DOI:
10.1039/D3MA00446E
(Paper)
Mater. Adv., 2024,
5, 525-538
Ferroelectric nematogens containing a methylthio group†
Received
21st July 2023
, Accepted 14th October 2023
First published on 16th October 2023
Abstract
The synthesis and characterisation of eight nematogens containing a terminal methylthio group is reported. The compounds are based on RM734 and differ in the number and position of fluorine substituents, and in the position of the lateral methoxy substituent. Seven of these compounds exhibit a monotropic ferroelectric nematic phase, NF, including examples of NF-isotropic phase transitions. Two of the compounds show the anti-ferroelectric NX phase. Their transitional behaviour is discussed in terms of molecular shape and changes in electronic properties. Their phase behaviour is similar to that of the corresponding compounds containing a terminal methoxy group, but the methoxy terminated materials consistently show the higher transition temperatures. This is accounted for by the larger reduction in molecular shape anisotropy associated with the methylthio group, and the associated change in the electronic properties. The NX phase seen for the methylthio substituted compounds are thought to reflect the suppression of the NF phase by the methylthio group rather than any specific stabilising effect. Specific interactions between methylthio groups thought to stabilise nematic behaviour do not appear to stabilise the NF phase.
Introduction
The ferroelectric nematic phase, NF, was experimentally discovered in 2017,1–3 and is now the hottest topic in liquid crystal research. In the conventional nematic phase, N, the rod-like molecules are more or less aligned along a common direction, the director, whereas their centres of mass are distributed randomly. The director is described by a unit vector, n, which possesses inversion symmetry such that n = −n, and the phase is apolar, Fig. 1. In the NF phase, the director no longer possesses inversion symmetry i.e.n ≠ −n, and the phase is polar, Fig. 1.4 This phase has generated very significant excitement not only given the fundamental importance of a fluid ferroelectric phase, but also because it has huge application potential in a range of electrooptic technologies. A small number of materials exhibit a further liquid crystal phase referred to as the NX or SmZA phase.2,5–8 The NX phase is thought to consist of regions of polar nematic phase arranged in a regular antiferroelectric structure.5,7,9
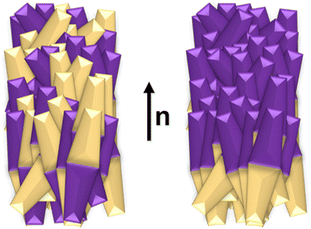 |
| Fig. 1 Schematic representations of the conventional nematic, N, phase (left) and ferroelectric nematic, NF, phase (right). | |
To date there have been around 150 molecules reported to show the NF phase and bar a handful of exemptions such as polymers,10,11 these materials may be described as conventional low molar mass mesogens.3,6,8,12–25 The empirical structure–property relationships describing the links between the formation of the NF phase and molecular structure have yet to be established let alone understood. The archetypal ferroelectric nematic compounds RM7343 and DIO2 have large longitudinal dipole moments and possess lateral bulk in the form of either a methoxy group or fluorine substituents. It has been suggested that these structural factors are prerequisites for the observation of the NF phase. Indeed, this view is supported by computer simulations of tapered Gay–Berne particles possessing longitudinal dipole moments that revealed NF behaviour.26 Exceptions have been reported to these generalisations (see, for example, ref. 18,24,27), and in order to design new ferroelectric nematogens having tailored properties in a rational manner we must first explore and understand the relationships between structure and properties for the NF phase.
Sulfur has been used extensively in the design of liquid crystals including, for example, in terminal alkylthio chains,28–34 alkylthio spacers,35–40 thiophene moieties,41–44 thiocyanate terminal groups,45,46 and thioester linking groups.47,48 One driving force for this research is the enhanced values of optical birefringence exhibited by compounds that contain sulfur.45,49–52 Highly birefringent nematogens have considerable application potential not only in liquid crystal display technologies, but also have an important role to play in emerging technologies such as liquid crystal lenses53,54 and as holographic materials.55 Surprisingly, sulfur has very largely been overlooked in the design of ferroelectric nematogens, and to address this surprising omission, here we report the synthesis and characterisation of eight new compounds, S1–8, designed to exhibit the NF phase and that contain a methylthio substituent, Table 1. These are based on the archetypal ferroelectric nematogen RM734.3 The properties of these compounds are compared with those of the corresponding materials in which the methylthio group has been replaced by a methoxy unit, O1–8, Table 1. We note that O1 is RM734. Critically, this is the first time that specifically the effect of replacing the terminal methoxy group with a terminal methylthio group on the formation of the NF phase has been studied, while also sequentially changing the degree of fluorination incorporated into the molecular backbone.
Table 1 Molecular structures of the Sn and On compounds
Name |
Structure |
Name |
Structure |
S1
|
|
O1
|
|
S2
|
|
O2
|
|
S3
|
|
O3
|
|
S4
|
|
O4
|
|
S5
|
|
O5
|
|
S6
|
|
O6
|
|
S7
|
|
O7
|
|
S8
|
|
O8
|
|
Experimental
Synthesis
The synthetic route used to prepare S1–8 is shown in Scheme 1 and for O5 and O6 in Scheme 2. A detailed description of the preparation of these compounds, including the structural characterisation data for all intermediates and final products, is provided in the Supplementary Information.
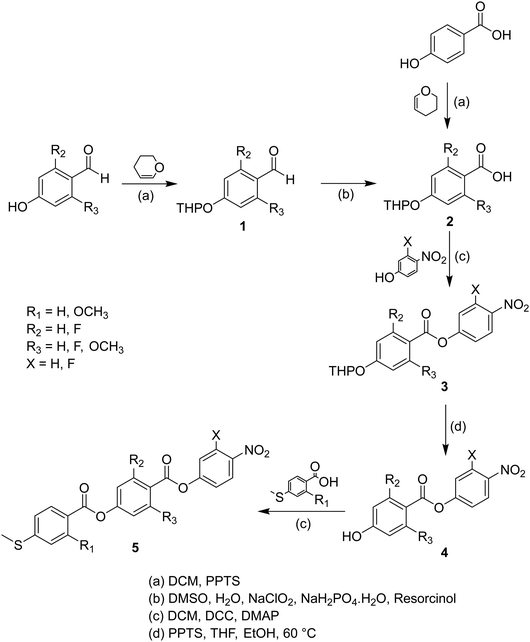 |
| Scheme 1 Synthetic route used to obtain the Sn compounds. | |
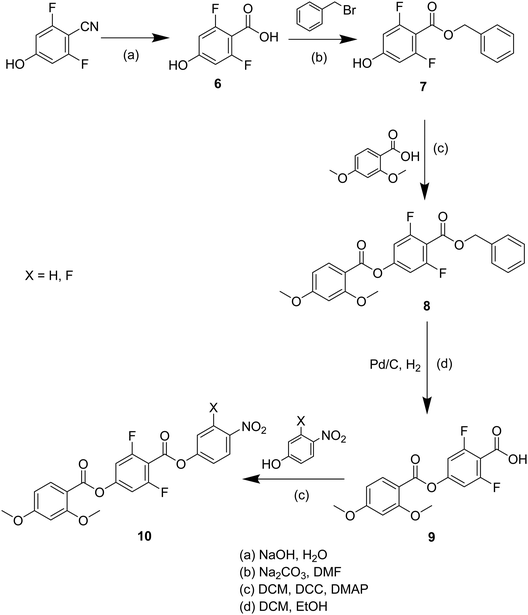 |
| Scheme 2 Synthetic route used to obtain compounds O5 and O6. | |
Optical studies
Phase characterisation was performed by polarised light microscopy, using an Olympus BH2 polarising light microscope equipped with a Linkam TMS 92 hot stage. Slides treated for planar alignment were purchased from INSTEC or AWAT with a thickness between 2.9–3.5 μm or 1.8 μm, respectively and both sets of cells were ITO conducting.
Differential scanning calorimetry
The phase behaviour of the materials was studied by differential scanning calorimetry performed using a Mettler Toledo DSC1 or DSC3 differential scanning calorimeter equipped with TSO 801RO sample robots and calibrated using indium and zinc standards. Heating and cooling rates were 10 K min−1, with a 3 min isotherm between either heating or cooling, and all samples were measured under a nitrogen atmosphere. Transition temperatures and associated enthalpy changes were extracted from the heating traces unless otherwise noted. For each sample, two aliquots were measured, and the data listed are the average of the two sets of data.
Molecular modelling
The geometric parameters of the reported compounds were obtained using quantum mechanical DFT calculations with Gaussian09 software.56 Optimisation of the methylthio-containing structures (S1–8) was carried out at the B3LYP/6-311G(d,p) level of theory. Comparison of the results of optimisation of the corresponding ether-linked (O1–8) materials at the B3LYP/6-311G(d,p) and the 6-31G(d) levels showed no discernible difference in the geometries found, and so their optimisation was carried out at the B3LYP/6-31G(d) level. Visualisations of the electronic surfaces were generated from the optimised geometries using the GaussView 5 software, and visualisations of the space-filling models were produced post-optimisation using the QuteMol package.57
Birefringence measurements
Birefringence was measured with a setup based on a photoelastic modulator (PEM-90, Hinds) working at a modulation frequency f = 50 kHz; as a light source, a halogen lamp (Hamamatsu LC8) was used equipped with narrow bandpass filters (633 nm and 690 nm). The signal from a photodiode (FLC Electronics PIN-20) was deconvoluted with a lock-in amplifier (EG&G 7265) into 1f and 2f components to yield a retardation induced by the sample. Knowing the sample thickness, the retardation was recalculated into optical birefringence. Samples were prepared in 4.9-micron-thick cells with planar anchoring. The alignment quality was checked prior to measurement by inspection under the polarised light optical microscope.
Spontaneous electric polarization measurements
Values of the spontaneous electric polarization were obtained from the current peaks recorded during Ps switching upon applying a triangular-wave voltage at a frequency of 2 Hz. The 9.7 μm-thick cells with ITO electrodes and no polymer aligning layers were used and the switching current was determined by recording the voltage drop at the resistivity of 50 kΩ in serial connection with the sample. The current peak was integrated over time to calculate the surface electric charge and evaluate the polarization value.
Dielectric spectroscopy
The complex dielectric permittivity, ε*, was studied using a Solatron 1260 impedance analyser. Measurements were conducted in the 1 Hz–1 MHz frequency (f) range, with the probe voltage of 20 mV, and it was checked by optical observations that such a voltage is below the Fredericks transition threshold. The material was placed in 9.7 μm-thick glass cells with ITO electrodes and no polymer aligning layers. Lack of a surfactant layer resulted in the random configuration of the director in the LC phases; microscopic observations of optical textures suggested a dominant planar orientation without preferable direction of the long molecular axis. The relaxation frequency, fr, and dielectric strength of the mode, Δε, were evaluated by fitting the complex dielectric permittivity to the Cole–Cole formula:
where ε∞ is the high frequency dielectric constant, α is the distribution parameter of the mode and δ is the low frequency conductivity, respectively.
X-ray diffraction
The X-ray diffraction (XRD) measurements were performed with a Bruker D8 GADDS system (CuKα line, Goebel mirror, point beam collimator, Vantec2000 area detector). The temperature of the sample was controlled with precision of ±0.1 K. Samples were prepared as droplets on a heated surface.
Results and discussion
The transitional properties of the methylthio-containing Sn materials are listed in Table 2. The conventional nematic phase, N, was assigned based on the observation of a characteristic uniform texture in a cell treated for planar alignment, Fig. 2(a), or on the observation of a characteristic schlieren texture containing two- and four-brush point defects when the sample was sandwiched between two untreated glass slides, Fig. 3(a). These textures flashed when subjected to mechanical stress. For S1 and S7 on cooling the uniform texture of the nematic phase, a chevron pattern was observed at the transition to the NX phase, Fig. 2(b). In XRD studies (these will be discussed in more detail later) the NX phase shows only short-range correlations of the molecular positions which are characteristic for nematic phases and hence why the phase is named as such. However, in previous diffraction experiments performed at synchrotron facilities very weak Bragg-type diffraction signals were detected in the NX phase,9 revealing a kind of long-range periodicity. This periodicity was attributed to existence of regular domains with antiparallel orientation of the polarization, and so this phase is referred to by other groups as the antiferroelectric SmZA phase.5–7 Cooling S1 from the NX phase saw a series of twisted states emerge a few degrees below the transition to the NF phase, Fig. 2(c)–(e), and we have previously reported observing these textures for the NF phase.8 There was also a clear change in birefringence which is also considered characteristic of the NF phase. In S4 and S6, the NF phase formed directly from the isotropic phase. In thicker cells treated for planar alignment, spherical droplets developed at the NF-I transition and coalesced to form banded textures which are also characteristic of the NF phase, Fig. 3(b) and (c).10,13,18,20,25 This banded texture is observed due to the domains which form within the cell separated by distinct domain boundaries. These domains show regions in which the director has different orientations and so the polarisation direction is similarly changed. The banding within the domains is due to areas of lower and higher birefringence, with the domains themselves thought to form due to director splay deformations which are necessary to connect opposite polarisation vectors on the lower and upper cell surfaces.8 The scaled entropy changes, calculated using the enthalpy changes listed in Table 2, associated with the NF–I transitions of S4 and S6 are several times larger than those associated with the N–I transitions, Table 2. This presumably reflects the additional entropic contribution associated with the ordering of the dipoles in the NF phase compared to the conventional N phase. The values of the enthalpy changes listed in Table 2 are similar to those reported for other ferroelectric nematogens.8,12,18,20
Table 2 Transition temperatures for the Sn and On series (in °C) with their associated enthalpy changes (in parentheses, kJ mol−1)
Compound |
M.P. |
Phase sequence |
Values extracted from DSC cooling traces.
Measured using the polarised light microscope.
Combined ΔH value associated with both phase transitions due to peak overlap in the DSC trace.
Data extracted from Cruickshank et al.20
Data extracted from Mandle et al.3
Data extracted from Brown et al.8
Data extracted from Tufaha et al.18
|
S1
|
147 (38.6) |
I-169 (0.54)-N-a111 (c0.43)-NX-a106 (c0.43)-NF |
S2
|
147 (43.9) |
I-a135 (0.54)-N-a122 (1.29)-NF |
S3
|
162 (40.3) |
I-a152 (0.41)-N-a126 (0.57)-NF |
S4
|
150 (36.0) |
I-a129 (3.09)-NF |
S5
|
174 (47.8) |
I-a127 (0.40)-N-a121 (0.80)-NF |
S6
|
171 (52.9) |
I-a122 (3.56)-NF |
S7
|
164 (47.9) |
I-172 (1.22)-N-b97-NX |
S8
|
164 (40.3) |
I-a143 (0.74)-N-a124 (0.28)-NF |
O1
|
139 (34.3) |
I-188 (0.61)-N-131 (0.60)-NF |
O2
|
166 (50.4) |
I-155 (0.50)-N-140 (1.61)-NF |
O3
|
161 (54.1) |
I-165 (0.69)-N-143 (1.38)-NF |
O4
|
151 (53.2) |
I-139 (4.38)-NF |
O5
|
192 (50.6) |
I-a139 (0.34)-N-a132 (1.62)-NF |
O6
|
160 (45.4) |
I-a131 (4.33)-NF |
O7
|
192 (48.3) |
I-189 (0.81)-N-126 (0.11)-NF |
O8
|
180 (51.6) |
I-157 (10.4)-N-142 (1.17)-NF |
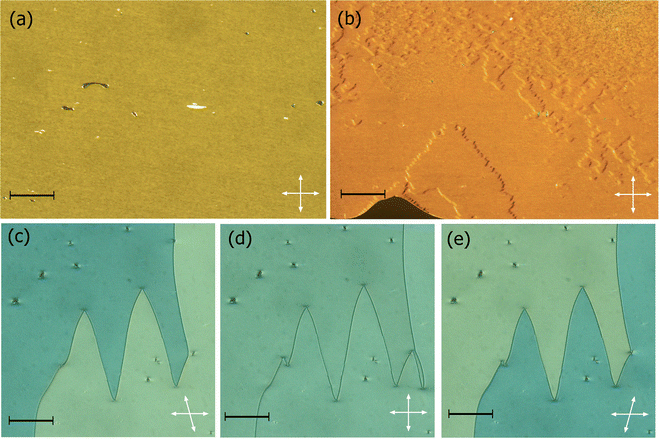 |
| Fig. 2 Polarised optical microscope textures observed for the compound, S1: (a) uniform texture of the N phase at 130 °C; (b) chevron texture of the NX phase at 110 °C; (c)–(e) twisted states of the NF phase at 100 °C, with (d) showing the texture when the polarisers are fully crossed. All textures were obtained in cells treated for planar alignment with the scale bars each representing 200 μm. | |
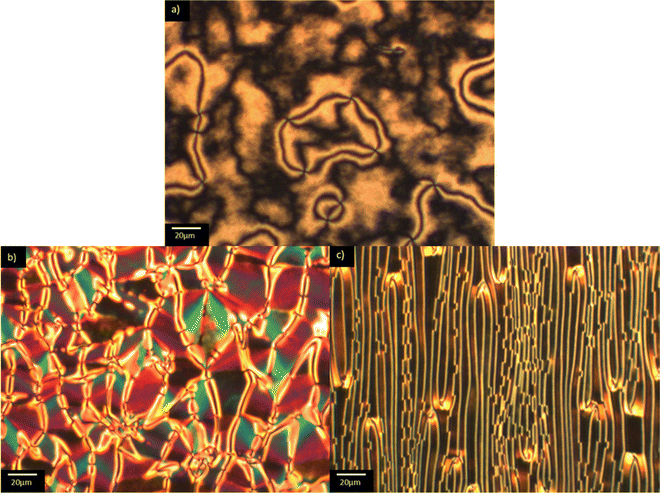 |
| Fig. 3 Polarised optical microscope textures observed for the Sn series: (a) schlieren texture of the N phase viewed between untreated glass slides for S2 at 130 °C; (b) banded texture of the NF phase viewed in a cell treated for planar alignment for S2 at 122 °C; and (c) banded texture of the NF phase viewed in a cell treated for planar alignment for S6 at 110 °C. | |
In order to confirm the phase assignments, dielectric measurements were undertaken using S1. In the N phase, a clear dielectric mode was found for which the relaxation frequency decreased and the strength increased on cooling, as the correlation length of polar order also increased. There was a clear increase in the value of ε upon entry to the NF phase, Fig. 4. The low frequency dielectric strength was over 10
000 and this is in agreement with values reported for other ferroelectric nematogens.2,8,10,12,14–16,18,58–60 However, it has been recently reported that there may be a relationship between these values and the cell thickness.61 The polar character of the NF phase was further confirmed by the polarisation switching behaviour. When an AC voltage was applied a single current peak per half cycle was registered and this corresponded to a large spontaneous polarisation of 3.1 μC cm−2, Fig. 5, but only around half the value reported for RM734.4 Although these measurements gave a clear indication of S1 exhibiting the ferroelectric nematic phase, they do not clearly reveal the NX phase between the N and NF phases that was seen using polarised light optical microscopy. The temperature dependence of the optical birefringence for S1 is shown in Fig. 6. The optical studies showed that when the sample is cooled from the isotropic to the nematic phase, there is a sharp increase in the birefringence at the transition that then follows a critical power-law dependence. At the transition to the NX phase there is a small change in the temperature dependence of birefringence showing that the phase is orientationally similar to the conventional nematic phase. This is followed by a small step-like increase at the transition to the NF phase, indicative of an increase of the order parameter, S. This observation is in agreement with the birefringence measurements that we have reported previously for the NX phase,8,9 also referred to as the smectic ZA phase.5,62 Based on both the textural observations and this change in birefringence, the NX phase in S1 may be assigned as being the same phase shown by DIO and some related compounds.2,6,21 X-ray diffraction was also used to confirm the assignment of the phases in compound S1 as nematics, Fig. 7. The X-ray diffraction patterns obtained for both the N and NX phases are essentially identical consisting of broad signals indicative of there being only short-range correlations of the molecular positions. Prior to the observation of the NF phase, however, the sample crystalised as shown by the observed diffraction pattern, Fig. 7 (c). The pattern seen for the NX phase, although not showing the long-range periodicity we have reported previously,9 does support its assignment as a nematic phase.
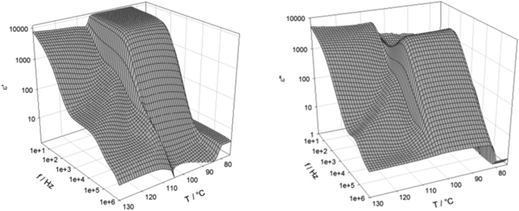 |
| Fig. 4 Real (left) and imaginary (right) parts of the complex dielectric permittivity measured as a function of temperature and frequency for S1, in a 9.7 μm-thick cell with ITO electrodes and no alignment layer. | |
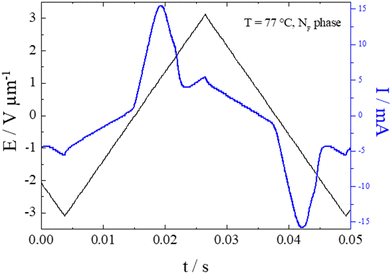 |
| Fig. 5 The switching current (blue line) associated with polarization reversal under applied triangular wave voltage (black line) for S1 at 77 °C. Measurements were performed in a 9.7 μm-thick cell with ITO electrodes and no alignment layer. | |
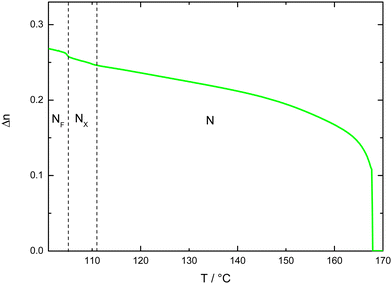 |
| Fig. 6 Optical birefringence of S1 measured with green light (λ = 532 nm) across the phase transitions with the light green line showing the birefringence measured on cooling. | |
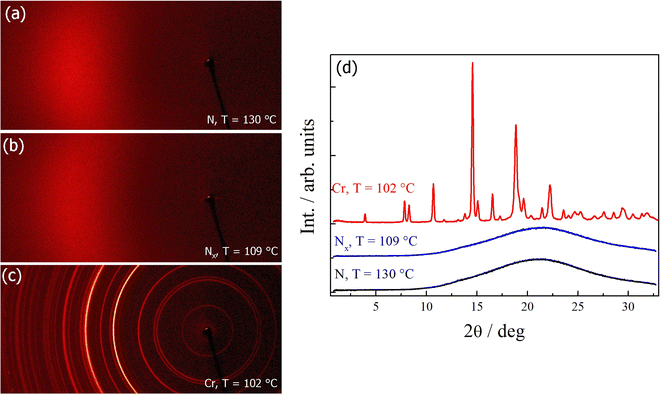 |
| Fig. 7 X-ray diffraction patterns of compound S1 in the (a) N phase; (b) NX phase and (c) crystalline solid phase. (d) Intensity profiles of the X-ray patterns of the N phase (black line), NX phase (blue line) and crystalline solid phase (red line). | |
We now turn our attention to the dependence of the transition temperatures on molecular structure for the methylthio-substituted compounds listed in Table 2. S1, the methylthio-substituted analogue of the extensively studied RM734, shows the phase sequence NF-NX-N-I, and forms the basis of comparison for compounds S2–6 in which one, two or three fluorine substituents are added to the structure. In S2 a fluorine substituent has been added ortho to the nitro group and this has reduced the nematic–isotropic transition temperature, TNI, by 34 K. This decrease is presumably associated with the reduction in shape anisotropy. Conversely, the ferroelectric nematic-nematic transition temperature, TNFN, of S2 is 16 K higher than that of the NF–NX transition temperature, TNFNX observed for S1. This increase in the stability of the NF phase may be attributed, in part, to an increase in the molecular dipole moment from 10.5 D for S1 to 11.6 D for S2. It has also been suggested,18 however, that this observation is consistent with the predictions of a molecular model developed by Madhusudana to describe the NF phase in which the molecules are described by a longitudinal surface charge density wave.63 Specifically, the model suggests that the parallel alignment of the molecules is enhanced by minimising the amplitude of the charge density wave at either end of the molecule. The addition of the fluorine atom ortho to the nitro group spreads the electron charge distribution reducing the amplitude of the charge density wave, and so predicted to increase the stability of the NF phase as observed.
In S3 a fluorine atom has been added to the central phenyl ring in S1 and this reduces TNI by 17 K. This is a much smaller reduction in TNI than seen between S1 and S2 of 34 K and reflects the different contributions to the molecular shape arising from substitutions in differing positions, Fig. 8. Again, the addition of a fluorine atom sees an increase in the stability of the NF phase and TNFN for S3 is some 20 K higher than TNFNX observed for S1. This is a larger difference than seen between the corresponding values of S2 and S1 although the dipole moment of S3 is 11.2 D and lies between those of S1 and S2. Indeed, TNFN for S3 is higher than that of S2 although we note that this is a small difference, just 4 K. This supports the view that the stability of the NF phase cannot be simply associated with the magnitude of the molecular dipole moment. Within Madhusudana's model, the addition of the fluorine atom increases the amplitude of the charge density on the central ring and in relative terms decreases that associated with the terminal rings. Such a change is predicted to increase the stability of the NF phase.
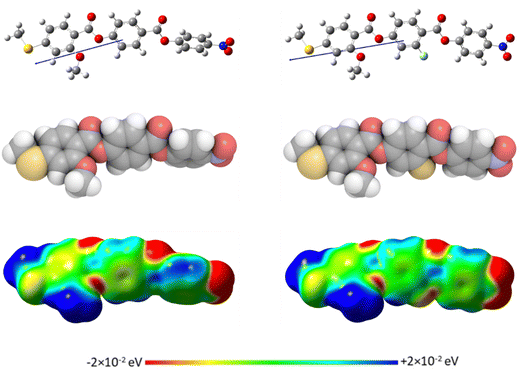 |
| Fig. 8 Molecular modelling of: S1 (left) and S3 (right) calculated at the B3LYP/6-31(d) level of theory. The molecules are visualized using: (top) ball and stick models, (middle) space-filling models and (bottom) electrostatic potential surfaces. The arrow indicates the direction of the calculated dipole moment, with the head representing positive charge moving to the base which is negative. | |
S4 contains two fluorine substituents in the same positions as the individual fluorine atoms in S2 and S3. This change has extinguished the nematic phase and a direct NF–I transition is observed, corresponding to a reduction in TNI of at least 40 K compared to S1. In contrast, the stability of the NF has increased again and TNFI for S4 is 23 K higher than TNFNX observed for S1. It is also noteworthy that TNFI for S4 is higher than TNFN of S2 by 7 K and of S3 by 3 K. The dipole moment of S4 is 12.4 D and higher than that of either S2 or S3. It is clear that the effects of the two fluorine atoms on the stability of the NF phase are not simply additive and such a relationship would predict a TNFI for S4 of around 142 °C. The rather modest increases in the stability of the NF phase between S4, and S2 and S3 is not immediately apparent from Madhusudana's model. If the F-substituent in S2 acts to reduce the amplitude of the charge density on the terminal ring, and that in S3 increases the amplitude of the charge density on the middle ring, then the combined effect in S4 should be to increase the difference between these two amplitudes, further increasing the stability of the NF phase. Presumably the change in molecular shape must also be considered and this will not be simply additive, nor is it straightforward to assess.
S5 also contains two fluorine substituents but both are attached to the middle aromatic ring, and these have reduced TNI by 42 K compared to S1. The single fluorine substituent on the middle ring in S3 reduced TNI by 17 K compared to S1, and so the second fluorine substituent has had a more pronounced effect on TNI than the first, reducing TNI by 25 K. The stability of the NF phase shown by S5 is higher than that of S1, but TNFN is lower than that of S3 by 5 K even though its dipole moment of 11.8 D is higher than that of S3 of 11.2 D. These effects may be attributed to the change in molecular shape on the addition of the second fluorine substituent, and presumably this also spreads the electron distribution on the middle phenyl ring reducing the amplitude of the charge density wave relative to that of the terminal ring.
All three fluorine substituents are included in S6, and this has extinguished nematic behaviour in comparison to S1 indicating a reduction in TNI of at least 47 K. The value of TNFI for S6 is essentially the same as TNFN shown by S2 and S5 although its dipole moment of 13.0 D is the highest in this collection of molecules. It is becoming increasingly apparent that the magnitude of the dipole moment alone is not a good indicator of the stability of the NF phase. S6 shows the largest reduction in TNI compared to S1 suggesting that the three fluorine substituents have a significant effect on molecular shape, and this presumably offsets a larger increase in the stability of the NF phase based on the predicted increment in stability associated with the addition of the fluorine substituent on the terminal phenyl ring.
For comparative purposes, the transitional properties of the corresponding methoxy-substituted materials to S1–6, the O1–6 compounds are listed in Table 2 and their structures shown in Fig. 1. O5 and O6 were prepared as part of this study and their phase assignments based on the observation of a characteristic schlieren texture for the nematic phase, Fig. 9(a) and a banded texture for the NF phase similar to that which we have reported previously, Fig. 9(b).18,20,25 For O5 our studies have revealed a previously overlooked N phase and the characterisation data for this are provided in the ESI.† The remaining data have been extracted from the literature.3,8,18,20 The trends in phase behaviour within these two sets of compounds, S1–6 and O1–6, are similar and tend to only differ in the magnitude of the temperature differences between compounds, Fig. 10. These temperature differences are most striking in the compounds containing more than one fluorine substituent for which the increase in the stability of the NF phase is significantly larger in the Sn compounds. For example, the stability of the NF phase shown by S6 is 16 K higher than that of S1, whereas the value of TNFI shown by O6 is the same as that of TNFN is for O1. This may reflect the weaker relative effect multiple fluorine substituents have on the shape of the Sn compounds given the larger methylthio group and we return to this issue later.
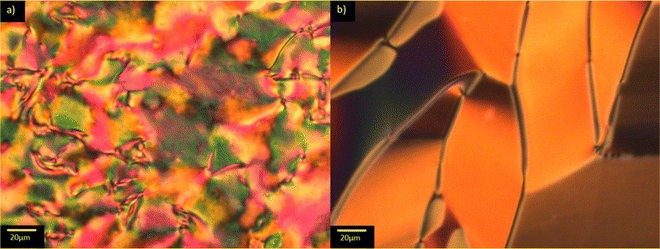 |
| Fig. 9 Polarised optical microscope textures observed for O6: (a) highly birefringent texture of the NF phase viewed between untreated glass slides at 125 °C; (b) banded texture of the NF phase in a cell treated for planar alignment at 125 °C. | |
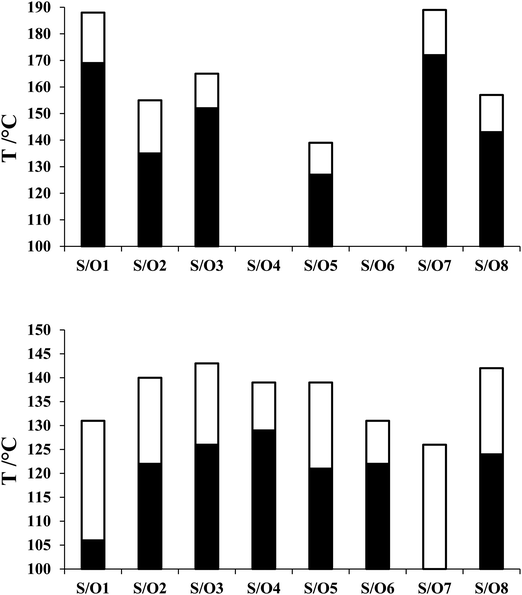 |
| Fig. 10 Comparison of the N–I (top) and NF–N/NX/I (bottom) transition temperatures of the Sn series (filled bar) and On series (open bar). | |
In S7 the methoxy substituent on the terminal ring has been moved to the middle ring and this increases TNI by just 3 K compared to S1. This small change reflects the small change in the shape anisotropy of the molecule. By contrast, TNXN has fallen by 14 K and the NF phase is no longer observed for S7. This suggests that the addition of the methoxy unit to the middle ring has reduced the amplitude of the charge density wave given its electron donating character. As would be expected the addition of a fluorine substituent ortho to the nitro group giving S8 promotes NF behaviour and reduces TNI compared to S7, Fig. 10. Again, similar trends in behaviour are observed for the corresponding methoxy substituted materials O7 and O8, see Table 2. The NX phase is again not observed in the On compounds.
The transitional behaviour of the corresponding Sn and On materials, Table 2, is identical except for the pairs S1 and O1 (RM734), and S7 and O7. In both S1 and S7 the NX phase is observed but not seen in the corresponding O compounds. In addition, the NF phase is not observed for S7 but is for O7. Indeed, isolated droplets of S7 can be cooled to 60 °C with no sign of a phase change within the NX phase, implying a reduction in TNFN of at least 66 K compared to O7. Comparing the transition temperatures of each pair of compounds, Fig. 10, the methoxy-substituted compound consistently shows the higher values of TNFN, TNFI and TNI. For each of the transitions, the larger methylthio group contributes to a larger reduction in shape anisotropy and accounts in part for the lower transition temperatures, Fig. 11. In addition, the calculated dipole moment of each On compound is higher than that of the corresponding Sn material. The sterically bulkier methylthio group presumably inhibits the ability of the compounds to pack together in the parallel manner found in the NF phase.63 The observation of the NX phase for S1 and S7 may simply reflect the greater suppression of the NF phase by the methylthio group revealing the underlying NX phase rather than from any specific stabilising effect. The generality of these observations now requires further investigation.
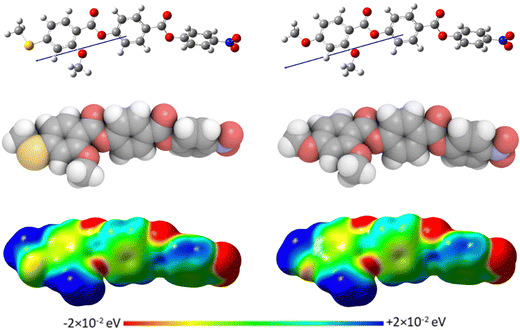 |
| Fig. 11 Molecular modelling of: S1 (left) and O1 (right) calculated at the B3LYP/6-31(d) level of theory. The molecules are visualized using: (top) ball and stick models, (middle) space-filling models and (bottom) electrostatic potential surfaces. The arrow indicates the direction of the calculated dipole moment, with the head representing positive charge moving to the base which is negative. | |
We recently reported the properties of the ethyl substituted compound, 2EC6F (Fig. 12), corresponding to S8 and O8. 2EC6F exhibits a NF–I transition at 132 °C.9 This implies that the decrease in TNI on replacing the methoxy group by an ethyl group is at least 25 K and for the methylthio group at least 11 K. By contrast, the value of TNFI is just 10 K lower than TNFN of O8 and 8 K higher than that of S8. At first sight the reduction in TNI between S8 and 2EC6F is surprising given that the ethyl group is more compact than the methylthio unit. We have shown, however, for other low molar mass systems that the values of TNI for systems containing methylthio and ethylthio terminal groups are higher than expected based on the behaviour of longer chain homologues, and attributed this to a specific interaction between the molecules, possibly a chalcogen bond.28 This would also account for the surprisingly high TNI seen for S8 but this interaction appears not to stabilise the NF phase. Instead, the ethyl group is a better electron donating group than methylthio, and this decreases the amplitude of the charge density at the end of the molecule stabilising the NF phase. This also accounts for the more stable NF phase shown by O8 given that the methoxy group is also a stronger electron donating group. In addition, in terms of molecule shape, the methoxy unit lies in the plane of the phenyl ring to which it is attached, whereas the ethyl group protrudes at an angle disrupting the formation of the NF phase.
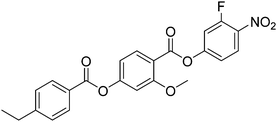 |
| Fig. 12 Molecular structure of 2EC6F. | |
Conclusions
We have reported the first set of ferroelectric nematogens containing a sulfur atom, S1–8, specifically in the form of a methylthio group, and compared their properties to those of the corresponding materials containing a methoxy group, O1–8. The difference in the transition temperatures within the Sn and On series may be accounted for in terms of the change in shape and electronic properties, based on the extent of fluorination along the molecular backbone. The behaviour of these materials can be accounted for within the framework of a molecular model developed by Madhusudana.63
The molecular dipole moment alone, however, is not necessarily a good indicator of the stability of the NF phase, with compounds possessing higher dipole moments exhibiting transitions to the NF phase at lower temperatures. On comparing the properties of corresponding members of the two sets of materials, the methoxy-substituted compound consistently shows the higher values of TNFN, TNFI and TNI. This is attributed to the larger reduction in molecular shape anisotropy associated with the methylthio group and its greater steric bulk. Evidently, the replacement of the terminal methoxy group by the methylthio group did not see a stabilisation of the NF phase, however, the NX phase was only observed for two compounds and both of which contained a terminal methylthio group. This may simply reflect the greater suppression of the NF phase by the methylthio group revealing the underlying NX phase rather than from any specific stabilising effect. A comparison using the reported behaviour of the ethyl substituted compound corresponding to S8 and O8 suggested that the interactions we have reported on previously,28 in terms of methylthio terminated low molar mass mesogens, do not appear to stabilise the NF phase, as they do for the conventional nematic phase, and this reinforces the view that molecular shape is an important factor in promoting the NF phase.
Conflicts of interest
There are no conflicts of interest to declare.
Acknowledgements
C.T.I. and J.M.D.S. acknowledge the financial support of the Engineering and Physical Sciences Research Council [EP/V048775/1]. The research was supported by the National Science Centre (Poland) under the grant no. 2021/43/B/ST5/00240.
References
- R. J. Mandle, S. J. Cowling and J. W. Goodby, A nematic to nematic transformation exhibited by a rod-like liquid crystal, Phys. Chem. Chem. Phys., 2017, 19, 11429–11435 RSC.
- H. Nishikawa, K. Shiroshita, H. Higuchi, Y. Okumura, Y. Haseba, S. I. Yamamoto, K. Sago and H. Kikuchi, A Fluid Liquid-Crystal Material with Highly Polar Order, Adv. Mater., 2017, 29, 1702354 CrossRef.
- R. J. Mandle, S. J. Cowling and J. W. Goodby, Rational Design of Rod-Like Liquid Crystals Exhibiting Two Nematic Phases, Chem. – A Eur. J., 2017, 23, 14554–14562 CrossRef CAS.
- X. Chen, E. Korblova, D. Dong, X. Wei, R. Shao, L. Radzihovsky, M. A. Glaser, J. E. Maclennan, D. Bedrov, D. M. Walba and N. A. Clark, First-principles experimental demonstration of ferroelectricity in a thermotropic nematic liquid crystal: Polar domains and striking electro-optics, Proc. Natl. Acad. Sci. U. S. A., 2020, 117, 14021–14031 CrossRef CAS PubMed.
- X. Chen, V. Martinez, E. Korblova, G. Freychet, M. Zhernenkov, M. A. Glaser, C. Wang, C. Zhu, L. Radzihovsky, J. E. Maclennan, D. M. Walba and N. A. Clark, The smectic ZA phase: Antiferroelectric smectic order as a prelude to the ferroelectric nematic, Proc. Natl. Acad. Sci. U. S. A., 2022, 120, e2217150120 CrossRef.
- Y. Song, M. Deng, Z. Wang, J. Li, H. Lei, Z. Wan, R. Xia, S. Aya and M. Huang, Emerging Ferroelectric Uniaxial Lamellar (Smectic AF) Fluids for Bistable In-Plane Polarization Memory, J. Phys. Chem. Lett., 2022, 13, 9983–9990 CrossRef CAS PubMed.
- X. Chen, V. Martinez, P. Nacke, E. Korblova, A. Manabe, M. Klasen-Memmer, G. Freychet, M. Zhernenkov, M. A. Glaser, L. Radzihovsky, J. E. Maclennan, D. M. Walba, M. Bremer, F. Giesselmann, N. A. Clark, N. Abbott and P. Palffy-Muhoray, Observation of a uniaxial ferroelectric smectic A phase, Proc. Natl. Acad. Sci. U. S. A., 2022, 119, e2210062119 CrossRef CAS.
- S. Brown, E. Cruickshank, J. M. D. Storey, C. T. Imrie, D. Pociecha, M. Majewska, A. Makal and E. Gorecka, Multiple Polar and Non-polar Nematic Phases, Chem. Phys. Chem., 2021, 22, 2506–2510 CrossRef CAS.
- E. Cruickshank, P. Rybak, M. M. Majewska, S. Ramsay, C. Wang, C. Zhu, R. Walker, J. M. D. Storey, C. T. Imrie, E. Gorecka and D. Pociecha, To be or not to be polar: the ferroelectric and antiferroelectric nematic phases, ACS Omega, 2023, 8, 36562–36568 CrossRef CAS.
- J. Li, R. Xia, H. Xu, J. Yang, X. Zhang, J. Kougo, H. Lei, S. Dai, H. Huang, G. Zhang, F. Cen, Y. Jiang, S. Aya and M. Huang, How Far Can We Push the Rigid Oligomers/Polymers toward Ferroelectric Nematic Liquid Crystals?, J. Am. Chem. Soc., 2021, 143, 46 CrossRef.
- S. Dai, J. Li, J. Kougo, H. Lei, S. Aya and M. Huang, Polar Liquid Crystalline Polymers Bearing Mesogenic Side Chains with Large Dipole Moment, Macromolecules, 2021, 54, 6045–6051 CrossRef CAS.
- D. Pociecha, R. Walker, E. Cruickshank, J. Szydlowska, P. Rybak, A. Makal, J. Matraszek, J. M. Wolska, J. M. D. Storey, C. T. Imrie and E. Gorecka, Intrinsically chiral ferronematic liquid crystals: an inversion of the helical twist sense at the chiral nematic – chiral ferronematic phase transition, J. Mol. Liq., 2021, 361, 119532 CrossRef.
- J. Li, H. Nishikawa, J. Kougo, J. Zhou, S. Dai, W. Tang, X. Zhao, Y. Hisai, M. Huang and S. Aya, Development of ferroelectric nematic fluids with giant-dielectricity and nonlinear optical properties, Sci. Adv., 2021, 7, eabf5047 CrossRef CAS PubMed.
- J. Li, Z. Wang, M. Deng, Y. Zhu, X. Zhang, R. Xia, Y. Song, Y. Hisai, S. Aya and M. Huang, General Phase-structure Relationship in Polar Rod-shaped Liquid Crystals: Importance of Shape Anisotropy and Dipolar Strength, Giant, 2022, 11, 100109 CrossRef CAS.
- R. Saha, P. Nepal, C. Feng, M. S. Hossain, M. Fukuto, R. Li, J. T. Gleeson, S. Sprunt, R. J. Twieg and A. Jákli, Multiple ferroelectric nematic phases of a highly polar liquid crystal compound, Liq. Cryst., 2022, 49, 1784–1796 CrossRef CAS.
- A. Manabe, M. Bremer and M. Kraska, Ferroelectric nematic phase at and below room temperature, Liq. Cryst., 2021, 48, 1079–1086 CrossRef.
- N. Sebastián, R. J. Mandle, A. Petelin, A. Eremin and A. Mertelj, Electrooptics of mm-scale polar domains in the ferroelectric nematic phase, Liq. Cryst., 2021, 48, 2055–2071 CrossRef.
- N. Tufaha, E. Cruickshank, D. Pociecha, E. Gorecka, J. M. D. Storey and C. T. Imrie, Molecular Shape, Electronic Factors and the Ferroelectric Nematic Phase, Chem. – A Eur. J., 2023, 29, e202300073 CrossRef CAS.
- J. Zhou, R. Xia, M. Huang and S. Aya, Stereoisomer effect on ferroelectric nematics: stabilization and phase behavior diversification, J. Mater. Chem. C, 2022, 10, 8762–8766 RSC.
- E. Cruickshank, R. Walker, J. M. D. Storey and C. T. Imrie, The effect of a lateral alkyloxy chain on the ferroelectric nematic phase, RSC Adv., 2022, 12, 29482–29490 RSC.
- H. Kikuchi, H. Matsukizono, K. Iwamatsu, S. Endo, S. Anan and Y. Okumura, Fluid Layered Ferroelectrics with Global C∞v Symmetry, Adv. Sci., 2022, 9, 2202048 CrossRef PubMed.
- R. J. Mandle, A new order of liquids: polar order in nematic liquid crystals, Soft Matter, 2022, 18, 5014–5020 RSC.
- N. Sebastián, M. Čopič and A. Mertelj, Ferroelectric nematic liquid-crystalline phases, Phys. Rev. E, 2022, 106, 021001 CrossRef.
- Y. Song, J. Li, R. Xia, H. Xu, X. Zhang, H. Lei, W. Peng, S. Dai, S. Aya and M. Huang, Development of emergent ferroelectric nematic liquid crystals with highly fluorinated and rigid mesogens, Phys. Chem. Chem. Phys., 2022, 24, 11536–11543 RSC.
- E. Cruickshank, A. Pearson, S. Brown, J. M. D. Storey, C. T. Imrie and R. Walker, The ferroelectric nematic phase: on the role of lateral alkyloxy chains, Liq. Cryst., 2023 DOI:10.1080/02678292.2023.2221651.
- R. Berardi, M. Ricci and C. Zannoni, Ferroelectric nematic and smectic liquid crystals from tapered molecules, ChemPhysChem, 2001, 2, 443–447 CrossRef CAS.
- R. J. Mandle, Supramolecular ferroelectric nematic materials, Liq. Cryst., 2022, 49, 2019–2026 CrossRef CAS.
- E. Cruickshank, G. J. Strachan, J. M. D. Storey and C. T. Imrie, Chalcogen bonding and liquid crystallinity: Understanding the anomalous behaviour of the 4′-(alkylthio)[1,1′-biphenyl]-4-carbonitriles (nSCB), J. Mol. Liq., 2021, 346, 117094 CrossRef.
- Y. Arakawa, S. Inui and H. Tsuji, Synthesis, phase transitions, and liquid crystal behavior of alkylthio azobenzenes, Tetrahedron, 2022, 122, 132958 CrossRef CAS.
- Y. Arakawa, S. Inui, K. Igawa and H. Tsuji, Alkylthio- and alkyl-substituted asymmetric diphenyldiacetylene-based liquid crystals: phase transitions, mesophase and single-crystal structures, and birefringence, Liq. Cryst., 2019, 46, 1621–1630 CrossRef CAS.
- Y. Arakawa, Y. Ishida, Y. Sasaki, S. Sasaki, M. Tokita and H. Tsuji, Alkylthio-based asymmetric liquid crystals: unravelling the substituent effects and intercalated cybotactic nematic and smectic phases, Mater. Adv., 2022, 3, 3218–3228 RSC.
- M. Alaasar, A. F. Darweesh, X. Cai, F. Liu and C. Tschierske, Mirror Symmetry Breaking and Network Formation in Achiral Polycatenars with Thioether Tail, Chem. – A Eur. J., 2021, 27, 14921–14930 CrossRef CAS.
- Y. Arakawa, S. Kang, J. Watanabe and G. I. Konishi, Assembly of thioether-containing rod-like liquid crystalline materials assisted by hydrogen-bonding terminal carboxyl groups, RSC Adv., 2015, 5, 8056–8062 RSC.
- E. Cruickshank, G. J. Strachan, M. M. Majewska, D. Pociecha, E. Górecka, J. M. D. Storey and C. T. Imrie, The effects of alkylthio chains on the properties of symmetric liquid crystal dimers, New J. Chem., 2023, 47, 7356–7368 RSC.
- E. Cruickshank, M. Salamończyk, D. Pociecha, G. J. Strachan, J. M. D. Storey, C. Wang, J. Feng, C. Zhu, E. Gorecka and C. T. Imrie, Sulfur-linked cyanobiphenyl-based liquid crystal dimers and the twist-bend nematic phase, Liq. Cryst., 2019, 46, 1595–1609 CrossRef CAS.
- Y. Arakawa, K. Komatsu and H. Tsuji, Twist-bend nematic liquid crystals based on thioether linkage, New J. Chem., 2019, 43, 6786–6793 RSC.
- K. Isoda, T. Ichikawa, K. Kaneko, M. Kondo, T. Sakurai, A. Seki, M. Hara, G. Watanabe, Y. Arakawa, Y. Arai, K. Horita, K. Komatsu and H. Tsuji, Twist-Bend Nematic Phase Behavior of Cyanobiphenyl-Based Dimers with Propane, Ethoxy, and Ethylthio Spacers, Crystals, 2022, 12, 1734 CrossRef.
- Y. Arakawa, K. Komatsu, Y. Ishida, T. Shiba and H. Tsuji, Thioether-Linked Liquid Crystal Trimers: Odd–Even Effects of Spacers and the Influence of Thioether Bonds on Phase Behavior, Materials, 2022, 15, 1709 CrossRef CAS.
- Y. Arakawa, K. Komatsu, S. Inui and H. Tsuji, Thioether-linked liquid crystal dimers and trimers: The twist-bend nematic phase, J. Mol. Struct., 2020, 1199, 126913 CrossRef CAS.
- Y. Arakawa, K. Komatsu, T. Shiba and H. Tsuji, Methylene- and thioether-linked cyanobiphenyl liquid crystal dimers CBn SCB exhibiting room temperature twist-bend nematic phases and glasses, Mater. Adv., 2021, 2, 1760–1773 RSC.
- A. J. Seed, K. J. Toyne and J. W. Goodby, Synthesis of some 2,4- and 2,5-disubstituted thiophene systems and the effect of the pattern of substitution on the refractive indices, optical anisotropies, polarisabilities and order parameters in comparison with those of the parent biphenyl and dithien, J. Mater. Chem., 1995, 5, 653–661 RSC.
- A. Seed, Synthesis of self-organizing mesogenic materials containing a sulfur-based five-membered heterocyclic core, Chem. Soc. Rev., 2007, 36, 2046–2069 RSC.
- M. K. Reddy, K. S. Reddy, M. Prakash and T. Narasimhaswamy, Synthesis and Characterization of Two Phenyl Ring Core-Based Thiophene Mesogens, Mol. Cryst. Liq. Cryst., 2013, 582, 1–14 CrossRef CAS.
- Y. Arakawa, S. Kang, S. Nakajima, K. Sakajiri, S. Kawauchi, J. Watanabe and G. Konishi, Synthesis of new wide nematic diaryl-diacetylenes containing
thiophene-based heteromonocyclic and heterobicyclic structures, and their birefringence properties, Liq. Cryst., 2014, 41, 642–651 CrossRef CAS.
- M. Hird, A. J. Seed, K. J. Toyne, J. W. Goodby, G. W. Gray and D. G. McDonnell, Synthesis, transition temperatures and optical anisotropy of some isothiocyanato-substituted biphenyls, J. Mater. Chem., 1993, 3, 851–859 RSC.
- G. J. Cross, A. J. Seed, K. J. Toyne, J. W. Goodby, M. Hird and M. C. Artal, Synthesis, transition temperatures, and optical properties of compounds with simple phenyl units linked by double bond, triple bond, ester or propiolate linkages, J. Mater. Chem., 2000, 10, 1555–1563 RSC.
- A. J. Seed, K. J. Toyne, J. W. Goodby and D. G. McDonnell, Synthesis, optical anisotropies, polarisabilities and order parameters of 4-cyanophenyl and 4-isothiocyanatophenyl 4′-butylsulfanylbenzoates with oxygen and sulfur substitution in the ester linkage, J. Mater. Chem., 1995, 5, 1–11 RSC.
- Z. Fang and C. Wu, Investigation on thermal behaviour and optical properties of non-symmetric cholesterol-based twin liquid crystals with thioester linkages, Liq. Cryst., 2020, 47, 1086–1099 CrossRef CAS.
- Y. Arakawa, Y. Sasaki, N. Haraguchi, S. Itsuno and H. Tsuji, Synthesis, phase transitions and birefringence of novel liquid crystalline 1,4-phenylene bis(4-alkylthio benzoates) and insights into the cybotactic nematic behaviour, Liq. Cryst., 2018, 45, 821–830 CrossRef CAS.
- Y. Arakawa and H. Tsuji, Phase transitions and birefringence of bistolane-based nematic molecules with an alkyl, alkoxy and alkylthio group, Mol. Cryst. Liq. Cryst., 2017, 647, 422–429 CrossRef CAS.
- Y. Arakawa and H. Tsuji, Selenium-linked liquid crystal dimers for twist-bend nematogens, J. Mol. Liq., 2019, 289, 111097 CrossRef.
- Y. Arakawa, H. Kuwahara, M. Tokita and G. Konishi, ichi & Tsuji, H. New fabrication approach to develop a high birefringence photo-crosslinked film based on a sulfur-containing liquid crystalline molecule with large temperature dependence of birefringence, Mol. Cryst. Liq. Cryst., 2018, 662, 197–207 CrossRef CAS.
- H. R. H. R. Stapert, S. Del Valle, E. J. K. Verstegen, B. M. I. Van der Zande, J. Lub and S. Stallinga, Photoreplicated Anisotropic Liquid-Crystalline Lenses for Aberration Control and Dual-Layer Readout of Optical Discs, Adv. Funct. Mater., 2003, 13, 732–738 CrossRef CAS.
- I. M. Syed, S. Kaur, H. E. Milton, D. Mistry, J. Bailey, P. B. Morgan, J. Cliff Jones, H. F. Gleeson, H. E. Milton, P. B. Morgan, J. H. Clamp, H. F. Gleeson, J. C. Jones and H. F. Gleeson, Novel switching mode in a vertically aligned liquid crystal contact lens, Opt. Express, 2015, 23, 9911–9916 CrossRef CAS.
- N. Kawatsuki, A. Yamashita, M. Kondo, T. Matsumoto, T. Shioda, A. Emoto and H. Ono, Photoinduced reorientation and polarization holography in photo-cross-linkable liquid crystalline polymer films with large birefringence, Polymer, 2010, 51, 2849–2856 CrossRef CAS.
-
M. J. Frisch, G. W. Trucks, H. B. Schlegel, G. E. Scuseria, M. A. Robb, J. R. Cheeseman, G. Scalmani, V. Barone, B. Mennucci, G. A. Petersson, H. Nakatsuji, M. Caricato, X. Li, H. P. Hratchian, A. F. Izmaylov, J. Bloino, G. Zheng, J. L. Sonnenberg, M. Hada, M. Ehara, K. Toyota, R. Fukuda, J. Hasegawa, M. Ishida, T. Nakajima, Y. Honda, O. Kitao, H. Nakai, T. Vreven, J. A. Montgomery, J. E. Peralta, F. Ogliaro, M. Bearpark, J. J. Heyd, E. Brothers, K. N. Kudin, V. N. Staroverov, R. Kobayashi, J. Normand, K. Raghavachari, A. Rendell, J. C. Burant, S. S. Iyengar, J. Tomasi, M. Cossi, N. Rega, J. M. Millam, M. Klene, J. E. Knox, J. B. Cross, V. Bakken, C. Adamo, J. Jaramillo, R. Gomperts, R. E. Stratmann, O. Yazyev, A. J. Austin, R. Cammi, C. Pomelli, J. W. Ochterski, R. L. Martin, K. Morokuma, V. G. Zakrzewski, G. A. Voth, P. Salvador, J. J. Dannenberg, S. Dapprich, A. D. Daniels, J. B. Farkas Foresman, J. V. Ortiz, J. Cioslowski and D. J. Fox, Gaussian 09, Revision B.01, Gaussian, Inc., Wallingford CT. ( 2010) Search PubMed.
- M. Tarini, P. Cignoni and C. Montani, Ambient occlusion and edge cueing to enhance real time molecular visualization, IEEE Trans. Vis. Comput. Graph., 2006, 12, 1237–1244 Search PubMed.
- H. Nishikawa, K. Sano, S. Kurihara, G. Watanabe, A. Nihonyanagi, B. Dhara and F. Araoka, Nano-clustering mediates phase transitions in a diastereomerically-stabilized ferroelectric nematic system, Commun. Mater., 2022, 3, 89 CrossRef CAS.
- H. Nishikawa, K. Sano and F. Araoka, Anisotropic fluid with phototunable dielectric permittivity, Nat. Commun., 2022, 13, 1142 CrossRef CAS PubMed.
- X. Zhao, J. Zhou, J. Li, J. Kougo, Z. Wan, M. Huang and S. Aya, Spontaneous helielectric nematic liquid crystals: Electric analog to helimagnets, Proc. Natl. Acad. Sci. U. S. A., 2021, 118, e2111101118 CrossRef CAS.
- A. Erkoreka, J. Martinez-Perdiguero, R. J. Mandle, A. Mertelj and N. Sebastián, Dielectric spectroscopy of a ferroelectric nematic liquid crystal and the effect of the sample thickness, J. Mol. Liq., 2023, 387, 122566 CrossRef CAS.
- X. Chen, Z. Zhu, M. J. Magrini, E. Korblova, C. S. Park, M. A. Glaser, J. E. Maclennan, D. M. Walba and N. A. Clark, Ideal mixing of paraelectric and ferroelectric nematic phases in liquid crystals of distinct molecular species, Liq. Cryst., 2022, 49, 1531–1544 CrossRef CAS.
- N. V. Madhusudana, Simple molecular model for ferroelectric nematic liquid crystals exhibited by small rodlike mesogens, Phys. Rev. E, 2021, 104, 014704 CrossRef CAS.
Footnotes |
† Electronic supplementary information (ESI) available. See DOI: https://doi.org/10.1039/d3ma00446e |
‡ Present Address: School of Pharmacy and Life Sciences, Robert Gordon University, Aberdeen, AB10 7GJ, U.K. |
|
This journal is © The Royal Society of Chemistry 2024 |