DOI:
10.1039/D4OB00984C
(Communication)
Org. Biomol. Chem., 2024,
22, 5718-5723
Phosphine-promoted intramolecular Rauhut–Currier/Wittig reaction cascade to access (hetero)arene-fused diquinanes†
Received
12th June 2024
, Accepted 20th June 2024
First published on 21st June 2024
Abstract
We describe the first phosphine-promoted intramolecular Rauhut–Currier reaction that triggers an intramolecular Wittig process assembling new classes of diquinanes. The one-pot strategy provides ready access to simple diquinanes and various (hetero)arene-fused diquinanes incorporated with up to two contiguous all-carbon quaternary centers under metal-free and neutral conditions. We showcased the generality of the method on a broad range of substrates and demonstrated its synthetic utility in accessing various advanced intermediates relevant to natural product synthesis and material science.
Benzannulated diquinanes (cyclopenta[a]indenes) are the key structural elements of many bioactive natural products and medicinally important molecules (Fig. 1).1 Therefore, several synthetic protocols have been developed to synthesize cyclopenta[a]indene derivatives.2 While most of them are metal-promoted strategies, the only metal-free approach to our knowledge is Ghorai's work on constructing benzannulated diquinanes employing a tandem iminium and enamine catalysis.3 Here, we report the first organophosphine-promoted Rauhut–Currier/Wittig reaction sequence to synthesize complex diquinane analogues.
 |
| Fig. 1 Representative bioactive natural products possessing cyclopenta[a]indene core. | |
The Rauhut–Currier (RC) reaction is a versatile carbon–carbon bond-forming reaction between two electron-deficient olefins promoted by an organic nucleophilic base.4 Since the RC reaction is atom-economic and organocatalytic, it has extensive applications in accessing diverse classes of highly functionalized molecules.5 Recently, several Lewis base-promoted intramolecular cascade transformations triggered by the RC reaction have been developed to create various cyclic structures.4,6 However, to our knowledge, the RC reaction is yet to be explored in assembling an valuable diquinane scaffold. This manuscript describes an efficient approach to simple diquinanes and (hetero)arene-fused diquinanes and demonstrates its utility in preparing some advanced intermediates relevant to natural product synthesis.
To assemble cyclopenta[a]indenes by incorporating the mechanistic features of the RC reaction, the tethered bis-enone A was designed in such a way that the phospha-Michael addition preferentially occurs to the β-unsubstituted enone leading to the formation of the zwitterionic intermediate B, Scheme 1. The role of R1 is to discourage a cross-RC reaction since activated α- and β-unsubstituted olefins tend to dimerize or oligomerize under the RC conditions. The enolate-mediated displacement of phosphine could generate dihydroindeno[2,1-c]pyran-9-one C. On the other hand, we expected that a proton transfer event might generate the phosphorous ylide D, which is poised to undergo an intramolecular Wittig reaction to deliver the benzo-fused diquinane E. At this stage, we do not rule out the phospha-Michael addition to the β-substituted enone generating the zwitterionic species F, a subsequent 1,3-proton transfer and an enolate-induced elimination of phosphine providing benzo-fused cycloheptenone G.
 |
| Scheme 1 Substrate design for cyclopenta[a]indenes and the possible mechanistic scenarios with a phosphine. | |
Although the substrate design (A) allows different mechanistic pathways leading to C, E or G, we prepared the bis-enone 7a to ascertain the preferred product under experimental conditions, Table 1. Since no reaction was observed under catalytic conditions, we exposed 7a to stoichiometric amounts of PPh3 and PPh2Et, but the starting material remained as such (entries 1 and 2). Interestingly, a more nucleophilic trialkylphosphine such as PCy3 gave 8a over the other expected products, although in poor yields (entries 3–5). However, better results were achieved with PBu3 as the Lewis base. An initial reaction of 7a with PBu3 in DMF at room temperature delivered 8a in a good yield (entry 6). During the solvent screening, we realized that the yield of 8a improved in tert-butanol medium (entries 7–9). A few variations in the temperature with PBu3 as the Lewis base and tert-butanol as the solvent gave 8a in an excellent yield (entries 10–12). We were delighted that we could establish the first synthesis of benzo-fused diquinanes, such as 8a, under the RC set up.
Table 1 Optimization of reaction parametersa,b

|
Entry |
PR3 (1.2 equiv.) |
Solvent |
Temperature (°C) |
Time (h)/yieldc (%) |
See the ESI† for a detailed procedure.
The reactions were performed on a 0.1 mmol scale.
Isolated yields after column chromatography.
|
1 |
PPh3 |
DMF |
30 |
48/— |
2 |
PPh2Et |
DMF |
30 |
48/— |
3 |
PCy3 |
DMF |
30 |
2/28 |
4 |
PCy3 |
Toluene |
30 |
2/14 |
5 |
PCy3 |
MeCN |
30 |
2/32 |
6 |
PBu3 |
DMF |
30 |
1/64 |
7 |
PBu3 |
MeCN |
30 |
2/56 |
8 |
PBu3 |
DMSO |
30 |
1/62 |
9 |
PBu3 |
t-BuOH |
30 |
2/73 |
10 |
PBu3 |
t-BuOH |
50 |
2/78 |
11 |
PBu3 |
t-BuOH |
70 |
1/87 |
12 |
PBu3 |
t-BuOH |
80 |
1/81 |
Encouraged by the result, we evaluated the generality of the method under optimized conditions, Scheme 2. An array of benzannulated diquinanes (8b–8aa) and a heteroarene-fused diquinane (8ab) were assembled in good yields. The reaction fared well across a wide range of substrates irrespective of the electronic or steric nature of R1, R2 or R3. Different types of alkyl groups can be accommodated at R1 (8b–8g, 8k, 8l, 8y, 8z and 8aa), and various kinds of electron-donating (methyl, and mono-, di- and trimethoxy) and marginally electron-withdrawing (fluoro) substituents were well-tolerated at R2. Apart from the phenyl backbone, substrates with naphthyl (8l, 8n and 8o) and a benzothiophene backbone (8ab) were prepared in moderate to good yields. We could conveniently prepare a diverse range of cyclopenta[a]indenes possessing non-aryl (8p and 8q), aryl groups (8i, 8o, 8r–8v, 8y–8aa), and heteroaryl substituents (8w and 8x) at R3. The structure of a representative example (8m) was confirmed by the single crystal X-ray diffraction analysis (CCDC 2325678†). The poor yields in case of 8p and 8ab are attributed to the partial decomposition of the respective starting compounds leading to unidentifiable products.
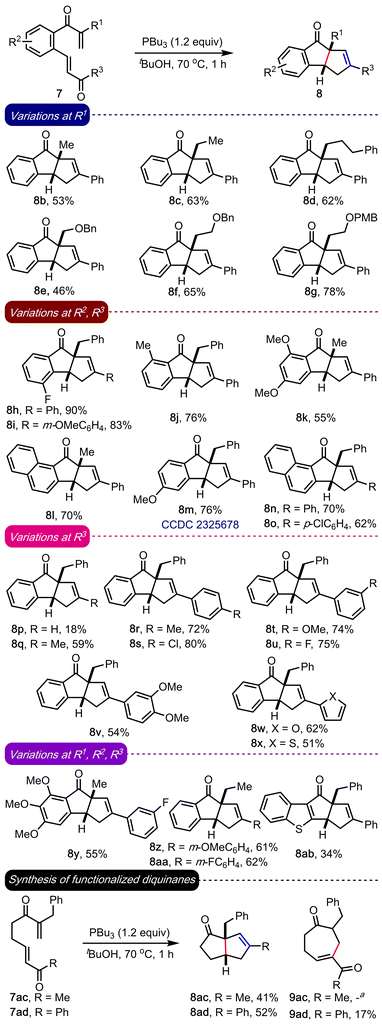 |
| Scheme 2 Substrate scope. See the ESI† for a detailed procedure. The reactions were performed on 0.1–0.2 mmol scale and the yields reported are after isolation by column chromatography aNot detected. | |
It is worth highlighting that the method can also be extended to synthesizing functionalized diquinanes such as 8ac and 8ad in moderate yields, Scheme 2. The intramolecular RC adduct 9ad was isolated, but 9ac was not observed. Accessing a fully alkyl-substituted diquinane 8ac significantly enhances the scope of the work. Further, these structures are amenable to further synthetic manipulations and the prevalence of numerous di- and triquinane-based bioactive natural products make it an attractive strategy.7
This method was also extended to synthesizing cyclopenta[a]indenes with two contiguous quaternary carbons, Scheme 3. The substituents at R1 and R2 in 10 would eventually translate to the quaternary carbons in 11. Accordingly, the required bis-enones 10 were prepared. By subjecting 10a–10d to the optimized conditions, we accessed 11a–11d in good to excellent yields. All the examples accommodated alkyl groups at R1 and R2, and aryl (11a and 11b), alkyl (11c) and heteroaryl (11d) groups at R3. Notably, a cyclopenta[a]indene derivative with all-alkyl substituents (11c) at R1, R2 and R3 can also be synthesized.
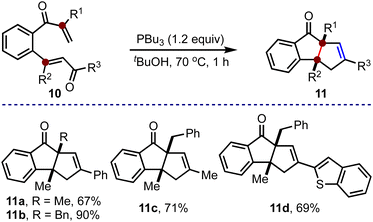 |
| Scheme 3 Synthesis of cyclopenta[a]indenes with two contiguous quaternary carbons. | |
Next, we focused on establishing the synthetic utility of the benzannulated diquinanes accessed herein. We transformed 8g to 12gvia PMB deprotection followed by dehydration of the primary alcohol, Scheme 4. To undertake an oxy-Cope rearrangement, we converted 12g to the allyl vinyl carbinol 13g by the addition of the vinyl Grignard reagent, which, upon exposure to potassium hydride, prompted a cascade oxy-Cope rearrangement and intramolecular ene-type cyclization8 to generate an unexpected octahydrobenzo[e]-as-indacene 14g in 54% yield. Further, 14g was converted to the tetrahydrobenzo[e]-as-indacene 15g under acidic conditions. Indacenes such as 15 can exhibit interesting medicinal and photophysical properties.9
 |
| Scheme 4 Elaboration of 8g to 15gvia oxy-Cope and an ene-type cyclization sequence. Reagents and conditions: (a) (i) DDQ (1.5 equiv.), DCM : water (9 : 1), RT, 2 h. (ii) 2-Nitrophenyl selenocyanate (3 equiv.), PBu3 (3 equiv.), anh. THF, RT, 4 h, then H2O2, 2 h, 65% (over two steps). (b) CH2 CHMgBr (3 equiv.), anh. THF, 0 °C, 2 h, 95%. (c) KH (1.2 equiv.), 18-C-6 (1.1 equiv.), anh. THF, 0 °C-RT, 30 min, 54%. (d) p-TSA (0.1 equiv.), toluene, RT, 5 h, 72%. | |
The cyclopenta[a]indenes were also extended to synthesizing natural product-like polycyclic cyclopentanoids. For example, the benzo-fused diquinane 8a was converted to the diol 16a under OsO4-catalyzed dihydroxylation conditions, Scheme 5. Transforming the diol 16a to the cyclic carbonate 17a represents the construction of the tetracyclic framework of clavulyne1c and jejucarboside family of natural products.10
 |
| Scheme 5 Elaboration of 8a to the tetracyclic framework of clavulyne and jejucarboside family of natural products. Reagents and conditions: (a) OsO4 (10 mol%), NMO (2.5 equiv.), THF : water (5 : 1), RT, 24 h, 90%. (b) CDI (2 equiv.), Et3N (2 equiv.), dry DCM, RT, 6 h, 86%. | |
We also planned to extend cyclopenta[a]indenes 8 to other polycyclic structures, Scheme 6. Accordingly, we converted 8a to 19gvia PMB deprotection, IBX oxidation of the primary alcohol, and a methylene Wittig reaction of the resulting aldehyde. Next, 19g was treated with the vinyl Grignard reagent to achieve the respective allyl vinyl carbinol, which was subjected to ring-closing metathesis reaction using the Grubbs’ 2nd generation catalyst and isolated the dicyclopenta[a,b]indene 20g. Similarly, we accessed cyclopenta[k]fluorene 21g and benzo[a]cyclopenta[c]azulene 22g, which are part structures of several pharmaceutically relevant molecules and materials.1d,11
 |
| Scheme 6 Elaboration of 8g to various tetracyclic structures. Reagents and conditions: (a) (i) DDQ (1.5 equiv.), DCM : water (9 : 1), RT, 2 h, 79%. (ii) IBX (1.2 equiv.), DMSO, RT, 1 h, 81%. (iii) CH2 PPh3 (1.5 equiv.), anh. THF, 0 °C, 2 h, 89%. (b) (i) CH2 CHMgBr (3 equiv.), anh. THF, 0 °C, 2 h. (ii) Grubbs’ 2nd generation catalyst (G-II, 5 mol%), dry DCM, reflux, 1 h, 88% (over two steps). (c) (i) CH2 CHCH2MgCl (3 equiv.), anh. THF, 0 °C, 2 h, dr = 5 : 1. (ii) G-II (5 mol%), dry DCM, reflux, 1 h, 85% (over two steps), dr = 5 : 1. (d) (i) CH2 CHCH2CH2MgBr (3 equiv.), anh. THF, 0 °C, 2 h. (ii) G-II (5 mol%), dry DCM, reflux, 1 h, 82% (over two steps). | |
Sativamide A 4 possesses a unique 6-5-5-5 tetracyclic rearranged nor-lignan carbon skeleton and exhibits potential neuroprotective activity on several cell models, Scheme 7.1d To assemble the core structure of 4, we prepared the bis-enone 7ae, subjected it to the optimized conditions described in Scheme 3, and obtained the respective cyclopenta[a]indene 8ae in 46% yield. Then, we transformed 8ae to 20ae in five steps following the synthetic protocol described for 20g. With the presence of 6-5-5-5 core and the required functionalities in place, 20ae represents an advanced precursor for synthesizing sativamides.
 |
| Scheme 7 Synthesis of an advanced intermediate 20ae to synthesize sativamide A. Reagents and conditions: (a) PBu3 (1.2 equiv.), tBuOH, 70 °C, 1 h, 46%. (b) (i) DDQ (1.5 equiv.), DCM : water (9 : 1), RT, 2 h. (ii) IBX (1.2 equiv.), DMSO, RT, 1 h. (iii) CH2 PPh3 (1.5 equiv.), anh. THF, 0 °C, 2 h, 55% over three steps. (c) (i) CH2 CHMgBr (3 equiv.), anh. THF, 0 °C, 2 h. (ii) Grubbs’ 2nd generation catalyst (G-II, 5 mol%), dry DCM, reflux, 1 h, 81% over two steps. | |
In summary, we developed a phosphine-promoted Rauhut–Currier/Wittig reaction cascade12 to assemble diquinanes and a wide range of arene- and heteroarene-fused diquinanes. The scope and generality of the method were quite broad. The utility of the concept was thoroughly exemplified by synthesizing several complex cyclopentanoids and various advanced intermediates for natural product synthesis and materials chemistry. A few other merits of this strategy are (i) neutral and metal-free conditions, (ii) easily accessible starting compounds, and (iii) the occurrence of numerous bioactive molecules with the kind of molecular architectures accessed herein. We are applying this method to synthesize complex bioactive natural products, and the results will be communicated in due course.
Conflicts of interest
The authors declare no competing financial interest.
Acknowledgements
The authors thank IISER Mohali for the central and departmental NMR and X-ray facilities, and the mass spectrometry facility. S. S. V. R. thanks SERB for the Swarnajayanti fellowship (DST/SJF/CSA-01/2017-18), Elsevier's Reaxys grant and IISER Mohali for funding. J. P. M. thanks IISER Mohali and S. S. S. thanks CSIR for research fellowships.
References
- For kadsuphilins, see:
(a) Y.-C. Shen, Y.-C. Lin, Y.-B. Cheng, C.-J. Chang, T.-W. Lan, S.-S. Liou, C.-T. Chien, C.-C. Liaw and A. T. Khalil, New Oxygenated Lignans from Kadsura Philippinensis, Helv. Chim. Acta, 2008, 91, 483–494 CrossRef CAS;
(b) For cyanosporasides, see: A. L. Lane, S.-J. Nam, T. Fukuda, K. Yamanaka, C. A. Kauffman, P. R. Jensen, W. Fenical and B. S. Moore, Structures and Comparative Characterization of Biosynthetic Gene Clusters for Cyanosporasides, Enediyne-Derived Natural Products from Marine Actinomycetes, J. Am. Chem. Soc., 2013, 135, 4171–4174 CrossRef CAS PubMed;
(c) For clavulynes, see: E. J. Han, S. R. Lee, C. A. Townsend and M. R. Seyedsayamdost, Targeted Discovery of Cryptic Enediyne Natural Products via FRET-Coupled High-Throughput Elicitor Screening, ACS Chem. Biol., 2023, 18, 1854–1862 CrossRef CAS PubMed;
(d) For sativamides: G.-Y. Zhu, J. Yang, X.-J. Yao, X. Yang, J. Fu, X. Liu, L.-P. Bai, L. Liu and Z.-H. Jiang, (±)-Sativamides A and B, Two Pairs of Racemic Nor-Lignanamide Enantiomers from the Fruits of Cannabis Sativa, J. Org. Chem., 2018, 83, 2376–2381 CrossRef CAS PubMed;
(e) For an anti-Alzheimer agent, see: A. A. Titov, M. S. Kobzev, M. Catto, M. de Candia, N. Gambacorta, N. Denora, L. Pisani, O. Nicolotti, T. N. Borisova, A. V. Varlamov, L. G. Voskressensky and C. D. Altomare, Away from Flatness: Unprecedented Nitrogen-Bridged Cyclopenta[a]Indene Derivatives as Novel Anti-Alzheimer Multitarget Agents, ACS Chem. Neurosci., 2021, 12, 340–353 CrossRef CAS PubMed;
(f) For theacitrins, see: Y. Matsuo, K. Okuda, H. Morikawa, R. Oowatashi, Y. Saito and T. Tanaka, Stereochemistry of the Black Tea Pigments Theacitrins A and C, J. Nat. Prod., 2016, 79, 189–195 CrossRef CAS PubMed.
- A few representative metal-promoted strategies to cyclopenta[a]indenes:
(a) A. C. F. Cruz, N. D. Miller and M. C. Willis, Intramolecular Palladium-Catalyzed Direct Arylation of Alkenyl Triflates, Org. Lett., 2007, 9, 4391–4393 CrossRef CAS PubMed;
(b) D. Aburano, F. Inagaki, S. Tomonaga and C. Mukai, Synthesis of a Core Carbon Framework of Cyanosporasides A and B, J. Org. Chem., 2009, 74, 5590–5594 CrossRef CAS PubMed;
(c) B. Yao, Y. Li, Z. Liang and Y. Zhang, Ni-Catalyzed Intramolecular Cycloaddition of Methylenecyclopropanes to Alkynes, Org. Lett., 2011, 13, 640–643 CrossRef CAS PubMed;
(d) A. Schweinitz, A. Chtchemelinine and A. Orellana, Synthesis of Benzodiquinanes via Tandem Palladium-Catalyzed Semipinacol Rearrangement and Direct Arylation, Org. Lett., 2011, 13, 232–235 CrossRef CAS PubMed;
(e) H. Xu, J.-P. Qu, S. Liao, H. Xiong and Y. Tang, Highly Enantioselective [3 + 2] Annulation of Cyclic Enol Silyl Ethers with Donor–Acceptor Cyclopropanes: Accessing 3a-Hydroxy[n.,3.0]Carbobicycles, Angew. Chem., Int. Ed., 2013, 52, 4004–4007 CrossRef CAS PubMed;
(f) C. R. Reddy, K. Warudikar and B. Sridhar, Synthetic Access to Cyclopenta[a]Inden-2(1H)-Ones from Morita–Baylis–Hillman Products of 2-Alkynyl Benzaldehydes, ACS Omega, 2018, 3, 15734–15742 CrossRef PubMed;
(g) L. Zhou, X. Liu, H. Lu, G. Deng, Y. Liang, Y. Yang and J.-H. Li, Copper-Catalyzed [3 + 2]/[3 + 2] Carboannulation of dienynes and arylsulfonyl chlorides enabled by smiles rearrangement: access to cyclopenta[a]indene-fused quinolinones, Org. Chem. Front., 2021, 8, 5092–5097 RSC.
- A. Midya, L. D. Khalse and P. Ghorai, Chiral Amine Catalyzed Reductive Aldol/Reductive Michael Addition Cascade Towards Enantioselective Synthesis of Benzannulated Diquinanes, Eur. J. Org. Chem., 2023, e202201409 CrossRef CAS.
- Selected reviews:
(a) C. E. Aroyan, A. Dermenci and S. J. Miller, The Rauhut–Currier reaction: a history and its synthetic application, Tetrahedron, 2009, 65, 4069–4084 CrossRef CAS;
(b) K. Kaur and I. N. N. Namboothiri, Morita-Baylis-Hillman and Rauhut-Currier Reactions of Conjugated Nitroalkenes, Chimia, 2012, 66, 913–920 CrossRef CAS PubMed;
(c) K. C. Bharadwaj, Intramolecular Morita–Baylis–Hillman and Rauhut–Currier reactions. A catalytic and atom economic route for carbocycles and heterocycles, RSC Adv., 2015, 5, 75923–75946 RSC;
(d) Y.-N. Gao and M. Shi, Phosphine-mediated enantioselective synthesis of carbocycles and heterocycles, Chin. Chem. Lett., 2017, 28, 493–502 CrossRef CAS;
(e) H. Ni, W.-L. Chan and Y. Lu, Phosphine-Catalyzed Asymmetric Organic Reactions, Chem. Rev., 2018, 118, 9344–9411 CrossRef CAS PubMed;
(f) S. Biswas, N. Bania and S. C. Pan, Recent Developments in Intermolecular Cross-Rauhut-Currier Reactions, Chem. Rec., 2023, 23, e202200257 CrossRef CAS PubMed.
- Selected papers from among several prominent works:
(a) L.-C. Wang, A. L. Luis, K. Agapiou, H.-Y. Jang and M. J. Krische, Organocatalytic Michael Cycloisomerization of Bis(enones): The Intramolecular Rauhut-Currier Reaction, J. Am. Chem. Soc., 2002, 124, 2402–2403 CrossRef CAS PubMed;
(b) C. E. Aroyan and S. J. Miller, Enantioselective Rauhut-Currier reactions promoted by protected cysteine, J. Am. Chem. Soc., 2007, 129, 256–257 CrossRef CAS PubMed;
(c) E. Marqués-López, R. P. Herrera, T. Marks, W. C. Jacobs, D. Könning, R. M. de Figueiredo and M. Christmann, Crossed Intramolecular Rauhut-Currier-Type Reactions via Dienamine Activation, Org. Lett., 2009, 11, 4116–4119 CrossRef PubMed;
(d) X.-F. Wang, L. Peng, J. An, C. Li, Q.-Q. Yang, L.-Q. Lu, F.-L. Gu and W.-J. Xiao, Enantioselective Intramolecular Crossed Rauhut–Currier Reactions through Cooperative Nucleophilic Activation and Hydrogen-Bonding Catalysis: Scope and Mechanistic Insight, Chem. – Eur. J., 2011, 17, 6484–6491 CrossRef CAS PubMed;
(e) J.-J. Gong, T.-Z. Li, K. Pan and X.-Y. Wu, Enantioselective intramolecular Rauhut–Currier reaction catalyzed by chiral phosphinothiourea, Chem. Commun., 2011, 47, 1491–1493 RSC;
(f) S. Takizawa, T. M.-N. Nguyen, A. Grossmann, D. Enders and H. Sasai, Enantioselective Synthesis of α-Alkylidene-γ-Butyrolactones: Intramolecular Rauhut-Currier Reaction Promoted by Acid/Base Organocatalysts, Angew. Chem., Int. Ed., 2012, 51, 5423–5426 CrossRef CAS PubMed;
(g) Z. Shi, P. Yu, T.-P. Loh and G. Zhong, Catalytic Asymmetric [4 + 2] Annulation Initiated by an Aza-Rauhut-Currier Reaction: Facile Entry to Highly Functionalized Tetrahydropyridines, Angew. Chem., Int. Ed., 2012, 51, 7825–7829 CrossRef CAS PubMed;
(h) R. Kumar, T. Kumar, S. M. Mobin and I. N. N. Nambothiri, Rauhut-Currier Reaction of Nitroalkenes with Vinyl Sulfones, J. Org. Chem., 2013, 78, 5073–5077 CrossRef CAS PubMed;
(i) R. J. H. Scanes, O. Grossmann, A. Grossmann and D. R. Spring, Enantioselective Synthesis of Chromanones via a Peptidic Phosphane Catalyzed Rauhut-Currier Reaction, Org. Lett., 2015, 17, 2462–2465 CrossRef CAS PubMed;
(j) X. Su, W. Zhou, Y. Li and J. Zhang, Design, Synthesis, and Application of a Chiral Sulfinamide Phosphine Catalyst for the Enantioselective Intramolecular Rauhut-Currier Reaction, Angew. Chem., Int. Ed., 2015, 54, 6874–6877 CrossRef CAS PubMed;
(k) X. Wu, L. Zhou, R. Maiti, C. Mou, L. Pan and Y. R. Chi, Sulfinate and Carbene Co-catalyzed Rauhut-Currier Reaction for Enantioselective Access to Azepino[1,2-a]indoles, Angew. Chem., Int. Ed., 2019, 58, 477–481 CrossRef CAS PubMed;
(l) S. Bae, C. Zhang, R. M. Gillard and D. W. Lupton, Enantioselective N-Heterocyclic Carbene Catalyzed Bis(enoate) Rauhut-Currier Reaction, Angew. Chem., Int. Ed., 2019, 58, 13370–13374 CrossRef CAS PubMed;
(m) S. B. Thopate, L. R. Magham, S. Dinda and R. Chegondi, Solvent-mediated Enantioselective Rauhut-Currier Cyclization via Iminium and Enamine Activation, Org. Lett., 2023, 25, 1072–1077 CrossRef CAS PubMed.
- Selected papers from among several prominent works:
(a) W. Yao, Y. Wu, G. Wang, Y. Zhang and C. Ma, Tertiary Amine Mediated Tandem Cross-Rauhut-Currier/Acetalization Reactions: Access to Functionalized Spiro-3,4-Dihydropyrans, Angew. Chem., Int. Ed., 2009, 48, 9713–9716 CrossRef CAS PubMed;
(b) P. Xie, Y. Huang, W. Lai, X. Meng and R. Chen, Bifunctional phosphine-catalyzed cross-Rauhut-Currier/Michael/aldol condensation triple domino reaction: synthesis of functionalized cyclohexenes, Org. Biomol. Chem., 2011, 9, 6707–6714 RSC;
(c) W. Liu, J. Zhou, C. Zheng, X. Chen, H. Xiao, Y. Yang, Y. Guo and G. Zhao, Tandem cross-Rauhut-Currier/cyclization reactions of activated alkenes to give densely functionalized 3,4-dihydropyrans, Tetrahedron, 2011, 67, 1768–1773 CrossRef CAS;
(d) P. Xie and Y. Huang, Domino cyclization initiated by cross-Rauhut-Currier reactions, Eur. J. Org. Chem., 2013, 6213–6226 CrossRef CAS;
(e) Y.-Y. Zhang, R. Gurubrahamam and K. Chen, Rauhut-Currier-Initiated Organocascade Reaction: Synthesis of Substituted Dispirocyclohexanes through a [2 + 2 + 2] Strategy Between 2-Arylideneindan-1,3-diones and Activated Alkenes, Adv. Synth. Catal., 2015, 357, 2457–2463 CrossRef CAS;
(f) Z. Zhou, Q. He, Y. Jiang, Q. Ouyang, W. Du and Y.-C. Chen, Double Thiol-Chiral Bronsted Base Catalysis: Asymmetric Cross Rauhut-Currier Reaction and Sequential [4 + 2] Annulation for Assembly of Different Activated Olefins, Org. Lett., 2019, 21, 7184–7188 CrossRef CAS PubMed;
(g) N. Gigant, E. Drège and D. Joseph, Carbon Nucleophile-Initiated Rauhut-Currier Reaction: An Atom-Economical Synthesis of Highly Functionalized Carbocycles, J. Org. Chem., 2023, 88, 12069–12073 CrossRef CAS PubMed.
- Selected reviews:
(a) G. Mehta and A. Srikrishna, Synthesis of Polyquinane Natural Products: An Update, Chem. Rev., 1997, 97, 671–720 CrossRef CAS PubMed;
(b) A. J. Ferreira and C. M. Beaudry, Synthesis of natural products containing fully functionalized cyclopentanes, Tetrahedron, 2017, 73, 965–1084 CrossRef CAS;
(c) H. Li, J. Zhang and X. She, The Total Synthesis of Diquinane-Containing Natural Products, Chem. – Eur. J., 2021, 27, 4839–4858 CrossRef CAS PubMed;
(d) T. Saito, J. M. Awad and W. Zhang, Synthetic Studies on Tetracyclic Diquinane Lycopodium Alkaloids Magellanine, Magellaninone and Paniculatine, Molecules, 2023, 28, 1501 CrossRef CAS PubMed.
- For tandem oxy-Cope/ene reactions, see:
(a) J. M. Warrington, G. P. A. Yap and L. Barriault, Tandem Oxy-Cope/Transannular Ene Reaction of 1,2-Divinylcyclohexanols, Org. Lett., 2000, 2, 663–665 CrossRef CAS PubMed;
(b) M. Kourgiantaki, V. P. Demertzidou and A. L. Zografos, Short Scalable Route to Apiaceae Sesquiterpene Scaffolds: Total Synthesis of 4-epi-Epiguaidiol A, Org. Lett., 2022, 24, 8476–8480 CrossRef CAS PubMed.
-
(a) B. S. Young, D. T. Chase, J. L. Marshall, C. L. Vonnegut, L. N. Zakharov and M. M. Haley, Synthesis and properties of fully-conjugated indacenedithiophenes, Chem. Sci., 2014, 5, 1008–1014 RSC;
(b) Y. Ie, C. Sato, K. Yamamoto, M. Nitani and Y. Aso, A Thiazole-fused antiaromatic compound containing an s-Indacene chromophore with a high electron affinity, Chem. Lett., 2018, 47, 1534–1537 CrossRef CAS;
(c) P. J. Mayer and G. London, Stable Monoareno-pentalenes with Two Olefinic Protons, Org. Lett., 2023, 25, 42–46 CrossRef CAS PubMed.
- J. H. Im, D. Shin, Y. H. Ban, W. S. Byun, E. S. Bae, D. Lee, Y. E. Du, J. Cui, Y. Kwon, S.-J. Nam, S. Cha, S. K. Lee, Y. J. Yoon and D.-C. Oh, Targeted Discovery of an Enediyne-Derived Cycloaromatized Compound, Jejucarboside
A, from a Marine Actinomycete, Org. Lett., 2022, 24, 7188–7193 CrossRef CAS PubMed.
-
(a) M. Seifert and D. Kuck, Naphtho-anellated [5.6.5]- and [6.5.5.5]Fenestranes, Tetrahedron, 1996, 52, 13167–13180 CrossRef CAS;
(b) W. Thaharn, D. Soorukram, C. Kuhakarn, V. Reutrakul and M. Pohmakotr, Synthesis of C2–Symmetric gem-Difluoromethylenated Angular Triquinanes, J. Org. Chem., 2018, 83, 388–402 CrossRef CAS PubMed.
- For Rauhut–Currier/Wittig cascades to access cyclopentanoids, see:
(a) R. Zhou, J. Wang, J. Yu and Z. He, Highly Chemoselective Rauhut-Currier Reaction between Maleimides and Enones and Dual Phosphine-Mediated One-Pot Synthesis of Bicyclic and Polycyclic Skeletons, J. Org. Chem., 2013, 78, 10596–10604 CrossRef CAS PubMed;
(b) S. S. Vagh, B.-J. Hou, A. Edukondalu, P.-C. Wang, Y.-R. Chen and W. Lin, Phosphine-Mediated Rauhut-Currier-Type/Acyl Transfer/Wittig Strategy for Synthesis of Spirocyclopenta[c]chromene-Indolinones, Adv. Synth. Catal., 2021, 363, 5429–5435 CrossRef;
(c) Y.-Q. Li, G.-D. Xu and Z.-Z. Huang, An organophosphorus-mediated cross-Rauhut-Currier/Wittig domino reaction for the efficient synthesis of trisubstituted cyclopentenes, Org. Biomol. Chem., 2021, 19, 2487–2491 RSC.
Footnote |
† Electronic supplementary information (ESI) available: Experimental details and characterisation data for new compounds. CCDC 2325678 [for 8m] and 2327817 [for 17a]. For ESI and crystallographic data in CIF or other electronic format see DOI: https://doi.org/10.1039/d4ob00984c |
|
This journal is © The Royal Society of Chemistry 2024 |