DOI:
10.1039/D4PM00010B
(Review Article)
RSC Pharm., 2024,
1, 204-217
Potential of ultrasonic processing in biomedical applications
Received
16th January 2024
, Accepted 20th April 2024
First published on 2nd May 2024
Abstract
Ultrasound waves are sound waves with frequencies higher than the human audible frequencies and application of these waves in biomedical science is explored in this article. A novel approach that involved the use of ultrasound was discovered in around 1950 and since then, it is experimented on to obtain various applications like gene/drug delivery, diagnosis, theranostics, tissue engineering, etc. Ultrasound waves are sound waves travelling at frequencies above human audible frequencies and are further classified into three types: high frequency, medium frequency and low frequency, each showing different therapeutic applications. Ultrasound has shown its application in various fields like dentistry, wastewater management, etc. Apart from therapeutic use, ultrasound is also implemented in synthesis, extraction, tissue engineering, gene delivery and many more applications. This article mentions the recent applications of ultrasound as a non-invasive route for the treatment of several diseases also due to its enhanced penetration of cells which helped greatly in the delivery of drugs/genes, in the extraction of various essential biological components from plants, in the synthesis of several compounds, in the field of theranostics – a combination of diagnosis and therapy, in tissue engineering, etc.
1 Introduction
Transportation of macromolecules (lipids, proteins, nucleic acids) and poorly soluble drugs (Griseofulvin, Acyclovir, Aceclofenac) or permeable drugs (Desmopressin, Vancomycin) poses a challenge for researchers in the development of active therapeutic systems. Various methods like electroporation, radiofrequency, ablation, increased vascular pressure, etc. are employed to enhance the solubility and permeability of actives.1 Sonophoresis can be considered as a novel method used for delivery of drugs since the 1950s.2 Sonophoresis is a non-invasive method that uses ultrasound for the delivery of drugs through thin and soft tissues. Ultrasound is a branch of acoustics that makes use of acoustic frequencies greater than the audible frequencies (≥16 kHz) and is further classified into three types: high frequency, medium frequency and low frequency (Fig. 1).
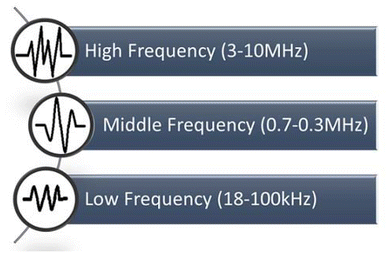 |
| Fig. 1 Classification of different ultrasound ranges. | |
In 1954, ultrasound was first applied in the delivery of hydrocortisone for the treatment of digital polyarthritis. Later, Mitragotri revealed the ideal frequency range of ultrasound (50–60 kHz) and suggested that along with the frequency, dispersion also increases.3 Similarly, Cameron used ultrasound for the delivery of Carbocaine in the treatment of Colle's fracture. Sonophoresis was tested for the penetration of several drugs like hydrocortisone, local anesthetics, etc. mainly for localized action.4 For the delivery of corticosteroids, initially high frequency ultrasound (1–3 MHz) was used for their local action.1 Low/medium ultrasound ranges are typically used in drug delivery, and high frequency ultrasound is used in the field of physiotherapy, imaging, kidney stone and gallstone pulverization, etc., along with drug delivery applications.5
Ultrasound is generated by using either a piezoelectric method or a magnetostrictive method as shown in Fig. 2 and 3.
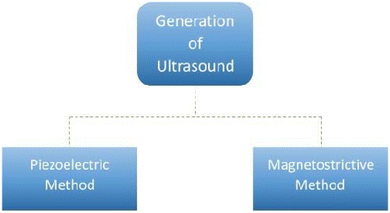 |
| Fig. 2 Different ways for the generation of ultrasound. | |
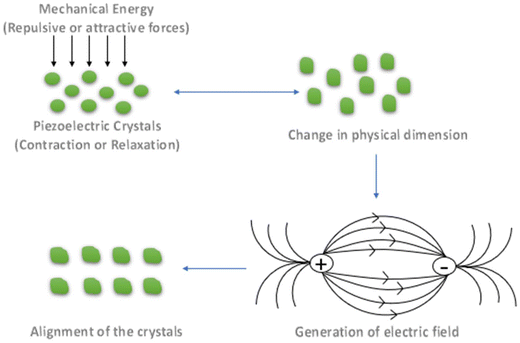 |
| Fig. 3 Mechanism for the generation of ultrasound by the piezoelectric method. | |
Ultrasound is generated either by the vibration of piezoelectric crystals or in the presence of an alternating current that is passed through a material such as quartz or a polycrystalline substance like lead-zirconate-titanium or barium titanate.4 The effects of ultrasound on biological tissues can be classified into: (1) thermal effects (on exposure to ultrasound there is an increase in temperature) and (2) cavitational effects (formation of gaseous cavities) and (3) acoustic streaming effects4 (Fig. 4).
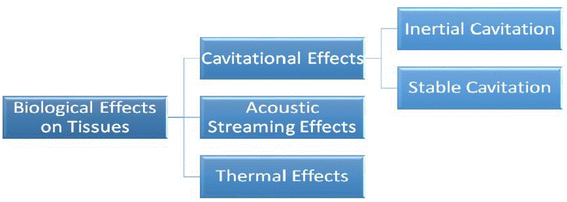 |
| Fig. 4 Biological effects of ultrasound on tissues. | |
In addition to the non-ionizing, non-invasive, and relatively inexpensive strategy, multiple advantages of ultrasound in the field of biomedicine are as follows:
• Non-viral delivery: ultrasound-mediated gene therapy enables delivery of non-viral vectors to provide reduced host immunogenicity response.
• Targeted drug delivery with therapeutic efficacy: effective personalized theranostic targeted therapy cross-linked with gene therapy, chemotherapeutics with minimized off-target effects.
• Permeability: the transient localized permeation ability of ultrasound enables enhanced drug delivery.
• Tissue regeneration: ultrasound mediated therapy results in significant tissue regeneration for diseases including vascular and bone tissue regeneration.6,7
2 Applications of ultrasonic processing
The two main reasons for the widespread applications of ultrasound are as follows: (1) it propagates slower than electromagnetic waves, providing sufficient time for the generation of information and (2) it has the ability to penetrate through opaque objects that helps in the diagnosis and imaging of internal organs with less attenuation at frequencies of several MHz. A non-thermal phenomenon is a recent mechanism used for drug delivery whose applications can be further classified into three categories: (1) helps in penetrating the agents through various tissues, (2) directly affects the membrane by changing the permeability or adsorption of the drug and (3) it can change the physical properties of the drug (like activation of the drug).8 The applications of ultrasound are explained further with relevant examples.
2.1 Synthesis
Ultrasound shows its application in the synthesis of various compounds especially metal oxides that are used extensively in various applications such as catalysts, adsorbents, semiconductors, antifungal agents, superconductors, etc. Synthesis of resveratrol liposomes by ultrasonic dispersing shows antioxidant and cardio-protective effects along with antineoplastic properties in the treatment of cancers like breast, colon, ovarian, etc. The p53 gene is considered one of the important targets for treating different cancers as almost 50% of cancers involves the mutation of the p53 gene. Combined delivery of resveratrol and p53 gene not only reduced the side effects of resveratrol but also increased the inhibitory effect of the p53 gene. Resveratrol was formulated as a liposome, prepared by the ultrasonic dispersing method.9 The ultrasonic-assisted emulsion (o/w) method was found to be effective in the formation of amidoxime functionalized silica (SiO2-AO). This functionalized complex was utilized for its easy and efficient removal of uranium, a toxic pollutant from water bodies, by the adsorption method.10 Ultrasonic irradiation was also applied in the synthesis of MnO2 nanoparticles implementing the mechanism of cavitation for the synthesis that makes use of physical or chemical processes. The physical process of shock waves was utilized for the synthesis of nanomaterials since they help in increasing the collision between nanoparticles.11 Loratadine, an anti-histaminic drug, helps in treating allergic disorders, is a BCS Class 2 drug showing poor solubility. Therefore, to increase the solubility it was formulated as a nanoparticle system by using US-RESSAS (ultrasonic-assisted rapid expansion of supercritical solution into aqueous solution).12 Na batteries prepared by MnO2 have a stable structure, Na0.44MnO2 was synthesized by using the ultrasonic method as the conventional method involved the use of excessive heat treatment. Sonication was provided for 10 min with an ultrasonic frequency of 20 kHz and an intensity of 500 W.13 Alkylated pyridines (1-alkyl 2 pyrrolidone and 2-alkyl 2 pyrrolidone) were prepared by green chemistry synthesis and evaluated as intermediates in the synthesis of several biologically active compounds. 91–99% yield was obtained by using ultrasound for the synthesis for 10 min whereas, longer chain pyrrolidones showed antifungal activity.14 PEG stearate, a condensate product, synthesized by the ultrasound-driven esterification process using Candida antarctica Lipase B as a catalyst helps in reducing the high temperature and use of a solvent in the conventional synthesis of the condensate. Here, the condensate was prepared with an operating frequency of 22–40 kHz with a power output of 200 W. About 84.34% conversion into the condensate was obtained by using this method and the product was confirmed by using FTIR and 1H NMR spectroscopy studies.15 Polyethylene terephthalate is widely used in hospitals and medical institutes due to its thermal stability, reusability, cost-effectiveness, etc. but is susceptible to microbial infections. As a result, antimicrobial Ag2O-loaded PET fabrics were prepared by an ultrasound method to avoid nosocomial infection. The ultrasonic apparatus was run at 47 kHz frequency at 120 W power for 1 h for the synthesis of Ag2O-loaded PET fabrics showing an improvement in the anti-bacterial activity.16 Antibacterial fibers for packaging purposes were prepared using polyvinyl alcohol by D-limonene fibers. These fibers were prepared by using an ultrasonic method with a frequency of 40 Hz and a power 50 W for 15 min and the fibers obtained along with good antibacterial activity showed reduction in oxygen permeability and also improvement in mechanical properties.17 Tribological materials have emerged to reduce the total consumption of energy and among them, tungsten disulfide synthesized using ultrasound shows promising applications due to chemical stability, resistance to degradation, non-toxic and inert properties.18 A polymeric semiconductor, graphitic carbon nitride, is utilized as a photocatalyst to remove pollutants such as chromium and dyes (RhB) from water. The semiconductor was synthesized by using ultrasound for 30 min while the polymer showed better adsorption capacity due to a higher surface area and led to the reduction of chromium and oxidation of RhB.19 The catalytic properties of metal oxides such as manganese oxide and tin dioxide are affected by the method of preparation, use of ultrasound for the preparation and avoidance of exhaustion of energy and chemicals.20 MnO2 nanostructured thin films were formulated for the storage of renewable energy by using an ultrasound-assisted electrodeposition method which helped in improving stability, enhanced electrochemical activity along with low resistance were the characteristic features of these films.21 Polyacrylamide/bentonite hydrogel nanocomposites, formulated using ultrasound, help in reducing pollutants in water due to their adsorption capacity ascribed to higher surface area, physical and chemical stability, etc. and consequently enhanced adsorption capacity.22 Hollow nanostructures of catalysts such as nickel and nickel oxides were synthesized by the ultrasonic spray pyrolysis method using 1.7 MHz ultrasonic frequency.23 In the synthesis of zinc oxide (ZnO) nanoparticles, ultrasound was used to eliminate the use of solvents and heat treatment required during the synthesis process.24 ZnO and ZnO–ZrO2 nanocomposites synthesized using ultrasound-assisted wet chemical methods were utilized due to their enhanced humidity sensing properties. Ultrasound eliminates the aggregation of large-sized particles produced by the wet chemical method.25 LiFePO4 (LFP) nanoparticles prepared in an Impinging Jet Reactor (IJR) by using a low ultrasonic frequency of 20 kHz for intensifying micromixing would help in the controlled synthesis of nanoparticles.26 Lead ion-imprinted polymers (Pb IIPs) for removal of metal ions from the air, water, soil, etc. were synthesized by using the ultrasound-assisted precipitation method. These IIPs show an advantage over adsorbents such as long-term storage without loss of activity, chemical and thermal stability and easy manufacturing as ultrasonic energy was provided for a duration of 5 h in the synthesis that helped in the reduction of reaction time.27 Silica nanoparticles were synthesized using ultrasound to reduce the synthesis reaction time and were coated with titanium dioxide.28 Synthesis of nanorods containing zinc oxide,29 carbon nanotubes containing iron oxide for removal of toxins,30 and copper oxide nanoflakes for reducing water pollution caused due to hydroquinone31 are some of the other examples of application of ultrasound in the synthesis process. The applications of ultrasound in the synthesis of various substances are summarized in Table 1.
Table 1 Parameters and methods of preparation of various formulations
Sr. No. |
Formulation |
Parameter |
Method of preparation |
Ref. |
1. |
Nanocomposites (Ag/ZnO) |
Frequency-2.5 MHz |
Ultrasonic spray pyrolysis |
32
|
2. |
Nanorods (ZnO) |
Frequency-40 kHz |
Ultrasonic pyrolysis |
29
|
Temperature-350 °C |
Spray rate-30 mL h−1 |
3. |
Nanoparticle (MnO2) |
Frequency-24 kHz |
Ultrasonic irradiation |
11
|
Power-200 W |
4. |
Multi-walled carbon nanotubes (Iron oxide) |
Frequency-20 kHz |
Green chemistry |
30
|
5. |
Nanoparticle (Loratadine) |
Frequency-20 kHz |
Ultrasonic-assisted rapid expansion of supercritical solution into an aqueous solution |
12
|
Intensity-70 W L−1 |
6. |
Tungsten disulphide powder |
Frequency-1.7 MHz |
Ultrasonic pyrolysis method |
18
|
Time-10 min |
7. |
Graphitic carbon nitride nanocomposites |
Frequency-50 Hz |
Ultrasonic irradiation |
33
|
Power-100 W |
8. |
Mesoporous MnO2/SO2 nanomaterials |
Frequency-30 kHz |
Ultrasonic-assisted Co-precipitation Method |
20
|
Power-50 W |
9. |
MnO2 nanostructured thin films |
Frequency-45 kHz |
Ultrasound assisted-electrodeposition method |
21
|
Power-300 W |
Time-6 h |
10. |
Polyacrylamide/bentonite hydrogel nanocomposites |
Frequency-30 kHz |
Ultrasonic irradiation |
22
|
Time-30 min |
11. |
Copper oxide nanoflakes |
Frequency-20 kHz |
Ultrasonic irradiation |
31
|
Power-100 W |
12. |
Zinc oxide nanoparticles |
Frequency-40 kHz |
Ultrasound-assisted precipitation method |
24
|
13. |
ZnO and ZnO–ZrO2 nanocomposites |
Frequency-35 kHz |
Ultrasonic assisted wet chemical method |
25
|
Power-60 W |
14. |
Fe3O4 magnetic nanoparticles |
Frequency-1.5 MHz |
Co-precipitation method |
26
|
Time-30 min |
15. |
Silica nanoparticles |
Frequency-20 kHz |
Ultrasonic irradiation |
28
|
Power-700 W |
16. |
Manganese nanocomposites |
Frequency-17.5–20 kHz |
Ultrasonic irradiation |
34
|
Power-1.5–4 kW |
17. |
Fabricated antimony sulpho-iodide nanowire composites |
Frequency-20 kHz |
Ultrasonic irradiation |
35
|
Intensity-565 W cm−2 |
2.2 Extraction
Application of ultrasound in the extraction process of various components like antioxidants,36 oils, etc., provides a green method and advantages when compared to traditional extraction methods such as reduction in the use of toxic solvents, low-temperature requirement, good yield, easy isolation of functional compounds, inexpensive, simple, economical and reduced extraction times without modification of the molecular structure and molar mass.37 Factors responsible for increasing the efficiency of extraction are disruption of cells and mass transfer.38 Extraction of natural dyes (tannins) from the bark of neem trees utilized in the dyeing of wool, cotton, etc. is one of the important applications. The extraction process involves 15–60 min of irradiation with ultrasound, however, an increase in isolation efficiency was reported upon the exposure to ultrasound for 45 min. Extraction efficiency increases when ultrasound helps in the extraction process as it uses less amount of powder for extraction.37 Extraction of thymol from Plectranthus amboinicus follows the cavitation mechanism wherein gas bubbles formed on the application of ultrasound were attached to the cells and later due to a change in pressure the bubbles collapsed that resulted in the rupture of the cell wall causing the components to diffuse into the solvent.38 Ultrasound was also employed for the extraction of oil from papaya seeds for antioxidant properties. Ultrasound application resulted in a reduced extraction time, the oil obtained showed oxidative stability and the amount of solvent used for extraction was also less.39 Extraction of tropane alkaloids from Radix physochlainae was carried out using optimum ultrasonic extraction parameters: 90 W for 30 min, which was based on the formation of hydrogen bonds via mass transfer by ionic liquids.40 Extraction of chemicals such as silica, lignocellulose, etc. from rice husk was established by ultrasound which in turn converted rice husk wastes into a valuable product and also made use of ionic liquids for efficient extraction.41Cassia fistula consists of Rhein, an anthraquinone, that acts as a laxative was extracted using ultrasound from the fruit pulp, wherein a yield twice the conventional decoction method was obtained from the UAE-optimized Central Composite Design (CCD).42 Polysaccharides from Trametes orientalis were also extracted using ultrasonic-microwave assisted extraction for hepatoprotection.43 Enzymatic extraction of β carotene from orange peel waste was performed using ultrasound (20 kHz, 500 W) as the pectinase enzyme results in increased penetration of the solvent into cell walls.44 Enzyme assisted extraction by using ultrasound was also used for the extraction of flavonoids from Cyclocarya paliurus for its antioxidant and antimicrobial activities. Ultrasound helped increase the mass transfer between the two phases, consequently increasing the efficiency of the process.45 Ultrasound-assisted extraction of polyphenols from Aegle marmelos is another application where ultrasound is used in the extraction process using eutectic solvents (a mixture of choline chloride and oxalic acid). An ultrasonic frequency of 50 kHz and a power of 100 W during the extraction resulted in an increase in the yield (greater than 60%) of polyphenol due to the formation of hydrogen bonds between the eutectic solvents used and the solute.46 Bioactive components present in Salvia miltiorrhiza are extracted by the application of ultrasound using a eutectic mixture. Extraction of phenolic compounds from lime peel waste is also performed with the assistance of ultrasound (4 min) wherein the method of extraction largely influenced the antioxidant properties.47 Extraction of lycopene (antioxidant) from tomato paste waste is yet another example of ultrasound-assisted extraction that was carried out in the presence of sunflower oil and mild temperature conditions48 Artemisinin was extracted using ultrasound from Artemisia annua for its application in the treatment of Malaria. Ultrasound exposure resulted in the rupture of the plant cell walls leading to the diffusion of the cell contents into the solvent. Optimum extraction parameters were found to be an ultrasonic power of 150 W for 30 min at a temperature of 30 °C.49 The paprika pigment was also extracted using ultrasound for its antioxidant activity, anti-tumor activity, etc. An ultrasonic power of 10 W for a duration of 600 s were the optimum parameters for the extraction process and the extraction time increased with the increase in the color value (final value 147) of paprika.50 Extraction of flavonoids from Propolis was conducted using ultrasound with the help of ethanol as the solvent exposing the process to an ultrasound frequency of 20 kHz for a duration of 15 min which resulted in the increase in the yield of extraction as well as an increase in the total phenolic content of the extract.51 Around 43% of proteins are obtained from Rock Lobster from Australia and these proteins are efficiently extracted by using ultrasound during extraction as it results in the formation of microcavities that help in the extraction process. The efficiency of extraction was increased using a co-precipitation agent, chitosan, which is used for the recovery of the proteins. 93% of protein recovery was obtained after using ultrasound for a duration of 5 min during the extraction process.52 Mucilage from Opuntia ficus was obtained by the ultrasound-assisted extraction process, wherein an ultrasonic frequency of 40 kHz was used during extraction for a duration of 30 min increasing the yield of the extract.53 Extraction of flavonoids from sweet potato leaves is also an application of the ultrasound-assisted extraction (40 kHz, 50 W) process but under the influence of microwaves and RSM was utilized for the optimization of this process.51 Extraction of cyaniding-3-o-galactoside from Aronia melanocarpa by using ultrasound is yet another example of ultrasound-assisted extraction to overcome the limitations such as high instability, heat sensitivity and damage during purification.54 Phosvitin (Pv) is extracted from egg yolk by using ultrasonic thermal assisted extraction (UTAE) for its antioxidant properties and its use resulted in the increase in protein recovery.55 Jackfruit peels contain phenols that are extracted by using ultrasound along with a microwave-assisted extraction technique to obtain a yield of 8.14 mg gallic acid equivalent after the extraction process.56 Ultrasound (240 W, 30 min) is employed for the extraction of polyphenols from rapeseed meal but results in the formation of free radicals causing a decrease in its antioxidant activity. To prevent radical formation, extraction was performed along with nitrogen (5 L min−1) which could be recycled making the process effective.57 Lipids obtained from microalgae chlorella are used as biodiesel by using alumina particles along with ultrasound (60–360 W, 15–60 min) for increasing the surface area further enhancing the generation of bubbles during the cavitation process.58 The extraction of bioactive components from Nepeta binaludensis was carried out for its antioxidant properties by using an ultrasound-assisted extraction process to obtain total phenolic content of about 402.6 mg mL−1.59 Antioxidants from the common bean Phaseolus vulgaris have been extracted using ultrasound (68 min, 480 W) for its anti-tumor, anti-mutagenicity, etc.60 Flavonoids are also extracted from Euonymus alatus with the help of ultrasound for extraction and by using an aqueous base consisting of PEG as its solvent system. Optimization of the extraction process was carried out using RSM while the time required for extraction was 15 min at a temperature 90 °C and an ultrasonic frequency of 20 MHz.61 Raspberries contain polyphenols like tannins and anthocyanins that are extracted for their antioxidant and anticarcinogenic properties using UTAE along with microwave resulting in an increase in the extraction efficiency. An ultrasonic frequency of 35 kHz for 120 min at a temperature of 40 °C was applied for the extraction along with microwave at a rate of 90 W min−1.62 Ultrasound is also employed for the extraction of micro traces of arsenic present in soil by using solid phase extraction along with the use of magnetic ion impregnated polymer. An ultrasonic frequency of 50 Hz with an ultrasonic power of 330 W for 50 min at 60 °C temperature was used.63 Extraction of ginsenosides from flower buds of Panax ginseng,64 pectin from pistachio65 and eggplant,66 phenolic compounds from Citrus aurantium,67 essential oils from clove,68 caffeine from guarana seeds69 and phenolic compounds from Punica granatum70 was also conducted under the influence of ultrasound. The ultrasonic applications in the extraction process and their yields are summarized in Table 2.
Table 2 Ultrasonic parameters used and yield obtained for various extraction processes
Sr. No. |
Purpose |
Parameter |
Yield |
Ref. |
1. |
Oil from Papaya seeds |
Power-250 W |
32.27% |
39
|
Time-20 min |
2. |
Thymol from Plectranthus amboinicus |
Frequency-20 kHz |
5.51% |
38
|
Power-130 W |
Time-23 min |
Temperature-55 °C |
3. |
Silica and Lignocellulose from Rice Husk |
Time-70 min |
40% |
41
|
4. |
Ginsenosides from Panax ginseng |
Frequency-40 kHz |
64.53% |
64
|
Power-250 W |
5. |
Psoralen, Isopsoralen, bavachin from Psoralea corylifolia |
Power-300 W |
28.4% |
71
|
Time-20 min |
6. |
Rhein from Cassia fistula |
Frequency-45 kHz |
14.98% |
42
|
7. |
Polysaccharides from Orchis chusua |
Power-390 W |
48.6% |
72
|
Temperature-60 °C |
Time-50 min |
8. |
Polysaccharides from Trametes orientalis |
Power-114 W |
7.52% |
43
|
Time-11 min |
9. |
Flavonoids from Cyclocarya paliurus |
Power-108.3 W |
34.24% |
45
|
Time-30 min |
10. |
Lycopene from Tomato paste waste |
Intensity-70 W m−2 |
74.58–87.25% |
48
|
Time-10 min |
11. |
Cyaniding-3-o-galactoside (C3G) from Aronia melanocarpa |
Frequency-75 kHz |
13.01 g |
54
|
Time-6 h |
Temperature-18.8 °C |
12. |
Pectin from Pistachio |
Power-150 W |
59.33% |
65
|
Time-30 min |
13. |
Pectin from Eggplant |
Power-50 W |
33.64 mg |
66
|
Time-30 min |
14. |
Phenols from Jackfruit peels |
Frequency-40 Hz |
8.14 mg |
56
|
Power-50 W |
15. |
Arabinoxylan |
Power-50 W |
27.78% |
73
|
Time-25 min |
16. |
Oil from Camellia sinensis |
Power-440 550 W |
31.52% |
74
|
Time-38 min |
17. |
Phenolic compounds from Citrus aurantium |
Frequency-40 kHz |
95.84 mg |
67
|
Power-100 W |
18. |
Polysaccharides from Mentha haplocalyx |
Power-300 W |
9.41% |
75
|
Time-28 min |
Temperature-70 °C |
19. |
Anthocyanins from Pyrus communis |
Power-162 W |
0.343 mg g−1 |
76
|
Time-11 min |
Temperature-71 °C |
20. |
Bioactive components from Nepeta binaludensis |
Temperature-25 °C |
10.93% |
59
|
Time-15 min |
21. |
Proanthocyanidins from Brewers spent grains |
Frequency-400 W |
1.01 mg g−1 |
77
|
Time-55 min |
22. |
Oil from clove |
Power-100–500 W |
71.55% |
68
|
Time-20 min |
23. |
Phenolic components from Punica granatum |
Frequency-20 kHz |
42.45% |
70
|
Power-500 W |
Time-15.12 min |
24. |
Saponins from Medicago sativa |
Power-112 W |
1.61% |
78
|
Time-2.84 h |
Temperature-76.8 °C |
Despite the advantages, ultrasound assisted extraction includes limitations like complete removal of chemical solvents in extraction to achieve satisfactory yields. Future studies are warranted to understand the mechanisms and compare the results. Analysis of the ultrasound power used for extraction, the control and temperature compared to non-conventional extraction methods, pretreatment and sequential extraction technological variations needs to be conducted.79,80
2.3. Gene therapy
Gene therapy is considered as a minimally invasive mode for the treatment of various diseases like cancer, alopecia, etc. by bringing about replacement or modulation of a multifunctional gene.81 Gene therapy uses two basic approaches – the viral approach, involving the use of recombinant vectors like adenovectors, lentivectors, etc. and the non-viral approach that makes the use of nanobubbles, nanodroplets, microbubbles, micelles, etc. Non-viral approach is a better alternative for gene delivery as it can undergo the scale-up process easily and shows low immunogenic toxicity.82 In the case of the non-viral approach, microbubbles are one of the most commonly utilized vectors for gene delivery through cavitation-induced pores. Exposure of low amplitude ultrasound to microbubbles results in the formation of synchronized vibrations through cavitation, growth, oscillation and eventually collapse of small gas bubbles in the fluid. Increasing the pressure also results in an increase in amplitude shifting the vibrations to propagate through the bubble surface. On further increasing the pressure, the bubbles collapse and the internal gas penetrates the shell in the form of a microjet, helping in the delivery of drugs and genes. The adsorption of the microbubbles on the tissue surface takes place due to the ultrasound-produced Bjerknes force.83 These gas-filled microspheres or microbubbles are protected by a shell that helps in developing rigidity and is frequently used in imaging techniques with the help of ultrasound. Cavitation increases the permeability of cell membranes to allow nucleic acids to passively diffuse into the cytoplasm via cavitation-induced pores.7 Excess retention of microbubbles in the blood vessels due to the size is one of the limitations of this approach.84 Application of ultrasound results in the generation of pores that help in the transportation of microbubbles.85 Several experiments are conducted wherein microbubbles are employed for the delivery of genes.
In the case of delivery of the miR-449a gene for the treatment of lung cancer via microbubbles, miRNA-449a is poorly expressed in lung cancer and the expression of this gene is reduced as lung cancer progresses stage-by-stage. Therefore, miR-449a delivery is essential in the case of lung cancer with the help of ultrasound in order to enhance the penetration of actives into the tumor cells.86 In the case of development of multi-drug resistance for chemotherapy, targeted delivery is used as an alternative. Knockdown of highly expressed ESE-1 by combinational use of microbubbles and ultrasound for the delivery of siRNA was found to be effective in treating breast cancer cell lines MDA-MB-468 and BT474.87 Ultrasound driven gene delivery is also used in the treatment of Alzheimer's disease that is characterized by excessive accumulation of amyloid β (Aβ). Microbubbles were used for this purpose, as viral gene delivery was found to be unsatisfactory due to toxicity and immune response. Human Neprilysin was delivered to the skeletal muscles with the help of ultrasound that acts as a rate-limiting step in Aβ degradation. Optimized ultrasound parameters of 1.7 MHz frequency, 1.0 W cm−2 intensity for 1 min were used during the experiment.88 Gene delivery for bone remodeling in the case of fracture for the delivery of the miR-29b-3p gene that helps in osteogenesis, which was confirmed in mesenchymal cells derived from the bone marrow cells of the mouse. On injecting miR-29b-3p into the bone marrow, there was an increase in bone volume fraction. The use of ultrasound helped to enhance cell permeability as a result of shear stress and thermal effects.89 Ultrasound is also used for the delivery of siRNA into non-proliferating T-cells wherein non-viral transfection is difficult. Thus, ultrasound of frequency 2.5 MHz and 1.29 W cm−2 intensity for 5 s was employed for the delivery of siRNA incorporated as microbubbles for efficient transfection. Incorporation of siRNA into T-cells was utilized for the treatment of various diseases by gene silencing, reducing the protein levels or preventing the entry of viruses.90 The use of microbubbles for the delivery of the Cas 9 protein complex with sg-RNA for the treatment of alopecia by the application of ultrasound is another application of ultrasound. Alopecia results in the deficiency of the SRD5A2 gene that is considered as a target for treating alopecia. Cas 9 protein helps in gene editing and its efficiency increases on complexation with sg-RNA. But the complex when used alone tends to undergo degradation by proteases or is neutralized by binding to blood and serum proteins. As a result, they were incorporated into microbubble conjugated nanoliposomes for efficient delivery of the gene to the targeted site. The encapsulation efficiency of nanoliposomes was found to be low (42%) which was increased by coating with PEI where it increased up to 82% due to the use of a high acoustic frequency of about 1–5 MHz.91
Other than microbubbles, nanodroplets are also adopted for the delivery of gene micro RNA-122 (miRNA-122) by the use of ultrasound for the treatment of hepatocellular carcinoma. Delivery of miRNA-122 resulted in the down-regulation of the HCC tissues but direct delivery led to degradation. To prevent the gene from degradation the nanodroplets consist of a shell made up of PGA-g-mPEG. Application of ultrasound (with parameters 1.2 W cm−2 intensity, 1 MHz frequency for 1 min) triggered perfluoropentane transition from the gas to liquid phase resulting in the formation of vapors. In vitro studies showed an increase in the transfection efficiency of nanodroplets due to their exposure to ultrasound.85 Nanobubble treated HCC by delivering shRNA by coating with PEI to prevent shRNA degradation from RNAase enzymes. Optimized parameters for the use of ultrasound were found to be 1 MHz frequency and 1.1 W cm−2 for 60 s.92 Another method for the delivery of genes is by using cationic biosynthetic nanobubbles coated with PEI prepared from Halophilic Archaea acting as a contrast agent where PEI was used not only for the protection of BNBs but also for increasing the loading capacity of the BNBs. It also converted the zeta potential of BNBs, which was previously negative to change to positive for gene transfection. Contrast signals obtained at 18 MHz were found to be the most satisfactory and other parameters selected were 0.6 MPa acoustic pressure for 60 s for the in-vitro gene delivery in the 293Tcells and 4T1 cells. Thus, CBNBs were found to be effective at delivering genes by using ultrasound as it showed improved transfection efficiency.93 In the case of neurological diseases, neural stem cells are considered as one of the targets as they are capable of generating new glial cells. Ultrasound responsive carriers were utilized for the delivery of the naked plasmid to the site of action. Optimum parameters for the delivery of nanocarriers-nanobubbles and bubble lipoplexes were found to be 1 W cm−2 for 60 s. This was found to be an effective way for treating neurological diseases as the levels of transgene expression of nanobubbles and bubble lipoplexes were found to be higher than that of the polyplexes and its expression was found to be higher in the brain and the spinal cord.94 Low-intensity ultrasound radiation helps in reducing the expression of the androgen receptor, a potential target in the case of prostate cancer, by using plasmid-based shRNA.95 The periodontal bone defect is a result of overexpression of the miR182 gene that results in the suppression of the transcription factor which is responsible for bone differentiation. Pulsatile ultrasound of low intensity helped in the inhibition of miRNA 182 to enhance bone differentiation in human primary periodontal ligament cells.96
2.4 Theranostic
Theranostic is a combination of therapeutic and diagnostic applications that shows a significant effect in the treatment of cancer (Fig. 5) and the delivery of stem cells.97,98 A combination of ultrasound and photoacoustic imaging was utilized for the visual tracking of stem cells with the help of gold nanospheres as the contrast agent.99 One of the problems faced during the delivery of anticancer drugs is the efflux of the drug caused due to transporting proteins. Microbubbles along with ultrasound could be utilized effectively for theranostic purposes in bone cancer as they possess the ability to permeate the cell membrane and enhance drug penetration into the tumor cells. A widely known anti-cancer drug doxorubicin was encapsulated in the microbubbles and CEUS imaging was used for diagnostic purposes. A 1.8 fold vascularity, 1.7 fold flow and 2.7 fold perfusion were detected after using CEUS technology, similarly, a 3.7 fold suppression of tumor cells was seen due to the controlled release of doxorubicin from the microbubbles.100 Another carrier for the delivery and diagnosis of doxorubicin to breast cancer cells was synthesized, wherein poly(MAA-co-MBA-co-AA) nanocontainers were modified using gold nanoparticles as a carrier. Ultrasound was applied for the synthesis of nanocontainers for 10 min and MTT assay was performed to determine the anti-tumor activity. A sustained release of doxorubicin was seen (29% at pH 4.6 while 8% at pH 7.4). Confocal laser scanning microscopy was performed for diagnosis purposes depicting that the nanocontainers were localized in the nucleus where doxorubicin was released and intercalated with the double-stranded DNA.101 A new nanodrug for theranostic applications was prepared by using Transferrin, Dihydroartemisinin, L-buthionine-sulfoximine and CellROX in the form of a liposome. Ultrasound was applied for the synthesis of Tf-DBC nanoparticles. The nanodrug synthesized resulted in the tumor-specific amplification of intracellular oxidative stress leading to cell death and the diagnostic properties were provided by CellROX, a fluorescent probe in imaging.102
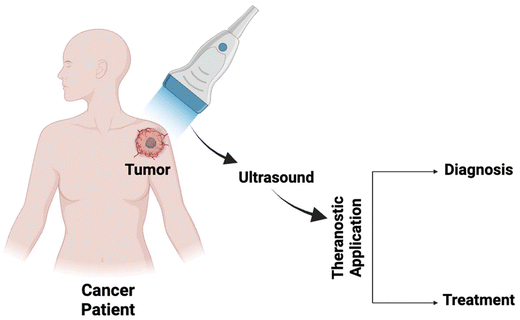 |
| Fig. 5 Theranostic application of ultrasound in cancer. | |
2.5 Tissue engineering
The use of ultrasound in tissue engineering is found to be yet another widely used application. LIPUS is an USFDA approved treatment in the case of fracture, osteoarthritis, also in the generation of several genes responsible for tissue healing.97 Synthesis of hydroxyapatite, a component of bone tissue along with collagen, by ultrasound is applied in tissue engineering, implants, drug delivery, orthopedics and inhibition propagation of cancer cells. Here, the synthesis was carried out via dual-frequency, generated by the combined use of an ultrasonic horn (25 kHz) and an ultrasonic bath (40 kHz). The introduction of the second wave helps in the reduction of standing wave formation leading to improvement in the dispersion of other reagents. Optimum conditions for the experiment were found to be an ultrasonic power of 280 W for 30 min.98 LIPUS can be utilized for tissue engineering in dental follicles as seen in the results of the study that was conducted on rats. An ultrasonic frequency of 1.5 MHz, an ultrasonic intensity of 90 mW cm−2, and a pulse duration of 200 μs for 20 min were the optimized experimental conditions. Tissue regeneration in rats was due to mRNA expression of genes related to osteogenesis which resulted in in vitro mineralization of nodules.99 Electrospinning is one of the techniques in the production of nanofiber scaffolds that could be devised for the natural wound healing process by exposure to ultrasound (frequency of 2.1 MHz) modifying the diameter of the fibers to obtain unique and controlled characteristics.100 Piezoelectric nylon-11 nanoparticles were synthesized by using an anti-solvent technique to enhance the differentiation of stem cells with the help of ultrasound. Ultrasound application proves to be a non-invasive way to enhance the DPSC osteogenic differentiation process.101 HIFU is a USFDA approved method for the treatment of bone metastasis. Thermal activation of the gene was achieved by HIFU exposure at different intensities for 2 min for inducing a two-component gene switch.102
2.6 Miscellaneous
Several other applications of ultrasound such as in therapeutics, diagnostics, etc. make use of ultrasound. Diagnosis of diseases by using ultrasound is one of the most commonly used applications of ultrasound. Microbubble is an ideal choice for diagnosis when exposed to high acoustic frequency as these microbubbles result in nonlinear oscillations causing an emission of harmonics. Ultrasound can be utilized for diagnostic purposes due to its ability to detect contrast agents. The presence of a disease conditions shows a difference in the uptake of contrast agents that are easily detected by using ultrasound.103 Ultrasound in combination with nanocrystals could be used for the generation of ROS affecting the viability of the cell as well as proliferation. The synergistic effect of amino propyl-functionalized ZnO nanocrystals with ultrasound resulted in the formation of ROS with the help of inertial cavitation. This regulation of ROS generated in the cells is necessary for the survival or death of the cells. Ultrasound (frequency of 1 MHz with a power of 0.9 W cm−2 for 20 min) acts as an external stimulus due to its enhanced penetration ability. Ultrasound was used for diagnosis due to real-time imaging, cost-effectiveness and portability but has low sensitivity. In order to overcome this drawback ultrasound was applied along with microbubbles to penetrate through the cells and produce the required actions and gold nanoparticles act as a contrast agent for imaging purposes. The acoustic energy generated due to the bubbles resulted in the enhancement of the ultrasonic contrast. The photocatalytic ability of the nanoparticles was controlled by laser irradiation.104 Another application of ultrasound is in analysis and it offers many advantages e.g. unlike NIR spectroscopy analysis, ultrasound prevents the pre-treatment of materials essential for analysis. Ultrasound for the monitoring of glucose in the cell cultures by using online ultrasound-assisted near-infrared spectroscopy with a transducer emitted an ultrasound frequency of 2.02 MHz. The concentration of glucose in the cells was obtained using the Calibration Model.105 Ultrasound helps in the cleaning process of the root canal, showing its application in dentistry. Ultrasound (30 kHz) helped in the removal of microorganisms by increasing the penetrability of endodontic irrigants such as chlorhexidine digluconate (2%), showing a high cleaning efficiency.105 A lab on chip ultrasonic device, an application of ultrasound, is a nondestructive way of measuring changes in the mechanical properties of the cell culture microenvironment. The measurement was performed with attenuated signals that reached the piezoelectric receivers and no changes were seen in the phenotype of the cells.106 Diagnosis of breast cancer with the help of the identification of vascular morphology features of the tumor was performed on a mouse model as another application of ultrasound. An ultrasonic frequency of 30 MHz was applied for conducting the experiment and a statistical clustering algorithm was used to identify the different torturous vessels.107
3 Conclusions
Ultrasound, discovered in the 1950s, has since then found several applications in the field of pharmaceuticals as well as biomedical science. The article mentions the recent applications of ultrasound as a non-invasive route for the treatment of several diseases and also for its enhanced penetration of cells which helped greatly not only in the delivery of drugs/genes, but also in the extraction of various essential biological components from plants, in the synthesis of several compounds, in the field of theranostics – a combination of diagnosis and therapy, in tissue engineering, etc. Multiple emerging research applications include targeting therapeutics effectively to tumors, exploration of synergistic effects of macro/micromolecules to enhance therapeutic effects, and achievement of real-time analysis in tumor treatment of ultrasound responsive therapeutics. Additionally, safety and biocompatibility concerns must be addressed for in vitro and in vivo translation. The ongoing research areas in disease conditions such as infections, cancer, cardiovascular, Alzheimer's, etc., are recently explored with the aid of nanoparticles for ultrasound-mediated drug delivery. Despite the advantages, multiple challenges are associated with it in biomedicine particularly in drug/gene delivery like development and optimization of multifunctional carrier systems, damage to the surrounding tissues and normal cells due to higher accumulation of therapeutics in the tumor site as a result of ultrasonication, etc. Additionally, ultrasound can cause heating and damage to the biological tissues due to ultrasonic heating by production of large pores with difficulty in resealing. Conversely, various ultrasound intensities can be modulated for multiple applications in biomedicine and further research is warranted for the same. The use of ultrasound makes the process cheap, safe, easy, convenient and a greener approach for providing a brighter future in several fields. Significant clinical trials on humans demonstrate the potential of ultrasound as an effective strategy in biomedicine.
Abbreviations
ESE-1 | Epithelium restricted transcription factor 1 |
ROS | Reactive oxygen species |
tF-DBC | Transferin-dihydroartemisinin |
MTT | 3-(4,5-Dimethylthiazolyl-2)-2,5-diphenyltetrazolium |
CEUS | Contrast-enhanced ultrasound |
HIFU | High intensity focused ultrasound |
DPSC | Dental pulp stem cells |
USFDA | U S Food and Drug Administration |
LIPUS | Low intensity pulsed ultrasound |
UTAE | Ultrasonic thermal assisted extraction |
GAE | Gallic acid equivalent |
PEG | Polyethylene glycol |
IL | Ionic liquids |
PET | Polyethylene terephthalate |
FTIR | Fourier transform infrared |
NMR | Nuclear magnetic resonance |
US-RESSAS | Ultrasonic-assisted rapid expansion of supercritical solution into aqueous solution |
CNT | Carbon nanotubes |
AR | Androgen receptor |
HCC | Hepatocellular carcinoma |
PEI | Polyethyleneimine |
NIR | Near infrared |
sg-RNA | Single guide RNA |
CBNBs | Cationic biosynthetic nanobubbles |
Ethical statements
This article does not contain any studies with human or animal subjects performed by any of the authors.
Conflicts of interest
The authors declare that there is no conflict of interest.
References
-
S. H. Yeo and H. Y. Zhang, in MEMS/NEMS, ed. C. T. Leondes, Techniques in Sonophoresis Biomedical Devices and Their Applications, Springer, Boston, MA, 2006, pp. 1329–1385 Search PubMed.
- D. Park, G. Song, Y. Jo, J. Won, T. Son and O. Cha,
et al., Sonophoresis using ultrasound contrast agents: Dependence on concentration, PLoS One, 2016, 11, 1–14 Search PubMed.
- S. Mitragotri, D. A. Edwards, D. Blankschtein and R. Langer, A mechanistic study of ultrasonically–enhanced transdermal drug delivery, J. Pharm. Sci., 1995, 84, 697–706 CrossRef CAS PubMed.
- A. Pahade, V. M. Jadhav and V. J. Kadam, Sonophoresis: An overview, Int. J. Pharm. Sci. Rev. Res., 2010, 3, 24–32 CAS.
- A. Azagury, L. Khoury, G. Enden and J. Kost, Ultrasound mediated transdermal drug delivery, Adv. Drug Delivery Rev., 2014, 72, 127–143 CrossRef CAS PubMed . Available from: https://www.sciencedirect.com/science/article/pii/S0169409X14000088.
- J. Sitta and C. M. Howard, Applications of ultrasound-mediated drug delivery and gene therapy, Int. J. Mol. Sci., 2021, 11491 CrossRef CAS PubMed.
- Z. Krut, D. Gazit, Z. Gazit and G. Pelled, Applications of Ultrasound-Mediated Gene Delivery in Regenerative Medicine, Bioengineering (Basel), 2022, 9, 190 CrossRef CAS PubMed.
- K. Tachibana and S. Tachibana, The use of ultrasound for drug delivery, Echocardiography, 2001, 18, 323–328 CrossRef CAS PubMed.
- X. Xu, A. Liu, Y. Bai, Y. Li, C. Zhang and S. Cui,
et al., Co-delivery of resveratrol and p53 gene via peptide cationic liposomal nanocarrier for the synergistic treatment of cervical cancer and breast cancer cells, J. Drug Delivery Sci. Technol., 2019, 51, 746–753 CrossRef CAS.
- M. Ahmad, J. Wang, Z. Yang, Q. Zhang and B. Zhang, Ultrasonic-assisted preparation of amidoxime functionalized silica framework via oil-water emulsion method for selective uranium adsorption, Chem. Eng. J., 2020, 389, 124441 CrossRef CAS.
- P. Yaghoubi, A. Hajnorouzi, M. S. Rahmanifar and A. H. Foruzan, Synthesis of MnO2 Nanoparticles in the Presence and Absence of Ultrasonic Irradiation, Iran. J. Sci. Technol., Trans. A: Sci., 2019, 43, 2619–2626 CrossRef.
- G. Sodeifian, S. A. Sajadian, N. S. Ardestani and F. Razmimanesh, Production of Loratadine drug nanoparticles using ultrasonic-assisted Rapid expansion of supercritical solution into aqueous solution (US-RESSAS), J. Supercrit. Fluids, 2019, 147, 241–253 CrossRef CAS.
- G. S. Shinde, P. D. Nayak, S. P. Vanam, S. K. Jain, A. D. Pathak and S. Sanyal,
et al., Ultrasonic sonochemical synthesis of Na0.44MnO2 insertion material for sodium-ion batteries, J. Power Sources, 2019, 416, 50–55 CrossRef CAS.
- M. Hagar, K. Chaieb, S. Parveen, H. A. Ahmed and R. B. Alnoman, N-alkyl 2-pyridone versus O-alkyl 2-pyridol: Ultrasonic synthesis, DFT, docking studies and their antimicrobial evaluation, J. Mol. Struct., 2020, 1199, 126926 CrossRef CAS.
- G. S. Nhivekar and V. K. Rathod, Acoustic cavitation assisted lipase B catalysed synthesis of polyethylene glycol stearate in a solvent free system via esterification: synthesis and optimization, J. Chem. Technol. Biotechnol., 2019, 94, 2465–2474 CrossRef CAS.
- A. Rajabi, M. J. Ghazali, E. Mahmoudi, A. H. Baghdadi, A. W. Mohammad and N. M. Mustafah,
et al., Synthesis, characterization, and antibacterial activity of ag 2 o-loaded polyethylene terephthalate fabric via ultrasonic method, Nanomaterials, 2019, 9, 450 CrossRef CAS PubMed.
- W. Lan, X. Liang, W. Lan, S. Ahmed, Y. Liu and W. Qin, Electrospun polyvinyl alcohol/d-limonene fibers prepared by ultrasonic processing for antibacterial active packaging material, Molecules, 2019, 24, 767 CrossRef PubMed.
- N. Gajić, Ž Kamberović, Z. Anđić, J. Trpčevská, B. Plešingerova and M. Korać, Synthesis of tribological WS2 powder from WO3 prepared by ultrasonic spray pyrolysis (USP), Metals, 2019, 9, 277 CrossRef.
- Y. Guo, C. Li, Y. Guo, X. Wang and X. Li, Ultrasonic-assisted synthesis of mesoporous g-C3N4/Na-bentonite composites and its application for efficient photocatalytic simultaneous removal of Cr(VI) and RhB, Colloids Surf., A, 2019, 578, 123624 CrossRef CAS.
- H. R. Mahmoud, S. A. El-Molla and M. A. Naghmash, Novel mesoporous MnO 2 /SnO 2 nanomaterials synthesized by ultrasonic-assisted co-precipitation method and their application in the catalytic decomposition of hydrogen peroxide, Ultrasonics, 2019, 95, 95–103 CrossRef CAS PubMed.
- J. Liu, L. Yang, Z. Song and C. Xu, Microstructures and capacitance performance of MnO 2 films fabricated by ultrasonic-assisted electrodeposition, Appl. Surf. Sci., 2019, 478, 94–102 CrossRef CAS.
- S. A. Khan, M. F. Siddiqui and T. A. Khan, Ultrasonic-assisted synthesis of polyacrylamide/bentonite hydrogel nanocomposite for the sequestration of lead and cadmium from aqueous phase: Equilibrium, kinetics and thermodynamic studies, Ultrason. Sonochem., 2020, 60, 104761 CrossRef CAS PubMed.
- M. H. Aghaali and S. Firoozi, Synthesis of nanostructured fcc/hcp hollow Ni particles by ultrasonic spray pyrolysis and its dry reforming catalytic properties, Powder Technol., 2019, 356, 119–128 CrossRef CAS.
- N. N. Huy, V. T. T. Thuy, N. H. Thang, N. T. Thuy, L. T. Quynh and T. T. Khoi,
et al., Facile one-step synthesis of zinc oxide nanoparticles by ultrasonic-assisted precipitation method and its application for H2S adsorption in air, J. Phys. Chem. Solids, 2019, 132, 99–103 CrossRef CAS.
- S. A. Arote, A. S. Pathan, Y. V. Hase, P. P. Bardapurkar, D. L. Gapale and B. M. Palve, Investigations on synthesis, characterization and humidity sensing properties of ZnO and ZnO-ZrO2 composite nanoparticles prepared by ultrasonic assisted wet chemical method, Ultrason. Sonochem., 2019, 55, 313–321 CrossRef CAS PubMed.
- Y. Guo, X. Yang, G. Li, B. Dong and L. Chen, Effect of ultrasonic intensification on synthesis of nano-sized particles with an impinging jet reactor, Powder Technol., 2019, 354, 218–230 CrossRef CAS.
- Abdullah, A. Balouch, F. N. Talpur, A. Kumar, M. T. Shah and A. M. Mahar,
et al., Synthesis of ultrasonic-assisted lead ion imprinted polymer as a selective sorbent for the removal of Pb 2+ in a real water sample, Microchem. J., 2019, 146, 1160–1168 CrossRef CAS.
- N. M. Bahadur, F. Chowdhury, M. Obaidullah, M. S. Hossain, R. Rashid and Y. Akter,
et al., Ultrasonic-Assisted Synthesis, Characterization, and Photocatalytic Application of SiO2@TiO2 Core-Shell Nanocomposite Particles, J. Nanomater., 2019, 2019, 1–11 CrossRef.
- F. Ynineb, D. E. Guitoume, D. Mendil, N. Attaf, M. S. Aida and H. Farh, Zno nanorods prepared by ultrasonic spray pyrolysis: Effect of deposition time on the structural morphological and optical properties, Defect Diffus. Forum, 2019, 397, 88–100 Search PubMed.
- X. Wu, G. Xu and J. J. Zhu, Sonochemical synthesis of Fe3O4/carbon nanotubes using low frequency ultrasonic devices and their performance for heterogeneous sono-persulfate process on inactivation of Microcystis aeruginosa, Ultrason. Sonochem., 2019, 58, 104634 CrossRef CAS PubMed.
- P. Karuppaiah, N. S. K. Gowthaman, V. Balakumar, S. Shankar and H. N. Lim, Ultrasonic synthesis of CuO nanoflakes: A robust electrochemical scaffold for the sensitive detection of phenolic hazard in water and pharmaceutical samples, Ultrason. Sonochem., 2019, 58, 104649 CrossRef CAS PubMed.
- L. Muñoz-Fernandez, G. Alkan, O. Milošević, M. E. Rabanal and B. Friedrich, Synthesis and characterisation of spherical core-shell Ag/ZnO nanocomposites using single and two – steps ultrasonic spray pyrolysis (USP), Catal. Today, 2019, 321–322, 26–33 CrossRef.
- S. Vinoth, P. Sampathkumar, K. Giribabu and A. Pandikumar, Ultrasonically assisted synthesis of barium stannate incorporated graphitic carbon nitride nanocomposite and its analytical performance in electrochemical sensing of 4-nitrophenol, Ultrason. Sonochem., 2020, 62, 104855 CrossRef CAS PubMed.
-
S. Murugan, Q. B. Nguyen and M. GuptaSynthesis of Magnesium Based Nano-composites, in Magnesium - The Wonder Element for Engineering/Biomedical Applications, 2020, p. 13 Search PubMed.
- P. Szperlich and B. Toroń, An ultrasonic fabrication method for epoxy resin/SbSI nanowire composites, and their application in nanosensors and nanogenerators, Polymers, 2019, 11, 479 CrossRef CAS PubMed.
- Y. Xu, Y. Pang, L. Luo, A. Sharma, J. Yang and C. Li,
et al., De Novo Designed Ru(II) Metallacycle as a Microenvironment-Adaptive Sonosensitizer and Sonocatalyst for Multidrug-Resistant Biofilms Eradication, Angew. Chem., Int. Ed., 2024, 63, e202319966, DOI:10.1002/anie.202319966.
- K. M. Zia, S. Adeel, F. U. Rehman, H. Aslam, M. K. Khosa and M. Zuber, Influence of ultrasonic radiation on extraction and green dyeing of mordanted cotton using neem bark extract, J. Ind. Eng. Chem., 2019, 77, 317–322 CrossRef CAS.
- N. A. A. R. Zahari, G. H. Chong, L. C. Abdullah and B. L. Chua, Ultrasonic-assisted extraction (UAE) process on thymol concentration from Plectranthus amboinicus leaves: Kinetic modeling and optimization, Processes, 2020, 8, 322 CrossRef CAS.
- W. Zhang, Y. G. Pan, W. Huang, H. Chen and H. Yang, Optimized ultrasonic-assisted extraction of papaya seed oil from Hainan/Eksotika variety, Food Sci. Nutr., 2019, 7, 2692–2701 CrossRef CAS PubMed.
- A. Yohannes, B. Zhang, B. Dong and S. Yao, Ultrasonic extraction of tropane alkaloids from radix physochlainae using as extractant an ionic liquid with similar structure, Molecules, 2019, 24, 2897 CrossRef CAS PubMed.
- Z. Ullah, Z. Man, A. S. Khan, N. Muhammad, H. Mahmood and O. Ben Ghanem,
et al., Extraction of valuable chemicals from sustainable rice husk waste using ultrasonic assisted ionic liquids technology, J. Cleaner Prod., 2019, 220, 620–629 CrossRef CAS.
- B. Yingngam, H. Zhao, B. Baolin, N. Pongprom and A. Brantner, Optimization of ultrasonic-assisted extraction and purification of rhein from cassia fistula pod pulp, Molecules, 2019, 24, 2013 CrossRef CAS PubMed.
- Y. Zheng, J. Cui, A. H. Chen, Z. M. Zong and X. Y. Wei, Optimization of Ultrasonic-Microwave Assisted Extraction and Hepatoprotective Activities of Polysaccharides from Trametes orientalis, Molecules, 2019, 24, 147 CrossRef PubMed.
- H. Shahram and S. T. Dinani, Optimization of ultrasonic-assisted enzymatic extraction of β-carotene from orange processing waste, J. Food Process Eng., 2019, 42, 1–16 Search PubMed.
- L. Xiong, W. B. Hu, Z. W. Yang, Hui-Chen, Wang-Ning and Liu-Xin,
et al., Enzymolysis-ultrasonic assisted extraction of flavanoid from Cyclocarya paliurus (Batal) Iljinskaja:HPLC profile, antimicrobial and antioxidant activity, Ind. Crops Prod., 2019, 130, 615–626 CrossRef.
- S. K. Saha, S. Dey and R. Chakraborty, Effect of choline chloride-oxalic acid based deep eutectic solvent on the ultrasonic assisted extraction of polyphenols from Aegle marmelos, J. Mol. Liq., 2019, 287, 110956 CrossRef CAS.
- P. Rodsamran and R. Sothornvit, Extraction of phenolic compounds from lime peel waste using ultrasonic-assisted and microwave-assisted extractions, Food Biosci., 2019, 28, 66–73 CrossRef CAS.
- S. Rahimi and M. Mikani, Lycopene green ultrasound-assisted extraction using edible oil accompany with response surface methodology (RSM) optimization performance: Application in tomato processing wastes, Microchem. J., 2019, 146, 1033–1042 CrossRef CAS.
- P. Prawang, Y. Zhang, Y. Zhang and H. Wang, Ultrasonic Assisted Extraction of Artemisinin from Artemisia annua L. Using Poly(Ethylene Glycol): Toward a Greener Process, Ind. Eng. Chem. Res., 2019, 58, 18320–18328 CrossRef CAS.
-
M. Pang, Q. Liu, Y. L. Yu and S. L. Cai, Ultrasonic-microwave synergistic extraction of paprika pigment. E3S Web of Conferences. 2019. pp. 2007–2010.
- J. Liu, T. Mu, H. Sun and M. L. Fauconnier, Optimization of ultrasonic–microwave synergistic extraction of flavonoids from sweet potato leaves by response surface methodology, J. Food Process. Preserv., 2019, 43, 1–10 Search PubMed.
- T. T. Nguyen, X. Luo, P. Su, B. Balakrishnan and W. Zhang, Highly efficient recovery of nutritional proteins from Australian Rock Lobster heads (Jasus edwardsii) by integrating ultrasonic extraction and chitosan co-precipitation, Innovative Food Sci. Emerging Technol., 2020, 60, 102308 CrossRef CAS.
- B. Loretta, M. Oliviero, M. Vittorio, E. Bojórquez-Quintal, P. Franca and P. Silvia,
et al., Quality by design approach to optimize cladodes soluble fiber processing extraction in Opuntia ficus indica (L.) Miller, J. Food Sci. Technol., 2019, 56, 3627–3634 CrossRef CAS PubMed.
- H. Y. Lee, Optimization of Cyanidin-3-O-galactoside production from Aronia melanocarpa Elliot from nonthermal ultrasonic extraction process by response surface methodology, Appl. Sci., 2019, 9, 1203 CrossRef CAS.
- B. Jiang, L. Wang, X. Wang, S. Wu, D. Li and C. Liu,
et al., Ultrasonic thermal-assisted extraction of phosvitin from egg yolk and evaluation of its properties, Polymers, 2019, 11, 1–16 Search PubMed.
- Z. Jiang, R. Shi, H. Chen and Y. Wang, Ultrasonic microwave-assisted extraction coupled with macroporous resin chromatography for the purification of antioxidant phenolics from waste jackfruit (Artocarpus heterophyllus Lam.) peels, J. Food Sci. Technol., 2019, 56, 3877–3886 CrossRef CAS PubMed.
- Q. Ding, H. Jiang, Y. Chen, L. Luo, R. He and H. Ma,
et al., Influence of nitrogen protection on the extraction yield and antioxidant activities of polyphenols by ultrasonic-assisted extraction from rapeseed meal, J. Food Process Eng., 2019, 42, 1–11 Search PubMed.
- S. Boonyubol, S. Kodama and H. Sekiguchi, Effect of alumina particles on simultaneous lipid extraction and biodiesel production from microalgae under ultrasonic irradiation, J. Chem. Eng. Jpn., 2020, 53, 153–159 CrossRef CAS.
- A. Azimi Mahalleh, P. Sharayei and E. Azarpazhooh, Optimization of ultrasonic-assisted extraction of bioactive compounds from Nepeta (Nepeta binaludensis Jamzad), J. Food Meas. Charact., 2020, 14, 668–678 CrossRef.
- Q. Q. Yang, R. Y. Gan, Y. Y. Ge, D. Zhang and H. Corke, Ultrasonic treatment increases extraction rate of common bean (Phaseolus vulgaris l.) antioxidants, Antioxidants, 2019, 8, 83 CrossRef PubMed.
- X. Mai, Y. Liu, X. Tang, L. Wang, Y. Lin and H. Zeng,
et al., Sequential extraction and enrichment of flavonoids from Euonymus alatus by ultrasonic-assisted polyethylene glycol-based extraction coupled to temperature-induced cloud point extraction, Ultrason. Sonochem., 2020, 66, 105073 CrossRef CAS PubMed.
- N. B. Eremeeva, N. V. Makarova, E. M. Zhidkova, V. P. Maximova and E. A. Lesovaya, Ultrasonic and microwave activation of raspberry extract: Antioxidant and anti-carcinogenic properties, Foods Raw Mater., 2019, 7, 264–273 CAS.
- R. Jalilian, M. Shahmari, A. Taheri and K. Gholami, Ultrasonic-assisted micro solid phase extraction of arsenic on a new ion-imprinted polymer synthesized from chitosan-stabilized pickering emulsion in water, rice and vegetable samples, Ultrason. Sonochem., 2020, 61, 104802 CrossRef CAS PubMed.
- Q. Liang, J. Zhang, X. Su, Q. Meng and J. Dou, Extraction and separation of eight ginsenosides from flower buds of Panax ginseng using aqueous ionic liquid-based ultrasonic-assisted extraction coupled with an aqueous biphasic system, Molecules, 2019, 24, 1–12 CrossRef PubMed.
- M. Kazemi, F. Khodaiyan, M. Labbafi and S. Saeid, Ultrasonic and heating extraction of pistachio by - product pectin : physicochemical, structural characterization and functional measurement, J. Food Meas. Charact., 2019, 679–693 Search PubMed.
- M. Kazemi, F. Khodaiyan and S. S. Hosseini, Eggplant peel as a high potential source of high methylated pectin: Ultrasonic extraction optimization and characterization, LWT, 2019, 105, 182–189 CrossRef CAS.
- K. Hao, W. Hu, M. Hou, D. Cao, Y. Wang and Q. Guan,
et al., Optimization of ultrasonic-assisted extraction of total phenolics from citrus aurantium L. Blossoms and evaluation of free radical scavenging, Anti-HMG-CoA Reductase Activities, Molecules, 2019, 24, 2368 CrossRef CAS PubMed.
- S. Yunusa, Y. Um and I. Haruna, Comparison of essential oils of clove buds extracted using soxhlet and ultrasonic-assisted extraction methods (short communication), J. Exper. Res., 2019, 7, 75–78 Search PubMed.
- K. Nomura and P. Terwilliger, Self-dual Leonard pairs guarana seed under the action, Spec. Metrices, 2019, 7, 1–19 CrossRef.
- R. Foujdar, M. B. Bera and H. K. Chopra, Optimization of process variables of probe ultrasonic-assisted extraction of phenolic compounds from the peel of Punica granatum Var. Bhagwa and it's chemical and bioactivity characterization, J. Food Process. Preserv., 2020, 44, 1–16 Search PubMed.
- M. Shi, J. Zhang, C. Liu, Y. Cui, C. Li and Z. Liu,
et al., Ionic liquid-based ultrasonic-assisted extraction to analyze seven compounds in Psoralea fructus coupled with HPLC, Molecules, 2019, 24, 1699 CrossRef CAS PubMed.
- R. Nuerxiati, A. Abuduwaili, P. Mutailifu, A. Wubulikasimu, N. Rustamova and C. Jingxue,
et al., Optimization of ultrasonic-assisted extraction, characterization and biological activities of polysaccharides from Orchis chusua D. Don (Salep), Int. J. Biol. Macromol., 2019, 141, 431–443 CrossRef CAS PubMed.
- Y. Jiang, X. Bai, S. Lang, Y. Zhao, C. Liu and L. Yu, Optimization of ultrasonic-microwave assisted alkali extraction of arabinoxylan from the corn bran using response surface methodology, Int. J. Biol. Macromol., 2019, 128, 452–458 CrossRef CAS PubMed.
- B. Hu, C. Li, W. Qin, Z. Zhang, Y. Liu and Q. Zhang,
et al., A method for extracting oil from tea (Camelia sinensis) seed by microwave in combination with ultrasonic and evaluation of its quality, Ind. Crops Prod., 2019, 131, 234–242 CrossRef CAS.
- G. Chen, C. Fang, X. Chen, Z. Wang, M. Liu and J. Kan, High-pressure ultrasonic-assisted extraction of polysaccharides from Mentha haplocalyx: Structure, functional and biological activities, Ind. Crops Prod., 2019, 130, 273–284 CrossRef CAS.
- T. Belwal, H. Huang, L. Li, Z. Duan, X. Zhang and H. Aalim,
et al., Optimization model for ultrasonic-assisted and scale-up extraction of anthocyanins from Pyrus communis ‘Starkrimson’ fruit peel, Food Chem., 2019, 297, 124993 CrossRef CAS PubMed.
- B. Martín-García, F. Pasini, V. Verardo, E. Díaz-De-cerio, U. Tylewicz and A. M. Gómez-Caravaca,
et al., Optimization of sonotrode ultrasonic-assisted extraction of proanthocyanidins from brewers’ spent grains, Antioxidants, 2019, 8, 282 CrossRef PubMed.
- M. Hadidi, A. Ibarz and J. Pagan, Optimisation and kinetic study of the ultrasonic-assisted extraction of total saponins from alfalfa (Medicago sativa) and its bioaccessibility using the response surface methodology, Food Chem., 2020, 309, 125786 CrossRef CAS PubMed.
- G. Linares and M. L. Rojas, Ultrasound-Assisted Extraction of Natural Pigments From Food Processing By-Products: A Review, Front Nutr., 2022, 891462 CrossRef CAS PubMed.
-
K. Kumar, S. Srivastav and V. S. Sharanagat, Ultrasound assisted extraction (UAE) of bioactive compounds from fruit and vegetable processing by-products: A review, Ultrason Sonochem, Elsevier B.V., 2021 Search PubMed.
- M. Bez, J. Foiret, G. Shapiro and G. Pelled,
et al., Nonviral ultrasound-mediated gene delivery in small and large animal models, Physiol. Behav., 2017, 176, 139–148 CrossRef PubMed.
- W. Dong, P. Wu, D. Zhou, J. Huang, M. Qin and X. Yang,
et al., Ultrasound-Mediated Gene Therapy of Hepatocellular Carcinoma Using Pre-microRNA Plasmid-Loaded Nanodroplets, Ultrasound Med. Biol., 2020, 46, 90–107 CrossRef PubMed.
- M. Nakata, N. Tanimura, D. Koyama and M. P. Krafft, Adsorption and Desorption of a Phospholipid from Single Microbubbles under Pulsed Ultrasound Irradiation for Ultrasound-Triggered Drug Delivery, Langmuir, 2019, 35, 10007–10013 CrossRef CAS PubMed.
- S. Hameed, M. Zhang, P. Bhattarai, G. Mustafa and Z. Dai, Enhancing cancer therapeutic efficacy through ultrasound-mediated micro-to-nano conversion, Wiley Interdiscip. Rev.: Nanomed. Nanobiotechnol., 2019, 1–23 Search PubMed.
- G. Huanling, M. Xu, Z. Cao, W. Li, L. Chen and X. Xie,
et al., Ultrasound-Assisted miR-122-Loaded Polymeric Nanodroplets for Hepatocellular Carcinoma Gene Therapy, Mol Pharm, 2019, 541–553 Search PubMed.
- L. Meng, S. Yuan, L. Zhu, Z. Shangguan and R. Zhao, Ultrasound-microbubbles-mediated microRNA-449a inhibits lung cancer cell growth via the regulation of notch1, OncoTargets Ther., 2019, 12, 7437–7450 CrossRef CAS PubMed.
- K. H. Song, T. Trudeau, A. Kar, M. A. Borden and A. Gutierrez-Hartmann, Ultrasound-mediated delivery of siESE complexed with microbubbles attenuates HER2+/-cell line proliferation and tumor growth in rodent models of breast cancer, Nanotheranostics, 2019, 3, 212–222 CrossRef PubMed.
- Y. Li, Y. Wang, J. Wang, K. Y. Chong, J. Xu and Z. Liu,
et al., Expression of Neprilysin in Skeletal Muscle by Ultrasound-Mediated Gene Transfer (Sonoporation) Reduces Amyloid Burden for AD, Mol. Ther. – Methods Clin. Dev., 2020, 17, 300–308 CrossRef CAS PubMed.
- W. Y. Lee, N. Li, S. Lin, B. Wang, H. Y. Lan and G. Li, miRNA-29b improves bone healing in mouse fracture model, Mol. Cell. Endocrinol., 2016, 430, 97–107 CrossRef CAS PubMed.
- A. Karki, E. Giddings, A. Carreras, D. Champagne, K. Fortner and M. Rincon,
et al., Sonoporation as an Approach for siRNA delivery into T cells, Ultrasound Med. Biol., 2019, 45, 3222–3231 CrossRef PubMed.
- J. Ryu, E. Won, H. A. R. Lee, J. H. Kim, E. Hui and H. P. Kim,
et al., Ultrasound-activated particles as CRISPR/Cas9 delivery system for androgenic alopecia therapy, Biomaterials, 2020, 119736 CrossRef CAS PubMed.
- H. Li, Z. Wang, J. Zhang, C. Yuan, H. Zhang and X. Hou,
et al., Enhanced shRNA delivery by the combination of polyethylenimine, ultrasound, and nanobubbles in liver cancer, Technol. Healthcare, 2019, 27, S263–S272 Search PubMed.
- B. Tayier, Z. Deng, Y. Wang, W. Wang, Y. Mu and F. Yan, Biosynthetic nanobubbles for targeted gene delivery by focused ultrasound, Nanoscale, 2019, 11, 14757–14768 RSC.
- K. Ogawa, Y. Fuchigami, M. Hagimori, S. Fumoto, K. Maruyama and S. Kawakami, Ultrasound-responsive nanobubble-mediated gene transfection in the cerebroventricular region by intracerebroventricular administration in mice, Eur. J. Pharm. Biopharm., 2019, 137, 1–8 CrossRef CAS PubMed.
- T. Wang, F. Zhang, H. Ye, L. An, L. Xiong and X. Huang,
et al., Targeting silencing androgen receptor gene by shRNA with low-intensity focused ultrasonic irradiation inhibits growth of prostate cancer xenografts in nude mice, Int. J. Clin. Exp. Pathol., 2019, 12, 1295–1304 CAS.
- D. Chen, M. Xiang, Y. Gong, L. Xu, T. Zhang and Y. He,
et al., LIPUS promotes FOXO1 accumulation by downregulating miR-182 to enhance osteogenic differentiation in hPDLCs, Biochimie, 2019, 165, 219–228 CrossRef CAS PubMed.
- T. Xue, W. Liang, Y. Li, Y. Sun, Y. Xiang and Y. Zhang,
et al., Ultrasensitive detection of miRNA with an antimonene-based surface plasmon resonance sensor, Nat. Commun., 2019, 10, 28 CrossRef CAS PubMed.
- X. Zhang, C. Li, Y. Zhang, X. Guan, L. Mei and H. Feng,
et al., Construction of Long-Wavelength Emissive Organic Nanosonosensitizer Targeting Mitochondria for Precise and Efficient In Vivo Sonotherapy, Adv. Funct. Mater., 2022, 32, 2207259, DOI:10.1002/adfm.202207259.
- K. P. Kubelick, E. J. Snider, C. R. Ethier and S. Emelianov, Development of a stem cell tracking platform for ophthalmic applications using ultrasound and photoacoustic imaging, Theranostics, 2019, 9, 3812–3824 CrossRef CAS PubMed.
- T. T. Kuo, C. H. Wang, J. Y. Wang, H. J. Chiou, C. H. Fan and C. K. Yeh, Concurrent osteosarcoma theranostic strategy using contrast-enhanced ultrasound and drug-loaded bubbles, Pharmaceutics, 2019, 11, 223 CrossRef CAS PubMed.
- M. Theodosiou, N. Boukos, E. Sakellis, M. Zachariadis and E. K. Efthimiadou, Gold nanoparticle decorated pH-sensitive polymeric nanocontainers as a potential theranostic agent, Colloids Surf., B, 2019, 183, 110420 CrossRef CAS PubMed.
- X. A. Yu, M. Lu, Y. Luo, Y. Hu, Y. Zhang and Z. Xu,
et al., A cancer-specific activatable theranostic nanodrug for enhanced therapeutic efficacy via amplification of oxidative stress, Theranostics, 2020, 10, 371–383 CrossRef CAS PubMed.
- J. Li, A. Xi, H. Qiao and Z. Liu, Ultrasound-mediated diagnostic imaging and advanced treatment with multifunctional micro/nanobubbles, Cancer Lett., 2020, 475, 92–98 CrossRef CAS PubMed.
- I. C. Sun and S. Emelianov, Gas-generating nanoparticles for contrast-enhanced ultrasound imaging, Nanoscale, 2019, 11, 16235–16240 RSC.
- T. Kambayashi, T. Noguchi, A. Nojima, S. Kono, S. I. Taniguchi and Y. Ozaki, Glucose Monitoring in Cell Culture with Online Ultrasound-Assisted Near-Infrared Spectroscopy, Anal. Chem., 2020, 92, 2946–2952 CrossRef CAS PubMed.
- A. Zareei, H. Jiang, S. Chittiboyina, J. Zhou, B. P. Marin and S. A. Lelièvre,
et al., A lab-on-chip ultrasonic platform for real-time and nondestructive assessment of extracellular matrix stiffness, Lab Chip, 2020, 20, 778–788 RSC.
- S. E. Shelton, J. Stone, F. Gao, D. Zeng and P. A. Dayton, Microvascular Ultrasonic Imaging of Angiogenesis Identifies Tumors in a Murine Spontaneous Breast Cancer Model, Int. J. Biomed. Imaging, 2020, 2020, 7862089 Search PubMed.
|
This journal is © The Royal Society of Chemistry 2024 |
Click here to see how this site uses Cookies. View our privacy policy here.