Cation/anion-exchange mode switching chromatography utilizing pH-responsive mixed charge polymer-modified silica beads†
Received
15th June 2023
, Accepted 2nd November 2023
First published on 3rd November 2023
Abstract
The separation capacity of a column typically remains constant. By applying stimuli-responsive materials to the stationary phase, the separation capacity in a single column can be tuned; however, the separation mode is not completely switched. In this study, we aimed to develop a cation/anion-exchange mode switching chromatography approach, in which the monomer ratio is adjusted, enabling the surface charge to become either negative or positive in response to mobile phase pH. Three types of beads were prepared, each modified with a pH-responsive mixed-charge polymer combining a cationic monomer, a pH-responsive carboxylic acid monomer, a neutral monomer, and a cross-linking monomer. The composition ratio of the cationic monomer to the pH-responsive carboxylic acid monomer was set at 1
:
2 so that the cation-exchange mode occurs at a pH above the pKa and the anion-exchange mode occurs below the pKa. At a pH below the pKa, the retention factor of the negatively charged compound increased. In contrast, at a pH above the pKa, the retention factor of the positively charged compound increased, confirming the charge switching on the bead surface. Switching to the cation- and anion-exchange mode enabled the separation of five basic antidepressants and acidic non-steroidal anti-inflammatory drugs, respectively. Utilizing a pH-responsive mixed-charge polymer, we attributed a cation/anion-exchange mode to a single column.
Design, System, Application
Among zwitterionic polymers, mixed-charge polymers have charges on different side chains, making it possible to design polymers with various combinations and ratios of cationic and anionic monomers. Furthermore, it is possible to drastically and completely switch the charge of the surfaces that are modified with these polymers. In this study, we designed and developed a chromatographic system for drastic and complete switching between cation- and anion-exchange modes by utilizing the properties of pH-responsive mixed-charge polymers. The pH at which the surface charge switched between positive and negative could be controlled by adjusting cationic, pH-responsive anionic, and neutral monomers. The elution order of cationic and anionic compounds was completely reversed by changing the pH of mobile phase. These findings suggest that pH-responsive mixed-charge polymers can modulate interactions with charged compounds, and can be applied to control the elution of these compounds by switching the mobile phase pH.
|
Introduction
High-performance liquid chromatography (HPLC) is an important analytical technique that can separate and detect target analytes with high accuracy and reproducibility. It is widely employed for pharmaceuticals,1 food,2 and environmental analysis.3 As analytical needs have diversified, columns with a variety of separation modes such as reversed-phase,4 ion-exchange,5 and hydrophilic interaction6 have been developed. In addition, the mixed-mode stationary phase of the separation modes mentioned above possesses several interaction mechanisms with the analysis target, and exhibits high selectivity even during the separation of complicated samples comprising charged, polar, and nonpolar compounds.7,8 However, the separation mode of the stationary phase is typically not switched.
‘Stimuli-responsive’ or ‘smart’ materials have properties that undergo reversible changes in response to external stimuli, such as temperature, pH, and light. Applying these stimuli to a stationary phase, stimuli-responsive chromatographies have been developed.9–13 The surface properties of a stimuli-responsive stationary phase can be adjusted by tuning these external stimuli. In the case of temperature-, and light-responsive chromatographies, the ‘response’ described above does not indicate a complete change in separation mode but rather a gradual change in polarity.9,10 Therefore, the order of retention does not change with the stimulus, but the retention capacity does. In the case of pH-responsive chromatographies, some studies with zwitterionic compound have achieved surface charge switching by pH change.11 However, pH changes did not result in drastic changes of retention. In contrast, when pH-responsive polymers were used for surface modification on stationary phase, the charge density was high and a drastic change in retention time was observed.12 To the best of our knowledge, a stationary phase in which the separation mode drastically switches depending on the stimuli–response has not been reported.
Hence, we developed a mobile phase pH-sensitive cation/anion-exchange mode switching chromatography system by utilizing a pH-responsive mixed-charge polymer for the stationary phase surface. Mixed-charge polymers include cationic and anionic units in the polymer chain.14 Using carboxylic acid as the anionic monomer enables the charge properties of mixed-charge polymers to be tuned by the pH.15–17 In addition, the charge balance of mixed-charge polymers can be regulated by changing the ratio of cationic and anionic monomers.18,19 Because of these properties, pH-switchable non-fouling/fouling surfaces modified with pH-responsive mixed charge polymers have been developed.14
In this study, a pH-responsive mixed charge polymer with more carboxylic acid monomers than cationic monomers was surface modified on the packing materials for HPLC. The higher amount of anionic monomers resulted in a net-negative charge at a pH higher than the pKa of the carboxylic acid moiety, resulting in a cation-exchange mode. In contrast, the pH-responsive mixed charge polymer switches to a net-positive charge at a pH lower than the pKa, resulting in an anion-exchange mode. Compared to temperature- and light-responsive chromatography systems, pH switching is considered simpler in a conventional chromatography system. Light-irradiation systems are not available on conventional HPLC systems and can only be used on capillary columns,9 as light cannot penetrate stainless-steel columns. Furthermore, temperature switching is possible with a conventional column oven; however, the temperature does not change rapidly.20 In contrast, the pH can easily be switched using the gradient function of conventional HPLC, making it a highly versatile switching method.
Experimental
Reagents
All chemical reagents were purchased from Tokyo Chemical Industries (Tokyo, Japan), Sigma-Aldrich (St. Louis, MO, USA), Fujifilm Wako Pure Chemical (Osaka, Japan), Peptide Institute (Osaka, Japan), and Nakalai Tesque (Kyoto, Japan) and used without further purification. CHEMCOSORB-NH2 (aminopropyl silica beads: particle diameter 3 μm, pore size 12 nm, surface area 200 m2 g−1) (APS) were purchased from Chemco Plus Scientific (Osaka, Japan). Ultrapure water (>18 MΩ cm) was obtained from a PURELAB flex water purification system (ELGA, Veolia Water, Marlow, U.K.) and used for the preparation of all aqueous solutions. Citric acid buffer was prepared from citric acid/trisodium citrate. Mono-2-(methacryloyloxy)ethyl cyclohexane-1,2-dicarboxylate (MTCHex) was synthesized according to previous reported work.16
General procedure for synthesis of pH-responsive mixed-charge hydrogel-modified silica beads
The 4,4′-azobis(4-cyanovaleric acid) (V-501) modified silica (V501S) beads were prepared according to previously reported work.21 The corresponding anionic monomer, 2-ethoxyethyl methacrylate (EEMA), [2-(methacryloyloxy)ethyl]trimethylammonium chloride (ca. 80% in water) (MTAC), ethylene glycol dimethacrylate (EGDMA), and 1000 mg V501S beads were added in 50 mL methanol using a 200 mL round-bottom flask. The reaction mixture was degassed by bubbling N2 for 30 min before reaction. The reaction was carried out for 5 h at 70 °C. The hydrogel-modified silica beads were filtered, rinsed in methanol (200 mL), and dried in vacuo.
ACSuc
MTAC (188 mg, 0.91 mmol), mono-2-(methacryloyloxy)ethyl succinate (MTSuc) (334 mg, 1.45 mmol), and EGDMA (8.68 mg, 0.04 mmol) were used as reaction mixture.
ACSucEE
MTAC (94 mg, 0.45 mmol), MTSuc (167 mg, 0.73 mmol), EEMA (186 mg, 1.18 mmol), and EGDMA (8.68 mg, 0.04 mmol) were used as reaction mixture.
ACCHexEE
MTAC (94 mg, 0.45 mmol), MTCHex (206 mg, 0.73 mmol), EEMA (186 mg, 1.18 mmol), EGDMA (8.68 mg, 0.04 mmol) were used as reaction mixture.
Characterization of pH-responsive mixed-charge hydrogel-modified silica beads
The prepared beads were characterized using a CHN elemental analyzer (UNICUBE; Elementar Analysensysteme GmbH, Langenselbold, Germany). The amount of immobilized initiator and hydrogel on the beads was estimated by the following equation. |  | (1) |
|  | (2) |
where %CA, %CI and %Cp are the carbon percentages obtained by elemental analysis of APS, V501S and hydrogel grafted silica beads, respectively; %CI(calcd) and %Cp(calcd) are the calculated weight carbon percentages in the initiator V-501 molecules and the pH-responsive mixed-charge hydrogel; and S is the surface area of the aminopropyl silica beads (200 m2 g−1). Zeta potentials of the prepared beads dispersed into each pH buffer (5 mM citric acid buffer) were measured using a zeta potential analyzer (ELS-8000, Otsuka Electronics Co., Ltd., Osaka, Japan).
Column packing
Synthesized pH-responsive mixed-charge hydrogel-modified beads were packed into a stainless-steel column (I.D. 2.1 mm × 100 mm). Prepared hydrogel-modified silica beads were dispersed in methanol/chloroform (50/50, v/v). The slurry was filled into a column packer connected to the column. Packing was performed by a HPLC pump (LC-20AR, SHIMADZU, Kyoto, Japan) under flow of methanol for 60 min, followed by methanol/water (50/50, v/v) for 30 min with a constant pressure of 45 MPa.
Chromatographic analyses
All chromatographic analyses were conducted on a HPLC system (Prominence, CMB-20A system controller, DGU-20A3R degasser, LC-20AR pump, SIL-20ACHT autosampler, SPD-M20A diode array detector, and CTO-20AC column oven; Shimadzu, Kyoto, Japan). UV detection was performed at 254 nm. Retention factors were calculated using the following equation and the data from the chromatograms.
Retention factor: k = (tR − t0)/t0, where tR and t0 are the retention times of the target analyte and solvent, respectively.
Results and discussion
Characterization of pH-responsive mixed-charge hydrogel-modified silica beads
A schematic illustration of the pH-responsive mixed-charge hydrogel-modified silica beads is shown in Fig. 1. To investigate the effect of the structure of the pH-responsive anionic moiety and the presence or absence of the neutral monomer, EEMA, on the column performance, three types of hydrogel-modified silica beads were synthesized with different monomer compositions. The composition ratio of the cationic to carboxylic acid monomers was set at 1
:
2 so that the cation-exchange mode occurs above the pKa and the anion-exchange mode below the pKa. Columns with zwitterionic phases with cationic and anionic groups present in a 1
:
1 molar ratio have been developed. HILIC columns such as ZIC-HILIC (silica gel support) and ZIC-pHILIC (polymer support), as well as Obelisc and iHILIC fusion as mixed-mode column are commercially available.22,23 These columns are always net-neutral regardless of the pH of mobile phase, whereas pH-responsive mixed-charge hydrogel-modified silica beads are designed to allow switching separation modes by significantly changing the net-charge of the surface depending on pH. A CHN elemental analysis was conducted, as previously reported.21 The amounts of the immobilized initiator and hydrogel on the beads were estimated and are summarized in Table S1.† V501S exhibited higher carbon and nitrogen contents than APS. The hydrogel-modified silica beads exhibited a higher carbon composition in comparison to V501S, indicating that the surface modification was successful in introducing the pH-responsive mixed-charge hydrogel onto the silica beads.
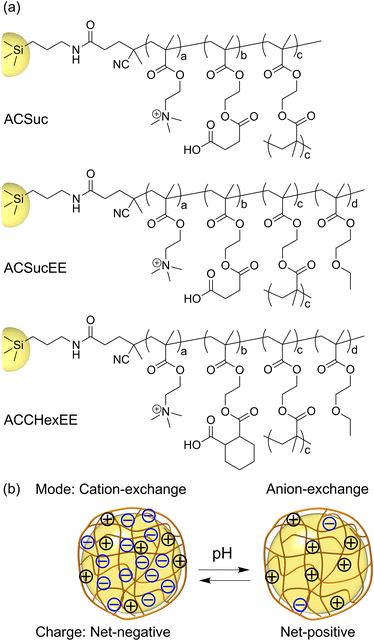 |
| Fig. 1 (a) Molecular structures of pH-responsive mixed-charge hydrogel-modified silica beads. (b) Schematic illustration of pH-dependent equilibrium state between mixed-charge and positive charge on the surface of silica beads modified with a pH-responsive mixed-charge hydrogel. | |
The pH-switchable net-negative/positive charge of the pH-responsive mixed-charge hydrogel modified silica beads was investigated using pH-dependent zeta potential measurements (Fig. 2). The zeta potentials of APS and V501S were 0 mV and became slightly positive with decreasing pH. In contrast, the zeta potential of the pH-responsive mixed-charge hydrogel-modified silica beads were net-negative at pH 7, increasing with decreasing pH, and then switching to net-positive. When the pH was sufficiently higher than the pKa of the polymers, the higher proportion of carboxylic acid than the cationic moiety resulted in a net-negative charge and changed to a net-positive charge with protonation of the carboxylate groups with decreasing pH. The pH-switching between the net-negative and net-positive charges on the surface of the silica beads was successfully achieved by tuning the anionic monomers and composition ratio.
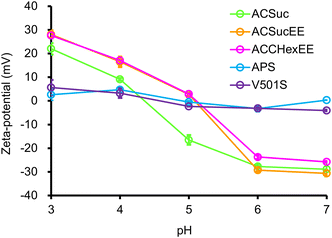 |
| Fig. 2 pH-dependent zeta potentials of silica beads (0.5 mg mL−1) for each pH in 5 mM citric acid buffers. | |
Chromatographic behaviour with neutral compounds
Before evaluating the pH-dependent cation- and anion-exchange mode switching, the basic properties of the ACSuc column composed of only cationic and anionic monomers without hydrophobic monomer was evaluated using naphthalene, and alkylbenzenes (C1–C5), as neutral compounds. First, the reproducibility of the modification of the pH-responsive mixed-charge hydrogel on the silica beads was evaluated using 5 columns packed with independently modified silica beads. The relative standard deviations (RSDs) of retention time and number of theoretical plates for naphthalene were 5.3 and 17.8%, respectively (Fig. S1 in ESI†). These results indicate that the modification method of the pH-responsive mixed-charge hydrogel is reproducible. The durability performance of a column is an important factor for practical use. Fig. S2 (ESI†) shows the chromatograms of naphthalene before and after using 5000 column volumes (CVs). Retention time and number of theoretical plates after using 5000 CVs were 96.5 and 90.5%, compared with those before using 5000 CVs, indicating that the ACSuc column is sufficiently stable for practical use. Next, the hydrophobic selectivity was evaluated by the retention behaviour of alkylbenzenes. The retention factors of alkylbenzenes decreased with increasing methanol ratio and decreased alkyl chain length (Fig. S3 in ESI†). This behaviour corresponds to reversed-phase separation mode, but retention is so weak that complete separation was achieved within 25 min with 10% methanol mobile phase as shown in Fig. 3. The hydrophobicity of the main chain of polymethacrylate may have affected the reversed-phase retention behaviour, but the positive and negative charge in the side chains may have contributed to weak retention behaviour.
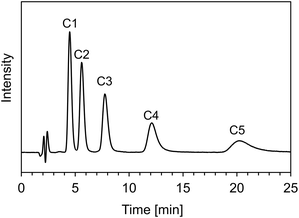 |
| Fig. 3 Chromatogram of alkyl benzenes (C1: toluene; C2: ethylbenzene; C3: propylbenzene; C4: butylbenzene; C5: pentylbenzene) obtained with an ACSuc column. Analytical conditions: mobile phase: 100 mM citric acid buffer (pH 7)/methanol (90/10, v/v); column temperature: 40 °C; flow rate: 0.2 mL min−1; analyte concentration: 0.5 mg mL−1; injection volume: 5 μL. | |
Investigation of pH-dependent cation- and anion-exchange mode switching chromatography
Dopamine (pKa = 8.9) and benzenesulfonic acid (pKa = −2.8) were employed to evaluate the retention by the cation- and anion-exchange modes, respectively. They can be considered to be positively and negatively charged, respectively, in the pH 3–7 buffer used as a mobile phase. The chromatograms of dopamine and benzenesulfonic acid with each pH-responsive mixed-charge column (ACSuc, ACSucEE, and ACCHexEE), using a buffer solution (pH 3–7) as the mobile phase, are shown in Fig. S4 (ESI†). The pH-dependent retention factors from the retention times of each peak in Fig. S4 (ESI†) are shown in Fig. 4. The retention factors of dopamine increased with increasing pH, whereas the retention factors of benzenesulfonic acid increased with decreasing pH. These results indicated that the retention in the anion-exchange mode was greater at higher pH, whereas the retention in the cation-exchange mode was greater at lower pH. It is clear from the pH-dependent zeta potential results (Fig. 2) that the switching of the surface charge of the mixed-charge polymer-modified silica beads caused the switching of the ion-exchange mode. There were good linearities between log k and pH for both dopamine and benzenesulfonic acid. Log
k of the compounds having single acid/base-dissociative group versus pH can be approximated by a linear-solvent-strength (LSS) relation.24 The linearities of them were considered to be obtained because dopamine and benzenesulfonic acid had a constant charge and the stationary phase changed the degree of dissociation of the carboxylic acid moieties depending on pH. The ion-exchange mode switches at the cross-point of the two straight lines of dopamine and benzenesulfonic acid. That is, the surface charge of the pH-responsive mixed-charge polymer is zero, and the pH is defined as pKCs (Cs: charge switching). The pKCs of ACSuc, ACSucEE, and ACCHexEE were 5.0, 5.3, and 5.7, respectively (Fig. 4). The pKCs increased with the introduction of the spacer by changing the pH-responsive anionic moiety from MTSuc to MTCHex (increasing hydrophobicity), correlating with the results of our previous study.16 The cation/anion mode switching pH can be tuned by changing the monomer composition.
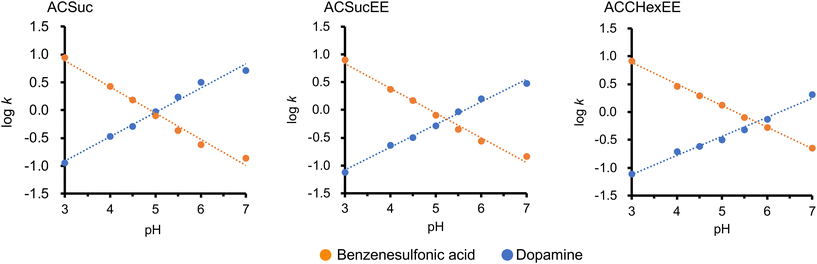 |
| Fig. 4 pH-dependent retention factors of benzenesulfonic acid and dopamine on ACSuc, ACSucEE, and ACCHexEE columns, extracted from the retention times of the chromatograms in Fig. S4 (ESI†). | |
Separation of acidic/basic pharmaceuticals with cation-/anion-exchange mode
Tricyclic antidepressants are widely employed to treat psychiatric disorders, such as depression and anxiety disorder.25 Herein, as a proof-of-concept experiment, an ACCHexEE (pKCs = 5.7) column was employed for the separation of basic tricyclic antidepressants, utilizing the cation-exchange mode. To confirm the retention mode of ACCHexEE at pH 7, the retention factors of five antidepressants were investigated by varying the methanol contents and ionic concentrations of the buffer solution. The chromatograms and retention factors at each condition are shown in Fig. 5, S5, and S6 (ESI†). The retention factors of the antidepressants decreased with increasing methanol content, indicating that the ACCHexEE column at pH 7 functions as a reversed-phase mode system based on hydrophobic interactions on the polymer backbones. However, the elution order differs from the reversed-phase mode columns.26–28 In addition, the retention factors of the antidepressants decreased with increasing ionic concentration of the buffer solution. The ACCHexEE column at pH 7 functions as a cation-exchange mode system based on ionic interactions between the positive charge of the antidepressants and the net-negative charge of the polymer. On investigating the five antidepressants, the retention at pH 5 (<pKCs of ACCHexEE) was found to be shorter than at pH 7 (Fig. S7, Table S2 in the ESI†). This is due to the net-positive charge of the ACCHexEE column at pH 5, preventing electrostatic interactions. These results indicated that the ACCHexEE column at pH 7 functioned as a mixed mode system with a cation-exchange and reversed-phase mode.
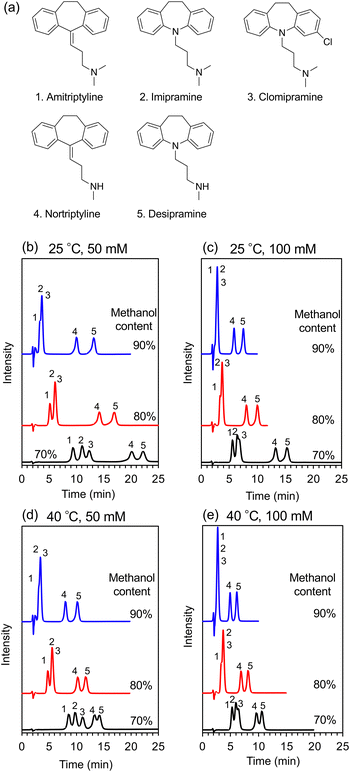 |
| Fig. 5 (a) Chemical structures of antidepressants. Chromatograms of mixed samples of five antidepressants on an ACCHexEE column at each ionic strength of mobile phase and temperature. Analytical parameters: flow rate: 0.2 mL min−1; concentration: 0.1 mg mL−1; injection: 10 μL; (b) mobile phase: 50 mM citrate buffer (pH 7)/methanol, column temperature: 25 °C; (c) 100 mM citrate buffer (pH 7)/methanol, column temperature: 25 °C; (d) mobile phase: 50 mM citrate buffer (pH 7)/methanol, column temperature: 40 °C; (e) 100 mM citrate buffer (pH 7)/methanol, column temperature: 40 °C. | |
We also applied the anion-exchange mode of pH-responsive mixed-charge columns to separate five types of non-steroidal anti-inflammatory drugs (NSAIDs). NSAIDs are a general term for drugs with anti-inflammatory, analgesic, and antipyretic effects, which are used to treat inflammatory conditions.29 Among these, the most frequently used are the acidic NSAIDs. The chromatograms of NSAIDs separated with a ACCHexEE column using 100 mM citrate buffer (pH 5)/methanol is shown in Fig. 6. As discussed above, ACCHexEE works as reversed-phase mode and an anion-exchange mode at pH 5, and these interactions enabled the separation of the acidic NSAIDs.
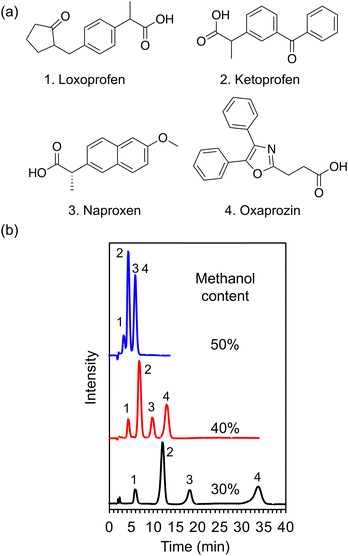 |
| Fig. 6 (a) Chemical structures of NSAIDs. (b) Chromatograms of mixed sample of four NSAIDs on ACCHexEE column. Analytical condition: mobile phase: 50 mM citrate buffer (pH 5)/methanol; column temperature: 25 °C; flow rate: 0.2 mL min−1; concentration: 0.1 mg mL−1, 10 μL injection. | |
Conclusions
Three types of pH-responsive mixed-charge polymers modified on silica beads were successfully utilized for cation/anion-exchange mode switching chromatography. The pH-switchable net-negative and net-positive charges of the stationary phase surface were confirmed by pH-dependent zeta potential measurements. The retention factors of dopamine as a cationic compound and benzenesulfonic acid as an anionic compound changed inversely with pH, indicating that the cation/anion mode can be switched with pH. In a proof-of-concept experiment, five types of tricyclic antidepressants and four types of NSAIDs were successfully separated using a cation/anion-exchange mode switching column. To the best of our knowledge, this study is the first example of drastic and complete switching of separation modes in a single column. Because the cation/anion-exchange mode can be reversed by pH-switching, it is expected to be applied to separating biomolecules, such as proteins, peptides, and nucleic acids.
Author contributions
Taisei Kaku: methodology, investigation, writing – original draft. Koichi Deura: investigation. Tomoka Yoshii: investigation. Daniel Citterio: writing – review & editing, supervision. Yuki Hiruta: conceptualization, methodology, writing – original draft, supervision, project administration.
Conflicts of interest
There are no conflicts to declare.
Acknowledgements
The authors thank Mr. Daiki Nomoto of Keio University for his support with HPLC analysis.
References
- V. D'Atri, S. Fekete, A. Clarke, J.-L. Veuthey and D. Guillarme, Anal. Chem., 2019, 91, 210–239 CrossRef PubMed.
- F. Cacciola, F. Rigano, P. Dugo and L. Mondello, TrAC, Trends Anal. Chem., 2020, 127, 115894 CrossRef CAS.
- O. Núñez, H. Gallart-Ayala, C. P. B. Martins and P. Lucci, J. Chromatogr. A, 2012, 1228, 298–323 CrossRef PubMed.
- P. Žuvela, M. Skoczylas, J. J. Liu, T. Bączek, R. Kaliszan, M. W. Wong and B. Buszewski, Chem. Rev., 2019, 119, 3674–3729 CrossRef PubMed.
- A. Nordborg and E. F. Hilder, Anal. Bioanal. Chem., 2009, 394, 71–84 CrossRef CAS.
- B. Buszewski and S. Noga, Anal. Bioanal. Chem., 2012, 402, 231–247 CrossRef CAS PubMed.
- L. Zhang, Q. Dai, X. Qiao, C. Yu, X. Qin and H. Yan, TrAC, Trends Anal. Chem., 2016, 82, 143–163 CrossRef CAS.
- D. Sýkora, P. Řezanka, K. Záruba and V. Král, J. Sep. Sci., 2019, 42, 89–129 CrossRef.
- Z. An, J.-M. Zhang, M.-Y. Lv, X.-Q. Li, L. Wu, H.-B. Shang and D. Li, Anal. Chem., 2021, 93, 17051–17059 CrossRef CAS.
- Y. Hiruta, Polym. J., 2022, 54, 1419–1430 CrossRef CAS.
- S. Bäurer, S. Polnick, O. L. Sánchez-Muñoz, M. Kramer and M. Lämmerhofer, J. Chromatogr. A, 2018, 1560, 45–54 CrossRef.
- Q. Ma, M. Chen, H.-R. Yin, Z.-G. Shi and Y.-Q. Feng, J. Chromatogr. A, 2008, 1212, 61–67 CrossRef CAS.
- J.-B. Qu, Y.-L. Xu, J.-Y. Liu, J.-B. Zeng, Y.-L. Chen, W.-Q. Zhou and J.-G. Liu, J. Chromatogr. A, 2016, 1441, 60–67 CrossRef CAS.
- S. Jiang and Z. Cao, Adv. Mater., 2010, 22, 920–932 CrossRef CAS.
- L. Mi, M. T. Bernards, G. Cheng, Q. Yu and S. Jiang, Biomaterials, 2010, 31, 2919–2925 CrossRef CAS.
- Y. Hiruta, K. Sawada, Y. Mizui and D. Citterio, Bull. Chem. Soc. Jpn., 2020, 93, 547–552 CrossRef CAS.
- Y. Nakamura, M. Nasu, Y. Shindo, K. Oka, D. Citterio and Y. Hiruta, Polym. J., 2023, 55, 1179–1188 CrossRef CAS.
- F.-I. Tai, O. Sterner, O. Andersson, T. Ekblad and T. Ederth, Soft Matter, 2014, 10, 5955–5964 RSC.
- Y.-J. Shih, Y. Chang, D. Quemener, H.-S. Yang, J.-F. Jhong, F.-M. Ho, A. Higuchi and Y. Chang, Langmuir, 2014, 30, 6489–6496 CrossRef CAS PubMed.
- D. Nomoto, K. Nagase, Y. Nakamura, H. Kanazawa, D. Citterio and Y. Hiruta, Colloids Surf., B, 2021, 205, 111890 CrossRef CAS PubMed.
- K. Okubo, K. Ikeda, A. Oaku, Y. Hiruta, K. Nagase and H. Kanazawa, J. Chromatogr. A, 2018, 1568, 38–48 CrossRef CAS.
- P. Jandera, Anal. Chim. Acta, 2011, 692, 1–25 CrossRef CAS PubMed.
- C. Vosse, C. Wienken, C. Cadenas and H. Hayen, J. Chromatogr. A, 2018, 1565, 105–113 CrossRef CAS.
- R. Kaliszan, P. Haber, T. Bączek, D. Siluk and K. Valko, J. Chromatogr. A, 2002, 965, 117–127 CrossRef CAS PubMed.
- M. Safari, M. Shahlaei, Y. Yamini, M. Shakorian and E. Arkan, Anal. Chim. Acta, 2018, 1034, 204–213 CrossRef CAS.
- J. Layne, J. Chromatogr. A, 2002, 957, 149–164 CrossRef CAS PubMed.
- C. Alves, C. Fernandes, A. J. dos Santos Neto, J. C. Rodrigues, M. E. C. Queiroz and F. M. Lanças, J. Chromatogr. Sci., 2006, 44, 340–346 CAS.
- K. Kaizu, M. Mochida, H. Imai, D. Citterio and Y. Hiruta, J. Chromatogr. A, 2022, 1677, 463294 CrossRef CAS PubMed.
- P. Gallo, S. Fabbrocino, G. Dowling, M. Salini, M. Fiori, G. Perretta and L. Serpe, J. Chromatogr. A, 2010, 1217, 2832–2839 CrossRef CAS.
|
This journal is © The Royal Society of Chemistry 2024 |
Click here to see how this site uses Cookies. View our privacy policy here.