veer, D. A. V. Morton, L. M. Kaminskas and M. P. McIntosh, New Developments in Dry Powder Pulmonary Vaccine Delivery, Trends Biotechnol., 2011, 29(4), 191–198, DOI:10.1016/j.tibtech.2010.12.009.
- M. Y. Yang, J. G. Y. Chan and H. K. Chan, Pulmonary Drug Delivery by Powder Aerosols, J. Controlled Release, 2014, 193, 228–240, DOI:10.1016/j.jconrel.2014.04.055.
- C. J. Musante, J. D. Schroeter, J. A. Rosati, T. M. Crowder, A. J. Hickey and T. B. Martonen, Factors Affecting the Deposition of Inhaled Porous Drug Particles, J. Pharm. Sci., 2002, 91(7), 1590–1600, DOI:10.1002/JPS.10152.
- L. Li, S. Sun, T. Parumasivam, J. A. Denman, T. Gengenbach, P. Tang, S. Mao and H. K. Chan, L-Leucine, as an Excipient against Moisture on in Vitro Aerosolization Performances of Highly Hygroscopic Spray-Dried Powders, Eur. J. Pharm. Biopharm., 2016, 102, 132–141, DOI:10.1016/j.ejpb.2016.02.010.
- Q. T. Zhou, P. Tang, S. S. Y. Leung, J. G. Y. Chan and H. K. Chan, Emerging Inhalation Aerosol Devices and Strategies: Where Are We Headed?, Adv. Drug Delivery Rev., 2014, 75, 3–17, DOI:10.1016/J.ADDR.2014.03.006.
- T. Sou, L. M. Kaminskas, T. H. Nguyen, R. Carlberg, M. P. McIntosh and D. A. V. Morton, The Effect of Amino Acid Excipients on Morphology and Solid-State Properties of Multi-Component Spray-Dried Formulations for Pulmonary Delivery of Biomacromolecules, Eur. J. Pharm. Biopharm., 2013, 83(2), 234–243, DOI:10.1016/j.ejpb.2012.10.015.
- K. B. Preston and T. W. Randolph, Stability of Lyophilized and Spray Dried Vaccine Formulations, Adv. Drug Delivery Rev., 2021, 171, 50–61, DOI:10.1016/j.addr.2021.01.016.
- A. Minne, H. Boireau, M. J. Horta and R. Vanbever, Optimization of the Aerosolization Properties of an Inhalation Dry Powder Based on Selection of Excipients, Eur. J. Pharm. Biopharm., 2008, 70(3), 839–844, DOI:10.1016/j.ejpb.2008.06.013.
- A. Lechanteur and B. Evrard, Influence of Composition and Spray-Drying Process Parameters on Carrier-Free DPI Properties and Behaviors in the Lung: A Review, Pharmaceutics, 2020, 12(1), 55, DOI:10.3390/pharmaceutics12010055.
- B. Chaurasiya and Y. Y. Zhao, Dry Powder for Pulmonary Delivery: A Comprehensive Review, Pharmaceutics, 2021, 13(1), 1–28, DOI:10.3390/pharmaceutics13010031.
- Y. Xu, L. Harinck, A. G. Lokras, P. Gerde, E. Selg, C.-O. Sjöberg, H. Franzyk, A. Thakur and C. Foged, Leucine Improves the Aerosol Performance of Dry Powder Inhaler Formulations of SiRNA-Loaded Nanoparticles, Int. J. Pharm., 2022, 621, 121758, DOI:10.1016/J.IJPHARM.2022.121758.
- H. Y. Li, H. Neill, R. Innocent, P. Seville, I. Williamson and J. C. Birchall, Enhanced Dispersibility and Deposition of Spray-Dried Powders for Pulmonary Gene Therapy, J. Drug Targeting, 2003, 11(7), 425–432, DOI:10.1080/10611860410001659786.
- Z. Wang, H. Wang and R. Vehring, Leucine Enhances the Dispersibility of Trehalose-Containing Spray-Dried Powders on Exposure to a High-Humidity Environment, Int. J. Pharm., 2021, 601, 120561, DOI:10.1016/j.ijpharm.2021.120561.
- S. Mangal, F. Meiser, G. Tan, T. Gengenbach, J. Denman, M. R. Rowles, I. Larson and D. A. V. Morton, Relationship between Surface Concentration of L-Leucine and Bulk Powder Properties in Spray Dried Formulations, Eur. J. Pharm. Biopharm., 2015, 94, 160–169, DOI:10.1016/j.ejpb.2015.04.035.
- D. Lechuga-Ballesteros, C. Charan, C. L. M. Stults, C. L. Stevenson, D. P. Miller, R. Vehring, V. Tep and M. C. Kuo, Trileucine Improves Aerosol Performance and Stability of Spray-Dried Powders for Inhalation, J. Pharm. Sci., 2008, 97(1), 287–302, DOI:10.1002/jps.21078.
-
M.-C. Kuo, D. Lechuga-Ballesteros and S. Clara, Dry Powder Compositions Having Improved DispersivityUS Pat., US6518239B1, 2003 Search PubMed.
- W. Kaialy, A. Alhalaweh, S. P. Velaga and A. Nokhodchi, Influence of Lactose Carrier Particle Size on the Aerosol Performance of Budesonide from a Dry Powder Inhaler, Powder Technol., 2012, 227, 74–85, DOI:10.1016/j.powtec.2012.03.006.
- H. Y. Li, P. C. Seville, I. J. Williamson and J. C. Birchall, The Use of Amino Acids to Enhance the Aerosolisation of Spray-Dried Powders for Pulmonary Gene Therapy, J. Gene Med., 2005, 7(3), 343–353, DOI:10.1002/jgm.654.
- P. C. Seville, T. P. Learoyd, H. Y. Li, I. J. Williamson and J. C. Birchall, Amino Acid-Modified Spray-Dried Powders with Enhanced Aerosolisation Properties for Pulmonary Drug Delivery, Powder Technol., 2007, 178(1), 40–50, DOI:10.1016/j.powtec.2007.03.046.
- N. Y. K. Chew, B. Y. Shekunov, H. H. Y. Tong, A. H. L. Chow, C. Savage, J. Wu and H. K. Chan, Effect of Amino Acids on the Dispersion of Disodium Cromoglycate Powders, J. Pharm. Sci., 2005, 94(10), 2289–2300, DOI:10.1002/jps.20426.
- H. Faghihi, A. Vatanara, A. R. Najafabadi, V. Ramezani and K. Gilani, The Use of Amino Acids to Prepare Physically and Conformationally Stable Spray-Dried IgG with Enhanced Aerosol Performance, Int. J. Pharm., 2014, 466(1–2), 163–171, DOI:10.1016/j.ijpharm.2014.03.020.
- S. Focaroli, P. T. Mah, J. E. Hastedt, I. Gitlin, S. Oscarson, J. V. Fahy and A. M. Healy, A Design of Experiment (DoE) Approach to Optimise Spray Drying Process Conditions for the Production of Trehalose/Leucine Formulations with Application in Pulmonary Delivery, Int. J. Pharm., 2019, 562, 228–240, DOI:10.1016/j.ijpharm.2019.03.004.
- T. Rattanupatam and T. Srichana, Budesonide Dry Powder for Inhalation: Effects of Leucine and Mannitol on the Efficiency of Delivery, Drug Delivery, 2014, 21(6), 397–405, DOI:10.3109/10717544.2013.868555.
- M. Y. T. Chow, Y. Qiu, F. F. K. Lo, H. H. S. Lin, H. K. Chan, P. C. L. Kwok and J. K. W. Lam, Inhaled Powder Formulation of Naked SiRNA Using Spray Drying Technology with L-Leucine as Dispersion Enhancer, Int. J. Pharm., 2017, 530(1–2), 40–52, DOI:10.1016/J.IJPHARM.2017.07.013.
- W. Wang, S. Nema and D. Teagarden, Protein Aggregation—Pathways and Influencing Factors, Int. J. Pharm., 2010, 390(2), 89–99, DOI:10.1016/J.IJPHARM.2010.02.025.
- R. Y. K. Chang and H. K. Chan, Advancements in Particle Engineering for Inhalation Delivery of Small Molecules and Biotherapeutics, Pharm. Res., 2022, 39(12), 3047–3061, DOI:10.1007/S11095-022-03363-2.
- B. Morgan, M. Manser, M. Jeyanathan, Z. Xing, E. D. Cranston and M. R. Thompson, Effect of Shear Stresses on Adenovirus Activity and Aggregation during Atomization To Produce Thermally Stable Vaccines by Spray Drying, ACS Biomater. Sci. Eng., 2020, 6(7), 4304–4313, DOI:10.1021/acsbiomaterials.0c00317.
- P. T. Mah, P. O'Connell, S. Focaroli, R. Lundy, T. F. O'Mahony, J. E. Hastedt, I. Gitlin, S. Oscarson, J. V. Fahy and A. M. Healy, The Use of Hydrophobic Amino Acids in Protecting Spray Dried Trehalose Formulations against Moisture-Induced Changes, Eur. J. Pharm. Biopharm., 2019, 144, 139–153, DOI:10.1016/J.EJPB.2019.09.014.
- D. A. Leclair, E. D. Cranston, Z. Xing and M. R. Thompson, Evaluation of Excipients for Enhanced Thermal Stabilization of a Human Type 5 Adenoviral Vector through Spray Drying, Int. J. Pharm., 2016, 506(1–2), 289–301, DOI:10.1016/J.IJPHARM.2016.04.067.
- S. Afkhami, D. A. LeClair, S. Haddadi, R. Lai, S. P. Toniolo, H. C. Ertl, E. D. Cranston, M. R. Thompson and Z. Xing, Spray Dried Human and Chimpanzee Adenoviral-Vectored Vaccines Are Thermally Stable and Immunogenic in Vivo, Vaccine, 2017, 35(22), 2916–2924, DOI:10.1016/j.vaccine.2017.04.026.
- S. P. Toniolo, S. Afkhami, A. Mahmood, C. Fradin, B. D. Lichty, M. S. Miller, Z. Xing, E. D. Cranston and M. R. Thompson, Excipient Selection for Thermally Stable Enveloped and Non-Enveloped Viral Vaccine Platforms in Dry Powders, Int. J. Pharm., 2019, 561, 66–73, DOI:10.1016/j.ijpharm.2019.02.035.
- B. Morgan, Z. Xing, E. D. Cranston and M. R. Thompson, Acoustic Levitation as a Screening Method for Excipient Selection in the Development of Dry Powder Vaccines, Int. J. Pharm., 2019, 563, 71–78, DOI:10.1016/j.ijpharm.2019.03.026.
- V. Singh, Y. J. Son, M. Dolovich, Z. Xing, E. D. Cranston and M. R. Thompson, Screening Amino Acid Additives as Aerosolization Modifiers for Spray Dried Inhalable Viral-Vectored Vaccines, J. Drug Delivery Sci. Technol., 2022, 76, 103815, DOI:10.1016/j.jddst.2022.103815.
- J. Wang, L. Thorson, R. W. Stokes, M. Santosuosso, K. Huygen, A. Zganiacz, M. Hitt and Z. Xing, Single Mucosal, but Not Parenteral, Immunization with Recombinant Adenoviral-Based Vaccine Provides Potent Protection from Pulmonary Tuberculosis, J. Immunol., 2004, 173(10), 6357–6365, DOI:10.4049/jimmunol.173.10.6357.
- D. A. LeClair, E. D. Cranston, Z. Xing and M. R. Thompson, Optimization of Spray Drying Conditions for Yield, Particle Size and Biological Activity of Thermally Stable Viral Vectors, Pharm. Res., 2016, 33(11), 2763–2776, DOI:10.1007/s11095-016-2003-4.
-
O. Lastow and L. Arvidsson, US Patent11058832; 2021, U. Single Dose Dry Powder Inhaler, 2021. https://patents.google.com/patent/US11058832B2/en (accessed 2022-01-29) Search PubMed.
- The United States Pharmacopeial Convention, Aerosols, Nasal Sprays, Metered-Dose Inhalers, and Dry Powder Inhalers, 2012, ch. 601.
- W. H. Finlay and C. Darquenne, Particle Size Distributions, J. Aerosol Med. Pulm. Drug Delivery, 2020, 178–180, DOI:10.1089/jamp.2020.29028.whf.
- N. R. Labiris and M. B. Dolovich, Pulmonary Drug Delivery. Part I: Physiological Factors Affecting Therapeutic Effectiveness of Aerosolized Medications, Br. J. Clin. Pharmacol., 2003, 56(6), 588–599, DOI:10.1046/j.1365-2125.2003.01892.x.
- A. L. Feng, M. A. Boraey, M. A. Gwin, P. R. Finlay, P. J. Kuehl and R. Vehring, Mechanistic Models Facilitate Efficient Development of Leucine Containing Microparticles for Pulmonary Drug Delivery, Int. J. Pharm., 2011, 409(1–2), 156–163, DOI:10.1016/j.ijpharm.2011.02.049.
-
D. A. Leclair and B. Eng, Thermally Stable Human Type 5 Adenovirus Through Spray Drying: Storage Efficacy and Process Optimization, 2016 (Doctoral Dissertation) Search PubMed.
- C. Weiler, M. Egen, M. Trunk and P. Langguth, Force Control and Powder Dispersibility of Spray Dried Particles for Inhalation, J. Pharm. Sci., 2010, 99(1), 303–316, DOI:10.1002/jps.21849.
- D. N. G. Krishna and J. Philip, Review on Surface-Characterization Applications of X-Ray Photoelectron Spectroscopy (XPS): Recent Developments and Challenges, Appl. Surf. Sci. Adv., 2022, 12, 100332, DOI:10.1016/J.APSADV.2022.100332.
- X. F. Yang, Y. Xu, D. S. Qu and H. Y. Li, The Influence of Amino Acids on Aztreonam Spray-Dried Powders for Inhalation, Asian J. Pharm. Sci., 2015, 10(6), 541–548, DOI:10.1016/j.ajps.2015.08.002.
- W. Glover, H. K. Chan, S. Eberl, E. Daviskas and J. Verschuer, Effect of Particle Size of Dry Powder Mannitol on the Lung Deposition in Healthy Volunteers, Int. J. Pharm., 2008, 349(1–2), 314–322, DOI:10.1016/j.ijpharm.2007.08.013.
- N. Alhajj, N. J. O'Reilly and H. Cathcart, Leucine as an Excipient in Spray Dried Powder for Inhalation, Drug Discovery Today, 2021, 26(10), 2384–2396, DOI:10.1016/j.drudis.2021.04.009.
- N. Islam and M. J. Cleary, Developing an Efficient and Reliable Dry Powder Inhaler for Pulmonary Drug Delivery – A Review for Multidisciplinary Researchers, Med. Eng. Phys., 2012, 34(4), 409–427, DOI:10.1016/J.MEDENGPHY.2011.12.025.
- L. Borgström, T. Bengtsson, E. Derom and R. Pauwels, Variability in Lung Deposition of Inhaled Drug, within and between Asthmatic Patients, with a PMDI and a Dry Powder Inhaler, Turbuhaler®, Int. J. Pharm., 2000, 193(2), 227–230, DOI:10.1016/S0378-5173(99)00341-5.
- M. Gomez, J. McCollum, H. Wang, M. Ordoubadi, C. Jar, N. B. Carrigy, D. Barona, I. Tetreau, M. Archer, A. Gerhardt, C. Press, C. B. Fox, R. M. Kramer and R. Vehring, Development of a Formulation Platform for a Spray-Dried, Inhalable Tuberculosis Vaccine Candidate, Int. J.
Pharm., 2021, 593, 120121, DOI:10.1016/j.ijpharm.2020.120121.
- J. Raula, F. Thielmann, M. Naderi, V. P. Lehto and E. I. Kauppinen, Investigations on Particle Surface Characteristics vs. Dispersion Behaviour of l-Leucine Coated Carrier-Free Inhalable Powders, Int. J. Pharm., 2010, 385(1–2), 79–85, DOI:10.1016/j.ijpharm.2009.10.036.
- V. Singh, B. A. Morgan, A. Schertel, M. Dolovich, Z. Xing, M. R. Thompson and E. D. Cranston, Internal Microstructure of Spray Dried Particles Affects Viral Vector Activity in Dry Vaccines, Int. J. Pharm., 2023, 640, 122988, DOI:10.1016/J.IJPHARM.2023.122988.
- Y. Chen, J. Ling, M. Li, Y. Su, K. S. Arte, T. T. Mutukuri, L. S. Taylor, E. J. Munson, E. M. Topp and Q. T. Zhou, Understanding the Impact of Protein-Excipient Interactions on Physical Stability of Spray-Dried Protein Solids, Mol. Pharm., 2021, 18(7), 2657–2668, DOI:10.1021/ACS.MOLPHARMACEUT.1C00189/SUPPL_FILE/MP1C00189_SI_001.PDF.
- M. A. Kennedy and R. J. Parks, Adenovirus Virion Stability and the Viral Genome: Size Matters, Mol. Ther., 2009, 17(10), 1664–1666, DOI:10.1038/MT.2009.202.
- R. Vogel, G. Willmott, D. Kozak, G. S. Roberts, W. Anderson, L. Groenewegen, B. Glossop, A. Barnett, A. Turner and M. Trau, Quantitative Sizing of Nano/Microparticles with a Tunable Elastomeric Pore Sensor, Anal. Chem., 2011, 83(9), 3499–3506, DOI:10.1021/AC200195N/SUPPL_FILE/AC200195N_SI_001.PDF.
- B. Morgan, E. Niinivaara, Z. Xing, M. R. Thompson and E. D. Cranston, Validation of a Diffusion-Based Single Droplet Drying Model for Encapsulation of a Viral-Vectored Vaccine Using an Acoustic Levitator, Int. J. Pharm., 2021, 605, 120806, DOI:10.1016/j.ijpharm.2021.120806.
- L. L. Bondoc and S. Fitzpatrick, Size Distribution Analysis of Recombinant Adenovirus Using Disc Centrifugation, J. Ind. Microbiol. Biotechnol., 1998, 20(6), 317–322, DOI:10.1038/SJ.JIM.2900529.
- M. Mcevoy, V. Razinkov, Z. Wei, J. R. Casas-Finet, G. I. Tous and M. A. Schenerman, Improved Particle Counting and Size Distribution Determination of Aggregated Virus Populations by Asymmetric Flow Field-Flow Fractionation and Multiangle Light Scattering Techniques, Biotechnol. Prog., 2011, 27(2), 547–554, DOI:10.1002/BTPR.499.
- J. Rexroad, R. K. Evans and C. R. Middaugh, Effect of PH and Ionic Strength on the Physical Stability of Adenovirus Type 5, J. Pharm. Sci., 2006, 95(2), 237–247, DOI:10.1002/jps.20496.
|
This journal is © The Royal Society of Chemistry 2024 |
Click here to see how this site uses Cookies. View our privacy policy here.