DOI:
10.1039/D4NA00414K
(Communication)
Nanoscale Adv., 2024,
6, 4360-4368
Magnetically recoverable Fe3O4@SiO2@SBA-3@2-ATP-Cu: an improved catalyst for the synthesis of 5-substituted 1H-tetrazoles†
Received
16th May 2024
, Accepted 6th July 2024
First published on 15th July 2024
Abstract
Functionalization of Fe3O4@SiO2@SBA-3 with double-charged 3-chloropropyltrimethoxysilane (CPTMS) and 2-aminophenol, followed by mechanical mixing of the solid product with copper(I) chloride produces a new, greener and efficient Fe3O4@SiO2@SBA-3@2-ATP-Cu catalyst for the synthesis of 5-substituted 1H-tetrazoles. XRD, SEM, atomic absorption, TGA, N2 adsorption–desorption, and VSM analyses were performed for the characterization of the Fe3O4@SiO2@SBA-3@2-ATP-Cu structure. Nitrogen adsorption–desorption analysis revealed that Fe3O4@SiO2@SBA-3@2-ATP-Cu has a surface area of 242 m2 g−1 and a total pore volume of 55.72 cm3 g−1. In synthesizing 5-substituted 1H-tetrazoles, Fe3O4@SiO2@SBA-3@2-ATP-Cu shows superior yields in short reaction times at 120 °C. This catalyst also showed high thermal stability and recyclability at least for 4 runs without apparent loss of efficiency.
Introduction
For decades, researchers have widely searched for ways to synthesize porous materials with high surface area and very diverse contents.1–5 Among these porous solid compounds, mesoporous materials such as MCM-41, MCM-48, SBA-15, and SBA-3 have attracted more attention than others due to their applications in many areas.6–10 The main differences in the preparation of mesoporous materials are the type of used templates (ionic surfactant for MCM-41 and triblock copolymer in the case of SBA-15) and the synthesis medium (MCM-41 prepared in the basic medium, and SBA-15 prepared in the acidic medium).11 SBA-type materials (SBA-15, SBA-3) show much higher stability than MCM-41 due to the contribution of some micropores connecting mesopores.12 However, the chemical inertness of SBA-15, which is mainly composed of silicon dioxide, greatly limits its application in chemical engineering, especially in heterogeneous catalysis. Incorporated heteroatoms are commonly used to form active sites in the mesoporous silica framework and confer acid sites and catalytic activities to the silica matrix.13–15 In the literature, applications of SBA-3 as a catalyst support are rarely reported.16–20 The use of magnetic nanoparticles provides both economic and ecological benefits due to their properties, low toxicity, and simple separation by an external magnetic field.21–23 Magnetic nanoparticles have been widely employed as novel magnetically recoverable catalysts in medicinal applications,24–26 drug delivery,27,28 and industry.29,30
On the other hand, tetrazoles are a valuable class of heterocycles, which have been recently used as both anticancer31,32 and antimicrobial agents.33,34 They have received increased attention due to their potential biological activities,35–37 and industrial applications.38–40 A versatile method for the preparation of tetrazole is based on [3 + 2] cycloaddition of the azide ion and organic nitriles in the presence of a catalyst.41–47 Although the reported methods are effective, they are limited due to the use of toxic solvents, tedious workup, difficulty in separation, and expensive catalysts. As part of our continuous efforts into the design of modified magnetic mesoporous silica nanoparticles and their application in organic synthesis,48,49 we report here the preparation of Fe3O4@SiO2@SBA-3@2-ATP-Cu (Scheme 1), and its performance for the synthesis of 5- substituted 1H-tetrazoles.
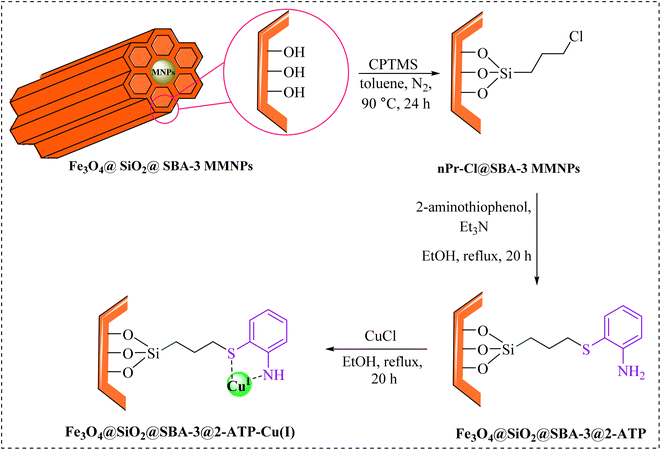 |
| Scheme 1 Preparation of Fe3O4@SiO2@SBA-3@2-ATP-Cu. | |
Experimental
Preparation of Fe3O4@SiO2@SBA-3@2-ATP-Cu
Fe3O4@SiO2@SBA-3 was readily synthesized similar to a previously reported study.48 Briefly, the catalyst was prepared by adding 1.5 mL of (3-chloropropyl)triethoxysilane (CPTES) to a suspension of Fe3O4@SiO2@SBA-3 (1 g) in 25 mL of toluene, followed by mechanical mixing of the solid product at 90 °C for 24 h. The Fe3O4@SiO2@SBA-3@CPTMS material (1 g) was then treated with 2-aminothiophenol (1.5 g) and trimethylamine (3 mL), followed by subsequent thermal treatment of the reaction mixture at 78 °C in ethanol for 20 h, affording Fe3O4@SiO2@SBA-3@CPTMS@ATP, and then the resulting material was then washed with ethanol, and dried at room temperature. Finally, Fe3O4@SiO2@SBA-3@CPTMS@ATP (1 g) in 25 mL of ethanol was sonicated for 10 min. Then, the resulting nanoparticles (Fe3O4@SiO2@SBA-3@CPTMS@ATP) were filtered, washed with ethanol (70% v/v), and dried at room temperature. The obtained Fe3O4@SiO2@SBA-3@CPTMS@ATP (0.5 g) was dispersed in 25 mL of ethanol and was sonicated for 10 min, and then copper(I) chloride (2 mmol) was added to the reaction mixture. The reaction mixture was stirred at 80 °C for 24 h. After the separation of the final product by an external magnet, Fe3O4@SiO2@SBA-3@2-ATP-Cu was washed with ethanol and consequently dried in an oven at 55 °C.
General procedure for the synthesis of 5-substituted 1H-tetrazoles
A sealed tube was charged with nitrile (1 mmol), sodium azide (1.1 mmol), and Fe3O4@SiO2@SBA-3@2-ATP-Cu (60 mg) at 120 °C, and then polyethylene glycol (1 mL) was added. The resulting mixture was stirred under reflux until the completion of the reaction. Then the reaction mixture was acidified with 10 N HCl and the product was extracted with ethyl acetate, washed with water, dried over sodium sulfate, and concentrated. The product was purified by column chromatography on silica gel (hexane
:
ethyl acetate (4
:
1)) to afford the desired product.
Results and discussion
After the preparation of the catalyst, Fe3O4@SiO2@SBA-3@2-ATP-Cu was extensively studied with several techniques such as XRD, SEM, atomic absorption, TGA, N2 adsorption–desorption, and VSM. The XRD patterns of mesoporous silica (1a), Fe3O4@SiO2@SBA-3@CPTMS (1b), Fe3O4@SiO2@SBA-3@CPTMS@ATP (1c), and Fe3O4@SiO2@SBA-3@2-ATP-Cu (1d) samples are shown in Fig. (1). The XRD patterns of arrayed silica nanoparticles show three diffraction peaks that can be indexed as the (111), (220), and (331) reflections that are arranged in a face-centered-cubic (fcc) structure (Fig. 1).50 For all samples, peaks at 30.39°, 35.66°, 53.96°, 57.30°, and 62.94° correspond to the (220), (400), (422), (511), and (440) planes, which is in agreement with the literature (Fig. 1).51 The XRD patterns of 2-aminothiophenol were not found in the XRD spectra.52 The copper nanoparticles are responsible for the diffraction planes of (002)53 and the XRD pattern of Fe3O4@SiO2@SBA-3@2-ATP-Cu, which showed the presence of characteristic peaks at 2θ values of 32, 42.3°, 55°, and 75°, related to (110), (112), (020), and (222) planes for both the conventional and green CuO, respectively.54
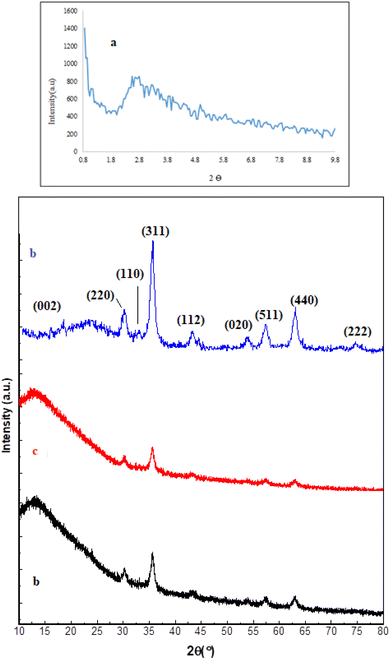 |
| Fig. 1 XRD patterns of mesoporous silica (a), Fe3O4@SiO2@SBA-3@CPTMS (b), Fe3O4@SiO2@SBA-3@CPTMS@ATP (c), and Fe3O4@SiO2@SBA-3@2-ATP-Cu (d). | |
The Cu content of the prepared mesoporous magnetic material was determined by atomic absorption spectroscopy (AAS) and calculated to be 3.6 × 10−4 mol g−1.
Fig. 2 displays the thermogravimetric analysis (TGA) of Fe3O4@SiO2@SBA-3@2-ATP-Cu in the temperature range of 50–1000 °C. Results revealed a weight loss of about 8 wt% at a temperature of under 250 °C, which was related to the loss of water and the organic solvents. The maximum mass loss that occurred in the temperature range of 250 to 750 °C is due to the decomposition of immobilized organic moieties on the surface of magnetic mesoporous silica nanoparticles, and the amount of organic components was found to be about 10% of the total solid catalyst.
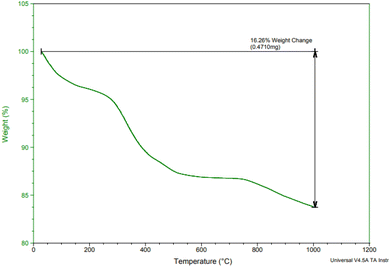 |
| Fig. 2 TGA graph of Fe3O4@SiO2@SBA-3@2-ATP-Cu. | |
Fig. 3 shows the dependence of magnetization on the applied field (M–H) for Fe3O4@SiO2@SBA-3@2-ATP-Cu at room temperature. The modified magnetic mesoporous silica nanoparticle sample exhibited decreased magnetism due to the loading of 2-ATP-Cu on the surface of Fe3O4@SiO2@SBA-3 compared to the bare Fe3O4@SiO2@SBA-3.48
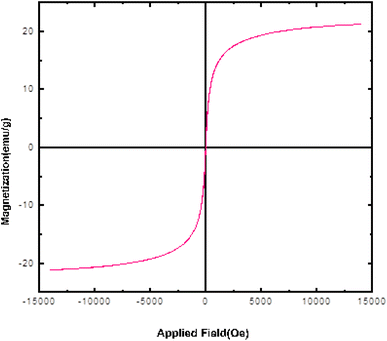 |
| Fig. 3 Magnetization curves for Fe3O4@SiO2@SBA-3@2-ATP-Cu at room temperature. | |
The size and structure of Fe3O4@SiO2@SBA-3@2-ATP-Cu were evaluated using FE-SEM (Fig. 4). The results of the analysis showed uniformity and sphere-like morphology.
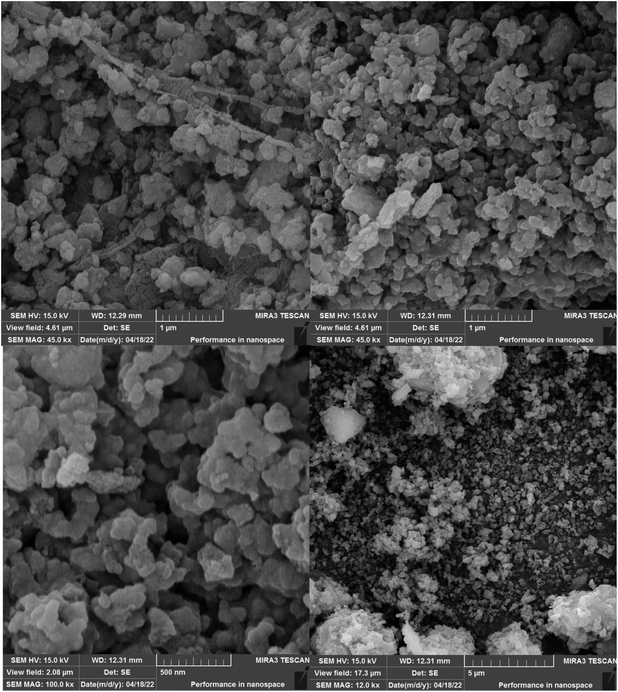 |
| Fig. 4 SEM images of Fe3O4@SiO2@SBA-3@2-ATP-Cu. | |
Nitrogen adsorption–desorption analysis gives us information on the mesoporous nature of Fe3O4@SiO2@SBA-3@2-ATP-Cu. Fig. 5 shows a type II isotherm with an H3 type hysteresis loop in the range of 0.0–0.97 (P/P0) for Fe3O4@SiO2@SBA-3@2-ATP-Cu. The BET surface area of the prepared material was found to be 242 m2 g−1. The textural data of the nanocatalysts are shown in Table 1. The calculated mean pore diameter from the adsorption–desorption data using the BET procedure is 2.48 nm which further confirms the formation of the mesoporous structure in the synthesized material as shown in (Fig. 6, and Table 2).
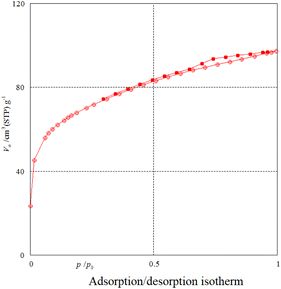 |
| Fig. 5 N2-adsorption isotherms of Fe3O4@SiO2@SBA-3@2-ATP-Cu. | |
Table 1 Nitrogen adsorption–desorption data for Fe3O4@SiO2@SBA-3@2-ATP-Cu
Entry |
BET plot |
V
m
|
55.72 cm3 g−1 |
a
s,BET
|
242 m2 g−1 |
Total pore volume (p/p0 = 0.990) |
0.15 cm3 g−1 |
Mean pore diameter |
2.48 nm |
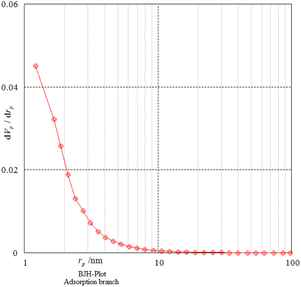 |
| Fig. 6 BJH-plot of Fe3O4@SiO2@SBA-3@2-ATP-Cu. | |
Table 2 BJH-plot data for Fe3O4@SiO2@SBA-3@2-ATP-Cu
Plot data |
Adsorption branch |
V
p
|
0.0.07 cm3 g−1 |
r
p, peak(area) |
1.22 nm |
a
p
|
82.52 m2g−1 |
Catalytic activity of Fe3O4@SiO2@SBA-3@2-ATP-Cu for the synthesis of 5-substituted 1H-tetrazoles
To explore the catalytic activity of magnetic mesoporous silica nanoparticles, cycloaddition reactions of nitriles with sodium azide were investigated. Initially, the reaction of benzonitrile with sodium azide was selected as a model reaction to optimize the reaction conditions, such as various amounts of catalyst, solvents, and temperature (Table 3). First, the effect of solvents was studied and it was observed that the reaction was highly effective in PEG medium. Furthermore, the high efficiency of the reaction depended strongly on the amount of catalyst, which the reaction was completed in the presence of 60 mg of Fe3O4@SiO2@SBA-3@2-ATP-Cu for 1 mmol of benzonitrile. The ideal temperature for the reaction was found to be 120 °C. Under these conditions, a wide range of benzonitrile derivatives were tolerated. This reaction proceeded well giving the desired products in high to excellent yields (Table 4).
Table 3 The optimization of reaction conditionsa

|
Entry |
Solvent |
Catalyst (mg) |
Temperature (°C) |
Time (min) |
Yieldb (%) |
Reaction conditions: benzonitrile (1 mmol), sodium azide (1.1 mmol), catalyst, solvent (1 mL).
Isolated yield.
|
1 |
PEG |
60 |
120 |
65 |
94 |
2 |
DMSO |
60 |
120 |
65 |
78 |
3 |
DMF |
60 |
120 |
65 |
82 |
4 |
H2O |
60 |
Reflux |
65 |
N. R |
5 |
PEG |
50 |
120 |
65 |
81 |
6 |
PEG |
40 |
120 |
65 |
73 |
7 |
PEG |
60 |
100 |
65 |
77 |
8 |
PEG |
60 |
80 |
65 |
48 |
Table 4 Synthesis of 5-substituted-1H-tetrazoles in the presence of Fe3O4@SiO2@SBA-3@2-ATP-Cu
Recyclability of the Fe3O4@SiO2@SBA-3@2-ATP-Cu catalyst
The recovery of a catalyst is valuable and interesting from both the economic and the environmental aspects. To gain a better insight into the heterogeneity of Fe3O4@SiO2@SBA-3@2-ATP-Cu, a catalytic reusability test was conducted using benzonitrile as a starting nitrile material. After each catalytic run, the catalyst was removed with an external magnet, washed, dried, and reused directly for the next run. Within four catalytic runs, the catalyst presented excellent reusability with no significant loss of the original catalytic yield (Fig. 7).
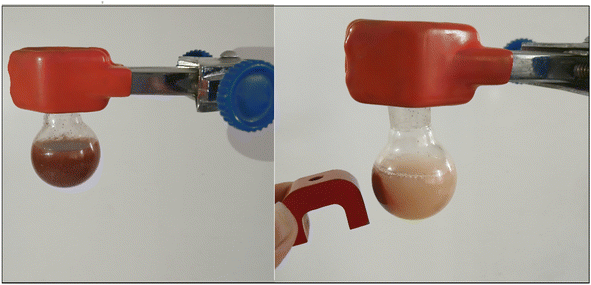 |
| Fig. 7 Recyclability of Fe3O4@SiO2@SBA-3@2-ATP-Cu. | |
To show the accessibility of the present work, we summarize some of the results for the preparation of 5-substituted-1H-tetrazoles in Table 5. The results show that modified magnetic mesoporous silica is the most efficient catalyst in terms of reaction time and yield.
Table 5 Comparison of the results for modified Fe3O4@SiO2@SBA-3@2-ATP-Cu with other catalysts for the formation of 5-phenyl-1H-tetrazole
Entry |
Catalyst |
Conditions |
Time (h) |
Yield (%) |
References |
1 |
CuO/aluminosilicate |
DMF, reflux |
8 |
89 |
59
|
2 |
SiO2-MTSA |
DMF, 120 °C |
6 |
83 |
60
|
3 |
Sm@l-MSN |
DMF, 100 °C |
5 |
94 |
61
|
4 |
Zn/Al hydrotalcite |
DMF, 120 °C |
12 |
84 |
62
|
5 |
Fe3O4@SiO2@SBA-3@2-ATP-Cu |
PEG, 120 °C |
65 min |
94 |
This work |
Conclusion
In conclusion, Fe3O4@SiO2@SBA-3@2-ATP-Cu was synthesized by a co-precipitation technique and exhibited efficient catalytic performance and outstanding recyclability for the synthesis of 5-substituted 1H-tetrazoles under relatively mild conditions. We hope the design and preparation of new magnetic mesoporous silica nanoparticles owing to their high surface area and thermal stability to be a step forward in the field of heterogenous catalysis development.
Data availability
All data generated or analyzed during this study are included in this published article [and its ESI† files].
Author contributions
Zahra Heidarnezhad: laboratory work, investigation, methodology. Arash Ghorbani-Choghamarani: resources, writing – review & editing, conceptualization, supervision. Zahra Taherinia: methodology, investigation, writing – original draft, validation.
Conflicts of interest
The authors declare that they have no known competing financial interests or personal relationships that could have appeared to influence the work reported in this paper.
Acknowledgements
The authors would like to thank the research facilities of Ilam University and Bu-Ali Sina University for financial support of this research project.
References
- Z. A. Alothman, Materials, 2012, 5, 2874–2902 CrossRef CAS.
- Y. Ma, W. Tong, H. Zhou and S. L. Suib, Microporous Mesoporous Mater., 2000, 37, 243–252 CrossRef CAS.
- W. L. Li, K. Lu and J. Y. Walz, Int. Mater. Rev., 2012, 57, 37–60 CrossRef CAS.
- K. Khanafer and K. Vafai, Renewable Energy, 2018, 123, 398–406 CrossRef CAS.
- L. Zhu, D. Shen and K. H. Luo, J. Hazard. Mater., 2020, 389, 122102 CrossRef CAS PubMed.
- L. Ge, Y. Feng, Y. Dai, R. Wang and T. Ge, Chem. Eng. J., 2023, 452, 139116 CrossRef CAS.
- I. Mahboob, S. Shafique, I. Shafiq, P. Akhter, A. S. Belousov, P. L. Show, Y. K. Park and M. Hussain, Environ. Res., 2023, 218, 114983 CrossRef CAS PubMed.
- X. Xing, X. Ren, N. S. Alharbi and C. Chen, J. Colloid Interface Sci., 2023, 629, 744–754 CrossRef CAS PubMed.
- C. Rieg, M. Kirchhof, K. Gugeler, A. K. Beurer, L. Stein, K. Dirnberger, W. Frey, J. R. Bruckner, Y. Traa, J. Kästner, S. Ludwigs, S. Laschat and M. Dyballa, Catal. Sci. Technol., 2022, 13, 410–425 RSC.
- R. Zaleski, M. Gorgol, J. Goworek, A. Kierys, M. Pietrow and B. Zgardzińska, Adsorption, 2019, 25, 881–887 CrossRef CAS.
- B. Kilos, A. Tuel, M. Ziolek and J. C. Volta, Catal. Today, 2006, 118, 416–424 CrossRef CAS.
- A. Galarneau, M. Nader, F. Guenneau, F. Di Renzo and A. Gedeon, J. Phys. Chem. C, 2007, 111, 8268–8277 CrossRef CAS.
- K. kang Miao, X. lin Luo, W. Wang, J. le Guo, S. fan Guo, F. jiu Cao, Y. qiao Hu, P. mei Chang and G. dong Feng, Microporous Mesoporous Mater., 2019, 289, 109640 CrossRef.
- H. Aghayan, A. R. Khanchi, T. Yousefi and H. Ghasemi, J. Nucl. Mater., 2017, 496, 207–214 CrossRef CAS.
- Y. Han, F. S. Xiao, S. Wu, Y. Sun, X. Meng, D. Li, S. Lin, F. Deng and X. Ai, J. Phys. Chem. B, 2001, 105, 7963–7966 CrossRef CAS.
- J. Florek-Milewska, P. Decyk and M. Ziolek, Appl. Catal., A, 2011, 393, 215–224 CrossRef CAS.
- A. Grünberg, X. Yeping, H. Breitzke and G. Buntkowsky, Chem.–Eur. J., 2010, 16, 6993–6998 CrossRef PubMed.
- M. S. Khayoon and B. H. Hameed, Appl. Catal., A, 2013, 460–461, 61–69 CrossRef CAS.
- E. Janiszewska, A. Held, K. Nowińska and S. Kowalak, RSC Adv., 2019, 9, 4671–4681 RSC.
- E. Janiszewska, M. Zieliński, M. Kot, E. Kowalewski and A. Śrębowata, ChemCatChem, 2018, 10, 4109–4118 CrossRef CAS.
- M. I. A. Abdel Maksoud, A. M. Elgarahy, C. Farrell, A. H. Al-Muhtaseb, D. W. Rooney and A. I. Osman, Coord. Chem. Rev., 2020, 403, 213096 CrossRef.
- F. S. A. Khan, N. M. Mubarak, M. Khalid, R. Walvekar, E. C. Abdullah, S. A. Mazari, S. Nizamuddin and R. R. Karri, Environ. Sci. Pollut. Res., 2020, 27, 24342–24356 CrossRef CAS PubMed.
- S. A. H. Martínez, E. M. Melchor-Martínez, J. A. R. Hernández, R. Parra-Saldívar and H. M. N. Iqbal, Fuel, 2022, 312, 122927 CrossRef.
- S. Khizar, E. Elkalla, N. Zine, N. Jaffrezic-Renault, A. Errachid and A. Elaissari, Expert Opin. Drug Deliv., 2023, 20, 189–204 CrossRef PubMed.
- Z. Hedayatnasab, A. Ramazani Saadatabadi, H. Shirgahi and M. R. Mozafari, Mater. Res. Bull., 2023, 157, 112035 CrossRef CAS.
- G. G. Flores-Rojas, F. López-Saucedo, R. Vera-Graziano, E. Mendizabal and E. Bucio, Macromol, 2022, 2, 374–390 CAS.
- K. Ghosal, S. Chatterjee, S. Thomas and P. Roy, AAPS PharmSciTech, 2023, 24, 25 CrossRef CAS PubMed.
- Z. Li, W. Wan, Z. Bai, B. Peng, X. Wang, L. Cui, Z. Liu, K. Lin, J. Yang, J. Hao and F. Tian, Sensor. Actuator. B Chem., 2023, 375, 132869 CrossRef CAS.
- P. Amruth Maroju, R. Ganesan and J. Ray Dutta, Mater. Today Proc., 2023, 72, 62–66 CrossRef CAS.
- T. Lü, X. Zhang, R. Ma, D. Qi, Y. Sun, D. Zhang, J. Huang and H. Zhao, Sep. Purif. Technol., 2023, 309, 123097 CrossRef.
- J. Zhang, S. Wang, Y. Ba and Z. Xu, Eur. J. Med. Chem., 2019, 178, 341–351 CrossRef CAS PubMed.
- A. Verma, B. Kaur, S. Venugopal, P. Wadhwa, S. Sahu, P. Kaur, D. Kumar and A. Sharma, Chem. Biol. Drug Des., 2022, 100, 419–442 CrossRef CAS PubMed.
- F. Gao, J. Xiao and G. Huang, Eur. J. Med. Chem., 2019, 184, 111744 CrossRef CAS PubMed.
- S. A. F. Rostom, H. M. A. Ashour, H. A. A. El Razik, A. E. F. H. A. El Fattah and N. N. El-Din, Bioorganic Med. Chem., 2009, 17, 2410–2422 CrossRef CAS PubMed.
- R. K. Uppadhayay, A. Kumar, J. Teotia and A. Singh, Russ. J. Org. Chem., 2022, 58, 1801–1811 CrossRef CAS.
- S. Bayomi, M. A. Moustafa, A. R. Maarouf and M. H. Aboutaleb, J. Am. Sci., 2016, 12, 40–56 Search PubMed.
- A. Ghorbani-Choghamarani and Z. Taherinia, Aust. J. Chem., 2017, 70, 1127–1137 CrossRef CAS.
- M. Nasrollahzadeh, Z. Nezafat, N. S. S. Bidgoli and N. Shafiei, Mol. Catal., 2021, 513, 111788 CrossRef CAS.
- A. H. Dabbagh and Y. Mansoori, Dyes Pigm., 2002, 54, 37–46 CrossRef CAS.
- M. Rösch, M. S. Gruhne, M. Lommel, S. M. J. Endraß and J. Stierstorfer, Inorg. Chem., 2023, 62, 1488–1507 CrossRef PubMed.
- S. K. Prajapti, A. Nagarsenkar and B. N. Babu, Tetrahedron Lett., 2014, 55, 3507–3510 CrossRef CAS.
- P. Mani, A. K. Singh and S. K. Awasthi, Tetrahedron Lett., 2014, 55, 1879–1882 CrossRef CAS.
- S. Bhagat and V. Telvekar, Synlett, 2018, 29, 874–879 CrossRef CAS.
- D. Amantini, R. Beleggia, F. Fringuelli, F. Pizzo and L. Vaccaro, J. Org. Chem., 2004, 69, 2896–2898 CrossRef CAS PubMed.
- F. Himo, Z. P. Demko, L. Noodleman and K. B. Sharpless, J. Am. Chem. Soc., 2003, 125, 9983–9987 CrossRef CAS PubMed.
- S. Kumar, S. Dubey, N. Saxena and S. K. Awasthi, Tetrahedron Lett., 2014, 55, 6034–6038 CrossRef CAS.
- B. Agrahari, S. Layek, R. Ganguly and D. D. Pathak, New J. Chem., 2018, 42, 13754–13762 RSC.
- Z. Heidarnezhad, A. Ghorbani-Choghamarani and Z. Taherinia, Catal. Lett., 2022, 152, 3178–3191 CrossRef CAS.
- B. Tahmasbi and A. Ghorbani-Choghamarani, New J. Chem., 2019, 43, 14485–14501 RSC.
- J. Wang, A. Sugawara-Narutaki, M. Fukao, T. Yokoi, A. Shimojima and T. Okubo, ACS Appl. Mater. Interfaces, 2011, 3, 1538–1544 CrossRef CAS PubMed.
- A. Aashima, S. Uppal, A. Arora, S. Gautam, S. Singh, R. J. Choudhary and S. K. Mehta, RSC Adv., 2019, 9, 23129–23141 RSC.
- T. E. Karpov, A. Rogova, D. R. Akhmetova, Y. A. Tishchenko, A. V. Chinakova, D. V. Lipin, N. V. Gavrilova, I. A. Gorbunova, S. A. Shipilovskikh and A. S. Timin, Biomater. Sci., 2024, 12, 3431–3445 RSC.
- R. O. Aljedaani, S. A. Kosa and M. Abdel Salam, Molecules, 2022, 28, 16 CrossRef PubMed.
- G. Anbalagan, B. Subramanian, V. Suresh and P. Sivaperumal, Cureus, 2024, 16(4), e57366 Search PubMed.
- M. A. Jani and K. Bahrami, Appl. Organomet. Chem., 2020, 34(12), e6014 CrossRef CAS.
- E. Aali, M. Gholizadeh and N. Noroozi-Shad, J. Mol. Struct., 2022, 1247, 131289 CrossRef CAS.
- P. Movaheditabar, M. Javaherian and V. Nobakht, J. Iran. Chem. Soc., 2022, 19, 1805–1816 CrossRef CAS.
- N. Moeini, M. Ghadermazi and S. Molaei, J. Mol. Struct., 2022, 1251, 131982 CrossRef CAS.
- P. Movaheditabar, M. Javaherian and V. Nobakht, React. Kinet. Mech. Catal., 2017, 122, 217–228 CrossRef CAS.
- D. H. Mahajan, D. Mistry and H. Tailor, Chem. Sci., 2018, 7(4), 676–686 CAS.
- P. K. Samanta, R. Biswas, T. Das, M. Nandi, B. Adhikary, R. M. Richards and P. Biswas, J. Porous Mater., 2019, 26, 145–155 CrossRef CAS.
- M. L. Kantam, K. B. Shiva Kumar and K. Phani Raja, J. Mol. Catal. A Chem., 2006, 247, 186–188 CrossRef CAS.
|
This journal is © The Royal Society of Chemistry 2024 |
Click here to see how this site uses Cookies. View our privacy policy here.