DOI:
10.1039/D4FO01395F
(Paper)
Food Funct., 2024,
15, 8459-8476
Nutritional, antioxidant and biological activity characterization of orange peel flour to produce nutraceutical gluten-free muffins†
Received
25th March 2024
, Accepted 20th July 2024
First published on 25th July 2024
Abstract
Celiac disease – a prevalent food intolerance – requires strict adherence to a lifelong gluten-free (GF) diet as the only effective treatment. However, GF products often lack soluble fibre and have a high glycaemic index. Consequently, there is a pressing need in the food industry to develop GF products with improved nutritional profiles. In this context, the impact of incorporating orange peel flour (OPF) into muffins undergoing sourdough fermentation was examined, focusing on their technological, antioxidant, and nutritional characteristics. The functional properties of OPF were investigated using human colon carcinoma HCT8 cells as a model system. Treatment with OPF extract demonstrated a notable reduction in malignant cell viability and intracellular ROS levels, indicating potent antioxidant capabilities. Western blot analysis revealed significant alterations in key signalling pathways, including increased phosphorylation of NF-kB at serine 536 and reduced intracellular levels of caspase-3, alongside increased phosphorylation of RIPK3 and MLKL, suggesting potential involvement in necroptosis. OPF incorporation in muffins with sourdough increased antioxidant activity, reduced glycaemic index, and affected the volatile profile. Furthermore, based on simulated colonic fermentation, muffins with OPF showed a slight prebiotic effect, supported by the significant increase in bacillus-shaped lactic acid bacteria and Clostridia population. Overall, OPF-enriched muffins demonstrated considerable antioxidant effects and impacts on cell viability, underscoring their potential as functional ingredients in GF products. These findings signify the prospect of OPF enhancing the nutritional profiles and conferring health benefits of GF muffins.
1. Introduction
Celiac disease (CD) is a chronic autoimmune disorder that causes an immune reaction of the body to the intake of gluten protein complex found in many grains, including barley, wheat, and rye.1 In Italy, more than 200
000 patients have a confirmed diagnosis of CD with a prevalence of approximately 1%.2 Typically, CD displays a benign resolution and alleviation of the main symptoms upon the onset of a gluten-free (GF) diet. However, failing to adhere to the GF diet increases the risk of developing gastrointestinal cancer or intestinal lymphoma.3 In this contest, GF baking is very challenging due to differences not only in production formulation but also in production technologies. From a technological point of view, gluten removal poses serious limitations in the management of GF bakery products.4 From a nutritional perspective, GF products have imbalanced nutritional profiles, containing high levels of saturated fatty acids and sugars and lacking several nutrients, such as dietary fibre, iron, zinc, magnesium, calcium, vitamin B12, and folic acid.5 Considering the related issues, various strategies are employed to meet the needs of individuals with CD and improve the quality of GF. Although additives are commonly used, fermentation processes, including natural leavening, are also key to improving product quality and acceptance.6,7 In addition, GF products are often characterized by a high predicted glycaemic index (pGI) due to their starch-based composition.8 It is well known the problem related to the high pGI, for individuals with metabolic disorders, such as diabetes and obesity increases the risk of cardiovascular diseases and the onset of certain diseases including cancer.9
In both CD and non-celiac gluten sensitivity, in which gluten triggers adverse gastrointestinal symptoms, a GF diet remains the only therapy adopted to date. However, several trials have shown how strict adherence to a GF diet leads to an imbalance in microbial composition related to the absence of wheat in GF baked goods, resulting in a critical deficiency of fructans.10 Fructans are a type of fibre with prebiotic properties, promoting the growth of beneficial bacteria and maintaining healthy gut microbiota.11 Oxidative stress, characterized by increased levels of reactive oxygen species (ROS), reduced antioxidant capacity, and a pro-inflammatory state are the main processes potentially involved in gluten toxicity.12 However, antioxidants and polyphenols play a pivotal role in the prevention of oxidative stress-induced human diseases.13 Fruits, known for their high polyphenol content, have been shown to exert anti-inflammatory, antithrombotic, and antiproliferative effects.13,14 Among them, the effects of table grape polyphenolic extracts on the mechanisms of oxidation, cellular inflammation, and metastasis have been studied, which can inhibit cell proliferation and growth by affecting cell morphology and inhibiting their ability to migrate.15 In addition, fruit- and vegetable-derived polyphenols modulated miRNAs involved in various cellular processes, such as inflammation and apoptosis.16–18
Citrus fruits, prominent in the Mediterranean Diet, offer significant nutraceutical benefits. Oranges, for instance, contain flavonoids like hesperidin, naringin, neohesperidin, naringenin, nobiletin, and tangeretin, predominantly found in the juice, which contribute to human health.19 Even orange peel flour (OPF) represents an excellent source of dietary fibre and polyphenols including flavonoids, amino acids, triterpenes, phenolic acids, carotenoids, and contains many nutrients, including vitamins C, A, and B, minerals (calcium, phosphorus, potassium).19,20 It is worth noting that oranges are widely consumed worldwide in both peel and juice form. However, during the production of orange juice, only about half of the weight of the fresh orange is processed into juice, resulting in substantial residues (peel, pulp, seeds) constituting the remaining 50% of the orange weight.21 In the vision of sustainability, one alternative for improving the management of these wastes is the implementation of new recovery processes.22 Orange by-products, due to the high content of bioactive compounds,23 can be of particular importance to the scientific community for their unique and enhanced therapeutic properties against various chronic diseases such as cancer, diabetes, and cardiovascular conditions. These by-products could also be used in the formulation of new GF foods with healthy properties. Numerous studies have explored the antioxidant activity and nutritional value of OPF in various food matrices, such as ice cream and yogurt.24–26
This study examined the functional responses of OPF extracts in HCT8 human colon cancer cells. In addition, research on the incorporation of OPF into GF baked goods is limited, and comprehensive studies assessing the impact of adding OPF to GF muffins undergoing fermentation with sourdough on technological, antioxidant, and nutritional profiles are lacking. Based on these observations, our study aimed to investigate the in vitro effects of OPF extract and OPF-enriched digested muffins on cell viability and intracellular oxidative balance. In addition, we evaluated the impact of OPF muffins on glycaemic index, simulated faecal microbiota, texture, and volatile profile. These analyses provide insights into the potential benefits and effects of incorporating OPF into GF baking.
2. Materials and methods
2.1 Chemical characterization of OPF
2.1.1 OPF extract preparation.
OPF – supplied by the company Packtin Srl (Italy) – was obtained by cold drying the orange pulp, without the addition of additives, colourings, or preservatives. OPF extract was prepared according to Caponio et al. with some modifications.27 Briefly, 3 g of OPF was mixed with water (1
:
10 w/v), vortexed for 10 min, sonicated for 15 min (Elmasonic S 60 H, ELMA, Singen, Germany), and finally centrifugated at 12
000g for 10 min (SL 16R Centrifuge, Thermo Scientific, Waltham, MA, USA) to recover the extract. Extractions were repeated twice more with 30 mL of water. The three extracts were combined, filtered as above reported, and stored at −20 °C until analysis. All extracts were prepared in triplicate.
2.1.2 Total phenol content and antioxidant activity.
OPF extract was evaluated for the total phenol content (TPC) according to the Folin–Ciocalteu method, as reported in Caponio et al.28 Briefly, to 980 μL of H2O Milli-Q, 20 μL of appropriately diluted extract and 100 μL of Folin–Ciocalteu reagent were added. After 3 min, 800 μL of 7.5% Na2CO3 were added and then the sample was stored in the dark for 60 min. The absorbance was read at 720 nm using an Evolution 60s UV-visible spectrophotometer (Thermo Fisher Scientific, Rodano, Italy). The results were expressed as mg of gallic acid equivalents (GAE) per g of dry weight (DW) sample (mg GAE per g DW). Each sample was analysed in triplicate. The antioxidant activities of the extracts were measured by ABTS and DPPH assays, as reported by Caponio et al.28 The DPPH (2,2-diphenyl-1-picrylhydrazyl) assay was performed by preparing a solution of DPPH 0.08 mM in ethanol. In cuvettes for spectrophotometry, 50 μL of each sample was added to 950 μL of DPPH solution. After 30 min in the dark, the decrease in absorbance was measured at 517 nm using an Evolution 60s UV-visible spectrophotometer (Thermo Fisher Scientific, Rodano, Italy). The ABTS [2,2′-azino-bis(3-ethylbenzothiazoline-6-sulfonic acid)] radical was generated by a chemical reaction with potassium persulfate (K2S2O8). Briefly, 25 mL of ABTS (7 mM in H2O) was spiked with 440 μL of K2S2O8 (140 mM) and kept in the dark at room temperature for 12–16 h. The working solution, by diluting with H2O, was prepared to obtain a final absorbance at 734 nm equal to 0.80 ± 0.02.29 The decrease in absorbance was measured at 734 nm after 8 min of incubation. Results were expressed as μmol Trolox equivalents (TE) per g of DW. Each sample was analysed in triplicate.
2.1.3 Naringin and neohesperidin determination by UHPLC-DAD.
A UHPLC Ultimate 3000RS Dionex (Thermo Fisher Scientific, Waltham, MA, USA) was used for the determination of naringin and neohesperidin. The UHPLC system accounted for a quaternary pump, autosampler, column compartment, and detector. The analytical separation was achieved as previously reported with some modification.30 A Hypersil GOLD aQ C18 column was used (length 100 mm, internal diameter 2.1 mm, and particle size 1.9 μm), held at 30 °C and a constant flow of 0.3 mL min−1 with water–formic acid (90
:
10 v/v) (solvent A) and acetonitrile–formic acid (99.9
:
0.1 v/v) (solvent B). The gradient program of solvent A was as follows: 0–26 min from 94% to 45%; 26–33 from 45% to 30%; 33–35 min isocratic at 30%. Then, equilibration was performed at the initial conditions for 9 min. The PDA detector was set to scan from 220 to 600 nm of wavelength managed by a 3D field. Quantitative analysis was performed according to the external standard method based on calibration curves obtained by injecting different concentrations of standard solutions of naringin and neohesperidin (Sigma Aldrich).
2.1.4 Proximate composition.
Moisture content was determined by a thermobalance (Ladwag MAC 110/NP, Radwag, Wagi Elektroniczne, Poland). Protein content (total nitrogen ×6.25), ash, lipid, and total dietary fibre were determined using the AOAC, 2006, methods 979.0, 923.03, 945.38, and, 985.29, respectively.31 Carbohydrates were calculated as a difference by subtracting the total dietary fiber, protein, ash, moisture, and lipid contents from 100. All the analyses mentioned were done in triplicate.
2.2
In vitro assays of OPF samples on cell cultures
2.2.1 Cell culture and treatments.
The human ileocecal adenocarcinoma cell line, HCT8, was cultured as previously described.27 Briefly, cells were grown in Advanced RPMI-1640 supplemented with 10% FBS, 100 i.u. per mL penicillin, 100 μg mL−1 streptomycin at 37 °C in 5% CO2. Cells were left under basal condition (untreated), or treated with OPF extract (200, 500, 700 μg mL−1) for 24 h.
2.2.2 Antibodies.
NFKβ (1
:
200), pNFkB (1
:
200), and RIPK3 (1
:
2000) were purchased by Santa Cruz Biotechnology (Santa Cruz, CA). Also, caspase 3 (1
:
300) and pRIPK3 (1
:
1000) were obtained from Cell Signalling Technology, (Danvers, MA, USA). MLKL (1
:
500) and pMLKL (1
:
500) were bought from Biorbyt, (Cambridge, UK, 1
:
500).
2.2.3 Calcein-AM cell viability assay.
Calcein-AM is a non-fluorescent and membrane-permeable compound. In viable cells, intracellular esterase converts calcein-AM to a green fluorescent and membrane-impermeant compound, that accumulates in viable cells having intact plasma membranes.
HCT8 cells were treated as described above. After treatment, cells were incubated with calcein-AM (1 μM) at 37 °C for 45 min, and then the fluorescence signal was measured and analysed. As an internal positive control, cells were incubated with ethanol (90%) for 1 min.
2.2.4 ROS detection.
ROS were detected as already shown.32 After treatments, cells were incubated with dihydro rhodamine-123 (10 μM) at 37 °C for 30 min and then recovered in a complete medium for 30 min. Cells were lysed in RIPA buffer containing 150 mM NaCl, 10 mM Tris-HCl pH 7.2, 0.1% SDS, 1.0% Triton X-100, 1% sodium deoxycholate, and 5 mM EDTA. Samples were centrifuged at 12
000g for 10 min at 4 °C and the supernatants were used for ROS detection. As a positive control, cells were treated with tert-butyl hydroperoxide (tBHP, 2 mM for 30 min). The fluorescence emission signal was recorded using a fluorimeter FLUOstar Omega (BMG LABTECH, Offenburg, Germania) at excitation and emission wavelengths of 508 and 529 nm, respectively.
2.2.5 Cell lysates and western blotting.
HCT8 cells were seeded onto 60 mm diameter Petri dishes, treated with 500 μg mL−1 of OFP and lysed in RIPA buffer containing 150 mM NaCl, 10 mM Tris-HCl pH 7.2, 0.1% SDS, 1.0% Triton X-100, 1% deoxycholate and 5 mM EDTA, 1 mM PMSF, 2 mg mL−1 leupeptin and 2 mg mL−1 pepstatin, 10 mM NaF and 1 mM sodium orthovanadate. Cellular debris was removed by centrifugation at 12
000g for 10 min at 4 °C. The supernatants were collected and used for the western blotting analyses. Proteins were separated using 10% or 12% stain-free polyacrylamide gels (Bio-Rad Laboratories, Inc., Hercules, CA, USA) under reducing conditions. Protein bands were electrophoretically transferred onto membrane PVDF Immobilon-P (Bio-Rad Laboratories, Inc., Hercules, CA, USA) and incubated in EveryBlot, blocking solution (Bio-Rad Laboratories, Inc., Hercules, CA, USA). Blots were then incubated with primary antibodies overnight. Immunoreactive bands were detected with secondary goat anti-rabbit and anti-mouse horseradish peroxidase-coupled antibodies obtained from Bio-Rad (Bio-Rad Laboratories, Inc., Hercules, CA, USA). Membranes were incubated with Clarity TM Western ECL Substrate (Bio-Rad Laboratories, Hercules, CA, USA), and the signals were visualized with the ChemiDoc System gels (Bio-Rad Laboratories, Inc., Hercules, CA, USA). Obtained bands were normalized to total protein using stain-free technology gels (Bio-Rad Laboratories, Inc., Hercules, CA, USA). Densitometry analysis was performed using Image Lab gels (Bio-Rad Laboratories, Inc., Hercules, CA, USA).
2.3 Chemical and nutritional characterization of OPF-enriched muffins
2.3.1 Sourdough preparation and selection.
Type-II sourdough (tII-SD) with a dough yield (DY, dough weight × 100/flour weight) 200 was made using a commercial brown rice flour (Oltre le Intolleranze, Ostuni, BR, Italy) and a multi-strain inoculum of Lactobacillus reuteri ATCC 23272 & Lactobacillus plantarum LPAL. Two batches of tII-SDs were prepared, varying the combination of incubation temperatures at 25 °C and 30 °C, along with a starter cell density of 6
log CFU per g. To confirm the initial cell density, counting was conducted on plates containing tII-SD samples on Man Rogosa and Sharpe (MRS) agar culture medium. After 24 h of incubation, the pH values and the lactic acid bacteria (LAB) density was collected. The pH was measured using an ultrabasic ub-10 pH meter (Denver Instrument Company, located in Arvada, Colorado, USA), equipped with a food penetration probe. The pH measurement of 3.49 ± 0.01 after 24 h of incubation suggests that the environment or mixture containing tII-SD became slightly acidic during that time. To determine the LAB cell density, 5 g of tII-SD was suspended in 45 mL of a sterile sodium chloride solution (0.9 g L−1) and homogenized in a Bag Mixer 400 P (Interscience, St Nom, France) at room temperature. Serial 10-fold dilutions were then plated with De Man, Rogosa, and Sharpe agar media (MRS agar; Oxoid, Basingstoke, Hampshire, UK) modified with the addition of 1% (w/w) maltose and 5% (w/w) yeast extract and adjusting the pH to 5.6 value. The plated LAB counts were incubated for 48 h at 30 °C. The LAB cell density of tII-SD was of about 8
log CFU per g.
The decision to incubate at 30 °C was based on the observed pH values. This temperature falls within the mesophilic range, which is typically suitable for the growth of a wide variety of microorganisms.
2.3.2 GF muffin preparation.
GF muffin batches were manufactured at the pilot plant of the Department of Soil, Plant, and Food Science of the University of Bari (Italy). Details of muffin formulations are explained in Table 1, in which six formulations were listed: (i) tII-SD GF muffin (SD); (ii) tII-SD GF muffin with OPF extract (SD-OPF); (iii) baker's yeast GF muffin produced without the addition OPF (YB); (iv) baker's yeast GF muffin produced with the addition of OPF (YB-OPF); (v) chemical's yeast GF muffin produced without the addition of OPF (YC); (vi) chemical's yeast GF muffin produced with the addition of OPF (YC-OPF). The sourdough muffins (SD and SD-OPF) formulations were obtained according to a double fermentation process accounting for, first, the production of tII-SD (fermentation for 24 h at 30 °C), which was then added (10% w/w of flour) in a dough containing brown rice flour, milk, baker's yeast (Lievital ®) (1.50% w/w). In OPF-containing muffin formulation (SD-OPF, YB-OPF, and YC-OPF), the powder extract of OPF was also added (6% w/w) as a substitute for the brown rice flour. Each dough was prepared by mixing the ingredients for 10 min using a planetary mixer (Knetmaschine KM 370 CB Bomann) at 25 °C. The chemical yeast, supplied from © PANEANGELI was first solubilized in water, while other ingredients were gradually added during the mixing. The SD- and YB-doughs were subjected to an incubation step (1.5 h at 30 °C) before being baked at 175 °C for 20 min (Combo3, Zucchelli, Verona, Italy), while the YC-doughs were directly baked after kneading. Three experimental replicates for each formulation were tested.
Table 1 Formulation of the experimental muffins. SD, tII-SD gluten-free muffin; YB, baker's yeast gluten-free muffin; YC, chemical's yeast gluten-free muffin; SD-OPF, tII-SD gluten-free muffin with OPF; YB-OPF, baker's yeast gluten-free muffin with OPF; YC-OPF, chemical's yeast gluten-free muffin with OPF
Ingredients |
SD |
YB |
YC |
SD-OPF |
YB-OPF |
YC-OPF |
Brown rice flour (g) |
200 |
200 |
200 |
186.8 |
186.8 |
186.8 |
Sugar (g) |
12 |
12 |
12 |
12 |
12 |
12 |
Stevia (g) |
8 |
8 |
8 |
8 |
8 |
8 |
Orange peel flour (g) |
— |
— |
— |
13.2 |
13.2 |
13.2 |
Partially skimmed milk (mL) |
200 |
200 |
200 |
200 |
200 |
200 |
Sunflower oil (mL) |
90 |
90 |
90 |
90 |
90 |
90 |
Bakery yeast (g) |
3.3 |
3.3 |
— |
3.3 |
3.3 |
— |
Chemical yeast (g) |
— |
— |
12.1 |
— |
— |
12.1 |
Sourdough (g) |
22 |
— |
— |
22 |
— |
— |
2.3.3 Antioxidant activity and total phenol content of muffin.
Phenol compounds were extracted from OPF muffins using a hydroalcoholic extraction according to Caponio et al., with slight modifications.27 Briefly, 1 g of muffin was mixed with methanol 80% (1
:
10 w/v), sonicated for 20 min (Elmasonic S 60 H, ELMA, Singen, Germany), vortexed for 30 min, and finally centrifugated at 8000g for 10 min (SL 16R Centrifuge, Thermo Scientific, Waltham, MA, USA) to recover the hydroalcoholic extract. Then extracts were stored at −20 °C until analysis. All extracts were prepared in triplicate. Muffin extracts were evaluated for the total phenol content (TPC) and the antioxidant activity (ABTS and DPPH assays) according to methods reported in paragraph 2.1.1. Each sample was analysed in triplicate.
2.3.4
In vitro starch hydrolysis.
In vitro starch hydrolysis of muffin was determined according to Difonzo et al. simulating the in vivo digestion of starch.33 Briefly, 1 g of samples were subjected to a determination of starch. Hydrolysed starch was measured as the amount of glucose released, using the D-glucose assay kit (GOPOD format) (Megazyme, Wicklow, Ireland). Starch was calculated as glucose (mg) × 0.9 (the conversion factor). Simulated digests were dialyzed (cut-off of the membrane: 12
400 Da) for 180 min. Aliquots of dialysate, containing free glucose, and partially hydrolysed starch were sampled every 30 min and further treated with amyloglucosidase. Then, free glucose was determined using the above-mentioned enzyme-based kit and finally converted into hydrolysed (digested) starch in the muffin. Control white wheat bread was used as the control to estimate the hydrolysis index (HI = 100). The predicted glycaemic index (pGI) was calculated using the equation pGI = 0.549 × HI + 39.71 as described by Caponio et al.34 Each sample was analysed in triplicate.
2.3.5 Muffin simulated colonic fermentation in vitro.
To prepare the faecal medium, a 0.9% w/v NaCl solution was supplemented with specific additives, including K2HPO4 (2 g L−1), C2H3NaO2 (5 g L−1), C6H17N3O7 (2 g L−1), MgSO4 (0.2 g L−1), MnSO4 (0.05 g L−1), glucose (2 g L−1), inulin (4 g L−1), fructo-oligosaccharides (4 g L−1), Tween 80 polysorbate (1 mL L−1), bacteriological peptone (5 g L−1), and yeast extract (5 g L−1).7 The pH was adjusted to 7.0 using a 6 M NaOH solution, followed by sterilization at 121 °C for 20 minutes. All reagents, except for inulin and fructo-oligosaccharides provided by Farmalabor Srl, were sourced from Sigma-Aldrich. Following Pérez-Burillo's recommendation,35 the faecal medium was modified by incorporating 0.5% (w/v) of experimental and control muffins derived from in vitro starch hydrolysis solutions of both permeate and retentate. The inoculum (faecal slurry) preparation followed the detailed procedure outlined previously.7 Fresh faeces from a healthy volunteer without recent antibiotics or probiotics usage were collected in sterile tubes, filled to 4/5 of the total volume. Within one hour of sampling, the faeces were processed in bags with a 250 μm filter and distilled water (32% w/v). The mixture was homogenized for 3 minutes in a lab stomacher, followed by centrifugation (8000g, 20 min, 4 °C). Pellets containing viable cells from 2 mL of the solution were recovered, while 10 mL of the faecal medium were added to constitute the faecal batch. These batches were anaerobically incubated at 37 °C for 48 hours under gentle stirring conditions (150 rpm).
2.3.6 Viable faecal microbiota profiling.
Profiling of viable faecal microbiota was conducted through plate counts based on serial 10-fold dilutions in a sterile saline solution (NaCl 0.9%). Various culturing media were employed, including plate count agar (PCA), Wilkins-Chalgren anaerobe agar (WCAn), de Man, Rogosa, and Sharpe (MRS) agar, M17 agar, violet red bile glucose agar (VRBGA), and modified Bifidobacterium agar (mBifA). These media facilitated the assessment of cell densities (log
10 CFU per mL) for total aerobes (TAMC), total anaerobes (TANMC), lactic acid bacteria (LAB – bacilli), LAB (cocci), Enterobacteriaceae, and faecal Bifidobacterium, respectively. Except for mBifA, obtained from Becton Dickinson GmbH (Heidelberg, Germany), all other media were procured from Oxoid Ltd (Basingstoke, Hampshire, England). WCAn and mBifA were subjected to anaerobic incubation, while the remaining media were incubated aerobically at 37 °C. The incubation duration adhered to the specifications provided by the respective medium manufacturers. To validate data obtained from MRS and M17 media, cells were randomly observed using optical microscopy.
2.3.7 Determination of volatile compounds of muffin.
Volatile organic compounds (VOCs) were determined through headspace solid phase micro-extraction (SPME) coupled to gas-chromatography/mass spectrometry (GC/MS) as reported by Caponio et al.34 Briefly, 0.5 g of muffin was weighed in 12 mL vials and 150 μL of 1-propanol was added as internal standard plus 4 mL of NaCl (20% w/v aqueous solution). Vials were sealed by butyl rubber septa and aluminium crimp caps. Before extraction of VOCs, vials were shaken for 2 min with a laboratory vortex to promote sample homogenization. The extraction of volatile compounds was carried out by exposing a 75 μm carboxen/polydimethylsiloxane (CAR/PDMS) SPME fibre (Supelco, Bellefonte, PA, USA) in the headspace of the vials at 40 °C for 50 min; then the fibre was desorbed for 6 min in the injection port of gas-chromatograph, operating in a split-less mode at 230 °C for 3.5 min. For the separation of volatile compounds, a Thermo Trace 1300 gas-chromatograph equipped with a Thermo ISQ series 3.2 SP1(Thermo Scientific, Waltham, MA, USA) mass-spectrometer was used under the following conditions: injector temperature, 250 °C; flow of 1.5 mL min−1; pressure of the carrier (helium) 30 kPa. The oven temperature was held for 5 min at 35 °C, then increased by 5 °C min−1 to 50 °C and held in isothermal conditions for 5 min, then raised to 210 °C at 5.5 °C min−1, and finally held constant at 210 °C for 5 min. The mass detector was set as: interface temperature 230 °C, source temperature 230 °C, ionization energy 70 eV, and scan range 33–260 amu. Peak identification was done using Xcalibur V2.0 Qual Browse software (Thermo Fisher Scientific, Waltham, MA, USA) by matching with the reference mass spectra of the NIST (National Institute of Standards and Technology, Gaithersburg, MD, USA) library. The volatile compounds were quantified by standardizing the peak areas of compounds of interest with the peak area of internal standard (1-propanol). The analysis was carried out in duplicate.
2.3.8 Texture analyses.
Texture parameters of muffins were carried out according to Caponio et al. 2024.36 Briefly, the analysis was performed on muffin (2 × 2 × 2 cm) using a texture analyser Z1.0 TN (Zwick Roell, Ulm, Germany), equipped with a stainless-steel cylindrical probe (36 mm diameter), and a 50 N load cell was used. Data were acquired by the TestXPertII version 3.41 software (Zwick Roell, Ulm, Germany). Two compressive cycles were performed at a 1 mm s−1 probe compression rate and 50% sample deformation in both the compression, with 5 s pauses before the second compression. The analyses were carried out on twenty-four muffins (four per type, in triplicate).
2.4
In vitro assays of digested muffins enriched with OPF on cell cultures
HCT8, human ileocecal adenocarcinoma cell line was cultured as mentioned in paragraph 2.2.1. Briefly, cells were incubated with digested muffins obtained from in vitro starch hydrolysis at different concentrations (1 mg mL−1, 0.1 mg mL−1, 0.01 mg mL−1, 0.001 mg mL−1 at 37 °C, 5% CO2, for 24 h). Calcein-AM assay and ROS detection were performed as indicated in paragraphs 2.2.3 and 2.2.4, respectively.
2.5 Statistical analysis
The results were expressed as the mean ± standard deviation (SD). Significant differences (p ≤ 0.05) were determined by analysis of variance unidirectional (ANOVA), followed by Tukey test for multiple comparisons. The statistical analysis was carried out using the statistical software Minitab (Minitab Inc., State College, PA, USA).
3. Results and discussion
3.1 Chemical characterization of OPF
The OPF extract was first evaluated for its chemical composition. Proximate composition, phenol content of the OPF extract, as well as the antioxidant activity, is detailed in Table 2. The proximate composition of OPF in terms of protein, fibre, and carbohydrates was consistent with those in the literature.23,37 As expected, OPF was low in fat and protein. On the contrary, OPF was high in fibre, containing 34.4 g/100 g fibre. However, results from other authors reported higher results for fibre, about 64.3%, but similar results for protein and lipids, 6.70% and 0.89%, respectively.38 These differences in composition are most likely due to variations in fruit growth and maturity conditions.39
Table 2 Chemical composition, antioxidant activity and polyphenol content of OPF
Parameters |
OPF |
Data are represented as means ± SD of three lots of OPF. Abbreviations: ABTS, 2,2′-azino-bis(3-ethylbenzothiazoline-6-sulfonic acid); DPPH, 2,2-diphenil-1-picrylhydrazyl; OPF, orange peel flour; TPC, total phenol content. |
Moisture (g per 100 g) |
8.14 ± 0.05 |
pH |
4.15 ± 0.01 |
Protein (g per 100 g) |
5.3 ± 0.03 |
Lipid (g per 100 g) |
1.1 ± 0.08 |
Fibre (g per 100 g) |
34.4 ± 0.06 |
Carbohydrates (g per 100 g) |
45.1 ± 0.29 |
Salt (g per 100 g) |
<0.1 |
ABTS (μmol TE per g) |
19.71 ± 1.15 |
DPPH (μmol TE per g) |
18.43 ± 0.94 |
TPC (mg GAE per g) |
19.08 ± 0.83 |
Neohesperidin (mg g−1) |
2.02 ± 0.04 |
Naringin (mg g−1) |
0.87 ± 0.01 |
Remarkably, the OPF extract exhibited high antioxidant activity in both ABTS and DPPH assays, with values of 19.71 ± 1.15 μmol TE per g and 18.43 ± 0.94 μmol TE per g, respectively, as recently published.40,41 Moreover, the TPC evaluated according to the Folin–Ciocalteu method was 19.08 mg GAE per g. OPF is a source of polyphenols that exert antioxidant activity. The main phenolic compounds have been detected and quantified in OPF (Table 2). According to the literature, orange peel waste mainly contains neohesperedin and naringin, according to previous research findings.37,42
3.2
In vitro characterization of OPF samples on cell cultures
3.2.1 ROS detection and cell viability.
To assess the antioxidant function of the OPF extract, colon carcinoma HCT8 cells were treated as described before and used for measuring the ROS content. As depicted in Fig. 1A, treatment with OPF extract caused a slight but significant increase in the intracellular ROS content compared to untreated cells, likely suggesting that OPF extract might exert a pro-oxidant effect. However, in the presence of tert-butyl hydroxy peroxide (tBHP), a synthetic pro-oxidant compound, incubation with the OPF extract significantly reduced intracellular ROS content, indicating an antioxidant action (Fig. 1B). Importantly, one of the main features of cancer cells compared to healthy cells is the continuous pro-oxidant intracellular condition that leads to oxidative stress.43 Of course, the increased intracellular ROS content is derived from an increased metabolism and the blocking of the antioxidant signal mechanisms. In this respect, ROS can accelerate cell growth and survival by acting as signalling molecules.44 Conversely, an increased level of intracellular ROS would also make cancer cells more prone to different forms of cell death. Therefore, the effect of the OPF extract on cell viability was further investigated by the calcein assay.
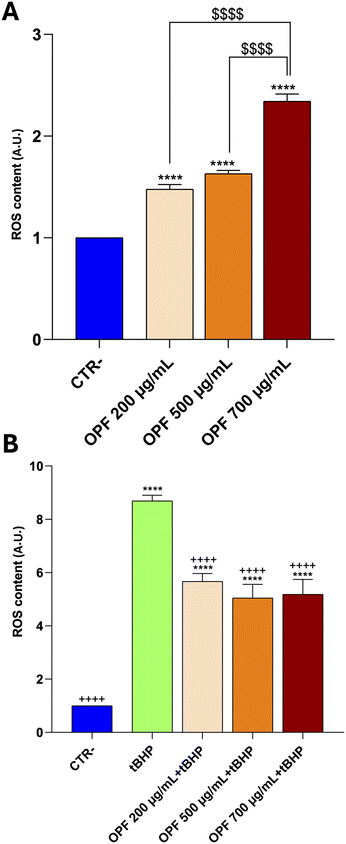 |
| Fig. 1 ROS content measured using dihydrorhodamine-123 fluorescence in HCT8 cells treated for 24 h at 200, 500, and 700 μg mL−1 indicated concentrations. Panel A shows the result of cells untreated (CTR−) compared to 200, 500, 700 μg mL−1 of OPF. Panel B shows the result of cells untreated (CTR−) and a positive control cell treated with the oxidant tBHP (tBHP) compared to 200, 500, 700 μg mL−1 of OPF + tBHP. Data are shown as mean ± SEMs and analyzed by one-way ANOVA followed by followed by Tukey's multiple comparison test. * means a significant difference (p ≤ 0.05) of samples vs. CTR−; + means a significant difference (p ≤ 0.05) of samples vs. tBHP; $ means a significant difference (p ≤ 0.05) among the concentrations of OPF. | |
HCT8 cells were left untreated (CTR−) or treated with increasing concentrations (200, 500, 700 μg mL−1) of the OPF extract for 24 h (Fig. 2). As an internal positive control (CTR+) cells were treated with 90% ethanol for 1 min. Compared to the untreated cells (CTR−), treatment with OPF at 500 μg mL−1 and 700 μg mL−1 significantly reduced cell viability. By contrast, treatment with OPF at 200 μg mL−1 does not alter cell viability compared to untreated cells. Altogether, these findings suggest that OPF extract, already at 500 μg mL−1, may promote cell death possibly by inducing oxidative stress. Therefore, 500 μg mL−1 concentration was selected for the subsequent experiments.
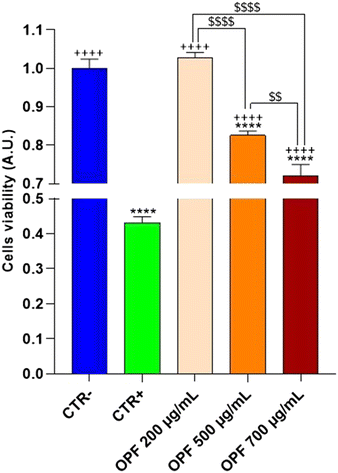 |
| Fig. 2 Cell viability of OPF. Cells were treated for 24 h at the indicated concentrations of 200, 500, 700 μg mL−1. Control (CTR−) cells were untreated (100% of vitality) and 90% of ethanol was used as a positive control (CTR+). Cells were then assayed for vitality by the calcein assay. Data are shown as mean ± SEMs and analyzed by one-way ANOVA followed by followed by Tukey's multiple comparison test. * means a significant difference (p ≤ 0.05) of samples vs. CTR−; + means a significant difference (p ≤ 0.05) of samples vs. CTR+; $ means a significant difference (p ≤ 0.05) among the concentrations of OPF. | |
3.2.2. Evaluation of cell death.
Apoptosis is a caspase-mediated programmed cell death.45 Caspase-3, a pivotal player in apoptosis, has been implicated in various cellular functions, including angiogenesis and tumour relapse. In the colon cancer cell line, HCT116, the caspase-3 knocking down correlated with a less proliferative and invasive phenotype.46 Immunoblotting analysis (Fig. 3) revealed that treatment of HCT8 cells with the OPF extract resulted in a significant decrease of the abundance of caspase-3. These observations likely suggest that incubation with OPF extract may reduce malignant cell viability (Fig. 2) by decreasing the expression of caspase-3. Anyhow, caspase-3 reduction might also result in a down-regulation of cell apoptosis.
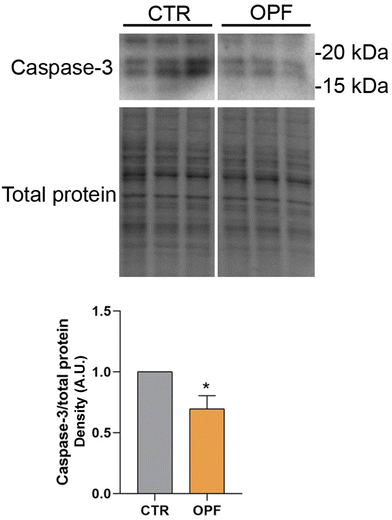 |
| Fig. 3 Western blotting analysis of proteins in HCT8 cells exposed with OPF extract for 24 h for caspase-3 and relative blots. Controls (CTR) used in the blot were run on the same gel of the OPF samples. Data are shown as mean ± SEMs and analyzed by one-way ANOVA followed by followed by Tukey's multiple comparison test. * means p < 0.01. | |
To further investigate the impact of the OPF extract on intracellular signalling modulating cell death, the function of the nuclear factor kB (NFkB) was investigated. Importantly, NFkB is a crucial modulator of inflammation and different forms of programmed cell death.47 Specifically, it has been reported that inhibition of NFkB promotes apoptosis of hepatocytes.48 Conversely, NFkB function counteracts the induction of apoptosis.49 In the present study, immunoblotting analysis showed that incubation with OPF extract caused a slight but significant increase in phosphorylation of NFkB at serine 536 which would explain the possible reduction in cell apoptosis (Fig. 4). On another hand, phosphorylation of NFkB at serine 536 reduces the stability of NFkB,47 likely indicating that the OPF extract would exert an anti-inflammatory function by decreasing the abundance of NFkB. Also, in vivo studies revealed that phosphorylation at serine 536 downregulates NFkB signalling to prevent abnormal and dangerous inflammation50 that, in this case, might be sustained by increased ROS (Fig. 1). Importantly, inhibition of NFkB signalling promotes cell necroptosis in murine keratinocytes.51 In hepatic cells, necroptosis was elicited by ROS-induced NFkB/RIPK1 pathways.52 Therefore, to further investigate the cell fate under OPF extract treatment, cell necroptosis was analysed. Immunoblotting studies (Fig. 5 and 6) revealed that incubation with the OPF extract significantly increased to phosphorylation of RIPK3 and MLKL which are key players in promoting necroptosis.53 Interestingly, the inhibition of certain programmed cell death, such as apoptosis made cells more prone to carcinogenesis.54 In this respect, HCT8 cells displayed a very low level of p53 which is a known oncosuppressor protein that functionally promotes cell apoptosis.55 Impaired apoptosis can be considered a hallmark of cancer. In some cancers, however, induction of necroptosis may represent an alternative type of programmed cell death playing a defensive action against carcinogenesis.56,57 Data showing the phenolic composition of OPF (Table 2) revealed the presence of substantial amounts of naringenin and hesperidin that have been suggested as promising bioactive molecules against cancer progression.58 Our data suggest for the first time that phenols in OPF might stimulate ROS/NFkB-induced cell necroptosis in cells showing possible impaired apoptosis (Fig. 3).
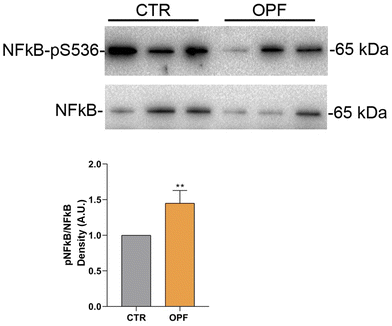 |
| Fig. 4 Western blotting analysis of proteins in HCT8 cells exposed with OPF extract for 24 h for NFkB-pS536, NFkB, and relative ratio. Data are shown as mean ± SEMs and analyzed by one-way ANOVA followed by followed by Tukey's multiple comparison test. ** means p < 0.001. | |
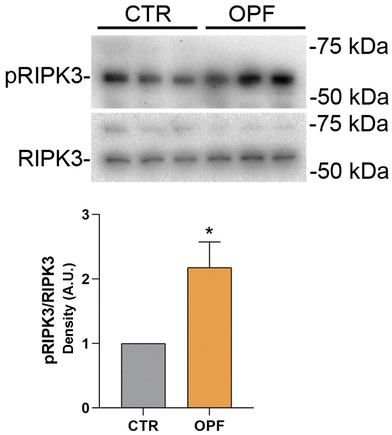 |
| Fig. 5 Western blotting analysis of proteins in HCT8 cells exposed with OPF extract for 24 h for pRIPK3, RIPK3, and relative ratio. Data are shown as mean ± SEMs and analyzed by one-way ANOVA followed by followed by Tukey's multiple comparison test. * means p < 0.01. | |
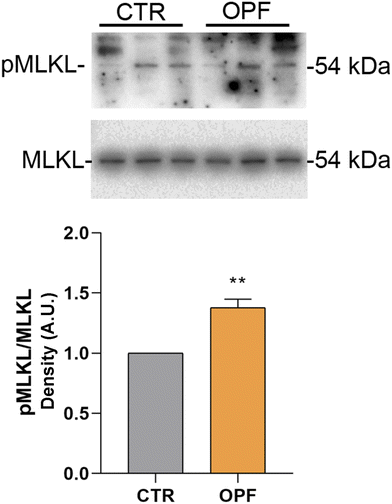 |
| Fig. 6 Western blotting analysis of proteins in HCT8 cells exposed with OPF extract for 24 h for pMLKL, MLKL, and relative ratio. Data are shown as mean ± SEMs and analyzed by one-way ANOVA followed by followed by Tukey's multiple comparison test. ** means p < 0.001. | |
3.3 Chemical and nutritional characterization of OPF-enriched muffins
3.3.1 Antioxidant activity and polyphenol content of muffins.
The antioxidant activity and TPC were assessed in muffin samples to investigate antioxidant properties (Table 2). These values tended to increase when OPF was added to the formulations. Specifically, the pairwise comparison between muffin samples with or without OPF revealed not significant ABTS differences between YC-OPF and YC muffins, by the contrast a significant increase in ABTS values in OPF-supplemented muffin samples, i.e., SD-OPF and YB-OPF. A similar trend was observed for DPPH results, highlighting a significant increase in YB-OPF and YC-OPF compared to YB and YC, respectively. The muffin samples showed greater ABTS radical inhibitory activity than DPPH radical inhibition, suggesting the hydrophilic nature of most of the antioxidant compounds produced.59 These results agree with the value of the TPC of muffins, which were observed higher TPC by the addition of OPF in muffins formulation, compared to those without OPF. The functionalization of muffin samples with OPF allowed us to obtain newly formulated products with an enhanced antioxidant capacity. Indeed, the evaluation of antioxidant capacity is a fundamental parameter to understand the real importance of this type of application in the food system. Our results are in line with those obtained from previous studies on functional cookies added with different amounts of kinnow (Citrus reticulate L.) peels, in which an increase in antioxidant activity (FRAP and DPPH) of the supplemented cookies was observed compared with the control, attributed to the higher phenolic content of the citrus peels used.60 Similarly, biscuits enriched with flour obtained from Bergamotto by-products and, other citrus fruits showed higher antiradical activity in ABTS and DPPH tests than control biscuits.61,62
Notably, the slight significant differences observed between products with and without OPF can also be attributed to the flour used in the muffin's formulation. Brown rice flour is rich in bioactive compounds, particularly phenolic acids, which contribute significantly to antioxidant activity.63
Regarding differences between fermentation types, the TPC value does not seem to be affected, as shown in Table 3 for both SD and YB samples, where only an increasing trend in the value appears for SD samples, but not significantly. A slight difference was observed for YC muffin in terms of ABTS and TPC content, while YC samples achieved the lowest DPPH value compared to SD and YB samples. Several yeast species, including Saccharomyces cerevisiae, are known to possess functional properties (such as potential probiotic effects) as well as improve the bioavailability of phenolic compounds of the products leading to an increased antioxidant capacity.64 The fermentation mixture may also affect the bioavailability of polyphenols. In this regard, scientific evidence exploited the role of Limosilactobacillus reuteri (Ls., basonym Lactobacillus reuteri) and Lactobacillus acidophilus, and their co-culturing, on fermentation performance and the resulting scavenging activity.59,65 The results showed higher values of antioxidant activity in the samples fermented by Ls. reuteri, rather than by the combination. Therefore, the simultaneous use of the two bacteria did not intensify proteolytic and antioxidant activity. Further, fermentative processes, as well as cooking steps, could deeply affect the antioxidant properties of the bakery products.66,67 Also, Saccharomyces cerevisiae and Lacticaseibacillus rhamnosus (basonym Lactobacillus rhamnosus) were able to increase TPC after fermentation of four different flours.68
Table 3 Antioxidant activity and polyphenol content of muffins enriched with OPF
Parameters |
SD |
YB |
YC |
SD-OPF |
YB-OPF |
YC-OPF |
Data are shown as mean ± SD and analyzed by one-way ANOVA followed by followed by Tukey's multiple comparison test. Different letters (a, b, and c) in the same row mean a significant difference (p ≤ 0.05) among all samples. Comparing the same yeast, “*” indicates a significant difference (P < 0.05) between samples without OFP and samples enriched with OPF. Abbreviations: SD, tII-SD gluten-free muffin; YB, baker's yeast gluten-free muffin; YC, chemical's yeast gluten-free muffin; SD-OPF, tII-SD gluten-free muffin with OPF; YB-OPF, baker's yeast gluten-free muffin with OPF; YC-OPF, chemical's yeast gluten-free muffin with OPF; OPF, orange peel flour. |
ABTS (μmol TE per g) |
1.28 ± 0.06c |
1.22 ± 0.05c |
3.14 ± 0.08a |
1.77 ± 0.03b,* |
1.95 ± 0.05b,* |
2.94 ± 0.18a |
DPPH (μmol TE per g) |
0.89 ± 0.08b |
0.79 ± 0.05b |
0.24 ± 0.04d |
0.98 ± 0.04ab |
1.06 ± 0.04a,* |
0.63 ± 0.05c,* |
TPC (mg GAE per g) |
2.75 ± 0.43b |
2.69 ± 0.24b |
2.54 ± 0.36b |
2.99 ± 0.28a |
3.32 ± 0.17a,* |
3.32 ± 0.22a,* |
3.3.2
In vitro starch hydrolysis of muffins.
In addition to antioxidant activity, we further investigated the potential functional activity of enriched muffins on the glycaemic index. To achieve this goal, GF muffins were tested in vitro to evaluate the predicted glycaemic index (pGI) (textured bar of Fig. 7), and hydrolysis index (HI) (textured bar of Fig. 7) of different muffin formulations. As shown in Fig. 7, samples with baker's yeast (YB) and chemical (YC) did not affect the reduction of pGI. Muffins with and without OPF extract presented a pGI value between 65.23% and 67.15%. In contrast, muffins with sourdough contributed to the reduction of pGI. In particular, the lowest value of pGI and HI was achieved in SD-OPF, 60.37% and 37.64%, respectively. Although there were no significant differences between SD and SD-OPF, it is noteworthy to observe the decreasing trend when OPF was added to the formulation, possibly suggesting that a higher level of OPF in the muffin would have improved the pGI.
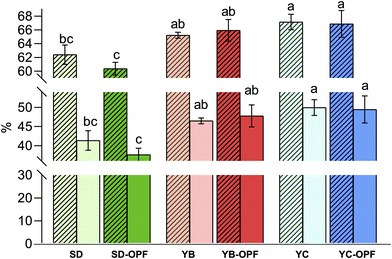 |
| Fig. 7 Results of predicted glycemic index (pGI) (textured bar) and hydrolysis index (HI) (smooth bar) of muffins with and without-OPF. Data are shown as mean of triplicates ±SD and analyzed by one-way ANOVA followed by Tukey's multiple comparison test. Different letters (a, b, and c) indicate a significant difference (p ≤ 0.05) among samples for pGI and HI determinations. | |
Data from the literature reported how a daily consumption of foods with a high glycaemic index is correlated with the risk of developing cardiovascular disease, insulin resistance, diabetes, and obesity.66,69 GF products often exhibit a higher glycaemic index compared to gluten-containing products. The presence of gluten in foods can inhibit the hydrolysis rates of starch in the small intestine, leading to an increased glycaemic response to carbohydrates upon its elimination from foods.70 Thus, it is important to adopt biotechnological strategies to develop new formulations with improved nutritional value. Bioconversion processes and fermentation strategies for agri-food by-products can both enhance digestibility and nutritional value and reduce levels of anti-nutritional factors in these substrates. Microbial fermentation of agri-food by-products is commonly used to produce ingredients with improved nutritional and health characteristics by promoting the release of bioactive molecules from the plant matrix or through bioconversion of compounds originally present in the by-products.71 Generally, the pGI of foods depends mainly on the amount and type of carbohydrates contained but is also influenced by several other factors, including the presence of organic acids, such as lactic and acetic acid produced by fermentation.72 Fermentation processes are well-known for their role in reducing the glycaemic index of foods.7,34 These studies have also evaluated the influence of the sourdough technique on the glycaemic index, in addition to the health characteristics offered by the enrichment of polyphenols and dietary fibre compounds in OPF.
Furthermore, our results were in line with previous studies, which assessed a decreasing pGI value in muffins enriched with fibre-rich orange bagasse products, achieving a pGI of approximately 70 compared to the control.73 Therefore, the consumption of GF products with a medium/low glycaemic index, such as the SD-OPF sample, could potentially improve the health status of many individuals through significant changes in the glycaemic index.
3.3.3 Viable profiling of faecal microbiota post-simulated colonic fermentation.
To better assess the effectiveness of dietary OPF supplementation on human health, faecal microbiota fermentations were conducted in vitro. Considering the faecal microbiota inoculum, which was characterized before proceeding further with the fermentation in vitro (Table 4), the experiments utilized retentate (Fig. 8) or permeate solutions (ESI Fig. S1†) resulting from simulated enzymatic digestion, which were added to supplement the bacterial culturing medium. However, the impact of the permeate solution was not discussed in detail, despite its representation of the hydro-soluble fraction of foods absorbed in the proximal tract of the intestine and, consequently, it does not accurately reflect the fraction available for microbiota fermentation in the colonic environment.74
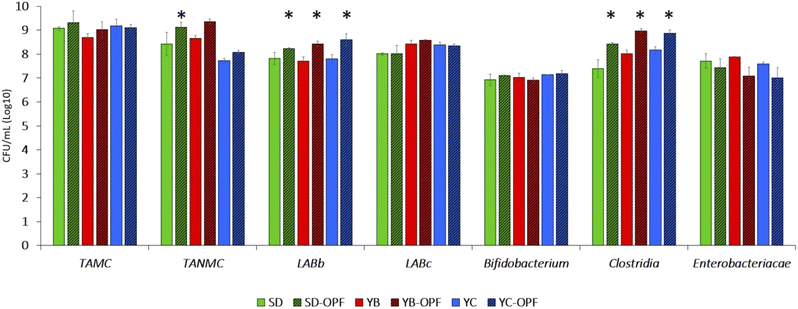 |
| Fig. 8 Viable bacterial count (log 10 CFU per mL ± SD) of faecal microbiota observed in batches simulating the colonic fermentation of retentates in vitro. Retentate solution contained the residual from the simulated digestion of muffin differing for the addition of OPF and type of fermentation (with chemical yeast, YC; baker's yeast YB; or type-II sourdough, SD). Asterisk (*) represents a significant difference (P < 0.05; two-tailed Student's t-test) compared cell density of samples without and with-OPF. Abbreviations: TAMC, total aerobic microbial count; TANMC, total anaerobic microbial count; LABb, bacillus-shaped lactic acid bacteria; LABc, coccus-shaped lactic acid bacteria. | |
Table 4 Viable bacterial count of faecal microbiota inoculum used for simulated colonic fermentation assay
Plated bacterial groups |
Viable cell density (log 10 CFU per mL) ± SD |
Abbreviations: TAMC, total aerobic microbial count; TANMC, total anaerobic microbial count; LABb, bacillus shaped lactic acid bacteria; LABc, coccus-shaped lactic acid bacteria. SD, standard deviation. |
TAMC |
6.3 ± 0.6 |
TANMC |
7.4 ± 0.7 |
LABb |
5.4 ± 0.5 |
LABc |
6.3 ± 0.3 |
Bifidobacterium
|
6.0 ± 0.2 |
Clostridia
|
8.2 ± 0.5 |
Enterobacteriaceae
|
5.1 ± 0.4 |
Regarding the results from retentate testing (Fig. 8), variations in OPF influence were observed among different bacterial groups. A 48-hour microbiota fermentation revealed that total aerobic microbial counts (TAMC) were unaffected by the presence or absence of OPF in the medium. However, OPF showed minimal stimulation of the growth of total anaerobes (TANMC), with statistical significance (P < 0.05) achieved only for the SD-OPF compared to itself without OPF. The LAB population was characterized by discerning bacillus-shaped (LABb) from coccus-shaped (LABc) cells, revealing a significant increase of 0.5–1 log in OPF-containing samples for the former group. By contrast, OPF did not stimulate the growth of LABc. Additionally, no prebiotic effect was observed on Bifidobacterium cell density. Therefore, although previous studies demonstrated how polyphenols also enhanced the growth and colonization of bifidobacterial strains, our results confirmed the effective suitability of OPF to stimulate the growth of some specific probiotics.22,75,76
The last two bacterial groups studied through plating were Enterobacteriaceae and cells belonging to the Clostridia class. Once again, OPF led to tendencies in decreasing the viability of Enterobacteriaceae, consistent with its recognized antimicrobial effectiveness against pathogens and pathobionts.77,78 However, it is worth noting how this effectiveness should be considered dose-dependently,78,79 with low concentrations unable to exert significant biological roles on microbes and, therefore, on gut microbiota.80 By contrast, the concentration of OPF used was sufficient to increase the viability of some faecal microbes mostly representative of healthy gut microbiota, including Ruminococcaceae (clostridial cluster IV) and Lachnospiraceae (clostridial cluster XIVa), which are important colonizers of the core microbiota in humans.81,82 In fact, different species within both these families play pivotal roles in the metabolism of undigested food by hosts, producing beneficial compounds such as short-chain fatty acids (SCFAs). These results were aligned with those recently obtained by Núñez-Gómez et al.,83 which observed an increased metabolism of SCFA as a consequence of OPF-fermentation by gut microbiota models in vitro (Núñez-Gómez et al., 2024
83). In line with this metabolic activity, while not species within Ruminococcaceae and Lachnospiraceae can be claimed as probiotics, they can be considered as health-promoting bacteria of the gut environment.84,85
3.3.4 Texture profile analysis of muffins.
Table 5 shows the results of the texture analysis (springiness, chewiness, cohesiveness, hardness) of the tested muffins. The data revealed significant variations in springiness, cohesiveness, and hardness based on the fermentation type, while chewiness remained unaffected in all samples except YC, where the value was significantly reduced. Moreover, the incorporation of OPF influenced the cohesiveness and hardness of the muffins. Although the addition of OPF, rich in fibre, could potentially enhance the structural properties of the samples and increase stiffness,86 our results found no changes in chewiness and hardness following the addition of OPF in SD-OPF and YB-OPF muffins compared to control muffins. This reduction in hardness was accompanied by lower chewiness values in YC-OPF, consistent with previous studies.87 In fact, OPF, and its fibre, are one of the contributing factors to these results, as assessed in a previous study, which emphasizes the reduction in chewiness and hardness after the addition of orange pomace in GF bread.88 The choice of chemical yeast also impacted springiness, with lower values observed in YC and YC-OPF samples compared to other muffins. Notably, muffins prepared with sourdough (SD-) and baker's yeast (YB-) exhibited higher springiness values. This result is also confirmed by other studies that found that sourdough influenced the textural properties of baked goods, improving their springiness and hardness.89,90 Additionally, according to Novotni et al. (2013),91 the strain of Lactobacillus plantarum used to produce sourdough positively influences product cohesion, as evidenced by higher cohesiveness values in SD samples compared to YB and YC samples. Replacing rice flour with OPF (SD-OPF) resulted in decreased cohesiveness, consistent with findings from other studies due to the increased ability of the fiber – introduced with OPF – to bind water.87
Table 5 Texture profile analysis of muffins enriched with OPF
Parameters |
SD |
YB |
YC |
SD-OPF |
YB-OPF |
YC-OPF |
Data are shown as mean ± SD and analyzed by one-way ANOVA followed by followed by Tukey's multiple comparison test. Different letters (a, b, and c) in the same row mean a significant difference (p ≤ 0.05) among all samples. Comparing the same yeast, “*” indicates a significant difference (P < 0.05) between samples between OFP and samples enriched with OPF. Abbreviations: SD, tII-SD gluten-free muffin; YB, baker's yeast gluten-free muffin; YC, chemical's yeast gluten-free muffin; SD-OPF, tII-SD gluten-free muffin with OPF; YB-OPF, baker's yeast gluten-free muffin with OPF; YC-OPF, chemical's yeast gluten-free muffin with OPF; OPF, orange peel flour. |
Springiness |
0.80 ± 0.02 a |
0.78 ± 0.13a |
0.64 ± 0.02bc |
0.71 ± 0.03abc |
0.77 ± 0.02ab |
0.62 ± 0.01c |
Chewiness (N) |
6.66 ± 0.52a |
6.44 ± 1.15a |
5.3 ± 0.45ab |
6.2 ± 0.62a |
6.17 ± 1.06a |
3.68 ± 0.49b |
Cohesiveness |
0.53 ± 0.02a,* |
0.46 ± 0.02b |
0.40 ± 0.02cd |
0.44 ± 0.03bc |
0.44 ± 0.03bc |
0.37 ± 0.02d |
Hardness (N) |
15.22 ± 1.24c |
17.37 ± 1.12bc |
20.83 ± 1.29a,* |
18.35 ± 1.35abc |
18.55 ± 1.51ab |
16.91 ± 0.66b |
3.3.5 Volatile profile of muffins.
The identification of volatile compounds contained in the muffins is reported in Table 6. Among the aldehydes, one of the most abundant compounds is hexanal, which showed no significant differences between the samples, except for YC-OPF in which the lowest concentration was found. The nonanal concentration was affected by the OPF addition but maintained the highest concentrations when sourdough and baker's yeast were used. A similar trend was found for the main carboxylic acids and alcohols according to Dingeo et al.92 Presumably, the higher content of aldehydes and alcohols in SD and YB could be due to the action of lactic acid bacteria and yeasts during the fermentation process promote the generation of hexanal, 1-hexanol, hexanoic acid.93 Ethanol was the most representative alcohol deriving from the pentose phosphate pathway of glucose, according to Lee et al.,94 or due to the heterofermentative LAB strains. The concentration of alcohols significantly decreased in OPF-added samples, probably due to an effect of the polyphenols on microorganism activity. Several studies highlighted the influence of phenolic compounds on the development of volatile compounds in the end products.95,96 According to Galvan-Lima et al.,97 the main volatile compounds in peel flours from citrus are terpenes, in fact, the addition of OPF in the product leads to an enrichment in these compounds, and especially in limonene.
Table 6 Volatile compounds of muffins
Volatile compounds |
SD |
YB |
YC |
SD-OPF |
YB-OPF |
YC-OPF |
The results are expressed in μg g−1. Data are represented as means ± SD of three lots. Different letters (a, b, and c) in the same row indicate significant differences at P < 0.05 according to two-way ANOVA followed by the Tukey's HSD test. Abbreviations: n.d., not detected. |
Aldehydes
|
Acetaldehyde |
0.26 ± 0.03b |
0.96 ± 0.24a |
n.d. |
0.96 ± 0.06a |
1.13 ± 0.26a |
n.d. |
Butanal, 2-methyl- |
1.12 ± 0.03b |
0.86 ± 0.10bc |
0.39 ± 0.02d |
0.79 ± 0.07c |
1.48 ± 0.15a |
0.13 ± 0.00d |
Butanal, 3-methyl- |
1.49 ± 0.05b |
3.10 ± 0.28a |
0.94 ± 0.08bc |
3.06 ± 0.12a |
1.51 ± 0.27b |
0.36 ± 0.03c |
Pentanal |
0.55 ± 0.00b |
0.83 ± 0.06a |
0.52 ± 0.02b |
0.86 ± 0.06a |
0.78 ± 0.09a |
0.19 ± 0.01c |
Hexanal |
20.59 ± 0.62ab |
23.03 ± 1.23a |
21.19 ± 0.94ab |
21.03 ± 0.81ab |
21.35 ± 1.16ab |
19.22 ± 2.56b |
Heptanal |
2.19 ± 0.56abc |
1.84 ± 0.23bc |
1.55 ± 0.06c |
3.38 ± 0.70ab |
3.94 ± 0.46a |
3.16 ± 0.36abc |
Octanal |
2.92 ± 0.25b |
2.36 ± 0.33b |
2.05 ± 0.29b |
6.74 ± 0.07a |
6.25 ± 0.28a |
5.98 ± 0.13a |
2-Heptenal, (Z)- |
1.91 ± 0.01bc |
1.93 ± 0.10bc |
0.73 ± 0.05c |
3.90 ± 0.58a |
4.83 ± 0.52a |
2.42 ± 0.16b |
Nonanal |
10.67 ± 1.60b |
9.38 ± 0.66b |
6.69 ± 1.10b |
23.78 ± 4.29a |
23.01 ± 1.03a |
13.38 ± 1.45b |
Furfural |
0.72 ± 0.01c |
0.33 ± 0.02c |
0.80 ± 0.10c |
3.69 ± 0.27a |
2.96 ± 0.07b |
0.33 ± 0.01c |
2-Octenal, (E)- |
2.80 ± 0.30b |
2.40 ± 0.37bc |
0.91 ± 0.01c |
5.81 ± 0.59a |
7.13 ± 0.88a |
2.98 ± 0.01b |
Benzaldehyde |
1.41 ± 0.08c |
1.74 ± 0.05bc |
1.69 ± 0.02bc |
3.05 ± 0.09a |
3.35 ± 0.39a |
2.29 ± 0.10b |
|
Ketones
|
2-Butanone |
0.85 ± 0.25a |
0.69 ± 0.07a |
0.59 ± 0.00a |
0.80 ± 0.00a |
0.63 ± 0.02a |
0.77 ± 0.04a |
2-Heptanone |
4.91 ± 0.19a |
6.60 ± 1.55a |
4.45 ± 0.34a |
5.39 ± 0.36a |
6.85 ± 0.53a |
5.24 ± 0.01a |
Acetoin |
1.58 ± 0.15b |
1.92 ± 0.02a |
0.20 ± 0.01d |
1.01 ± 0.11c |
1.56 ± 0.09b |
0.10 ± 0.00d |
5-Hepten-2-one, 6-methyl- |
1.43 ± 0.02c |
1.39 ± 0.09c |
1.06 ± 0.01c |
2.70 ± 0.25a |
3.00 ± 0.00a |
2.26 ± 0.05b |
2-Nonanone |
1.55 ± 0.13cd |
1.67 ± 0.27cd |
1.35 ± 0.07d |
2.47 ± 0.04bc |
3.54 ± 0.45a |
3.20 ± 0.20ab |
Ethanone, 1-(2-furanyl)- |
n.d. |
n.d. |
n.d. |
1.49 ± 0.00a |
1.75 ± 0.17a |
1.62 ± 0.22a |
|
Alcohols
|
Ethanol |
185.84 ± 19.13ab |
225.21 ± 25.83a |
6.76 ± 0.08c |
151.97 ± 10.88b |
156.97 ± 6.34b |
7.85 ± 0.81c |
1-Propanol, 2-methyl- |
8.35 ± 1.03b |
11.12 ± 0.53a |
0.73 ± 0.04d |
5.74 ± 0.06c |
5.93 ± 0.63c |
n.d. |
1-Butanol |
0.99 ± 0.04b |
1.62 ± 0.16a |
1.06 ± 0.03b |
n.d. |
n.d. |
n.d. |
1-Butanol, 3-methyl- |
155.25 ± 23.3a |
184.16 ± 7.09a |
6.45 ± 0.07c |
79.8 ± 2.22b |
72.01 ± 4.88b |
n.d. |
1-Pentanol |
6.13 ± 0.73b |
7.72 ± 0.04a |
4.40 ± 0.30c |
5.44 ± 0.08bc |
4.14 ± 0.15c |
2.51 ± 0.22d |
2-Buten-1-ol, 3-methyl- |
n.d. |
n.d. |
n.d. |
0.68 ± 0.00ab |
0.84 ± 0.21a |
0.34 ± 0.03bc |
1-Hexanol |
33.72 ± 4.49a |
37.52 ± 1.64a |
13.65 ± 0.57b |
31.40 ± 2.53a |
32.52 ± 1.55a |
15.95 ± 1.48b |
1-Octen-3-ol |
2.87 ± 0.01a |
3.02 ± 0.21a |
1.52 ± 0.05b |
n.d. |
n.d. |
n.d. |
1-Hexanol, 2-ethyl- |
2.37 ± 0.03c |
2.07 ± 0.24c |
3.09 ± 0.08c |
2.97 ± 0.16c |
4.47 ± 0.45b |
5.9 ± 0.41a |
1-Octanol |
1.86 ± 0.01c |
1.79 ± 0.04c |
0.83 ± 0.06c |
5.70 ± 0.52ab |
7.16 ± 1.08a |
4.24 ± 0.31b |
2-Furanmethanol |
0.77 ± 0.04bc |
0.56 ± 0.04c |
4.76 ± 0.01ab |
2.10 ± 0.19bc |
7.31 ± 0.93a |
7.18 ± 2.26a |
2-Methoxy-4-vinylphenol |
n.d. |
n.d. |
n.d. |
1.38 ± 0.13a |
1.31 ± 0.36a |
1.84 ± 0.60a |
Phenylethyl alcohol |
23.81 ± 0.50ab |
23.96 ± 1.00ab |
2.82 ± 0.28b |
22.85 ± 2.34ab |
38.31 ± 18.16a |
7.36 ± 0.27b |
|
Carboxylic acids
|
Acetic acid |
1.88 ± 0.40b |
1.45 ± 0.01bc |
0.46 ± 0.11c |
6.37 ± 0.44a |
6.45 ± 0.12a |
1.84 ± 0.01b |
Propanoic acid, 2-methyl- |
0.95 ± 0.20b |
1.28 ± 0.05b |
n.d. |
1.83 ± 0.09b |
8.03 ± 1.33a |
0.82 ± 0.05b |
Butanoic acid, 2-methyl- |
1.76 ± 0.16c |
1.67 ± 0.08c |
n.d. |
2.76 ± 0.35b |
4.29 ± 0.09a |
0.67 ± 0.00d |
Hexanoic acid |
3.00 ± 0.21cd |
1.96 ± 0.22d |
n.d. |
5.19 ± 0.35b |
7.36 ± 0.83a |
4.07 ± 0.33bc |
Octanoic acid |
1.38 ± 0.02ab |
1.18 ± 0.16ab |
0.37 ± 0.05b |
2.73 ± 0.50ab |
4.58 ± 2.46a |
2.66 ± 0.48ab |
Nonanoic acid |
1.66 ± 0.54bc |
1.45 ± 0.00bc |
0.65 ± 0.01c |
2.69 ± 0.57ab |
3.53 ± 0.09a |
1.57 ± 0.30bc |
|
Terpenes
|
3-Carene |
n.d. |
n.d. |
n.d. |
25.43 ± 1.52b |
29.67 ± 2.99ab |
34.95 ± 3.23a |
Limonene |
19.66 ± 0.50b |
23.81 ± 2.01b |
20.19 ± 2.86b |
7158.26 ± 227.09a |
8013.97 ± 417.53a |
8038.43 ± 310.27a |
Terpinene |
n.d. |
n.d. |
n.d. |
4.61 ± 0.66b |
5.33 ± 0.10ab |
6.35 ± 0.38a |
o-Cymene |
n.d. |
n.d. |
n.d. |
7.36 ± 0.14b |
8.59 ± 0.42a |
8.38 ± 0.53ab |
Linalool |
n.d. |
n.d. |
n.d. |
28.57 ± 2.66b |
34.76 ± 2.73ab |
41.02 ± 4.91a |
Terpineol |
1.09 ± 0.01b |
0.97 ± 0.12b |
0.79 ± 0.01b |
23.15 ± 2.26a |
30.19 ± 4.78a |
35.71 ± 6.79a |
|
Ester
|
Ethyl Acetate |
0.99 ± 0.06b |
1.44 ± 0.11a |
0.4 ± 0.01c |
0.87 ± 0.05b |
1.27 ± 0.24ab |
0.37 ± 0.03c |
|
Furan
|
Furan, 2-penthyl- |
5.45 ± 1.38a |
5.48 ± 1.63a |
1.59 ± 0.03b |
5.09 ± 0.28ab |
6.91 ± 0.04a |
4.48 ± 0.15ab |
Furfural |
0.72 ± 0.01c |
0.33 ± 0.02c |
0.80 ± 0.10c |
3.69 ± 0.27a |
2.96 ± 0.07b |
0.33 ± 0.01c |
3.3.6 ROS detection and cell viability in cells treated with digested OPF-enriched muffins.
To evaluate the effect of muffin extracts collected from in vitro digestion on the cell viability, HCT8 cells were left untreated (CTR−) or treated with the muffin extracts at different concentrations (1 mg mL−1, 0.1 mg mL−1, 0.01 mg mL−1, 0.001 mg mL−1). The concentrations of 0.1 mg mL−1, 0.01 mg mL−1, 0.001 mg mL−1 showed no statistical difference from CTR− (data not shown), thus the concentration used for subsequent investigations was 1 mg mL−1. As shown in Fig. 9, incubation of cells with the muffin extract at 1 mg mL−1 for 24 hours, significantly reduced cell viability compared to untreated cells in SD and YB fermented muffin. No significant reduction was instead found in YC fermented muffins. A slight but not significant reduction tendency has been found in cells treated with OPF muffin extract, likely suggesting that a higher level of OPF in the muffins would have been more effective in decreasing cell viability.
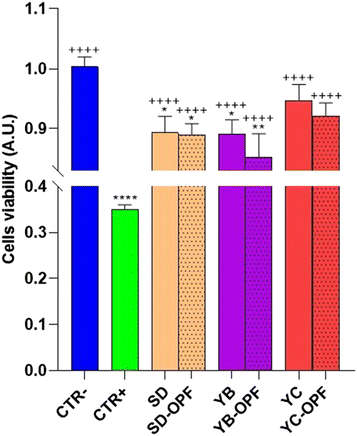 |
| Fig. 9 Cell viability of OPF-enriched muffins. HCT8 cells were treated for 24 h with 1 mg mL−1 of digested muffins. Control (CTR−) cells were untreated (100% of vitality) and 90% of ethanol was used as a positive control (CTR+). Cells were then assayed for vitality by the calcein assay. Data are shown as mean ± SEMs and analyzed by one-way ANOVA followed by followed by Tukey's multiple comparison test. * means a significant difference (p ≤ 0.05) of samples vs. CTR−; + means a significant difference (p ≤ 0.05) of samples vs. CTR+. | |
Subsequently, to investigate the effect of the muffin extracts on the intracellular oxidative state, HCT8 cells were left untreated or treated with 1 mg mL−1 of digested muffin extracts and assayed for ROS determination. Fig. 10 clearly shows the antioxidant effects observed in cells treated with the muffin extracts. Specifically, compared to cells treated with the pro-oxidant tBHP, a significant decrease in ROS content was detected in cells incubated with the muffin extracts regardless of the fermentation method. Nevertheless, a slight but not significant decrease in ROS content was found in cells receiving the extracts isolated from OPF muffins, compared to the extracts obtained by untreated muffins.
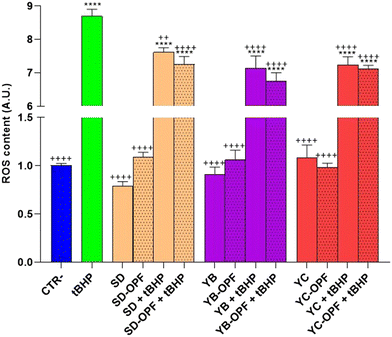 |
| Fig. 10 ROS content measured using dihydrorhodamine-123 fluorescence in HCT8 cells treated for 24 h with 1 mg mL−1 of digested muffins. Negative control cells were untreated (CTR−), and positive control cells were treated with the oxidant tBHP (tBHP). Data are shown as mean ± SEMs and analyzed by one-way ANOVA followed by followed by Tukey's multiple comparison test. * means a significant difference (p ≤ 0.05) of samples vs. CTR−; + means a significant difference (p ≤ 0.05) of samples vs. tBHP. | |
Conversely, under basal conditions, a slight but not significant increasing tendency in ROS content was detected in cells subjected to the extract isolated from OPF muffins SD and YB fermented. Again, a higher level of OPF in the muffin would have been more effective in promoting ROS release, which as stated above might regulate NFkB function. Nevertheless, together, these findings display a similar tendency in terms of cell viability and ROS content observed in cells treated with OPF extracts.
4. Conclusions
These results underscore the potential of utilizing the polyphenolic compounds and fibres found in OPF to improve the nutritional profile of GF products, particularly when combined with sourdough fermentation. The study conducted a comprehensive evaluation of the nutritional, structural, and volatile aromatic compounds present in these products. Consequently, this research lays the groundwork for exploring the potential advantages of orange by-products as a valuable source of bioactive compounds, which can be incorporated into the development of GF foods. From a nutritional perspective, OPF-enriched muffins fermented with sourdough exhibited increased antioxidant activity, reduced glycaemic index, and affected the volatile profile, influencing the viability of HCT8 cells. Additionally, OPF demonstrated a slight prebiotic effect. Overall, these findings suggest that incorporating OPF enhances the nutritional profiles of GF muffins and offers potential health benefits, highlighting its role as a functional ingredient.
Author contributions
Giusy Rita Caponio: formal analysis; data curation; investigation; writing – original draft preparation; Alessandro Annunziato: formal analysis; investigation; Mirco Vacca: formal analysis; data curation; writing – original draft preparation; Graziana Difonzo: formal analysis; data curation; investigation; writing – original draft preparation; Giuseppe Celano: formal analysis; data curation; conceptualization; writing – review & editing; Fabio Minervini: conceptualization; validation; writing – review & editing; Marianna Ranieri: formal analysis; data curation; investigation; Giovanna Valenti: writing – review & editing; Grazia Tamma: resources and funding acquisition; data curation; conceptualization; validation; writing – review & editing; Maria De Angelis: resources and funding acquisition; conceptualization; validation; writing – review & editing. All authors read and approved the final manuscript.
Data availability
The authors confirm that the data supporting the findings of this study are available within the article [and/or] its ESI.†
Conflicts of interest
There are no conflicts to declare.
Acknowledgements
This research was funded by project funded under the National Recovery and Resilience Plan (NRRP), Mission 4 Component 2 Investment 1.3 – Call for proposals no. 341 of 15 March 2022 of Italian Ministry of University and Research funded by the European Union – NextGenerationEU; award number: project code PE00000003, Concession Decree no. 1550 of 11 October 2022 adopted by the Italian Ministry of University and Research, CUP H93C22000630001, project title “ON Foods – Research and innovation network on food and nutrition Sustainability, Safety and Security – Working ON Foods”.
References
- M. Gobbetti, G. Rizzello, R. Di Cagno and M. De Angelis, Sourdough lactobacilli and celiac disease, Food Microbiol., 2007, 24, 187–196 CrossRef CAS PubMed.
- J. A. King, J. Jeong, F. E. Underwood, J. Quan, N. Panaccione, J. W. Windsor, S. Coward, J. deBruyn, P. E. Ronksley, A.-A. Shaheen, H. Quan, J. Godley, S. Veldhuyzen Van Zanten, B. Lebwohl, S. C. Ng, J. F. Ludvigsson and G. G. Kaplan, Incidence of Celiac Disease Is Increasing Over Time: A Systematic Review and Meta-analysis, Am. J. Gastroenterol., 2020, 115, 507–525 CrossRef PubMed.
- I. Marafini, G. Monteleone and C. Stolfi, Association Between Celiac Disease and Cancer, Int. J. Mol. Sci., 2020, 21, 4155 CrossRef CAS PubMed.
- Z. Šmídová and J. Rysová, Gluten-Free Bread and Bakery Products Technology, Foods, 2022, 11, 480 CrossRef PubMed.
- V. Melini and F. Melini, Gluten-Free Diet: Gaps and Needs for a Healthier Diet, Nutrients, 2019, 11, 170 CrossRef CAS PubMed.
-
M. Gobbetti and C. G. Rizzello, Biotecnologia dei prodotti lievitati da forno, Casa editrice ambrosiana CEA, Seconda Edizione, 2023 Search PubMed.
- M. Vacca, D. Pinto, A. Annunziato, A. Ressa, M. Calasso, E. Pontonio, G. Celano and M. De Angelis, Gluten-Free Bread Enriched with Artichoke Leaf Extract In Vitro Exerted Antioxidant and Anti-Inflammatory Properties, Antioxidants, 2023, 12, 845 CrossRef CAS PubMed.
- D. El Khoury, S. Balfour-Ducharme and I. J. Joye, A Review on the Gluten-Free Diet: Technological and Nutritional Challenges, Nutrients, 2018, 10, 1410 CrossRef PubMed.
- M. Di Cairano, F. Galgano, R. Tolve, M. C. Caruso and N. Condelli, Focus on gluten free biscuits: Ingredients and issues, Trends Food Sci. Technol., 2018, 81, 203–212 CrossRef CAS.
- D. Paduano, A. Cingolani, E. Tanda and P. Usai, Effect of Three Diets (Low-FODMAP, Gluten-free and Balanced) on Irritable Bowel Syndrome Symptoms and Health-Related Quality of Life, Nutrients, 2019, 11, 1566 CrossRef CAS PubMed.
- M. De Angelis, G. Garruti, F. Minervini, L. Bonfrate, P. Portincasa and M. Gobbetti, The Food-gut Human Axis: The Effects of Diet on Gut Microbiota and Metabolome, Curr. Med. Chem., 2019, 26, 3567–3583 CrossRef CAS PubMed.
- M. Rudrapal, S. J. Khairnar, J. Khan, A. B. Dukhyil, M. A. Ansari, M. N. Alomary, F. M. Alshabrmi, S. Palai, P. K. Deb and R. Devi, Dietary Polyphenols and Their Role in Oxidative Stress-Induced Human Diseases: Insights Into Protective Effects, Antioxidant Potentials and Mechanism(s) of Action, Front. Pharmacol., 2022, 13, 806470 CrossRef CAS PubMed.
- G. R. Caponio, T. Lippolis, V. Tutino, I. Gigante, V. De Nunzio, R. Milella, M. Gasparro and M. Notarnicola, Nutraceuticals: Focus on Anti-Inflammatory, Anti-Cancer, Antioxidant Properties in Gastrointestinal Tract, Antioxidants, 2022, 11, 1274 CrossRef CAS PubMed.
- L. Yi, S. Ma and D. Ren, Phytochemistry and bioactivity of Citrus flavonoids: a focus on antioxidant, anti-inflammatory, anticancer and cardiovascular protection activities, Phytochem. Rev., 2017, 16, 479–511 CrossRef CAS.
- I. Gigante, R. A. Milella, V. Tutino, G. Debiase, L. Notarangelo, M. A. Giannandrea, V. De Nunzio, A. Orlando, R. D'Alessandro, M. G. Caruso and M. Notarnicola, Autumn Royal and Egnatia Grape Extracts Differently Modulate Cell Proliferation in Human Colorectal Cancer Cells, Endocr. Metab. Immune Disord. Drug Targets, 2020, 20, 1740–1750 CrossRef CAS PubMed.
- T. Ohishi, S. Hayakawa and N. Miyoshi, Involvement of microRNA modifications in anticancer
effects of major polyphenols from green tea, coffee, wine, and curry, Crit. Rev. Food Sci. Nutr., 2023, 63, 7148–7179 CrossRef CAS PubMed.
- N. Latruffe, A. Lançon, R. Frazzi, V. Aires, D. Delmas, J. Michaille, F. Djouadi, J. Bastin and M. Cherkaoui-Malki, Exploring new ways of regulation by resveratrol involving miRNAs, with emphasis on inflammation, Ann. N. Y. Acad. Sci., 2015, 1348, 97–106 CrossRef CAS PubMed.
- T. A. Corrêa and M. M. Rogero, Polyphenols regulating microRNAs and inflammation biomarkers in obesity, Nutrition, 2019, 59, 150–157 CrossRef PubMed.
- L. A. De Castro, J. M. Lizi, E. G. L. Das Chagas, R. A. De Carvalho and F. M. Vanin, From Orange Juice By-Product in the Food Industry to a Functional Ingredient: Application in the Circular Economy, Foods, 2020, 9, 593 CrossRef CAS PubMed.
- N. O'Shea, A. Ktenioudaki, T. P. Smyth, P. McLoughlin, L. Doran, M. A. E. Auty, E. Arendt and E. Gallagher, Physicochemical assessment of two fruit by-products as functional ingredients: Apple and orange pomace, J. Food Eng., 2015, 153, 89–95 CrossRef.
- K. Rezzadori, S. Benedetti and E. R. Amante, Orange waste as raw material for new products, Food Bioprod. Process., 2012, 90, 606–614 CrossRef CAS.
- G. R. Caponio, F. Minervini, G. Tamma, G. Gambacorta and M. De Angelis, Promising Application of Grape Pomace and Its Agri-Food Valorization: Source of Bioactive Molecules with Beneficial Effects, Sustainability, 2023, 15, 9075 CrossRef CAS.
- P. Chavan, A. K. Singh and G. Kaur, Recent progress in the utilization of industrial waste and by–products of citrus fruits: A review, J. Food Process Eng., 2018, 41, e12895 CrossRef.
- T. De Moraes Crizel, A. Jablonski, A. De Oliveira Rios, R. Rech and S. H. Flôres, Dietary fiber from orange byproducts as a potential fat replacer, LWT – Food Sci. Technol., 2013, 53, 9–14 CrossRef CAS.
- P. R. Mary, S. Mutturi and M. Kapoor, Non-enzymatically hydrolyzed guar gum and orange peel fibre together stabilize the low-fat, set-type yogurt: A techno-functional study, Food Hydrocolloids, 2022, 122, 107100 CrossRef CAS.
- T. Erkaya-Kotan, In vitro angiotensin converting enzyme (ACE)-inhibitory and antioxidant activity of probiotic yogurt incorporated with orange fibre during storage, J. Food Sci. Technol., 2020, 57, 2343–2353 CrossRef CAS PubMed.
- G. Caponio, M. Noviello, F. Calabrese, G. Gambacorta, G. Giannelli and M. De Angelis, Effects of Grape Pomace Polyphenols and In Vitro Gastrointestinal Digestion on Antimicrobial Activity: Recovery of Bioactive Compounds, Antioxidants, 2022, 11, 567 CrossRef CAS PubMed.
- G. Caponio, M. Cofano, T. Lippolis, I. Gigante, V. De Nunzio, G. Difonzo, M. Noviello, L. Tarricone, G. Gambacorta, G. Giannelli, M. De Angelis and M. Notarnicola, Anti-Proliferative and Pro-Apoptotic Effects of Digested Aglianico Grape Pomace Extract in Human Colorectal Cancer Cells, Molecules, 2022, 27, 6791 CrossRef CAS PubMed.
- M. Troilo, G. Difonzo, V. M. Paradiso, A. Pasqualone and F. Caponio, Grape Pomace as Innovative Flour for the Formulation of Functional Muffins: How Particle Size Affects the Nutritional, Textural and Sensory Properties, Foods, 2022, 11, 1799 CrossRef CAS PubMed.
- A. Torreggiani, C. Demarinis, D. Pinto, A. Papale, G. Difonzo, F. Caponio, E. Pontonio, M. Verni and C. G. Rizzello, Up-Cycling Grape Pomace through Sourdough Fermentation: Characterization of Phenolic Compounds, Antioxidant Activity, and Anti-Inflammatory Potential, Antioxidants, 2023, 12, 1521 CrossRef CAS PubMed.
-
Official Methods of Analysis of AOAC International, ed. W. Horwitz, AOAC International, Arlington County, VA, USA, 2000.
- G. Tamma, M. Ranieri, A. Di Mise, M. Centrone, M. Svelto and G. Valenti, Glutathionylation of the Aquaporin-2
Water Channel, J. Biol. Chem., 2014, 289, 27807–27813 CrossRef CAS PubMed.
- G. Difonzo, G. De Gennaro, G. R. Caponio, M. Vacca, G. Dal Poggetto, I. Allegretta, B. Immirzi and A. Pasqualone, Inulin from Globe Artichoke Roots: A Promising Ingredient for the Production of Functional Fresh Pasta, Foods, 2022, 11, 3032 CrossRef CAS PubMed.
- G. R. Caponio, G. Difonzo, G. De Gennaro, M. Calasso, M. De Angelis and A. Pasqualone, Nutritional Improvement of Gluten-Free Breadsticks by Olive Cake Addition and Sourdough Fermentation: How Texture, Sensory, and Aromatic Profile Were Affected?, Front. Nutr., 2022, 9, 830932 CrossRef PubMed.
- S. Pérez-Burillo, S. Molino, B. Navajas-Porras, Á. J. Valverde-Moya, D. Hinojosa-Nogueira, A. López-Maldonado, S. Pastoriza and J. Á. Rufián-Henares, An in vitro batch fermentation protocol for studying the contribution of food to gut microbiota composition and functionality, Nat. Protoc., 2021, 16, 3186–3209 CrossRef PubMed.
- G. R. Caponio, R. Miolla, M. Vacca, G. Difonzo and M. De Angelis, Wine lees as functional ingredient to produce biscuits fortified with polyphenols and dietary fibre, LWT, 2024, 198, 115943 CrossRef CAS.
- L. A. D. Castro, J. M. Lizi, E. G. L. D. Chagas, R. A. D. Carvalho and F. M. Vanin, From Orange Juice By-Product in the Food Industry to a Functional Ingredient: Application in the Circular Economy, Foods, 2020, 9, 593 CrossRef PubMed.
- F. Figuerola, M. L. Hurtado, A. M. Estévez, I. Chiffelle and F. Asenjo, Fibre concentrates from apple pomace and citrus peel as potential fibre sources for food enrichment, Food Chem., 2005, 91, 395–401 CrossRef CAS.
- K. Sturm, D. Koron and F. Stampar, The composition of fruit of different strawberry varieties depending on maturity stage, Food Chem., 2003, 83, 417–422 CrossRef CAS.
-
E. S. H. Al-Janabi and S. S. Yasen, Determination of chemical composition and antioxidants of wheat flour, orange peel powder and manufactured biscuits, Cape Town, South Africa, 2023, p. 060006 Search PubMed.
- R. O. Obafaye and O. S. Omoba, Orange peel flour: A potential source of antioxidant and dietary fiber in pearl-millet biscuit, J. Food Biochem., 2018, 42, e12523 CrossRef.
- T. Ayora-Talavera, C. Ramos-Chan, A. Covarrubias-Cárdenas, A. Sánchez-Contreras, U. García-Cruz and N. Pacheco L., Evaluation of Pectin Extraction Conditions and Polyphenol Profile from Citrus x lantifolia Waste: Potential Application as Functional Ingredients, Agriculture, 2017, 7, 28 CrossRef.
- S. Toyokuni, K. Okamoto, J. Yodoi and H. Hiai, Persistent oxidative stress in cancer, FEBS Lett., 1995, 358, 1–3 CrossRef CAS PubMed.
-
E. G. Russell and T. G. Cotter, New Insight into the Role of Reactive Oxygen Species (ROS) in Cellular Signal-Transduction Processes, in International Review of Cell and Molecular Biology, Elsevier, 2015, vol. 319, pp. 221–254 Search PubMed.
- X. Wen, Z.-Q. Lin, B. Liu and Y.-Q. Wei, Caspase–mediated programmed cell death pathways as potential therapeutic targets in cancer, Cell Proliferation, 2012, 45, 217–224 CrossRef CAS PubMed.
- M. Zhou, X. Liu, Z. Li, Q. Huang, F. Li and C. Li, aspase–3 regulates the migration, invasion and metastasis of colon cancer cells, Int. J. Cancer, 2018, 143, 921–930 CrossRef CAS PubMed.
- J.-P. Pradère, C. Hernandez, C. Koppe, R. A. Friedman, T. Luedde and R. F. Schwabe, Negative regulation of NF-κB p65 activity by serine 536 phosphorylation, Sci. Signaling, 2016, 9, ra85 CrossRef PubMed.
- R. E. Bellas, M. J. FitzGerald, N. Fausto and G. E. Sonenshein, Inhibition of NF-kappa B activity induces apoptosis in murine hepatocytes, Am. J. Pathol., 1997, 151, 891–896 CAS.
- V. R. Baichwal and P. A. Baeuerle, Apoptosis: Activate NF-κB or die?, Curr. Biol., 1997, 7, R94–R96 CrossRef CAS PubMed.
- S. Conejeros-Lillo, F. Aguirre, D. Cabrera, F. Simon, L. Peñailillo and C. Cabello-Verrugio, Role of the ubiquitin-proteasome system in the sarcopenic-like phenotype induced by CCL5/RANTES, Eur. J. Transl. Myol., 2024, 34 DOI:10.4081/ejtm.2024.12249.
- S. Kumari, T.-M. Van, D. Preukschat, H. Schuenke, M. Basic, A. Bleich, U. Klein and M. Pasparakis, NF-κB inhibition in keratinocytes causes RIPK1-mediated necroptosis and skin inflammation, Life Sci. Alliance, 2021, 4, e202000956 CrossRef CAS PubMed.
- S. A. Joosse and K. Pantel, Genetic traits for hematogeneous tumor cell dissemination in cancer patients, Cancer Metastasis Rev., 2016, 35, 41–48 CrossRef CAS PubMed.
- K. Newton, A. Strasser, N. Kayagaki and V. M. Dixit, Cell death, Cell, 2024, 187, 235–256 CrossRef CAS PubMed.
- M. Wang, F. Yu, Y. Zhang and P. Li, Programmed cell death in tumor immunity: mechanistic insights and clinical implications, Front. Immunol., 2024, 14, 1309635 CrossRef PubMed.
- E. E. Gestl and S. A. Böttger, Cytoplasmic sequestration of the tumor suppressor p53 by a heat shock protein 70 family member, mortalin, in human colorectal adenocarcinoma cell lines, Biochem. Biophys. Res. Commun., 2012, 423, 411–416 CrossRef CAS PubMed.
- Y. Gong, Z. Fan, G. Luo, C. Yang, Q. Huang, K. Fan, H. Cheng, K. Jin, Q. Ni, X. Yu and C. Liu, The role of necroptosis in cancer biology and therapy, Mol. Cancer, 2019, 18, 100 CrossRef PubMed.
- G. Pistritto, D. Trisciuoglio, C. Ceci, A. Garufi and G. D'Orazi, Apoptosis as anticancer mechanism: function and dysfunction of its modulators and targeted therapeutic strategies, Aging, 2016, 8, 603–619 CrossRef CAS PubMed.
- M. B. Madureira, V. M. Concato, E. M. S. Cruz, J. M. Bitencourt De Morais, F. S. R. Inoue, N. Concimo Santos, M. D. Gonçalves, M. Cremer De Souza, T. Basso Scandolara, M. Fontana Mezoni, M. Galvani, F. Rodrigues Ferreira Seiva, C. Panis, M. M. Miranda-Sapla and W. R. Pavanelli, Naringenin and Hesperidin as Promising Alternatives for Prevention and Co-Adjuvant Therapy for Breast Cancer, Antioxidants, 2023, 12, 586 CrossRef CAS PubMed.
- A. Nikokavoura, D. Christodouleas, E. Yannakopoulou, K. Papadopoulos and A. C. Calokerinos, Evaluation of antioxidant activity of hydrophilic and lipophilic compounds in edible oils by a novel fluorimetric method, Talanta, 2011, 84, 874–880 CrossRef CAS PubMed.
- M. Yaqoob, P. Aggarwal, N. Rasool, W. N. Baba, P. Ahluwalia and R. Abdelrahman, Enhanced functional properties and shelf stability of cookies by fortification of kinnow derived phytochemicals and residues, Food Meas., 2021, 15, 2369–2376 CrossRef.
- V. Laganà, A. M. Giuffrè, A. De Bruno and M. Poiana, Formulation of Biscuits Fortified with a Flour Obtained from Bergamot By-Products (Citrus bergamia, Risso), Foods, 2022, 11, 1137 CrossRef PubMed.
- A. Krajewska and D. Dziki, Enrichment of Cookies with Fruits and Their By-Products: Chemical Composition, Antioxidant Properties, and Sensory Changes, Molecules, 2023, 28, 4005 CrossRef CAS PubMed.
- M. Palla, M. Blandino, A. Grassi, D. Giordano, C. Sgherri, M. F. Quartacci, A. Reyneri, M. Agnolucci and M. Giovannetti, Characterization and selection of functional yeast strains during sourdough fermentation of different cereal wholegrain flours, Sci. Rep., 2020, 10, 12856 CrossRef CAS PubMed.
- L. De Vuyst, H. Harth, S. Van Kerrebroeck and F. Leroy, Yeast diversity of sourdoughs and associated metabolic properties and functionalities, Int. J. Food Microbiol., 2016, 239, 26–34 CrossRef CAS PubMed.
- L. Cui, G. Yang, S. Lu, X. Zeng, J. He, Y. Guo, D. Pan and Z. Wu, Antioxidant peptides derived from hydrolyzed milk proteins by Lactobacillus strains: A BIOPEP-UWM database-based analysis, Food Res. Int., 2022, 156, 111339 CrossRef CAS PubMed.
- D. Restuccia, L. Esposito, U. G. Spizzirri, M. Martuscelli, P. Caputo, C. O. Rossi, M. L. Clodoveo, R. Pujia, E. Mazza, A. Pujia, T. Montalcini and F. Aiello, Formulation of A Gluten-Free Carob-Based Bakery Product: Evaluation of Glycemic Index, Antioxidant Activity, Rheological Properties, and Sensory Features, Fermentation, 2023, 9, 748 CrossRef CAS.
- H. Debelo, M. Li and M. G. Ferruzzi, Processing influences on food polyphenol profiles and biological activity, Curr. Opin. Food Sci., 2020, 32, 90–102 CrossRef.
- Y.-S. Zhao, A. S. Eweys, J.-Y. Zhang, Y. Zhu, J. Bai, O. M. Darwesh, H.-B. Zhang and X. Xiao, Fermentation Affects the Antioxidant Activity of Plant-Based Food Material through the Release and Production of Bioactive Components, Antioxidants, 2021, 10, 2004 CrossRef CAS PubMed.
- D. J. A. Jenkins, M. Dehghan, A. Mente, S. I. Bangdiwala, S. Rangarajan, K. Srichaikul, V. Mohan, A. Avezum, R. Díaz, A. Rosengren, F. Lanas, P. Lopez-Jaramillo, W. Li, A. Oguz, R. Khatib, P. Poirier, N. Mohammadifard, A. Pepe, K. F. Alhabib, J. Chifamba, A. H. Yusufali, R. Iqbal, K. Yeates, K. Yusoff, N. Ismail, K. Teo, S. Swaminathan, X. Liu, K. Zatońska, R. Yusuf and S. Yusuf, Glycemic Index, Glycemic Load, and Cardiovascular Disease and Mortality, N. Engl. J. Med., 2021, 384, 1312–1322 CrossRef CAS PubMed.
- G. Zuccotti, V. Fabiano, D. Dilillo, M. Picca, C. Cravidi and P. Brambilla, Intakes of nutrients in I talian children with celiac disease and the role of commercially available gluten–free products, J. Hum. Nutr. Diet., 2013, 26, 436–444 CrossRef CAS PubMed.
- C. Sabater, L. Ruiz, S. Delgado, P. Ruas-Madiedo and A. Margolles, Valorization of Vegetable Food Waste and By-Products Through Fermentation Processes, Front. Microbiol., 2020, 11, 581997 CrossRef PubMed.
- M. De Angelis, C. G. Rizzello, G. Alfonsi, P. Arnault, S. Cappelle, R. Di Cagno and M. Gobbetti, Use of sourdough lactobacilli and oat fibre to decrease the glycaemic index of white wheat bread, Br. J. Nutr., 2007, 98, 1196–1205 CrossRef CAS PubMed.
- M. R. Romero-Lopez, P. Osorio-Diaz, L. A. Bello-Perez, J. Tovar and A. Bernardino-Nicanor, Concentrate from Orange (Citrus sinensis L.) Bagase: Characterization and Application as Bakery Product Ingredient, Int. J. Mol. Sci., 2011, 12, 2174–2186 CrossRef CAS PubMed.
- K. Wojtunik-Kulesza, A. Oniszczuk, T. Oniszczuk, M. Combrzyński, D. Nowakowska and A. Matwijczuk, Influence of In Vitro Digestion on Composition, Bioaccessibility and Antioxidant Activity of Food Polyphenols—A Non-Systematic Review, Nutrients, 2020, 12, 1401 CrossRef CAS PubMed.
- P. Li, X. Yao, Q. Zhou, X. Meng, T. Zhou and Q. Gu, Citrus Peel Flavonoid Extracts: Health-Beneficial Bioactivities and Regulation of Intestinal Microecology in vitro, Front. Nutr., 2022, 9, 888745 CrossRef PubMed.
- A. Manthei, P. Elez-Martínez, R. Soliva-Fortuny and P. Murciano-Martínez, Prebiotic potential of pectin and cello-oligosaccharides from apple bagasse and orange peel produced by high-pressure homogenization and enzymatic hydrolysis, Food Chem., 2024, 435, 137583 CrossRef CAS PubMed.
- S. Saha, T. Do, J. Maycock, S. Wood and C. Boesch, Antibiofilm Efficacies of Flavonoid-Rich Sweet Orange Waste Extract against Dual-Species Biofilms, Pathogens, 2023, 12, 657 CrossRef CAS PubMed.
- T. Anwar, H. Qureshi, A. Fatima, K. Sattar, G. Albasher, A. Kamal, A. Ayaz and W. Zaman, Citrus sinensis Peel Oil Extraction and Evaluation as an Antibacterial and Antifungal Agent, Microorganisms, 2023, 11, 1662 CrossRef CAS PubMed.
- M. H. Baky, M. Elshahed, L. Wessjohann and M. A. Farag, Interactions between dietary flavonoids and the gut microbiome: a comprehensive review, Br. J. Nutr., 2022, 128, 577–591 CrossRef CAS PubMed.
- D. Plamada and D. C. Vodnar, Polyphenols—Gut Microbiota Interrelationship: A Transition to a New Generation of Prebiotics, Nutrients, 2021, 14, 137 CrossRef PubMed.
- C. Milani, S. Duranti, F. Bottacini, E. Casey, F. Turroni, J. Mahony, C. Belzer, S. Delgado Palacio, S. Arboleya Montes, L. Mancabelli, G. A. Lugli, J. M. Rodriguez, L. Bode, W. De Vos, M. Gueimonde, A. Margolles, D. Van Sinderen and M. Ventura, The First Microbial Colonizers of the Human Gut: Composition, Activities, and Health Implications of the Infant Gut Microbiota, Microbiol. Mol. Biol. Rev., 2017, 81 CrossRef PubMed , e00036-17.
- M. Vacca, G. Celano, F. M. Calabrese, P. Portincasa, M. Gobbetti and M. De Angelis, The Controversial Role of Human Gut Lachnospiraceae, Microorganisms, 2020, 8, 573 CrossRef CAS PubMed.
- V. Núñez-Gómez, M. Jesús Periago, J. Luis Ordóñez-Díaz, G. Pereira-Caro, J. Manuel Moreno-Rojas and R. González-Barrio, Dietary fibre fractions rich in (poly)phenols from orange by-products and their metabolisation by in vitro digestion and colonic fermentation, Food Res. Int., 2024, 177, 113718 CrossRef PubMed.
- F. P. Douillard and W. M. De Vos, Biotechnology of health-promoting bacteria, Biotechnol. Adv., 2019, 37, 107369 CrossRef CAS PubMed.
- J. Zhang, L. Song, Y. Wang, C. Liu, L. Zhang, S. Zhu, S. Liu and L. Duan, Beneficial effect of butyrate–producing Lachnospiraceae on stress–induced visceral hypersensitivity in rats, J. Gastroenterol. Hepatol., 2019, 34, 1368–1376 CrossRef CAS PubMed.
- A. R. Karnopp, A. M. Figueroa, P. R. Los, J. C. Teles, D. R. S. Simões, A. C. Barana, F. T. Kubiaki, J. G. B. D. Oliveira and D. Granato, Effects of whole-wheat flour and bordeaux grape pomace (Vitis labrusca L.) on the sensory, physicochemical and functional properties of cookies, Food Sci. Technol., 2015, 35, 750–756 CrossRef.
- J. Korus, L. Juszczak, M. Witczak and R. Ziobro, Effect of Citrus Fiber on the Rheological Properties of Dough and Quality of the Gluten-Free Bread, Appl. Sci., 2020, 10, 6633 CrossRef CAS.
- N. O'Shea, L. Doran, M. Auty, E. Arendt and E. Gallagher, The rheology, microstructure and sensory characteristics of a gluten-free bread formulation enhanced with orange pomace, Food Funct., 2013, 4, 1856 RSC.
- E. Campo, L. Del Arco, L. Urtasun, R. Oria and A. Ferrer-Mairal, Impact of sourdough on sensory properties and consumers’ preference of gluten-free breads enriched with teff flour, J. Cereal Sci., 2016, 67, 75–82 CrossRef.
- C. G. Rizzello, A. Lorusso, M. Montemurro and M. Gobbetti, Use of sourdough made with quinoa (Chenopodium quinoa) flour and autochthonous selected lactic acid bacteria for enhancing the nutritional, textural and sensory features of white bread, Food Microbiol., 2016, 56, 1–13 CrossRef CAS PubMed.
- D. Novotni, N. Čukelj, B. Smerdel and D. Ćurić, Quality attributes and firming kinetics of partially baked frozen wholewheat
bread with sourdough, Int. J. Food Sci. Technol., 2013, 48, 2133–2142 CrossRef CAS.
- C. Dingeo, G. Difonzo, V. M. Paradiso, C. G. Rizzello and E. Pontonio, Teff Type-I Sourdough to Produce Gluten-Free Muffin, Microorganisms, 2020, 8, 1149 CrossRef CAS PubMed.
- K. Kaseleht, T. Paalme, A. Mihhalevski and I. Sarand, Analysis of volatile compounds produced by different species of lactobacilli in rye sourdough using multiple headspace extraction, Int. J. Food Sci. Technol., 2011, 46, 1940–1946 CrossRef CAS.
- S. Lee, Y. Hwang, M. Kim, M. Chung and Y.-S. Kim, Comparison of Volatile and Nonvolatile Compounds in Rice Fermented by Different Lactic Acid Bacteria, Molecules, 2019, 24, 1183 CrossRef PubMed.
- L. W. Zheng, H. Chung and Y.-S. Kim, Effects of dicarbonyl trapping agents, antioxidants, and reducing agents on the formation of furan and other volatile components in canned-coffee model systems, Food Res. Int., 2015, 75, 328–336 CrossRef CAS PubMed.
- G. De Gennaro, G. Difonzo, C. Summo, A. Pasqualone and F. Caponio, Olive Cake Powder as Functional Ingredient to Improve the Quality of Gluten-Free Breadsticks, Foods, 2022, 11, 552 CrossRef CAS PubMed.
- Â. Galvan-Lima, S. C. Cunha, Z. E. Martins, A. G. Soares, I. M. P. L. V. O. Ferreira and A. Farah, Headspace volatolome of peel flours from citrus fruits grown in Brazil, Food Res. Int., 2021, 150, 110801 CrossRef PubMed.
|
This journal is © The Royal Society of Chemistry 2024 |