DOI:
10.1039/D3PM00058C
(Paper)
RSC Pharm., 2024,
1, 333-343
Elucidation of processing parameters for the reverse engineering of tablets
Received
1st December 2023
, Accepted 3rd April 2024
First published on 22nd April 2024
Abstract
Reverse engineering can assist in decoding the formula and manufacturing parameters employed in innovator formulations. Generic pharmaceutical industries use it to develop generic cheaper versions of innovator tablets. Herein, we report the systematic application of reverse engineering in determining the manufacturing process utilized by innovators to prepare tablet formulations. The outcome inferred that the critical information such as the granulation and solvent type in the innovator formulation could be identified by systematic analysis via scanning electron microscopy (SEM) images and sieve and texture analysis. Furthermore, critical investigation of the levels of fines generated during sieve analysis could reveal the tablet manufacturing process. It was observed that the maximum amount of fines was generated in the case of post-compression granules obtained by tablets prepared by direct compression. The hardness of granules is yet another major factor that could help to delineate the type of drying technique used in innovator manufacturing. Granules obtained from crushing a tablet prepared by wet granulation with tray drying were harder than those prepared by drying on a fluidized bed dryer (FBD). The outcome of this investigation may be helpful for formulation scientists working on the development of generic formulations.
Introduction
Several dosage forms are available for the treatment of allied diseases and disorders, including tablets, capsules, creams, ointments, suspensions, emulsions, gels, mixtures, pellets, lozenges, liniments, lotions, pastes, suppositories, sprays, and inhalants.1 Among these dosage forms, tablets are the most widely employed due to the advantages of a superior stability profile, high patient compliance, economical dosage form, ease of handling, ease of administration, and ease of transformation into specialized drug products (such as for delayed enteric release).2 The tablets prepared by innovators are usually costly due to the involvement of enormous research and development costs. Hence, the pharma industry is inclined to manufacture generic versions of innovator products.3 Mapping a generic version of a tablet with that of the innovator is a very tricky juncture in the development of generics.
Reverse engineering is defined as the process involving the identification, separation, and quantitation of individual components or ingredients in a drug product. The information concerning these process parameters is necessary to create a generic version of medication and requires various chemical processes to discover the exact contents of a formula.4 To achieve this goal, reverse engineering usually follows three steps, i.e., decoding the quantitative formula, solid-state characterization of the active pharmaceutical ingredient (API), and identifying the manufacturing process.
Once the formula for the dosage form is obtained, various separation processes have to be performed to separate all the ingredients for decoding the quantitative formula. Generally, the separation techniques used to separate the components include differential solubility, high-performance liquid chromatography (HPLC), and thin-layer chromatography.5
After separating all the ingredients, their quantitative analysis is carried out using different techniques such as gravimetric analysis, differential scanning calorimetry (DSC) and UV-visible spectroscopy.5 After the successful determination of the quantitative formula, in the second step, the characterization of the API is performed. In this step, many techniques are used to characterize the API, including DSC, which is used to identify the physical interaction between the API and the formulation excipients;6 Fourier-transform infrared spectroscopy (FTIR), which is used to find out any chemical interaction between the API and the excipients present;7 powder X-ray diffraction (XRD), which is used to check the polymorphic forms of the API;8 and microscopic techniques, which are executed to observe the morphology as well as to measure the particle size of the API.9
In the last step, the identification of the manufacturing step is carried out. In this step, the method used for manufacturing the tablets can be predicted based on the physicochemical properties of the API by checking the disintegration pattern under a microscope or by visual examination of tablet fracture.5
If the API is sensitive to moisture, then a wet granulation process cannot be employed to prepare the tablet, and so the tablets are prepared using direct compression (DC) or dry granulation method. If the API remains stable in moisture, it can be formulated into a tablet using any of three main processes. Hence, it becomes challenging to rely on one method to determine the process used for manufacturing.5,10
Further, the disintegration pattern of the tablet can be observed under a microscope by putting a small piece of tablet in a disintegrating medium under a microscope. During disintegration, if the tablet first disintegrates into granules and then into smaller particles, it can be inferred that the granulation technique was used to manufacture the tablet. If small particles are observed directly, then it can be concluded that the method used for manufacturing the tablet was DC. However, in this technique, it is also extremely difficult to identify the granulation method used to prepare the tablets, i.e., dry granulation or wet granulation.5
Fracture of the tablet can also be employed to confirm the manufacturing process of the tablet. If the fracture is smooth, then it infers that the DC method was used for manufacturing. On the other hand, if the fracture is rough, then granulation was used. It may be noted that the exact granulation process, i.e., whether wet or dry granulation has been used, cannot be precisely predicted.5 Hence, there is an urgent need to devise a rapid tool to identify the information concerning these process parameters toward creating a generic tablet formulation.
The tablet, being the most commonly exercised conventional dosage form, has been the most explored in research setups, leaving us with very narrow areas to explore in this domain. Researchers are already trying to fill knowledge gaps, enhance tablet quality, and accelerate the production of generic pharmaceuticals through methodical examinations of the various formulation elements, production procedures, and analytical techniques. Toward this avenue, this research reports different techniques to identify the characteristic features to conclude on the manufacturing process. Tablets prepared using different approaches were evaluated using various methods, including SEM, a texture analyzer, and a sieve shaker to determine the characteristic patterns associated with particular manufacturing processes. Hence, the reported literature is bound to possess overlapping similarities in respective studies. However, to the best of our knowledge we are the first to evaluate the effect of the method of granulation and the solvent used on the morphological features using SEM. Pre-compression and post-compression hardness studies are also distinctively featured in this manuscript.
Material and methods
Materials
Lamivudine was received as a gift sample (Aurigene Discovery Technologies Limited, Bangalore, India). Lactose anhydrous (DuraLac® H) was purchased from Meggle Excipient and Technology (Mumbai, India). Microcrystalline cellulose (MCC, Avicel PH-101) and polyvinyl pyrrolidine (PVP) k-30 were purchased from FMC Biopolymer (Mumbai, India). Magnesium stearate was procured from Loba Chemie (Mumbai, India). Talc was procured from Luzenac Pharma (Mumbai, India), and isopropyl alcohol was purchased from Thermo Fischer Scientific Pvt. Ltd (Mumbai, India).
Blend preparation
The excipients had lumps in them, so sieving was done to break those lumps up. For the blend preparation, lactose anhydrous and MCC were sifted through sieve #40 (0.425 mm nominal sieve opening) and mixed for 20 min. Then magnesium stearate and talc were sifted through sieve #60 (0.250 mm nominal sieve opening) and added to the above-mixed blend, followed by further mixing for 5 min. A similar procedure was followed for the lamivudine (API)-containing blend, except the API was not passed through the sieve.
Evaluation of the flow property of the blend for optimization of the lubricant and glidant concentration
Powders with good flow properties are vital for proper die-filling during compression and for the maintenance of the content uniformity. Hence, the flow of the powder blend was determined first by using the angle of repose, Carr's compressibility index, and Hausner ratio. Based on the results obtained, glidants and lubricants were added in a powder blend with a good flow behavior.
Angle of repose.
The angle of repose is one of the critical physical properties that is used to characterize the bulk of particulate materials, like powder or granules. When granular solids are piled on a flat surface, the sides of the pile are at a definite reproducible angle with the horizontal leveled surface. This angle is called the angle of repose of that material.11 The angle of repose assessment was performed using the funnel technique. For this, a 50 g powder blend was passed through a funnel and placed 4 inches above a flat bottom surface. Then, the height and diameter of the powder cone were measured to determine the angle of repose.12
Carr's compressibility index and Hausner ratio.
The Carr's compressibility index is used to measure the capability of powder to consolidate. In a free-flowing powder, interparticulate interactions are less significant, and the bulk and tapped densities are close to each other. In a poor-flowing material, where interparticle interactions are much higher, bridging between particles often results in a lower bulk density and a more significant difference between the bulk and tapped densities. These differences in particle interactions are reflected in Carr's compressibility index. Hausner's ratio is also very closely related to Carr's compressibility index. A higher value for both indicates poor flow, whereas a lower one indicates better flow. In this study, 50 g of powder was weighed and poured into a 250 mL volumetric cylinder, and the volume was noted as the bulk volume. Further, 100 tappings were given to the cylinder, and the volume after tapping was denoted as the tapped volume. From this, the bulk and tapped density of the powder blend were calculated. From the bulk and tapped density, Carr's compressibility index and Hausner ratio were calculated.13
Tablet preparation
Tablets can be prepared using three principal methods: DC, dry granulation, and wet granulation. In the DC method, all the materials are mixed and then compressed into tablets. In the dry granulation method, mechanical force densifies the powder and makes it compact to obtain dry granules. For dry granulation, either a slugging tooling can be used or a roller compactor/chilsonator. A binder solution is used in the wet granulation method, which forms a bond between the powder particles, thus forming a granule. In industry, research scientists will not directly go for manufacturing a big-size batch. During the pre-formulation stage, batches are manufactured by manual methods many times, where there will not be any impact on the tablet specifications. The manual method helps develop a prototype formulation in a very short time.
Tablet preparation using the wet granulation method.
For the preparation of placebo tablets, all the excipients were weighed as per the quantity listed in Table 1 and then were passed through sieve #40, except for magnesium stearate and talc, which were passed through sieve #60. Following this, the diluents and disintegrants were mixed for 25 min. The moisture content of the blend was determined using a moisture analyzer (H34B, Mettler-Toledo, Mumbai, India). Simultaneously, a 3% w/w PVP solution (in water and IPA) was prepared, and then this solution was added to the mixed blend to prepare the dough. Then this dough was passed through sieve #20 to obtain the granules. The granules were distributed into two equal portions. One portion of the granules was dried using the tray drying technique in the oven (105 °C for granules prepared by water, 60 °C for granules made by IPA). The second portion was dried using a FBD (TG200, Retsch) with a 70 °C inlet air temperature and airflow of 50 m3 h−1 for granules prepared using water, 45 °C inlet air temperature, and airflow of 30 m3 h−1 for granules prepared using IPA, till the moisture content became constant (1.7% w/w).
Table 1 Formulas for tablets prepared by the wet granulation technique
|
Ingredients |
Placebo tablets (%) |
10% drug (%) |
30% drug (%) |
50% drug (%) |
Intragranular material |
Lamivudine |
— |
10 |
30 |
50 |
Lactose anhydrous |
68.27 |
58.27 |
38.27 |
18.27 |
MCC |
15 |
15 |
15 |
15 |
PVP K30 |
0.73 |
0.73 |
0.73 |
0.73 |
Solvent |
Q.S. |
Q.S. |
Q.S. |
Q.S. |
Extragranular material |
MCC |
15 |
15 |
15 |
15 |
Magnesium stearate (0.5%) |
0.5 |
0.5 |
0.5 |
0.5 |
Talc (0.5%) |
0.5 |
0.5 |
0.5 |
0.5 |
Once the granules were dried, they were separated using a sieve shaker. A small quantity was taken from the granules obtained on sieves #40 and #60 to determine the hardness (granules were referred to as pre-compression granules) by using a texture analyzer (TA.XT. PLUS, Stable Micro Systems), and the remaining granules were mixed again. In these granules, extragranular materials were added and mixed for 5 min. Then this powder blend was compressed into 350 mg tablets using a tablet compression machine (KMPC-08, Kambert). Also, ‘D-tooling’ round flat-faced punches (10 mm diameter) were used to compress the tablets. The filling depth was adjusted so that the tablet weight was 350 mg, and the hardness was set through the thickness valve. In the Kambert compression machine, hardness is changed by regulating the thickness valve present on the left side of the compression machine. Rotating the thickness by adjusting the valve in a clockwise direction decreases the thickness because the machine puts greater force on the blend, making a hard, compact tablet. Similarly, if the valve is rotated in an anti-clockwise direction, the machine will put comparatively less force on the blend, making a less hard tablet, which will be thicker than the tablet having more hardness. So, the thickness and hardness are inversely proportional to each other if the tablet weight is constant. Similarly, tablets were also compressed for the granules prepared using RMG (HSMG-10, Kevin) with an impeller speed 100 rpm and chopper speed of 1000 rpm, for 25 min, at 70% occupancy in a 2-liter bowl for the mixing and granulation.
Tablet preparation by dry granulation.
In the dry granulation method, the slugging technique was used. For placebo tablets, all the excipients were weighed as per the quantity listed in Table 2 and then passed through sieve #40, except for magnesium stearate and talc, which were passed through sieve #60. Then the diluent and disintegrant were mixed for 25 min. Then big tablets (slugs) were prepared in the tablet compression machine (KMPC-08, Kambert). Also, ‘B-tooling’ round flat-faced punches (12 mm diameter) were used to compress the tablets. The filling depth was adjusted so that the weight of the tablet was 550 mg, and the hardness (5–7 kiloponds) was set through the thickness valve. These slugs were broken down using a mortar and pestle to obtain granules. Then, extragranular materials were added and mixed for 5 min, followed by compression in the tablet compression machine (KMPC-08, Kambert). Also, ‘D-tooling’ round flat-faced punches (10 mm diameter) were used to compress the tablets. The filling depth was adjusted so that the weight of a tablet was 350 mg, and the hardness was set through the thickness of the valve. The quantity of binder was calculated by reverse calculating the amount of binder solution used to prepare tablets using the wet granulation technique.
Table 2 Formulas for tablets prepared by the dry granulation technique
|
Ingredients |
Placebo tablets (%) |
10% drug (%) |
30% drug (%) |
50% drug (%) |
Intragranular material |
Lamivudine |
— |
10 |
30 |
50 |
Lactose anhydrous |
68.27 |
58.27 |
38.27 |
18.27 |
MCC |
15 |
15 |
15 |
52.5 |
PVP K30 |
0.73 |
0.73 |
0.73 |
2.56 |
Extragranular material |
MCC |
15 |
15 |
15 |
52.5 |
Magnesium stearate (0.5%) |
0.5 |
0.5 |
0.5 |
1.75 |
Talc (0.5%) |
0.5 |
0.5 |
0.5 |
1.75 |
Tablet preparation by direct compression.
All the excipients were weighed for placebo tablets as per the quantity mentioned in Table 3 and passed through sieve #40, except for magnesium stearate and talk, which were passed through sieve #60. Then the diluent and disintegrant were mixed for 25 min, followed by the addition of the lubricant and glidant and mixing for 5 more min. After this, the powder blend was compressed into 350 mg tablets using a tablet compression machine (KMPC-08, Kambert). Also, ‘D-tooling’ round flat-faced punches (10 mm diameter) were used to compress the tablets. The filling depth was adjusted so that the weight of the tablet was 350 mg, and the hardness was set through the thickness of the valve. The quantity of binder was calculated by reverse calculating the amount of binder solution used to prepare the tablets using the wet granulation technique.
Table 3 Formulas for tablets prepared by the direct compression technique
Ingredients |
Placebo tablets (%) |
10% drug (%) |
30% drug (%) |
50% drug (%) |
Lamivudine |
— |
10 |
30 |
50 |
Lactose anhydrous |
68.27 |
58.27 |
38.27 |
18.27 |
MCC |
30 |
30 |
30 |
30 |
PVP K30 |
0.73 |
0.73 |
0.73 |
0.73 |
Magnesium stearate (0.5%) |
0.5 |
0.5 |
0.5 |
0.5 |
Talc (0.5%) |
0.5 |
0.5 |
0.5 |
0.5 |
Drug-containing tablets were also prepared by following all the above three processes but replacing the amount of diluent with the added drug. The drug was added at three levels, i.e., 10%, 30%, and 50% of the total tablet weight.
Evaluation of the pre-compression granules for their hardness
The main aim of this experiment was to identify the effect of the drying process on the hardness of granules. First, the prepared granules were separated based on their particle size by sieve analysis. All the sieves were first washed and dried. The sieves were arranged by keeping a collecting pan at the bottom, followed by sieves #100, #80, #60, #40, and #20 at the top. The granules were placed on sieve #20, and the sieves were covered with a lid. The instrument was operated at a power setting of 20 for 10 min. Then the granules retained on sieves #40 and #60 were taken, and their hardness was checked using a texture analyzer (TA.XT. PLUS, Stable Micro Systems) by following the below-mentioned protocol.
Sample preparation.
A strip of double-sided tape with dimensions of approximately 55 mm × 150 mm was cut and placed over a plane and clean surface. Then the granules were poured over it, the tape was lifted, and gentle shaking was performed to form a uniform and single layer of granules on the tape. The parameters were set as follows: test speed 0.1 mm s−1, post-test speed 10.0 mm s−1, distance 2.5 mm. The probe (P/25) was then calibrated against the base with a return distance of 3 mm. The prepared tape was placed under the investigation, and a compression test was commenced.
Evaluation of the tablets
Weight variation.
For the weight variation analysis, 20 tablets were weighed individually, and the minimum and maximum weights were noted. As the average tablet weight was 350 mg, as per USP, a 5% weight variation was allowed.
Friability.
The friability of the prepared tablets was checked by using a Roche friabilator (EF-2, Electrolab). The tablets were dedusted properly. After this, the tablets were weighed till their weight reached 6.5 g. The weighed tablets were then put into the friabilator, and the friabilator was operated at a speed of 25 rotations per minute (rpm) for 4 min. Then, the tablets were dedusted and weighed again to check for any weight loss. The tablets were also visually observed for any cracks. Principally, the % friability or % weight loss should be less than 1%. The % friability was calculated.14
Tablet fracture evaluation observed under SEM.
Tablets were broken from the middle into two pieces manually. Then, they were made flat from the curve side by rubbing over sandpaper. The tablets prepared were round in shape. When a round tablet is broken into two halves, two 3D semicircular tablets are obtained. The new surface that appears after breaking the tablet must be observed under SEM. The broken tablet should be placed on the sample holder of the SEM device so that the newly formed surface should face upward. Being a semicircle, it is difficult for the tablet to remain stationary on the sample holder as the sample holder has a flat surface. Hence, sand paper is used to scrap the semicircular portion to make it flat without touching the newly appeared surface so that the sample can be easily placed on the flat surface of the sample holder. The tablets were then stuck to carbon tape from the rubbed end, followed by gold coating for 10 min at 10 mA current. Then the fracture was observed under SEM (Sigma 300, Zeiss).
Crushing the tablets to obtain post-compression granules
The prepared tablets were crushed to obtain granules; these granules are referred to as post-compression granules. The tablets were crushed using a texture analyzer (TA.XT. PLUS, Stable Micro Systems). The probe P/25 was attached to the texture analyzer, and the sample of tablet hardness was loaded. The probe was raised 30 mm up from the base. The tablet was kept diametrically on the platform, and the test was commenced. The test distance was kept at 28 mm so that the tablet could be crushed into granular/powdered material.
Evaluation of the post-compression granules
Sieve analysis.
All the sieves were first washed, dried, and weighed. The sieves were then arranged by keeping the collecting pan at the bottom, followed by sieve #100, #80, #60, #40, and at the top sieve #20. Granules were placed on sieve #20, and the lid was applied. The sieve shaker (EMS-8, Electrolab) was operated at a power of 20 for 10 min. After 10 min, the instrument was stopped, and the sieves containing the granules were weighed.
Hardness.
A similar procedure was followed as that for measuring the hardness of the pre-compression granules. Then the hardness of pre- and post-compression granules was compared to determine whether there was any relationship between the patterns of the hardness of the granules when the drying process was changed.
Results and discussion
Evaluation of the flow property of the blend for optimization of the lubricant and glidant concentration
The angle of repose, Carr's compressibility index, and Hausner ratio for the powder blend before lubrication inferred that the flow was poor. Hence, to increase the flow property of the powder blend, lubricant (0.5%) and glidant (0.5%) were added. After lubrication, it was observed that there was an enhancement in the flow behavior of the blend, because it lowered the friction at the die-wall-to-tablet interface, minimized the probability of the punch sticking, as well as safeguarded the tablet tooling. As shown in Table 4, the flow property of the powder blend before lubrication was inadequate, but after lubrication, it was good.
Table 4 Flow properties of powder blends before and after lubrication
Parameters |
Before lubrication |
After lubrication (addition of 0.5% talc and 0.5% magnesium stearate) |
Mass (g) |
50 ± 0.50 |
50 ± 0.55 |
Angle of repose (°) |
45.2 ± 1.56 |
32.1 ± 1.25 |
Bulk density (g mL−1) |
0.62 ± 0.02 |
0.76 ± 0.02 |
Tapped density (g mL−1) |
0.86 ± 0.02 |
0.90 ± 0.03 |
Carr's compressibility index |
27.90 ± 0.45 |
15.55 ± 0.25 |
Hausner ratio |
1.38 ± 0.05 |
1.18 ± 0.04 |
Flow |
Poor |
Good |
Evaluation of the tablets
Tablets were evaluated for a range of physical parameters, such as hardness and friability. These parameters were within their acceptable criteria (weight variation < 5% and friability < 1%).
Weight variation.
As per the USP, all the batches of tablets were in a 5% deviation range from the mean value.15 The average weight of 20 tablets was 350 mg and the 5% deviation range for the 350 mg tablet was 332.5–367.5 mg. As shown in Table 5, all the batches of tablets were in the weight variation range, i.e., <5%, as specified by USP. These findings implied that throughout the preparation and manufacturing phases, the powder blend maintained its homogeneity.
Table 5 Weight variation of prepared tablets
Method |
Solvent used |
Dryer type |
Drug% |
Average weight (mg) |
% difference |
Result |
Direct compression |
— |
— |
— |
351.65 |
4.14 |
Pass |
10% |
348.30 |
4.31 |
Pass |
30% |
350.55 |
2.99 |
Pass |
50% |
350.75 |
1.57 |
Pass |
Dry granulation |
— |
— |
– |
350.25 |
4.86 |
Pass |
10% |
350.50 |
2.21 |
Pass |
30% |
351.35 |
4.26 |
Pass |
50% |
352.75 |
3.98 |
Pass |
Wet granulation |
Water |
Tray |
— |
351.70 |
4.24 |
Pass |
10% |
350.55 |
3.86 |
Pass |
30% |
351.85 |
2.64 |
Pass |
50% |
351.00 |
1.23 |
Pass |
FBD |
— |
351.35 |
2.15 |
Pass |
10% |
348.75 |
2.82 |
Pass |
30% |
355.80 |
2.67 |
Pass |
50% |
350.25 |
1.61 |
Pass |
IPA |
Tray |
— |
351.60 |
2.62 |
Pass |
10% |
349.85 |
1.61 |
Pass |
30% |
351.95 |
1.91 |
Pass |
50% |
350.00 |
3.17 |
Pass |
FBD |
— |
351.65 |
3.51 |
Pass |
10% |
349.90 |
3.70 |
Pass |
30% |
353.80 |
4.21 |
Pass |
50% |
349.25 |
3.46 |
Pass |
Friability.
The friability test was performed on the prepared tablets to check their strength. The friability test is closely related to tablet hardness and is designed to evaluate the mechanical strength of tablets. As per the pharmacopeia, the % friability should be less than 1%.15 All the prepared tablet batches passed the friability test, as shown in Table 6, and no single tablet was cracked or broken. In comparison to all methods, DC methods show maximum friability, because the powder particles could have weaker interparticulate bonding, which increases the risk of tablet breaking, whereby a large variety of shapes and sizes of particles may result in inconsistent packing and weakened tablet architectures. This indicates that the tablets had enough strength to withstand abrasion in packaging, handling, and shipping.
Table 6 Percent friability of the prepared tablets
Granulation method |
Solvent used |
Dryer type |
Drug% |
Initial weight (g) |
Final weight (g) |
% friability |
Direct compression |
— |
— |
— |
6.719 ± 0.019 |
6.700 ± 0.026 |
0.293 ± 0.157 |
10% |
6.830 ± 0.121 |
6.773 ± 0.115 |
0.820 ± 0.071 |
30% |
6.546 ± 0.040 |
6.506 ± 0.045 |
0.611 ± 0.154 |
50% |
6.514 ± 0.005 |
6.463 ± 0.015 |
0.777 ± 0.178 |
Dry granulation |
— |
— |
— |
6.754 ± 0.027 |
6.732 ± 0.031 |
0.325 ± 0.107 |
10% |
6.541 ± 0.024 |
6.516 ± 0.015 |
0.376 ± 0.140 |
30% |
6.533 ± 0.023 |
6.510 ± 0.026 |
0.357 ± 0.089 |
50% |
6.636 ± 0.015 |
6.615 ± 0.021 |
0.326 ± 0.242 |
Wet granulation |
Water |
Tray |
— |
6.626 ± 0.011 |
6.609 ± 0.010 |
0.261 ± 0.069 |
10% |
6.724 ± 0.014 |
6.696 ± 0.020 |
0.406 ± 0.164 |
30% |
6.714 ± 0.008 |
6.697 ± 0.007 |
0.248 ± 0.042 |
50% |
6.822 ± 0.007 |
6.810 ± 0.018 |
0.175 ± 0.165 |
FBD |
— |
6.798 ± 0.005 |
6.771 ± 0.012 |
0.397 ± 0.169 |
10% |
6.736 ± 0.006 |
6.706 ± 0.018 |
0.437 ± 0.192 |
30% |
6.620 ± 0.011 |
6.570 ± 0.010 |
0.760 ± 0.073 |
50% |
6.542 ± 0.002 |
6.517 ± 0.002 |
0.371 ± 0.008 |
IPA |
Tray |
— |
6.732 ± 0.006 |
6.722 ± 0.007 |
0.158 ± 0.047 |
10% |
6.757 ± 0.006 |
6.748 ± 0.003 |
0.132 ± 0.063 |
30% |
6.554 ± 0.003 |
6.541 ± 0.006 |
0.371 ± 0.008 |
50% |
6.751 ± 0.002 |
6.731 ± 0.001 |
0.296 ± 0.025 |
FBD |
— |
6.681 ± 0.007 |
6.668 ± 0.005 |
0.199 ± 0.127 |
10% |
6.657 ± 0.001 |
6.646 ± 0.004 |
0.162 ± 0.044 |
30% |
6.626 ± 0.007 |
6.613 ± 0.006 |
0.206 ± 0.067 |
50% |
6.761 ± 0.003 |
6.739 ± 0.005 |
0.335 ± 0.081 |
Tablet fracture evaluation under SEM.
The fractured surface of the tablets was observed under SEM to determine if any characteristic feature could be observed when there is any change in the manufacturing process, granulation solvent, or drying technique. The surface characteristics of the tablet, including texture, roughness, and the existence of layers or coatings, can be seen using SEM. Distinct surface impressions are left by various manufacturing processes. For example, tablets made via wet granulation may have grains or agglomerates on the surface, whereas tablets made by direct compression usually have a smoother surface. Cross-sectional SEM imaging offers an insight into a tablet's interior composition. Different internal architectures are produced by different production processes. For example, tablets made via wet granulation may show discrete granule layers, whereas tablets made by direct compression usually have a homogenous interior structure. SEM is able to produce fine-grained pictures of specific tablet formulation particles. Analysts can deduce the manufacturing process utilized to create a tablet's particles by examining their morphology. When used in conjunction with other spectroscopy techniques, SEM can determine the elemental makeup of particular tablet sections. Through an examination of the tablet matrix's elemental composition, analysts can deduce the kinds of excipients utilized and, in turn, the manufacturing process that was implemented.
In the case of the placebo tablet, it could be observed that the surface of the A, B, C, D tablets prepared by wet granulation was not clean, as the water was used for the preparation of the granulating solution. When this solution comes in contact with the water-soluble excipients (lactose, MCC) present in the blend, these excipients get solubilized and form a layer/coating on other excipients. Such a coating of excipients by other excipients was not observed in the case where IPA was used to prepare a granulating solution. The excipients were visible on the E, F, G, and H tablets. So, this was one of the characteristic features that confirmed which solvent could be used to prepare the granulating solution. No distinguishing feature was observed in the DC process. In the case of the dry granulation technique, as in image J, the tablet fracture showed many cracks, and these cracks developed due to the two-times compression, one during slugging and the second during the actual punching of the tablets (Fig. 1).
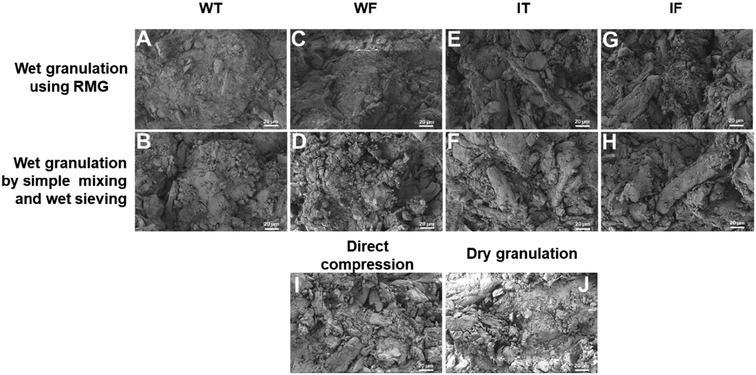 |
| Fig. 1 SEM images of placebo tablets fracture. WT – water was used as a solvent for preparation of the binder solution and the granules were dried by a tray drying technique, WF – water was used as a solvent for preparation of the binder solution and the granules were dried in FBD, IT – IPA was used as a solvent for preparation of the binder solution and the granules were dried by a tray drying technique, and IF – IPA was used as a solvent for preparation of the binder solution and the granules were dried in a FBD. (A–H) Wet granulation technique was used for manufacturing the tablets; (I) direct compression technique was used; and (J) dry granulation technique was used. Scale bar represents 20 μm. | |
All the API-containing tablets were prepared by the wet granulation technique and wet sieving method. There was no difference observed in the SEM images of the tablet fracture prepared by RMG and by simple mixing followed by wet sieving. For dry granulation and DC, blend mixing was done. Irrespective of the drug content, similar results were observed for the drug-containing tablets (Fig. 2) as for the placebo tablets. In the case of wet granulation, the SEM images were distinguishable based on the solvent utilized, i.e., water and IPA. In the case of the DC process, the layering pattern was observed in the tablets because of DC, one during slugging and the other during the actual compression.
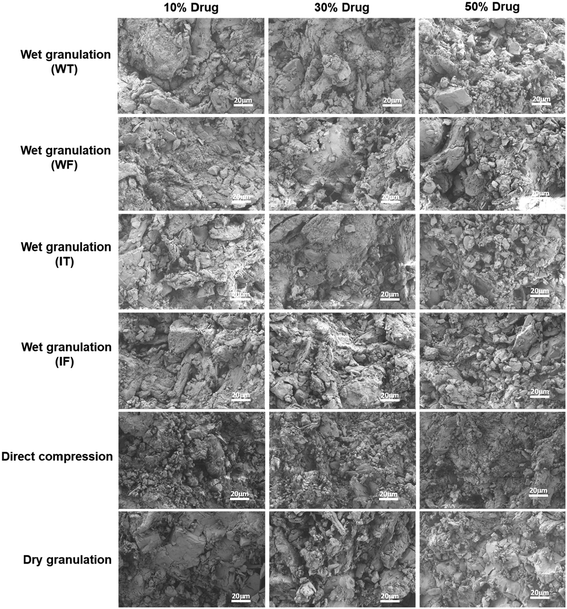 |
| Fig. 2 SEM images of API-containing tablets fracture. WT – water was used as a solvent for preparation of the binder solution and the granules were dried by a tray drying technique, WF – water was used as a solvent for preparation of the binder solution and the granules were dried in FBD, IT – IPA was used as a solvent for preparation of the binder solution and the granules were dried by a tray drying technique, and IF – IPA was used as a solvent for preparation of the binder solution and the granules were dried in a FBD. Scale bar represents 20 μm. | |
Sieve analysis of the post-compression granules
Sieve analysis can offer valuable details regarding the distribution of the particle and granule sizes, blend homogeneity, agglomerate presence, and general material quality during the manufacturing process. Analysts can deduce the manufacturing technique utilized and enhance tablet production procedures to enhance uniformity and caliber by examining these variables. The primary goal of sieve analysis is to determine the proportions of various drug particle sizes present.16 Sieve analysis of post-compression granules was done here by crushing the tablet using a texture analyzer to check which method generated the maximum amount of fines. The amount of granules/powder retained on each sieve was calculated by taking the difference in weight in the sieve before and after the sieve analysis. The percentage of granules/powder obtained on each sieve and in the collecting pan was calculated (Table 7). The granules/powder collected in the collecting pan were termed fines. Table 7 shows that the maximum fines were generated in the post-compression granules obtained by crushing the tablets prepared by the DC process. This may be because, in the case of DC, the excipient's size remains unchanged. In the case of the granulation technique, the excipients come together and form a bulk called a granule, due to granulation. The granules were found to be bigger than the parent excipients; hence, the maximum amount of fines was observed in the DC method by sieve analysis of the post-compression granules.
Table 7 Percentage fines obtained after sieve analysis of post-compression granules
Granulation |
Solvent used |
Drying condition |
Placebo |
10% drug |
30% drug |
50% drug |
Wet granulation |
Water |
Tray |
13.12 ± 1.83 |
19.28 ± 1.26 |
23.81 ± 2.41 |
24.36 ± 1.92 |
Water |
FBD |
20.20 ± 1.20 |
22.36 ± 1.34 |
20.69 ± 1.79 |
21.28 ± 2.38 |
IPA |
Tray |
18.05 ± 1.52 |
21.34 ± 1.68 |
19.84 ± 1.67 |
21.92 ± 1.64 |
IPA |
FBD |
29.14 ± 1.34 |
30.12 ± 2.41 |
28.62 ± 1.68 |
32.27 ± 1.95 |
Dry granulation |
— |
— |
14.81 ± 1.36 |
28.82 ± 1.63 |
25.47 ± 1.42 |
28.96 ± 1.37 |
Direct compression |
— |
— |
37.92 ± 2.31 |
38.36 ± 1.35 |
36.34 ± 1.33 |
38.21 ± 1.67 |
Hardness of pre- and post-compression granules
The hardness of the pre- and post-compression granules was compared to find out the change in the hardness of the granules when solvent was used to prepare the granulating solution and the drying technique was changed. The texture analyzer was used to break the tablets by keeping the working distance of the texture analyzer arm constant for each sample. When force is applied by the moving arm of the texture analyzer on the stationary tablet, the table is crushed into granular material. The hardness of this granular material was analyzed using a texture analyzer, and the same working distance was kept for each sample. When the texture analyzer arm covers a set distance toward the sample, it exerts force to break the granules, and this force was recorded as a measure of the hardness.
From Fig. 3A, in the case of the granules prepared using RMG and retained on sieve #40, the pre- and post-compression hardness values for the granules prepared by water and dried in a tray (WT) were 4.185 ± 0.147 kg and 4.721 ± 0.152 kg respectively. In contrast, for the granules prepared by water and dried in FBD (WF), their pre- and post-compression hardness values were 3.694 ± 0.070 kg and 4.266 ± 0.030 kg, respectively. The difference in the pre-compression granule hardness of the WT and WF granules was approximately 0.5 kg, and for post-compression it was also 0.5 kg. This indicated that there was a linear increase in the hardness after compression. However, the hardness of the WT granules was 0.5 kg more than that of the WF granules irrespective of pre- or post-compression because FBD produces more porous granules than tray drying, and the hardness of the porous granules remains less than that of the non-porous granules. The pre- and post-compression hardness for the granules prepared by IPA and dried in a tray (IT) was 3.541 ± 0.225 kg and 4.067 ± 0.121 kg, respectively, whereas the pre- and post-compression hardness values for the granules prepared by IPA and dried in FBD (IF) were 2.906 ± 0.167 kg and 3.409 ± 0.143 kg, respectively. The difference in the pre-compression and post-compression granule hardness of the IT and IF granules was approximately 0.6 kg, which indicated a linear increase in the hardness after compression. However, the hardness of the IT granules was 0.6 kg more than that of the IF granules irrespective of pre- or post-compression.
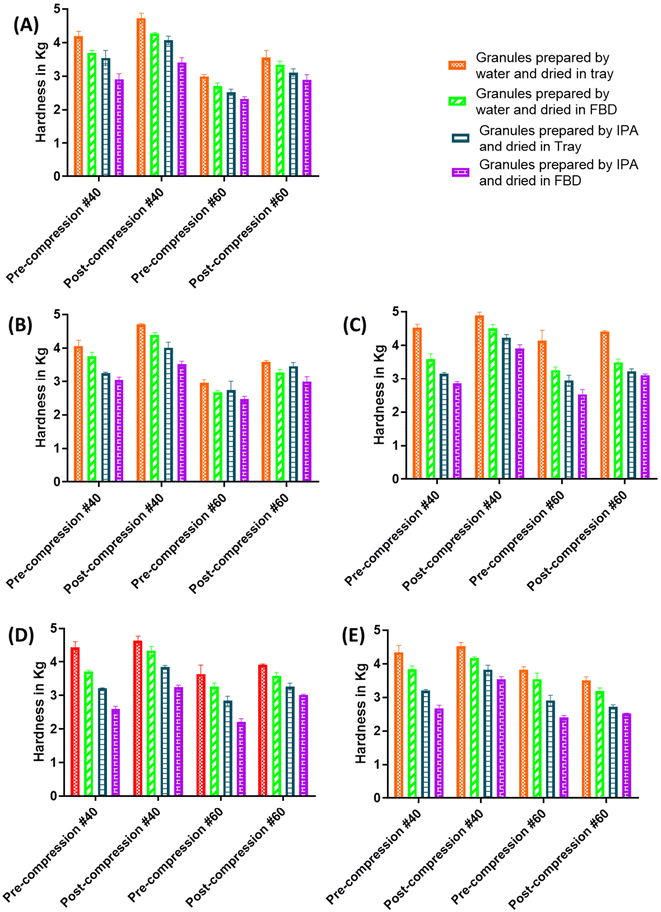 |
| Fig. 3 Hardness of the pre- and post-compression granules retained on sieve no. 40 and 60. (A) Placebo granules prepared in RMG, (B) placebo granules prepared by simple mixing and wet sieving, (C) 10% API-containing granules prepared by a simple mixing and wet sieving, (D) 30% API-containing granules prepared by a simple mixing and wet sieving, and (E) 50% API-containing granules prepared by a simple mixing and wet sieving. | |
Similarly, for the granules retained on sieve #60, the hardness of the WT granules was 0.2 kg more than that of the WF granules, and the hardness of the IT granules was 0.2 kg more than that of the IF granules. Similar results were observed for the placebo and API granules prepared by simple mixing and wet sieving (Fig. 3A–C). From Fig. 3, it can be observed that the granules prepared using water were harder than those prepared using IPA, while the tray-dried granules were harder than the FBD-dried granules, irrespective of the wet granulation technique.
The above results indicate that the granules prepared using water were harder than those prepared using IPA, because the excipients used for the granules preparation were water-soluble, which helps in the proper binding of the excipients with each other. Also, previous reports demonstrated that water forms an irreversible hydrogen bonding with excipients.17 Researchers have also hypothesized that these differences in strength are due to the conversion of some intramolecular hydrogen-bonded amorphous fibrils at the surface of MCC particles to intermolecular hydrogen-bonded fibrils with other MCC particles.18 It was also observed that the change in strength could also be due to internal hydrogen bonding and C bonding.17 The granules prepared using the tray drying technique were harder than those prepared using FBD. This hardness pattern was observed in all the cases of the granules (from simple mixing and wet sieving, RMG). Researchers have carried out studies on granules prepared by different drying techniques and confirmed that using FBD as a drying technique results in porous granules.19
Comparing the hardness of the pre- and post-compression granules, the hardness of post-compression granules increased due to the compression force applied during tablet compression. However, the characteristic pattern, i.e., tray-dried granules were harder than the FBD-dried granules, remained the same (Fig. 3). Therefore, the hardness of FBD-dried granules was lower because of their porous nature compared to that of the tray-dried granules. Measuring the granule hardness with a texture analyzer thus provides valuable information about the tablet production process, including the difference between wet granulation and direct compression, optimization of the binder content and compression strength, identification of the compression profiles, particle size distribution, and process optimization. Analysts can infer the manufacturing method used and optimize tablet manufacturing processes to improve consistency and quality by analyzing grain hardness measurements along with other process parameters. However, the prototype of each combination should be ready so that the results of the tablet whose manufacturing process needs to be identified can be compared with the results of tablets with known manufacturing processes.
Conclusion
By observing the fracture pattern under SEM, the process and type of solvent used to fabricate a tablet can be distinguished provided that the properties of the ingredients present in it are known. If ingredients are soluble in water, they can form a layer over other excipients and the drug, but being insoluble in IPA, remains per se. Here, sieve analysis was employed to distinguish the DC process from the granulation technique, as the amount of fines generated from post-compression granules is the maximum in DC compared to granulation techniques. The results obtained from the texture analyzer for the hardness of the granules conclude that the FBD-dried granules were less hard as they were porous compared to tray-dried granules. The information reported in this work may serve as a guiding principle for formulators working toward developing a generic version of a tablet following the reverse engineering process.
Abbreviations
API | Active pharmaceutical ingredient |
cm | Centimeter |
DC | Direct compression |
DG | Dry granulation |
FBD | Fluidized bed dryer |
g | Gram |
IF | Granules prepared by IPA and dried in FBD |
IPA | Isopropyl alcohol |
IT | Granules prepared by IPA and dried in tray |
mL | Milliliter |
Q.S. | Quantity sufficient |
RMG | Rapid mixer granulator |
SEM | Scanning electron microscopy |
USP | United States Pharmacopeia |
WF | Granules prepared by water and dried in FBD |
WT | Granules prepared by water and dried in tray |
Conflicts of interest
The authors disclose no conflict of interest.
Acknowledgements
The research was carried out at the National Institute of Pharmaceutical Education and Research Ahmedabad with financial support from the Department of Pharmaceuticals, Ministry of Chemicals and Fertilizers, Government of India. R. K. T. would like to acknowledge the Science and Engineering Research Board (Statutory Body Established through an Act of Parliament: SERB Act 2008), Department of Science and Technology, Government of India, for a grant (ECR/2016/001964) and Core Research grant funding (CRG/2021/005402) in Dr Tekade's laboratory. R. K. T. would also like to acknowledge the Indian Council of Medical Research (ICMR), New Delhi, for grant file ID: 2021-14161 and grant file ID: IIRP-2023-4849/F1 for supporting research in the R. K. T. lab.
References
-
R. Virmani, T. Virmani and Kerur, Pharmaceutics1, 2017 Search PubMed.
- M. Kottke and E. Rudnic, Modern Pharmaceutics, 2002 DOI:10.1201/9780824744694.ch10.
-
B. Mittal, How to Integrate Quality by Efficient Design (QbED) in Product Development, Academic Press, 2020, pp. 27–57. DOI:10.1016/B978-0-12-816813-4.00002-7.
- F. Khan, J. Innovations Pharm. Biol. Sci., 2016, 3, 17–25 Search PubMed.
- A. K. Bansal, Pharm. Technol., 2005, 29, 50–55 Search PubMed.
- B. Rojek and M. Wesolowski, J. Therm. Anal. Calorim., 2019, 138, 4531–4539 CrossRef CAS.
- A. Segall, J. Innovations Appl. Pharm. Sci., 2019, 4, 1–6 Search PubMed.
- O. R. Klisurica, S. J. Rakica, M. J. Cvetinov, M. M. Stojanovic, A. R. Nikolic, S. S. Jovanovic Santa, A. R. Markovic and L. N. Solomun, J. Res. Phys., 2013, 37, 55 Search PubMed.
- M. Šimek, V. Grünwaldová and B. Kratochvíl, BioMed Res. Int., 2014, 2014, 832452 CrossRef PubMed.
- T. Warad, V. Karbhari and S. Dhanasure, Indo Am. J. Pharm. Res., 2018, 8, 1671–1675 Search PubMed.
-
T. F. Teferra, in Handbook of Farm, Dairy and Food Machinery Engineering, ed. M. Kutz, Academic Press, 3rd edn, 2019, pp. 45–89. DOI:10.1016/B978-0-12-814803-7.00003-8.
- B. Dhakal, J. K. Thakur, R. K. Mahato, I. Rawat, D. C. Rabin, R. R. Chhetri, K. P. Shah, A. Adhikari and J. Pandey, Sci. World J., 2022, 2022, 9618344 CrossRef PubMed.
- N. Erdoğar, Turk. J. Pharm. Sci., 2021, 18, 589–596 CrossRef PubMed.
- H. Zhao, Y. Yu, N. Ni, L. Zhao, X. Lin, Y. Wang, R. Du and L. Shen, Int. J. Pharm., 2022, 611, 121339 CrossRef CAS PubMed.
-
G. o. I, Indian Pharmacopoeia Commission, 2018, II, 1924–1925 Search PubMed.
- C. O. Migoha, M. Ratansi, E. Kaale and G. Kagashe, BioMed Res. Int., 2015, 2015, 307032 CAS.
- G. E. Reier and R. F. Shangraw, J. Pharm. Sci., 1966, 55, 510–514 CrossRef CAS.
- S. Westermarck, A. M. Juppo, L. Kervinen and J. Yliruusi, Eur. J. Pharm. Biopharm., 1999, 48, 199–206 CrossRef CAS PubMed.
- Á. Hegedűs and K. Pintye-Hódi, Int. J. Pharm., 2007, 330, 99–104 CrossRef PubMed.
|
This journal is © The Royal Society of Chemistry 2024 |
Click here to see how this site uses Cookies. View our privacy policy here.