A pyridine-N-oxide catenane for cation recognition†
Received
2nd February 2024
, Accepted 17th March 2024
First published on 22nd March 2024
Abstract
The rapid preparation of a pyridine-N-oxide containing [2]catenane is described. The [2]catenane was characterized by NMR spectroscopy, mass spectrometry and X-ray single crystal structure determination. 1H NMR titration experiments reveal the [2]catenane may be reversibly protonated, as well as an ability to bind lithium cations more strongly than sodium cations.
Introduction
Hydrogen bonding – often augmented with other non-covalent interactions – has been widely used in the templated synthesis of mechanically interlocked molecules (MIMs)1 such as catenanes2 and rotaxanes.3 Examples of MIMs prepared by hydrogen bonding templation strategies,4,5 have been explored as nanotechnological components such as molecular switches6 and rotors,7 as well as catalysts8 and receptors for anionic guests.9
Pyridine-N-oxides (PNOs) are hydrogen bond acceptors and as such have been used in the construction of several hydrogen bond templated MIMs,10 as well as MIMs prepared using other non-covalent interactions.11 When included in MIMs, the PNO motif may offer the potential of protonation12 and/or metal ion coordination.13
The development of molecular receptors capable of the selective binding and/or sensing of lithium cations14 is of relevance to society, due to the increased extraction of lithium to support lithium ion-battery technology – activity which risks mobilizing toxic levels of lithium salts into the environment.15 Only a handful of MIMs have been prepared by lithium cation templation16,17 or shown to bind lithium cations.18
Here we report on the rapid synthesis of a rare example of a PNO containing [2]catenane13c capable of binding of cationic guests. The catenane was characterized by NMR spectroscopy, mass spectrometry and X-ray crystallography. 1H NMR titrations were then undertaken to assess the solution phase cationic guest recognition ability of the [2]catenane. These titrations revealed both reversible protonation and the binding of alkali metal cations – notably lithium more strongly than sodium.
Results and discussion
PNO [2]catenane: design, synthesis and characterization
To prepare a PNO [2]catenane, we proposed to use our previously reported hydrogen bond templation synthetic methodology,5n but substituting the central CH2OCH2 unit of the glycol chain for a 2,6-pyridyl unit. Oxidation of pyridyl group(s) of the pyridyl [2]catenane would then furnish a PNO [2]catenane.
The pyridyl [2]catenane 2 (Scheme 1) was thus prepared. Simultaneous addition of previously reported dimethanamine 1 (preparable in two steps)5j and commercially available isophthaloyl chloride into a solution of triethylamine in dichloromethane under pseudo-high dilution conditions led to the formation of the desired pyridyl [2]catenane 2 and non-interlocked macrocycle 3. Upon aqueous workup and purification by column chromatography, [2]catenane 2 was isolated in a 15% yield (a yield broadly in line with our previously reported [2]catenane5n). The novel catenane was characterized by 1H and 13C NMR spectroscopy and mass spectrometry (see ESI, pp. S2 and S3†).
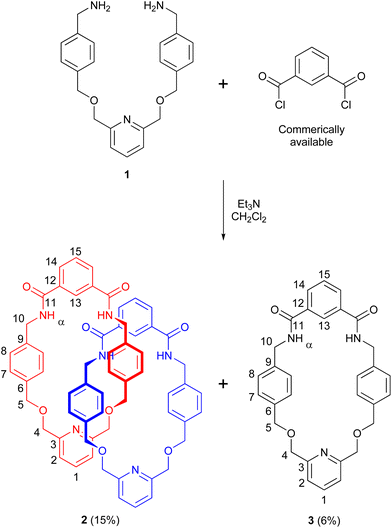 |
| Scheme 1 Synthesis of [2]catenane 2. | |
The PNO [2]catenane 4 was then synthesized by reacting [2]catenane 2 with an excess of m-chloroperoxybenzoic acid (mCPBA) to achieve oxidation of the two pyridine units within the catenane (Scheme 2).19 After aqueous workup and column chromatography, PNO [2]catenane 4 was afforded in 82% yield, and characterized by 1H and 13C NMR spectroscopy and mass spectrometry (see Fig. 1 and ESI, pp. S6 and S7†).
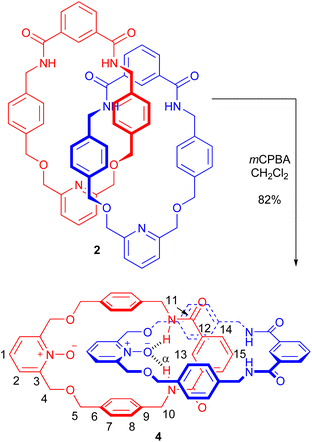 |
| Scheme 2 Synthesis of target PNO [2]catenane 4. | |
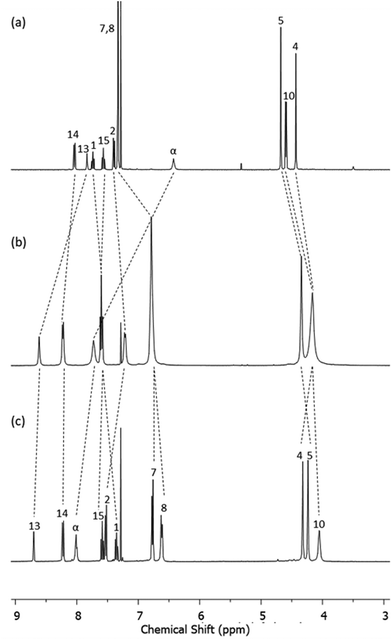 |
| Fig. 1
1H NMR spectra of (a) macrocycle 3, (b) [2]catenane 2 and (c) PNO [2]catenane 4 (CDCl3, 400 MHz, 298 K). For atom labels, see Schemes 1 and 2. | |
Evidence for the interlocked structures of [2]catenanes 2 and 4 may be found by comparison of their 1H NMR spectra with macrocycle 3 (Fig. 1). Large downfield shifts in amide protons α and internal isophthalamide proton 13 for [2]catenane 2 compared to macrocycle 3 is indicative of inter-ring hydrogen bonding in the catenane. The upfield shift of protons 7 and 8 are consistent with the threading of one macrocyclic ring through the other. Upon oxidation to PNO [2]catenane 4, there is a further downfield shift in the amide protons α, attributed to hydrogen bonding now being possible to the negatively charged oxygen atom of the PNO.
Unequivocal evidence for the interlocked structures of both pyridyl [2]catenane 2 and PNO [2]catenane 4 were provided by X-ray structure determination using single crystals grown by slow evaporation of separate chloroform solutions. As depicted in Fig. 2a, the amide N–Hs of the left-hand macrocycle of [2]catenane 2 are participating in hydrogen bonds with the carbonyl oxygen of the right-hand macrocycle (N–H⋯O
C distances: 2.15(2) Å and 2.29(2) Å). When oxidized to PNO [2]catenane 4 (Fig. 2b), these hydrogen bonding events are retained (N–H⋯O
C distances: 2.14(2) Å and 2.36(4) Å), and in addition the pyridine-N-oxide oxygen of the macrocycle on the left-hand ring participates in intramolecular hydrogen bonding events with the isophthalamide N–Hs of the right-hand macrocycle (N–H⋯−O–N+ distances: 2.06(0) Å and 2.25(3) Å).
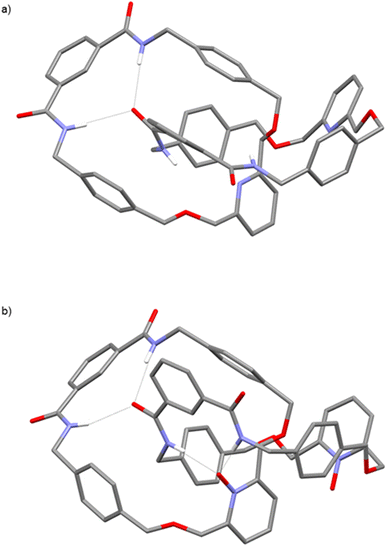 |
| Fig. 2 X-ray crystal structures of (a) pyridyl [2]catenane 2 and (b) PNO [2]catenane 4. Hydrogen atoms (except N–Hs) are omitted for clarity. | |
PNO [2]catenane: 1H NMR titration binding studies
With PNO [2]catenane 4 in hand, attention turned to investigating whether it could act as a host for positively charged guest species. It was hypothesized that a single atom cation may bind within the central cavity of the catenane, coordinated by both pyridine-N-oxide units, achieved by pirouetting of the interlocked rings (Scheme 3).201H NMR titration studies were undertaken in conjunction with BindFit21 to determine association constants.
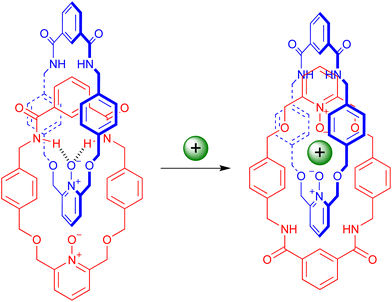 |
| Scheme 3 Potential binding motif of [2]catenane 4 with a cationic guest. | |
An initial titration study was undertaken with aliquots of trifluoroacetic acid (TFA) being added to [2]catenane 4 in CDCl3 (Fig. 3 & ESI, pp. S9–S11†).22–24 Several perturbations in the aromatic protons are observed, indicating the coordination of the proton by [2]catenane 4. For example, proton 13 experiences an upfield shift, consistent with reduction in hydrogen bonding arising from protonation inducing pirouetting of the catenane rings.25 Furthermore, the protonation event could be reversed upon addition of base (d5-pyridine) resulting in a restoration of the initial proton signal shifts in the 1H NMR spectrum.
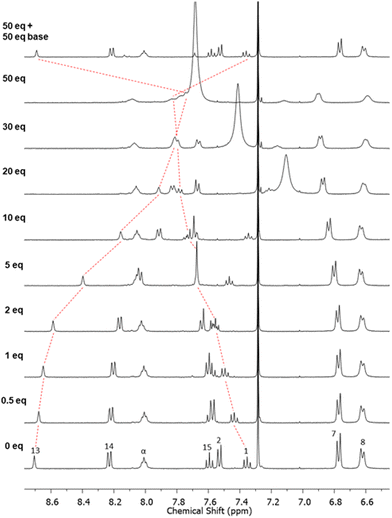 |
| Fig. 3 Partial 1H NMR spectra and highlighted chemical shifts of diagnostic proton resonances of PNO [2]catenane 4 with set equivalent additions of TFA followed by reversal with the addition of d5-pyridine (CDCl3, 400 MHz, 298 K). NB: The large peak that appears in spectra for 20, 30 and 50 eq. of TFA is protic O–H. | |
We then proceeded to study if [2]catenane 4 could act as a host for alkali metal cations.26,27 Due to the poor solubility of alkali metal salts in neat CDCl3, a solvent system of 4
:
1 CDCl3/CD3CN was used in the titration studies. Upon addition of LiClO4 to [2]catenane 4, shifts in several resonances are observed (Fig. 4). The most obvious is the upfield shift for isophthalamide proton 13, which is consistent with coordination of the Li+ cation to the PNO oxygen atom once again disrupting inter-ring hydrogen bonding. Beyond 1 equivalent, there is a reverse in shift direction – implying a second binding mode occurring. BindFit analysis of non-overlapping resonances of catenane protons 2, 4, 7, 13 and 14, determined 1
:
2 stoichiometric host–guest associations constants of K1:1 = 980 M−1 and K1:2 = 20 M−1 respectively.28
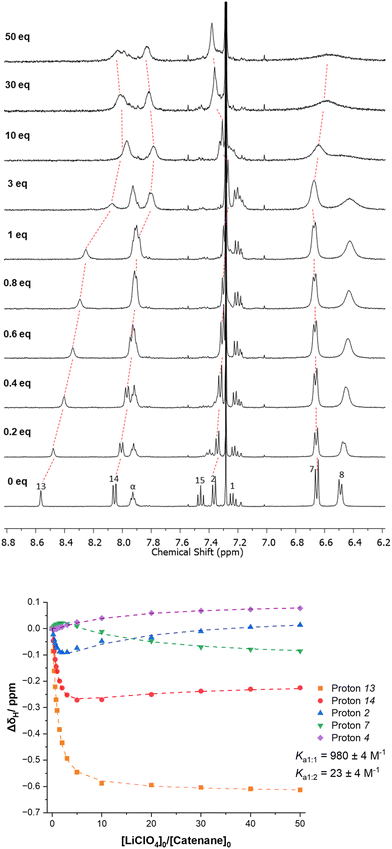 |
| Fig. 4 Partial 1H NMR spectra and highlighted chemical shifts of diagnostic proton resonances of PNO [2]catenane 4 with set equivalent additions of LiClO4 (4 : 1 CDCl3/CD3CN, 400 MHz, 298 K) and globally fitted data (by BindFit) for a 1 : 2 binding isotherm using change in peak positions against a ratio of initial cation and catenane concentration. | |
The addition of NaBArF to a fresh sample of [2]catenane 4 showed smaller perturbations of the diagnostic protons (Fig. 5). Furthermore, there is no evidence of a second binding event upon addition of excess NaBArF. BindFit analysis affords a 1
:
1 stoichiometric association constant for Na+ of K = 80 M−1.29 The observed differences in binding of Li+ and Na+ may be attributed to the higher charge density of the Li+ cation compared to that of the Na+ cation and better size complementarity with the small cavity available within [2]catenane 4.
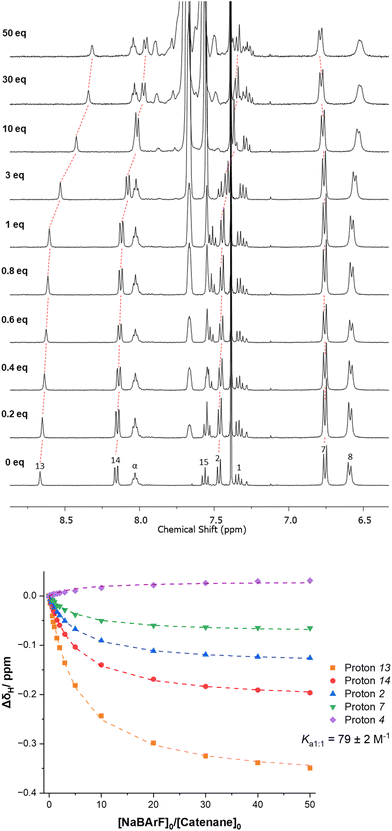 |
| Fig. 5 Partial 1H NMR spectra and highlighted chemical shifts of diagnostic proton resonances of PNO [2]catenane 4 with set equivalent additions of NaBArF (4 : 1 CDCl3/CD3CN, 400 MHz, 298 K) and globally fitted data (by BindFit) for a 1 : 1 binding isotherm using change in peak positions against a ratio of initial cation and catenane concentration. | |
Conclusions
The rapid synthesis and characterization of a pyridine-N-oxide [2]catenane was undertaken. A series of 1H NMR titrations were undertaken with cationic guests. Addition of acid leads to protonation and pirouetting of the interlocked rings, which could be reversed with the addition of base. The PNO [2]catenane also showed a strong binding affinity for the Li+ cation (compared to Na+). To the best of our knowledge this paper represents the first reported quantitative study of alkali metal cation binding by a PNO containing interlocked molecule.30 Future work will be focused on enhancing the selectivity of such catenanes for Li+ and other alkali metal cation guests. In general, the use of the pyridine-N-oxide motif in mechanical bond formation and investigations into MIMs capable of ion binding are continuing in our laboratory.
Experimental section
General information
All reagents and solvents were used as obtained from commercial suppliers, unless otherwise stated. Dry solvents and Et3N were purchased dry and stored under an inert atmosphere. Deionised water was used in all cases. All aqueous solutions are saturated unless otherwise stated.
Silica gel with a 60 Å particle size was used as the stationary phase for column chromatography. Analytical TLC was used to monitor the progress of column chromatography, with analytical TLC plates examined under short wavelength (254 nm) UV light or staining with potassium permanganate and phosphomolybdic acid solutions as appropriate.
IR spectra were recorded on an Agilent Technologies Cary 630 FTIR spectrometer. NMR spectra were recorded on a Bruker AVANCE III 400 at 298 K (unless otherwise stated). Mass spectra were recorded on Shimadzu LCMS-8040, Shimadzu LCMS IT ToF and Bruker Compact ToF coupled to an Agilent 1260 Infinity LC. Melting points were recorded on a Gallenkamp capillary melting point apparatus and are uncorrected.
Bis-amine 1 was synthesized by adaptation of a previously reported procedure.5j
Experimental procedures
Pyridyl [2]catenane 2.
To a three-neck flask containing Et3N (0.83 mL, 6.00 mmol) in dry CH2Cl2 (100 mL) under Ar (g) was added a solution of bis-amine 1 (907 mg, 2.40 mmol) in dry CH2Cl2 (50 mL) and a solution of isophthaloyl chloride (487 mg, 2.40 mmol) in dry CH2Cl2 (50 mL) in simultaneous dropwise addition. After the addition was complete, the reaction mixture was stirred for a further 20 h, then washed with 1 M HCl (aq) (2 × 50 mL) and brine (1 × 50 mL). The organic layer was then dried (MgSO4) and concentrated in vacuo. The crude material was purified by silica gel column chromatography (9
:
1 EtOAc/CH2Cl2) to afford the title product2 (364 mg, 15%) as a colourless solid.
R
f
: 0.31 [EtOAc/CH2Cl2 9
:
1]; mp: 111–113 °C; IR νmax/cm−1(neat): 3321 (N–H), 2857 (C–H), 2365 (C–H), 1647 (C
O), 1522 (C–O), 1079 (C–O); δH(400 MHz, CDCl3): 8.61 (2H, s, H13), 8.22 (4H, d, J = 7.5 Hz, H14), 7.72 (4H, br s, Hα), 7.60 (4H, app. t, H1 & H15), 7.21 (4H, d, J = 6.9 Hz, H2), 6.84–6.70 (16H, m, H7 & H8), 4.34 (8H, s, H5), 4.22–4.09 (16H, m, H10 & H4); δC(100 MHz, CDCl3): 166.1 (C11), 157.0 (C3), 137.5 (C15 or 1), 136.8 (C9), 135.9 (C6), 133.4 (C12), 131.7 (C14), 128.8 (C7 or 8), 128.6 (C1 or 15), 128.4 (C8 or 7), 124.9 (C13), 121.1 (C2), 72.7 (C5), 71.5 (C4), 44.3 (C10); MS (ESI +ve) m/z: 1037.4258 ([M + Na]+ C62H58N6NaO8 requires 1037.4208).
Macrocycle 3.
Macrocycle 3 was isolated from the above catenation reaction upon purification of the crude reaction mixture by silica gel column chromatography as a colourless solid (60 mg, 6%).
R
f
: 0.40 [EtOAc/CH2Cl2 4
:
1]; mp: 130–132 °C; IR νmax/cm−1(neat): 3296 (N–H), 2864 (C–H), 1643 (C
O), 1537 (C–H), 1090 (C–O); δH(400 MHz, CDCl3): 8.04 (2H, dd, J = 7.8, 1.7 Hz, H14), 7.84 (1H, s, H13), 7.74 (1H, t, J = 7.8 Hz, H1), 7.56 (1H, t, J = 7.7 Hz, H15), 7.40 (2H, d, J = 7.7 Hz, H2), 7.33 (8H, s, H7 & H8), 6.44 (2H, bs, Hα), 4.67 (4H, s, H5), 4.59 (4H, d, J = 5.6 Hz, H10), 4.43 (4H, s, H4); δC(100 MHz, CDCl3): 166.5 (C12), 157.4 (C3), 137.5 (C6 or 9), 137.1 (C1), 134.8 (C12), 130.7 (C14), 129.3 (C15), 129.0 (C7 or 8), 128.7 (C7 or 8), 124.0 (C13), 120.1 (C2), 72.2 (C5), 71.3 (C4), 44.2 (C10); MS (ESI +ve) m/z: 530.2067 ([M + Na]+ C31H29N3NaO4 requires 530.2050).
PNO [2]catenane 4.
To a solution of pyridyl [2]catenane 2 (50 mg, 0.04 mmol) in CH2Cl2 (5 mL) was added mCPBA (35 mg, 0.14 mmol, 72% mixture in water). The reaction mixture was stirred for 18 h then NaHCO3 (aq) (10 mL) was added. The aqueous layer was then extracted with CH2Cl2 (3 × 10 mL). The combined organic layers were dried (MgSO4) and concentrated in vacuo. The crude material was purified by silica gel column chromatography (98
:
2 CH2Cl2/CH3OH) to afford the title product4 (42 mg, 82%) as a colourless solid.
R
f
: 0.31 [CH2Cl2/CH3OH 98
:
2]; mp: 121–122 °C; IR νmax/cm−1(neat): 3295 (N–H), 3062 (C–H), 2860 (C–H), 1729 (C
O), 1649 (N–H), 1537 (N–O); δH(400 MHz, CDCl3): 8.70 (2H, s, H13), 8.22 (4H, dd, J = 7.9 Hz & 1.4 Hz, H14), 8.01 (4H, br s, Hα), 7.58 (2H, t, J = 7.9 Hz, H15), 7.52 (4H, d, J = 7.8 Hz, H2), 7.35 (2H, t, J = 7.8 Hz, H1), 6.77 (8H, d, J = 7.8 Hz, H7), 6.61 (8H, d, J = 7.8 Hz, H8), 4.32 (8H, s, H4), 4.23 (8H, s, H5), 4.05 (8H, br s, H10); δC(100 MHz, CDCl3): 166.1 (C11), 148.7 (C3), 137.9 (C9), 135.7 (C6), 133.8 (C12), 131.5 (C14), 129.3 (C7), 128.9 (C15), 128.3 (C8), 126.4 (C1), 124.6 (C13), 123.7 (C2), 73.0 (C5), 65.4 (C4), 43.4 (C10); MS (ESI +ve) m/z: 1047.4245 ([M + H]+ C62H59N6O10 requires 1047.4287).
Author contributions
NHE proposed the study. SRB conducted the synthesis, characterization (with contribution from NRH) and analysis of all materials. NHE supervised SRB. SRB and NHE wrote the manuscript. All authors discussed and commented on the manuscript.
Conflicts of interest
There are no conflicts to declare.
Acknowledgements
SRB acknowledges EPSRC DTP PhD funding from the Engineering and Physical Sciences Research Council [EP/T518037/1] and a Sydney Andrew Scholarship from the Society of Chemical Industry. High resolution mass spectrometry data of 2 and 3 were recorded by Mr Karl Heaton at the University of York. High resolution mass spectrometry data of 4 was recorded by Mr Harry Mills (Lancaster University). We thank Dr Rebecca Spicer (Lancaster University) for the recording of low resolution mass spectrometry data and for useful discussions regarding analysis of the 1H NMR titration data. Underlying data for this paper are provided in the Experimental Section and ESI†. Electronic copies of NMR spectra (including fid files) will be available upon publication from: DOI:10.17635/lancaster/researchdata/640.
References
-
C. J. Bruns and J. F. Stoddart, The Nature of the Mechanical Bond: From Molecules to Machines, Wiley & Sons, Hoboken, 2017 Search PubMed.
- G. Gil-Ramírez, D. A. Leigh and A. J. Stephens, Angew. Chem., Int. Ed., 2015, 54, 6100–6150 CrossRef PubMed.
- M. Xue, Y. Yang, X. Chi, X. Yan and F. Huang, Chem. Rev., 2015, 115, 7398–7501 CrossRef CAS PubMed.
- For a review see: N. H. Evans, Eur. J. Org. Chem., 2019, 3320–3343 CrossRef CAS.
- Select examples from the primary literature:
(a) C. A. Hunter, J. Am. Chem. Soc., 1992, 114, 5303–5311 CrossRef CAS;
(b) F. Vögtle, S. Meier and R. Hoss, Angew. Chem., Int. Ed. Engl., 1992, 31, 1619–1622 CrossRef;
(c) F. Vögtle, M. Händel, S. Meier, S. Ottens-Hildebrandt, F. Ott and T. Schmidt, Liebigs Ann., 1995, 739–743 CrossRef;
(d) A. G. Johnston, D. A. Leigh, R. J. Pritchard and M. D. Deegan, Angew. Chem., Int. Ed. Engl., 1995, 34, 1209–1212 CrossRef CAS;
(e) J. F. Stoddart, P. R. Aston, P. T. Glink, P. A. Tasker, A. J. P. White and D. J. Williams, Chem. – Eur. J., 1996, 2, 729–736 CrossRef;
(f) A. G. Johnston, D. A. Leigh, A. Murphy, J. P. Smart and M. D. Deegan, J. Am. Chem. Soc., 1996, 118, 10662–10663 CrossRef CAS;
(g) D. A. Leigh, A. Murphy, J. P. Smart and A. M. Z. Slawin, Angew. Chem., Int. Ed. Engl., 1997, 36, 728–732 CrossRef CAS;
(h) G. M. Hübner, J. Gläser, C. Seel and F. Vögtle, Angew. Chem., Int. Ed., 1999, 38, 383–386 CrossRef;
(i) F. G. Gatti, D. A. Leigh, S. A. Nepogodiev, A. M. Z. Slawin, S. J. Teat and J. K. Y. Wong, J. Am. Chem. Soc., 2001, 123, 5983–5989 CrossRef CAS PubMed;
(j) A. Vidonne and D. Philp, Tetrahedron, 2008, 64, 8464–8475 CrossRef CAS;
(k) N. I. Hassan, V. del Amo, E. Calder and D. Philp, Org. Lett., 2011, 13, 458–461 CrossRef CAS PubMed;
(l) A. Tron, P. J. Thorton, M. Rocher, H. P. J. de Rouville, J. P. Desvergne, B. Kauffmann, T. Buffeteau, D. Cavagnat, J. H. R. Tucker and N. D. McClenaghan, Org. Lett., 2014, 16, 1358–1361 CrossRef CAS PubMed;
(m) A. Tron, P. J. Thorton, C. Lincheneau, J.-P. Desvergne, N. Spencer, J. H. R. Tucker and N. D. McClenaghan, J. Org. Chem., 2015, 80, 988–996 CrossRef CAS PubMed;
(n) C. N. Marrs and N. H. Evans, Org. Biomol. Chem., 2015, 13, 11021–11025 RSC;
(o) B. E. Fletcher, M. J. G. Peach and N. H. Evans, Org. Biomol. Chem., 2017, 15, 2797–2803 RSC;
(p) G. De Bo, G. Dolphijn, C. T. McTernan and D. A. Leigh, J. Am. Chem. Soc., 2017, 139, 8455–8457 CrossRef CAS PubMed;
(q) S. D. P. Fielden, D. A. Leigh, C. T. McTernan, B. Pérez-Saavedra and I. J. Vitorica-Yrezabal, J. Am. Chem. Soc., 2018, 140, 6049–6052 CrossRef CAS PubMed;
(r) J. E. M. Lewis, Org. Biomol. Chem., 2019, 17, 2442–2447 RSC;
(s) N. Hoyas Pérez, P. S. Sherin, V. Posligua, J. L. Greenfield, M. J. Fuchter, K. E. Jelfs, M. K. Kuimova and J. E. M. Lewis, Chem. Sci., 2022, 13, 11368–11375 RSC;
(t) S. R. Barlow, G. R. Akien and N. H. Evans, Org. Biomol. Chem., 2023, 21, 402–414 RSC.
-
(a) A. Altieri, G. Bottari, F. Dehez, D. A. Leigh, J. K. Y. Wong and F. Zerbetto, Angew. Chem., Int. Ed., 2003, 42, 2296–2300 CrossRef CAS PubMed;
(b) A. Altieri, F. G. Gatti, E. R. Kay, D. A. Leigh, D. Martel, F. Paolucci, A. M. Z. Slawin and J. K. Y. Wong, J. Am. Chem. Soc., 2003, 125, 8644–8654 CrossRef CAS PubMed;
(c) C. M. Keaveney and D. A. Leigh, Angew. Chem., Int. Ed., 2004, 43, 1222–1224 CrossRef CAS PubMed;
(d) D. A. Leigh, V. Marcos, T. Nalbantoglu, I. J. Vitorica-Yrezabal, F. Tuba Yasar and X. Zhu, J. Am. Chem. Soc., 2017, 139, 7104–7109 CrossRef CAS PubMed;
(e) A. H. G. David, R. Casares, J. M. Cuerva, A. G. Campaña and V. Blanco, J. Am. Chem. Soc., 2019, 141, 18064–18074 CrossRef CAS PubMed.
-
(a) D. A. Leigh, J. K. Y. Wong, F. Dehez and F. Zerbetto, Nature, 2003, 424, 174–179 CrossRef CAS PubMed;
(b) J. V. Hernandez, E. R. Kay and D. A. Leigh, Science, 2004, 306, 1532–1537 CrossRef CAS PubMed;
(c) S. Erbas-Cakmak, S. D. P. Fielden, U. Karaca, D. A. Leigh, C. T. McTernan, D. J. Tetlow and M. R. Wilson, Science, 2017, 358, 340–343 CrossRef CAS PubMed;
(d) S. Borsley, E. Kreidt and D. A. Leigh, Nature, 2022, 604, 80–85 CrossRef CAS PubMed.
-
(a) Y. Tachibana, N. Kihara and T. Takata, J. Am. Chem. Soc., 2004, 126, 3438–3439 CrossRef CAS PubMed;
(b) V. Blanco, A. Carlone, K. D. Hänni, D. A. Leigh and B. Lewandowski, Angew. Chem., Int. Ed., 2012, 51, 5166–5169 CrossRef CAS PubMed;
(c) A. Martinez-Cuezva, A. Saura-Sanmartin, T. Nicolas-Garcia, C. Navarro, R.-A. Orenes, M. Alajarin and J. Berna, Chem. Sci., 2017, 8, 3775–3780 RSC;
(d) J. Y. C. Lim, N. Yuntawattana, P. D. Beer and C. K. Williams, Angew. Chem., Int. Ed., 2019, 58, 6007–6011 CrossRef CAS PubMed;
(e) C. Biagini, S. D. P. Fielden, D. A. Leigh, F. Schaufelberger, S. Di Stefano and D. Thomas, Angew. Chem., Int. Ed., 2019, 58, 9876–9880 CrossRef CAS PubMed.
-
(a) J. A. Wisner, P. D. Beer, M. G. B. Drew and M. R. Sambrook, J. Am. Chem. Soc., 2002, 124, 12469–12476 CrossRef CAS PubMed;
(b) M. R. Sambrook, P. D. Beer, J. A. Wisner, R. L. Paul and A. R. Cowley, J. Am. Chem. Soc., 2004, 126, 15364–15635 CrossRef CAS PubMed;
(c) K.-Y. Ng, A. R. Cowley and P. D. Beer, Chem. Commun., 2006, 3676–3678 RSC;
(d) J. J. Gassensmith, S. Matthys, J. J. Lee, A. Wojcik, P. V. Kamat and B. D. Smith, Chem. – Eur. J., 2010, 16, 2916–2921 CrossRef CAS PubMed;
(e) N. H. Evans, C. J. Serpell and P. D. Beer, Chem. Commun., 2011, 47, 8775–8777 RSC;
(f) C. G. Collins, E. M. Peck, P. J. Kramer and B. D. Smith, Chem. Sci., 2013, 4, 2557–2563 RSC.
-
(a) J. R. Romero, G. Aragay and P. Ballester, Chem. Sci., 2017, 8, 491–498 RSC;
(b) J. M. Mercurio, F. Tyrrell, J. Cookson and P. D. Beer, Chem. Commun., 2013, 49, 10793–10795 RSC;
(c) J. Y. C. Lim, M. J. Cunningham, J. J. Davis and P. D. Beer, Dalton Trans., 2014, 43, 17274–17282 RSC;
(d) N. H. Evans, C. E. Gell and M. J. G. Peach, Org. Biomol. Chem., 2016, 14, 7972–7981 RSC.
- F. Zapata, O. A. Blackburn, M. J. Langton, S. Faulkner and P. D. Beer, Chem. Commun., 2013, 49, 8157–8159 RSC.
-
(a) M. Chen, S. Han, L. Jiang, S. Zhou, F. Jiang, Z. Xu, J. Liang and S. Zhang, Chem. Commun., 2010, 46, 3932–3934 RSC;
(b) S. Saha, S. Santra and P. Ghosh, Org. Lett., 2015, 17, 1854–1857 CrossRef CAS PubMed.
-
(a) L. M. Hancock and P. D. Beer, Chem. Commun., 2011, 47, 6012–6014 RSC;
(b) R. C. Knighton and P. D. Beer, Chem. Commun., 2014, 50, 1540–1542 RSC;
(c) R. C. Knighton and P. D. Beer, Org. Chem. Front., 2021, 8, 2468–2472 RSC.
-
(a) M. Kamenica, R. R. Kothur, A. Willows, B. A. Patel and P. J. Cragg, Sensors, 2017, 17, 2430 CrossRef PubMed;
(b) E. Villemin and O. Raccurt, Coord. Chem. Rev., 2021, 435, 213801 CrossRef CAS.
- H.-B. Choi, J.-S. Ryu, W.-J. Shin and N. Vigier, Nat. Commun., 2019, 10, 5371 CrossRef CAS PubMed.
-
(a) C. Dietrich-Buchecker and J.-P. Sauvage, Chem. Commun., 1999, 615–616 RSC;
(b) G. Kaiser, T. Jarrosson, S. Otto, Y.-F. Ng, A. D. Bond and J. K. M. Sanders, Angew. Chem., Int. Ed., 2004, 43, 1959–1962 CrossRef CAS PubMed;
(c) S. A. Vignon, T. Jarrosson, T. Iijima, H.-R. Tseng, J. K. M. Sanders and J. F. Stoddart, J. Am. Chem. Soc., 2004, 126, 9884–9885 CrossRef CAS PubMed;
(d) T. Iijima, H. R. Tseng, T. Jarrosson, J. K. M. Sanders, F. Marchioni, M. Venturi, E. Apostoli, V. Balzani and J. F. Stoddart, Chem. – Eur. J., 2004, 10, 6375–6392 CrossRef CAS PubMed.
- Chiu and co-workers have prepared a range of MIMs using alkali metal cation templation: A. Inthasot, S.-T. Tung and S. H. Chiu, Acc. Chem. Res., 2018, 51, 1324–1337 CrossRef CAS PubMed.
-
(a) Y. Tokunga, S. Kakuchi and Y. Shimomura, Heterocycles, 2004, 63, 2713–2718 CrossRef;
(b) K. Hiratani, M. Kaneyama, Y. Nagawa, E. Koyama and M. Kanesato, J. Am. Chem. Soc., 2004, 126, 13568–13569 CrossRef CAS PubMed;
(c) Y. Nagawa, J.-I. Suga, K. Hiratani, E. Koyama and M. Kanesato, Chem. Commun., 2005, 749–751 RSC;
(d) V. K. Munasinghe, J. Pancholi, D. Manawadu, Z. Zhang and P. D. Beer, Chem. – Eur. J., 2022, 28, e202201209 CrossRef CAS PubMed.
- Initial attempts to oxidize the pyridine motif with Oxone®, were thwarted by the poor solubility of [2]catenane 2 under the reaction conditions.
- While it is anticipated the lowest energy co-conformation of uncomplexed [2]catenane 4 in solution is that depicted in Scheme 2 and in the X-ray crystal structure depicted in Fig. 2b, in CDCl3 solution there is rapid rotation of the rings relative to one another, as evidenced by the appearance of the 1H NMR spectrum in Fig. 1c.
-
(a) “Supramolecular.org – Binding Constant Calculators” can be found under https://supramolecular.org (accessed 15th July 2023);
(b) D. B. Hibbert and P. Thordarson, Chem. Commun., 2016, 52, 12792–12805 RSC;
(c) P. Thordarson, Chem. Soc. Rev., 2011, 40, 1305–1323 RSC.
- Attempts at calculating association constants for the protonation binding event are detailed in the ESI, pp. S10 and S11.†.
- Attempt to fit titration data for 4 with TFA for the 1
:
1 binding isotherm can be found under https://app.supramolecular.org/bindfit/view/57e295c3-57ca-410e-8d49-72bcf343dada (accessed 22nd June 2023).
- Attempt to fit titration data for 4 with TFA for the 1
:
2 binding isotherm can be found under https://app.supramolecular.org/bindfit/view/034b92d0-c048-4fa1-87e1-1601c5fe91c1 (accessed 15th July 2023).
- Considering the rapid rotation of the identical rings of uncomplexed [2]catenane 4, a 1H–1H ROESY NMR experiment will not unambiguously reveal whether pirouetting has occurred upon cation coordination.
- LiClO4 was used as the source of Li+ and NaBArF as the source of Na+. Different non-coordinating counter-anions were used due to issues with maintaining solubility in appropriate solvent systems for the determination of association constant data.
- Due to the insolubility of the oxidized analogue of macrocycle 3, it was not possible to undertake titrations to ascertain the alkali metal cation binding properties of the non-interlocked rings of catenane 4.
- Fitted titration data for 4 with LiClO4 can be found under https://app.supramolecular.org/bindfit/view/29ae08eb-c98c-4e4a-8538-d83822d473a7 (accessed 22nd June 2023).
- Fitted titration data for 4 with NaBArF can be found under https://app.supramolecular.org/bindfit/view/e26fdd6b-e548-42a4-80fa-da019c6239f5 (accessed 22nd June 2023).
- In addition to examples of Li+ binding by MIMs listed in ref. 18, select examples of quantitative determination of association constants for alkali metal cation binding by MIMs not containing pyridine-N-oxide(s) include:
(a) A. V. Leontiev, C. A. Jemmett and P. D. Beer, Chem. – Eur. J., 2011, 17, 816–825 CrossRef CAS PubMed;
(b) A. V. Leontiev, C. J. Serpell, N. G. White and P. D. Beer, Chem. Sci., 2011, 2, 922–927 RSC;
(c) H. M. Tay, Y. C. Tse, A. Docker, C. Gateley, A. L. Thompson, H. Kuhn, Z. Zhang and P. D. Beer, Angew. Chem., Int. Ed., 2023, 62, e202214785 CrossRef CAS PubMed;
(d) H. M. Tay, A. Docker, Y. C. Tse and P. D. Beer, Chem. – Eur. J., 2023, 29, e202301316 CrossRef PubMed;
(e) A. Arun, A. Docker, H. M. Tay and P. D. Beer, Chem. – Eur. J., 2023, 29, e202301446 CrossRef CAS PubMed.
Footnote |
† Electronic supplementary information (ESI) available: Copies of characterization data; further data and protocols for titrations and NMR spectra. CCDC 2305367 and 2305368 for 4 and 2. For ESI and crystallographic data in CIF or other electronic format see DOI: https://doi.org/10.1039/d4ob00176a |
|
This journal is © The Royal Society of Chemistry 2024 |
Click here to see how this site uses Cookies. View our privacy policy here.