DOI:
10.1039/D3FO04904C
(Paper)
Food Funct., 2024,
15, 3692-3708
Protective effect of Pediococcus pentosaceus Li05 on diarrhea-predominant irritable bowel syndrome in rats†
Received
8th November 2023
, Accepted 1st March 2024
First published on 4th March 2024
Abstract
Pediococcus pentosaceus Li05 (Li05) has demonstrated potential benefits in various intestinal and liver diseases, but its potential and mechanisms in relieving diarrhea have not been understood. The objective of this research was to examine the effects and mechanisms of Li05 in rats with diarrhea-predominant irritable bowel syndrome (IBS-D) induced by wrap restrain stress (WRS) and 4% acetic acid. The results demonstrated that Li05 effectively alleviated weight loss, visceral sensitivity and diarrhea in rats with IBS-D. It also improved intestinal and systemic inflammation by reducing the levels of chemokines and proinflammatory cytokines (GRO/KC, RANTES, IL-1β, IL-7, and IL-18). The 5-hydroxytryptamine (5-HT) signaling pathway is involved in regulating excessive intestinal motility and secretion in IBS-D. Li05 effectively reduced the expression levels of the 5-HT3B receptor (5-HT3BR) (p < 0.01) in the intestine. Additionally, Li05 intervention had a regulatory effect on the gut composition, with a decrease in the abundance of [Ruminococcus] gauvreauii group, Dubosiella, Erysipelatoclostridium and Blautia, and an increase in the abundance of Alloprevotella, Anaerotruncus and Mucispirillum. Furthermore, Li05 induced significant changes in fatty acid and amino acid metabolism in the gut of rats with IBS-D. These findings indicate that Li05 exhibits an effective improvement in IBS-D symptoms by reducing inflammation and modulating gut microbiota and metabolism. Based on the above results, Li05 holds promise as a potential probiotic for managing IBS-D.
1. Introduction
Affecting 7%–21% of the general population, irritable bowel syndrome (IBS) is a prevalent functional gastrointestinal disorder characterized by recurrent abdominal pain, spasms and bloating, accompanied by changes in bowel habits.1,2 The most common subtype of IBS is diarrhea-predominant irritable bowel syndrome (IBS-D), with its prevalence gradually increasing.3 Due to its recurrent and chronic nature, it significantly affects the social and overall quality of life of patients, resulting in substantial medical and societal burdens.2 The pathophysiological mechanisms of IBS-D have not been fully elucidated, but they mainly involve visceral hypersensitivity, small intestinal bacterial overgrowth, alterations in gastrointestinal motility, environmental factors (such as dietary habits), and disruptions in the gut microbiota.4,5 Traditional treatments (such as gastrointestinal motility regulators and antidiarrheal drugs) usually target specific mechanisms and may not be effective for all IBS-D patients, potentially causing some side effects.6
Increasing research indicates that IBS patients exhibit low-grade mucosal inflammation, elevated concentrations of proinflammatory cytokines, and an increased presence of mast cells in their colonic mucosa. Clinical studies have also found differences in the levels of cytokines (including IL-6 and TNF-α) and chemokines (such as CXCL9, CXCL10 and MCP-1) between IBS patients and healthy individuals.7 Furthermore, the severity of inflammation is associated with the severity of visceral hypersensitivity, and high concentrations of cytokines in IBS-D patients are also associated with anxiety and depression, which may be potential mechanisms underlying the development of IBS-D symptoms.8 In addition, enterochromaffin cells are primarily responsible for secreting 5-hydroxytryptamine (5-HT; serotonin), a vital paracrine and neuroendocrine signaling molecule that plays a crucial role in regulating gastrointestinal motility, inflammation and vasodilation in the gut.9,10 Research suggests that a significant correlation exists between the severity of symptomatic patients with IBS-D and the serum level of 5-HT.11 The 5-HT3 receptor (5-HT3R), an important excitatory receptor for 5-HT, may act as an accessory factor in the hypersensitive sensory component of the gastrointestinal response observed in IBS.12 Furthermore, the gut microbiota plays a role in regulating the levels of 5-HT, but the specific bacteria involved in regulating 5-HT and their molecular mechanisms are not yet clear.13
Bacteria in the intestines play a multifaceted and advantageous role in preserving the overall health and equilibrium of the host ecosystem, as well as supporting normal physiological functions.14 Mounting evidence suggest that gut microbiota plays a significant role in the development and progression of IBS-D.15 Research has indicated that individuals with IBS-D exhibit a decrease in the richness of gut microbiota, specifically a notable decrease in Firmicutes and an increase in Bacteroidetes when compared to healthy individuals.16 However, other studies have shown an elevated ratio of Firmicutes to Bacteroidetes in IBS patients.17 The reasons for the inconsistent results have not been elucidated. In addition, meta-analyses have shown that probiotics and fecal microbiota transplantation, as potential effective treatments, can alleviate IBS symptoms.18,19 However, it is still unclear which specific probiotic strains are beneficial for IBS-D, and the exact pathogenic mechanism of gut microbiota in IBS-D is still unknown. Pediococcus pentosaceus Li05 (Li05) has been identified as a potential probiotic strain with lipid-lowering, antioxidant, and anti-inflammatory effects.20,21 Our previous research has found that Li05, which can relieve Clostridium difficile infection and DSS-induced colitis, has a protective effect on the intestinal barrier and it promotes gut microbiota restoration.21,22 Due to the beneficial effects of Li05 on intestinal illness, it can be inferred and hypothesized that this particular probiotic strain may hold potential as an effective treatment for IBS-D.
This study involved an IBS-D rat model through the implementation of a combination of wrap restrain stress (WRS) and 4% acetic acid induction. The impact of Li05 and its potential mechanisms on IBS-D were investigated through assessments of diarrhea-related parameters, histopathology, serum indicators, 5-HT signaling pathway, gut microbiota and metabolism.
2. Materials and methods
2.1 Strain and culture conditions
Pediococcus pentosaceus Li05 (CGMCC 7049) was isolated from healthy human feces in our laboratory.20 As previously described, Li05 was cultured in Man–Rogosa–Sharpe (MRS) broth (Oxoid, Thermo Fisher Biochemicals) under anaerobic conditions at 37 °C for 24 hours.23 After centrifugation at 6000g for 15 minutes, the pellet was washed twice and resuspended in PBS (1 × 109 CFU ml−1) for oral gavage.
2.2 Animals
All animal experiments were performed following the guidelines outlined in the National Institutes of Health Guide for the Care and Use of Laboratory Animals and were approved by the Animal Ethics Committee of the First Affiliated Hospital, School of Medicine, Zhejiang University (approval no. 2022-1624). Male Wistar rats (4 weeks old) that were specific pathogen free (SPF) were procured from GemPharmatech Company (Jiangsu, China). All rats were housed under a 12/12-hour dark/light cycle and were provided with ad libitum access to food and water.
2.3 Experimental design and establishment of animal models
As depicted in Fig. 1, after adaptation for one week, 24 rats were randomly assigned into three groups (n = 8 per group): control group (Ctrl), IBS-D model group (IBS-D), and IBS-D treated with Li05 group (Li05). Firstly, rats in the IBS-D and Li05 groups underwent WRS. The rats were confined in a restraint bag with openings in the head section for adequate air circulation, and their tails were secured to the bag with a rubber band, immobilizing their limbs and preventing body movement while allowing normal respiration and defecation. WRS was performed daily from 10 am to 12 pm for a continuous period of 21 days. Apart from WRS, on the 15th day, the rats (IBS-D group and Li05 group) were mildly anesthetized with ether inhalation, and 1 ml of 4% acetic acid was infused into the colon at a distance of 8 cm from the anus, followed by a rinse with 1 ml of PBS after a 1 minute interval.24 The rats in the Ctrl group did not undergo WRS and were infused with 1 ml of PBS using the same method as above on the 15th day. From the 8th to the 21st day, the rats in the Ctrl and IBS-D groups were administered a daily gavage of 1 ml of PBS, while the rats in the Li05 group received 1 ml Li05 solution (1 × 109 CFU ml−1). The rats’ body weight was recorded daily. On the 21st day, fecal samples were collected for diarrhea assessment and abdominal withdrawal reflex (AWR) was assessed. All rats were sacrificed on the 22nd day, and samples of blood, colon tissue, and colon contents were collected for further analysis.
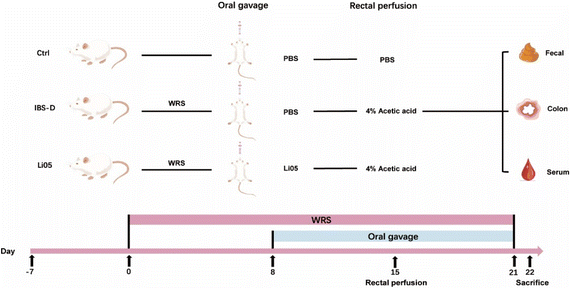 |
| Fig. 1 Flow chart of the animal experiments. The experiment was divided into three groups: control group (Ctrl), diarrhea-predominant irritable bowel syndrome group (IBS-D), and Pediococcus pentosaceus Li05 intervention group (Li05). The IBS-D group and Li05 group were subjected to wrap restrain stress (WRS) from Day 0 to Day 21, while the Ctrl group was not subjected to stress. On the 15th day, the IBS-D group and Li05 group were administered with 4% acetic acid rectally once, while the Ctrl group was administered with PBS. WRS combined with 4% acetic acid rectal administration was used to construct the IBS-D model. From the 8th day to the 21st day, all groups were subjected to gavage for 21 consecutive days, with the Ctrl group and IBS-D group receiving PBS, and the Li05 group receiving Li05. On the 22nd day, all rats were sacrificed and feces, colon, and serum were collected for subsequent experimental analysis. | |
2.4 Assessment of diarrhea
The severity of diarrhea in rats was assessed using four indicators: water content of feces, diarrhea rate, diarrhea index, and loose stool rate. Rat feces collected within a 2-hour period were weighed to measure the wet weight. After drying in an 80 °C drying oven for 48 hours, the dried feces were weighed as the dry weight. Each rat's fecal consistency was recorded, and the fecal consistency was classified according to the following criteria: Grade 1: cylindrical; Grade 2: loose, formed; Grade 3: sticky, unformed, high water content; Grade 4: liquid, unformed, watery stool. Grades 3 and 4 were considered as diarrhea. The calculation formulas for the four indicators are described in detail in the ESI.†
25,26
2.5 Assessment of visceral sensitivity
Abdominal withdrawal reflex (AWR) assessment is widely used to assess visceral sensitivity. A disposable silicone balloon catheter (6Fr, Bain Medical Device Co., Ltd, Suzhou, China) with a diameter of 2 mm was used for AWR evaluation, with a maximum inflation volume of 1.0 ml. After mild ether anesthesia, the balloon was carefully inserted into the rectum by 2 cm, and the catheter was fastened to the rat's tail to prevent displacement. After a half-hour period for the rat to awaken and acclimate, gradually increasing balloon pressure (from 0.2 ml to 1.0 ml, with increments of 0.2 ml each) was applied for 30 seconds, with a 1 minute interval (to prevent colonic ischemia) before reapplying the pressure. Two observers conducted the scoring assessment independently, and the AWR score was calculated as the average value. The specific scoring criteria for AWR are described in the ESI.†
27
2.6 Histological analysis and immunohistochemistry staining
Samples of distal colon tissue (1 cm) obtained from the rat were preserved in 10% neutral formalin solution for fixation (Solarbio, Beijing, China) for 24 hours. And then, samples were embedded in paraffin, sectioned into 2 μm thickness, and subjected to hematoxylin and eosin (H&E) staining for observation and scanning under a microscope.
To further conduct immunohistochemical analysis, the paraffin-embedded tissue sections were deparaffinized and rehydrated before subjecting them to antigen retrieval using 0.01 M citric acid buffer. Endogenous enzyme activity was inactivated, and blocking was performed using goat serum. The sections were then incubated with mouse serotonin monoclonal antibody (1
:
40; Thermo Fisher Scientific Inc., USA) in a humid chamber overnight at 4 °C. After washing with distilled water, the sections were incubated with horseradish peroxidase-conjugated secondary antibody (1
:
1000; Abcam, UK) at room temperature for 10 minutes. Following that, the sections were stained with diaminobenzidine tetrahydrochloride solution (DAB kit, Abcam) for 10 minutes. They were then washed, stained with hematoxylin, and observed and scanned under a microscope.
2.7 Serum cytokine and chemokines analysis
Serum cytokines were detected using the Bio-Plex Pro Rat Cytokine 23-Plex Assay kit (Bio-Rad, Hercules, CA, USA) according to the manufacturer's protocol.
2.8 ELISA
The collected blood samples were centrifuged at 3000g for 15 minutes at 4 °C to obtain the serum. The levels of 5-HT in the serum samples were quantified using the Serotonin/5-Hydroxytryptamine ELISA kit (Sangon Biotech, Shanghai, China) following the protocol provided by the manufacturer.
2.9 Real time quantitative PCR (RT-qPCR) analysis
The RNeasy Plus Mini Kit (Qiagen, CA, USA) was used to extract RNA from the rat colon tissue, following the manufacturer's protocol. The purity and concentration of the extracted RNA were measured using a NanoDrop 2000 spectrophotometer (Thermo Fisher Scientific Inc., USA). Following quantification, the RNA was then reverse transcribed into cDNA using the PrimeScript RT reagent kit (Takara Bio Inc., Shiga, Japan). To detect mRNA expression, the SYBR Premix Ex Taq II (Tli RNaseH Plus) reagent (Takara Biomedicals, Kusatsu, Japan) and the ViiA7 real-time fluorescence quantitative PCR system (Applied Biosystems) were utilized. The housekeeping gene β-actin was used as the internal reference gene, and all results were analyzed using the 2−ΔΔCT method. The primer sequences can be found in Table S1.†
2.10 16S rRNA sequencing
Immediately after collection, the colon contents were rapidly frozen and stored at −80 °C. Genomic DNA extraction was performed using the MagPure Soil DNA KF Kit (Magen) following the manufacturer's protocol. The extracted DNA was utilized as a template to amplify the V3–V4 regions of the bacterial gene using the barcoded universal PCR primers 343F (5′-TACGGRAGGCAGCAG-3′) and 798R (5′-AGGGTATCTAATCCT-3′). Subsequently, sequencing was performed using the Illumina NovaSeq 6000 platform, generating 250 bp paired-end reads.
The raw data were processed using QIIME 2 (2020.11) with default parameters for quality filtering, denoising, merging, and chimera removal, resulting in abundance tables for ASV and representative sequences. Using the QIIME 2 package, representative sequences were selected for each ASV and annotated against the Silva (v 138) database. The q2-feature-classifier software with default parameters was utilized to analyze species annotation. For estimating diversity, an unweighted Unifrac distance matrix was computed using R (v 3.5.1), followed by an unweighted Unifrac Principal coordinate analysis (PCoA). ANOVA statistical analysis was used to analyze the significant differences. The linear discriminant analysis effect size (LEfSe) method was employed to compare the taxonomy abundance spectrum, and linear discriminant analysis (LDA) scores were used to estimate the analysis results and quantify the effect size of each different classification unit.
The raw data were uploaded to the Sequence Read Archive database with the BioProject number PRJNA1081647.
2.11 Untargeted metabolomics
Collected colon content samples (60 mg) were placed in a 1.5 ml centrifuge tube, and pre-chilled steel beads and 600 μl of 80% methanol were added. The tube was then placed in a grinder and ground (60 Hz, 2 min). The sample was extracted by ultrasound in an ice-water bath for 10 minutes, followed by centrifugation for 10 minutes (12
000 rpm, 4 °C) after being placed at −40 °C overnight. The supernatant (150 μl) was filtered through a 0.22 μm organic phase syringe filter for detection. The derivatized samples were analyzed using both an Agilent 7890B gas chromatography system coupled with an Agilent 5977A MSD system (Agilent Technologies Inc., CA, USA) and an ACQUITY UPLC I-Class plus (Waters Corporation, Milford, USA) fitted with a Q-Exactive mass spectrometer. Progenesis QI V2.3 (Nonlinear, Dynamics, Newcastle, UK) software was used to process the LC-MS raw data, while Analysis Base File Converter was used to process the GC/MS raw data. Principal component analysis (PCA) was performed using R, while orthogonal partial least-squares-discriminant analysis (OPLS-DA) and partial least-squares-discriminant analysis (PLS-DA) were utilized to distinguish metabolites that differed between groups. Metabolites with p < 0.05 and VIP > 1 were regarded as statistically significant.
2.12 Correlation analysis
In order to analyze the correlation between gut microbiota and metabolites, we first separately filtered the microbiota and metabolite data. We selected the top 100 relationships between microbiota at the genus level and metabolites with the largest absolute values of correlation coefficients, satisfying the condition of significance, to plot the correlation network graph. If there were less than 100 relationships, we included all of them. The Pearson correlation analysis method was used to analyze the correlation. Furthermore, the Pearson correlation analysis was also used to analyze multiple correlation analyses of IBS-D treated with Li05, including inflammatory indicators, diarrhea-related metabolites, gut microbiota at the genus level and metabolites. We used R (v 3.5.1) to analyze and plot the correlation graph.
2.13 Data analysis
The data are presented as mean ± standard error. Statistical analysis was performed using SPSS 25.0 (IBM Corp., Armonk, NY, USA). For normally distributed data, one-way ANOVA was employed, while Kruskal–Wallis test was used for non-normally distributed data, with a p-value <0.05 considered statistically significant. Figures were prepared by using R and GraphPad Prism 9.0 (GraphPad Software Inc., San Diego, CA, USA).
3. Results and discussion
3.1 Li05 alleviated diarrhea and visceral hypersensitivity in IBS-D rats
IBS-D is a multifactorial functional bowel disease, and studies have shown that WRS in the 4% acetic acid-induced model has similar effects on the brain–gut axis, cytokine responses, and gut microbiota changes to IBS-D.24 Therefore, we chose restraint stress with the 4% acetic acid method to construct the IBS-D model. As shown in Fig. 2A, both the IBS-D and the Li05 group exhibited significantly slower weight gain after restraint stress compared to the Ctrl group. Starting from day 3, both the IBS-D group (113.01 ± 1.58 g) and the Li05 group (116.89 ± 1.60 g) exhibited significantly lower body weights compared to the Ctrl group (126.98 ± 2.46 g) (p ≤ 0.001 for both). On the second day (16th day of the experiment) after the administration of 4% acetic acid, the body weight significantly decreased due to severe diarrhea. After 3 days of Li05 administration, the body weight of the Li05 group was found to be higher than that of the IBS-D group (p = 0.023). The same phenomenon was observed on 14 days after Li05 gavage (Li05 group: 180.93 ± 4.99 g vs. IBS-D group: 161.15 ± 3.33 g, p = 0.002), but it was still lower than that of the Ctrl group.
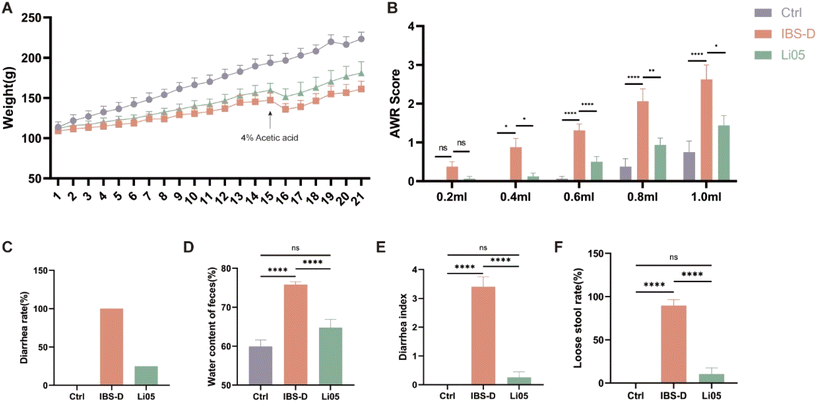 |
| Fig. 2
Pediococcus pentosaceus Li05 (Li05) improves weight loss, visceral sensitivity, and diarrhea in IBS-D rats. (A) Changes in the body weight of each group. (B) Abdominal withdrawal reflex (AWR) scores under different pressures. (C) Diarrhea rate in each group. (D) Fecal water content in each group. (E) Diarrhea index in each group. (F) Loose stool rate in each group. Data are presented as mean ± SEM, n = 8. Significant differences are denoted by “ns” (p ≥ 0.05), * (p < 0.05), ** (p < 0.01), *** (p < 0.001), and **** (p < 0.0001). | |
Stressful life events can lead to various mental and gastrointestinal disorders, including IBS-D. Rat models such as restraint stress and maternal separation can cause visceral hypersensitivity, anxiety-like behavior, and abnormal intestinal motility and diarrhea, which are among the pathological and physiological mechanisms of IBS-D.28,29 AWR is a reliable indicator for assessing visceral sensitivity in IBS-D.30 Our results revealed that there was no statistically significant difference observed among the three groups at a pressure of 0.2 ml (p > 0.05 for all pairwise comparisons). As the applied pressure increased, the AWR scores in the IBS-D group were significantly higher than that in the Ctrl group, while the AWR scores in the Li05 group exhibited a significant decrease compared the IBS-D group (p < 0.05) (Fig. 2B). If feasible, it is recommended to utilize electromyography for the evaluation visceral sensitivity, as it offers a more objective measure compared to AWR, despite the widespread use of the latter.
Diarrhea is the main clinical symptom of IBS-D, resulting in weight loss and severely impacting quality of life of patients,31 so alleviating diarrhea is the primary goal in treating IBS-D. The diarrhea rates in the Ctrl, IBS-D, and Li05 groups were 0%, 100%, and 25%, respectively (Fig. 2C). The fecal water content was significantly higher in the IBS-D group compared to the Ctrl group (75.82% ± 0.73% vs. 59.95% ± 1.65%, p < 0.001), and significantly lower in the Li05 group compared to the IBS-D group (64.79% ± 2.12% vs. 75.82% ± 0.73%, p = 0.003) (Fig. 2D). Additionally, the Li05 group had a significantly lower diarrhea index and loose stool rate compared to the IBS-D group (p < 0.001 for both) (Fig. 2E and F).
These results suggested that the combination of WRS and 4% acetic acid was successful in establishing the IBS-D model, and the probiotic Li05 can improve weight loss, diarrhea, and visceral sensitivity, effectively alleviating the symptoms of IBS-D.
3.2 Li05 reduced systemic and colonic inflammation in IBS-D rats
Studies have suggested that IBS patients have low-grade intestinal inflammation.32 To investigate the effects of Li05 on colonic and systemic inflammation in IBS-D, we performed colonic H&E staining and measured the cytokine levels in serum and the mRNA expression of cytokines in the colonic tissue. As shown in Fig. 3A, the colonic H&E staining demonstrated significant lymphoid hyperplasia and inflammatory cell infiltration in the IBS-D group, whereas the Li05 group exhibited mild inflammation that did not show a significant difference compared to the Ctrl group. Serum cytokine analysis in rats indicated that proinflammatory cytokines and chemokines (IL-1β, IL-6, IL-7, IL-18, GRO/KC and RANTES) were elevated in the IBS-D group compared to the Ctrl group (p < 0.05 for all). In the Li05 group, there was a significant decrease in proinflammatory cytokines IL-1β, IL-7 and IL-18, as well as chemokines GRO/KC and RANTES compared to the IBS-D group (Fig. 3B). Additionally, similar changes were observed in the colonic tissue. The mRNA expression levels of proinflammatory cytokines IL-6 (p = 0.004), IL-7 (p = 0.091), and IL-18 (p = 0.001), as well as chemokines GRO/KC (p = 0.006) and RANTES (p = 0.146), were elevated in the IBS-D group compared to the Ctrl group, while those in the Li05 group were decreased compared to the IBS-D group, with statistically significant differences observed for IL-7 (p = 0.003) and IL-18 (p < 0.001) (Fig. 3C).
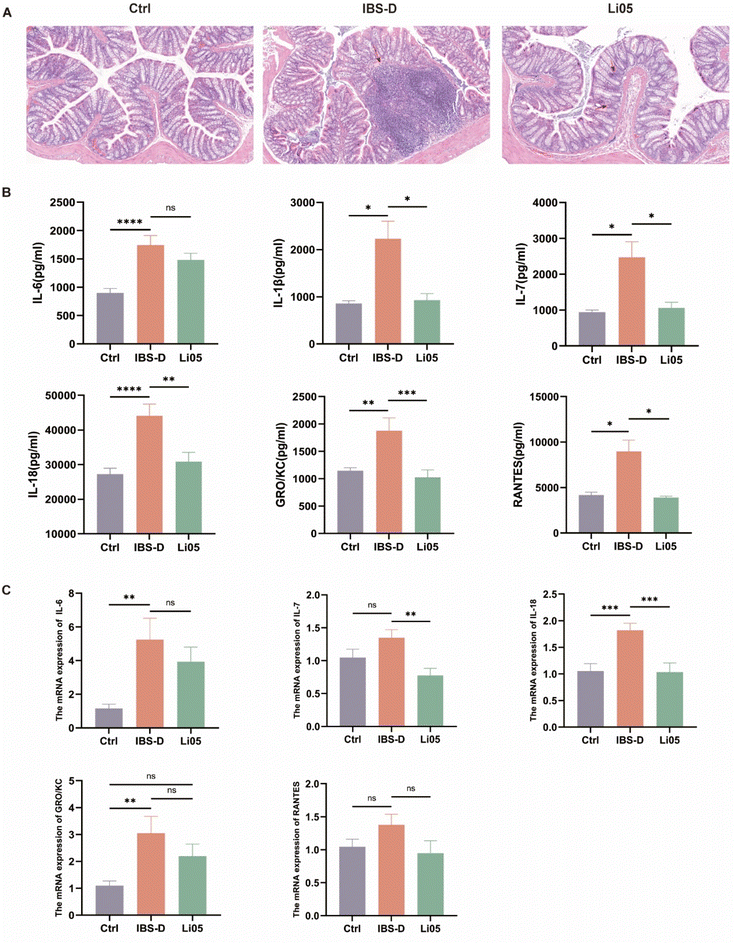 |
| Fig. 3 Li05 improves colonic and systemic inflammation in IBS-D rats. (A) Histopathological images of colonic tissues in each group of rats stained with H&E. The red arrows indicate areas of inflammatory cell infiltration (scale bar, 100 μm). (B) Expression levels of serum inflammatory cytokines (IL-1β, IL-6, IL-7, and IL-18) and chemokines (GRO/KC and RANTES). (C) mRNA expression levels of inflammatory cytokines (IL-6, IL-7, and IL-18) and chemokines (GRO/KC and RANTES). Data are shown as mean ± SEM, n = 8. Significant differences are denoted by “ns” (p ≥ 0.05), * (p < 0.05), ** (p < 0.01), *** (p < 0.001), and **** (p < 0.0001). | |
Low-grade mucosal inflammation and immune activation are the pathogenic mechanisms of IBS-D, and are closely related to clinical symptoms such as diarrhea, abdominal pain, anxiety, and depression, and may even serve as potential indicators for the diagnosis of IBS-D.33 In IBS patients, especially in IBS-D, proinflammatory cytokines are significantly increased in the peripheral blood, and high levels of cytokines are associated with anxiety and depression symptoms in IBS-D.34 Furthermore, the analysis of fecal supernatants from IBS-D patients suggests elevated levels of inflammatory cytokines IL-1β, IL-6, and TNF-α, and the concentrations of these inflammatory cytokines are correlated with pain frequency and severity.35 Therefore, alleviating mucosal and systemic inflammation is a crucial strategy in managing IBS-D. Our study demonstrated that Li05 intervention can significantly alleviate the colonic mucosal inflammatory infiltration and reduce the elevated levels of systemic and colonic inflammatory cytokines in IBS-D. These results indicate that Li05 can alleviate inflammation, which may lead to an improvement in clinical symptoms such as diarrhea, abdominal pain, anxiety, and depression.
3.3 Li05 alleviated diarrhea by modulating the 5-HT signaling pathway in IBS-D rats
5-HT, a critical gastrointestinal signaling molecule, is primarily synthesized and secreted by enterochromaffin cells, acting as a crucial neurotransmitter regulating the intestinal motility reflex and secretion reflex.36 We observed intestinal enterochromaffin cells through serotonin immunohistochemistry staining. As shown in Fig. 4A, the IBS-D group exhibited a significant increase in enterochromaffin cells compared to the Ctrl group. However, intervention with Li05 led to a notable decrease in enterochromaffin cell count, slightly more than that observed in the Ctrl group. To further examine the alterations in 5-HT levels in IBS-D rats, we measured serum 5-HT levels. As shown in Fig. 4B, the IBS-D group exhibited elevated serum 5-HT levels compared to the Ctrl group (1766.56 ± 6.83 vs. 1688.16 ± 35.43, p = 0.02), and the average 5-HT level in the Li05 group was lower than that in the IBS-D group, albeit the difference did not reach statistical significance. Thus, our data demonstrated that Li05 intervention partially alleviated the significantly elevated levels of enterochromaffin cells and serum 5-HT observed in IBS-D rats.
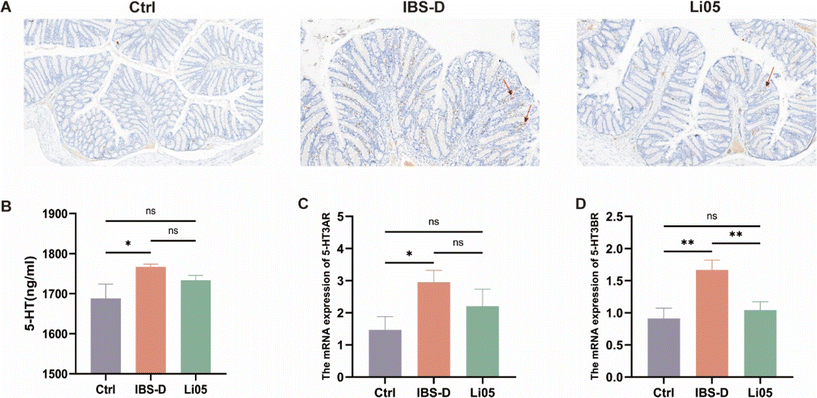 |
| Fig. 4 Li05 alleviates diarrhea in IBS-D rats by regulating the 5-hydroxytryptamine (5-HT) signaling pathway. (A) Immunohistochemical staining of colonic enterochromaffin cells in the Ctrl, IBS-D, and Li05 groups, with the brown staining indicating positive enterochromaffin cells (red arrows) (scale bar, 100 μm). (B) Serum 5-HT levels in each group. (C) mRNA expression levels of intestinal 5-HT3AR in each group. (D) mRNA expression levels of intestinal 5-HT3BR in each group. Data are shown as mean ± SEM, n = 8. Significant differences are denoted by “ns” (p ≥ 0.05), * (p < 0.05), ** (p < 0.01). | |
To further determine whether Li05 could alleviate diarrhea through the 5-HT signaling pathway, we examined the mRNA expression of 5-HT receptors. 5-HT can regulate intestinal motility and secretion through the activation of various 5-HT receptors. 5-HT3R is an important excitatory receptor for 5-HT, primarily activated in the myenteric intrinsic primary afferent neurons (IPANs), which are associated with giant migrating contractions in the gut.37,38 Currently, 5-HT3R antagonists, such as alosetron, are used to treat IBS-D.39 We conducted RT-qPCR analysis to examine the mRNA expression levels of two subtypes of 5-HT3R, namely 5-HT3AR and 5-HT3BR, in colonic tissues. We found that the expression of both 5-HT3AR and 5-HT3BR was notably elevated in the IBS-D group compared to the Ctrl group (p = 0.028 and p = 0.002, respectively). Nevertheless, the Li05 group exhibited decreased expression of 5-HT3AR and 5-HT3BR compared to the IBS-D group (p = 0.248 and p = 0.006, respectively) (Fig. 4C and D). These findings indicate that Li05 intervention leads to a downregulation in the mRNA expression of 5-HT3AR and 5-HT3BR in IBS-D, which subsequently reduces excessive intestinal motility and secretion, ultimately alleviating diarrhea symptoms.
Based on our research findings, Li05 intervention results in a decrease in the population of enterochromaffin cells in the intestine, as well as a reduction in the levels of 5-HT and the mRNA expression of 5-HT3R. By modulating 5-HT signaling pathway, Li05 demonstrates the ability to alleviate diarrhea in IBS-D rats.
3.4 Li05 altered the gut microbiota in IBS-D rats
The gut microbiota, as an organ with specific functions, plays a vital role in the pathophysiology of IBS.40 Several studies have identified disparities in the gut microbiota between healthy individuals and IBS-D patients, suggesting that the gut microbiota is disturbed in IBS-D.41 However, there is currently no consensus regarding the specific microbial features of IBS-D, and further research is needed. Identifying and screening new bacteria associated with IBS-D can contribute to the development of better probiotic treatment strategies. Therefore, we analyzed the changes in the gut microbiota after Li05 intervention in IBS-D rats.
We first conducted a principal coordinate analysis (PCoA) to analyze the β-diversity of the microbial community, which showed significant disparities in microbial composition between the IBS-D group and both the Ctrl and Li05 groups (p = 0.002) (Fig. 5A). At the phylum level (Fig. 5B), the abundance of Deferribacteraceae was significantly lower in IBS-D than in the Ctrl group (p < 0.001). In contrast, the Li05 group exhibited a significantly higher abundance of Deferribacteraceae (p < 0.01) and a lower abundance of Barnesiellaceae (p < 0.01) compared to the IBS-D group. Furthermore, at the genus level (Fig. 5C–E), we observed that compared to the Ctrl group, the abundance of Alloprevotella (p > 0.05) and Mucispirillum (p < 0.001) was decreased in the IBS-D group. However, after Li05 intervention, the abundance of Alloprevotella, Anaerotruncus, and Mucispirillum increased (p < 0.01 for all three genera). On the other hand, the abundance of Butyricicoccus UCG-009 (p < 0.01), Dubosiella (p < 0.001), Erysipelatoclostridium (p < 0.05), [Ruminococcus] gauvreauii group (p < 0.01), and Blautia (p < 0.05) was significantly higher in the IBS-D group than in the Ctrl group, but their relative abundance decreased in the Li05 group.
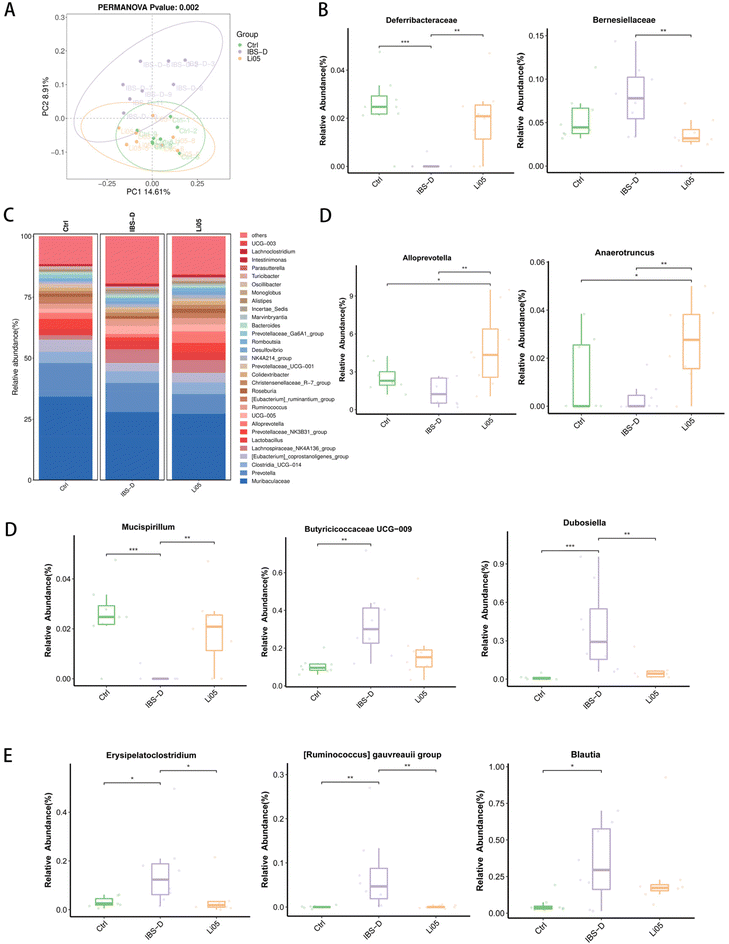 |
| Fig. 5 Changes in gut microbiota in the Ctrl, IBS-D, and Li05 groups. (A) Principal coordinate analysis (PCoA) based on weighted UniFrac distances. Relative abundance of taxa at family (B) and genus (C, D, and E) levels. Data are presented as mean ± SEM, * (p < 0.05), ** (p < 0.01), and *** (p < 0.001). | |
In order to explore potential biomarkers for IBS-D, we conducted LEfSe analysis. As shown in Fig. 6A and B, the IBS-D group exhibited a predominant increase in the relative abundance of Firmicutes, mainly represented by the classes Bacilli and Clostridia. Firmicutes, Bacteroidota, and Actinobacteriota showed significant differences in the Li05 group, with an increase in the relative abundance of five key genera (Alloprevotella, Butyrivibrio, Pediococcus, Frisingicoccus, Coriobacteriaceae UCG-002).
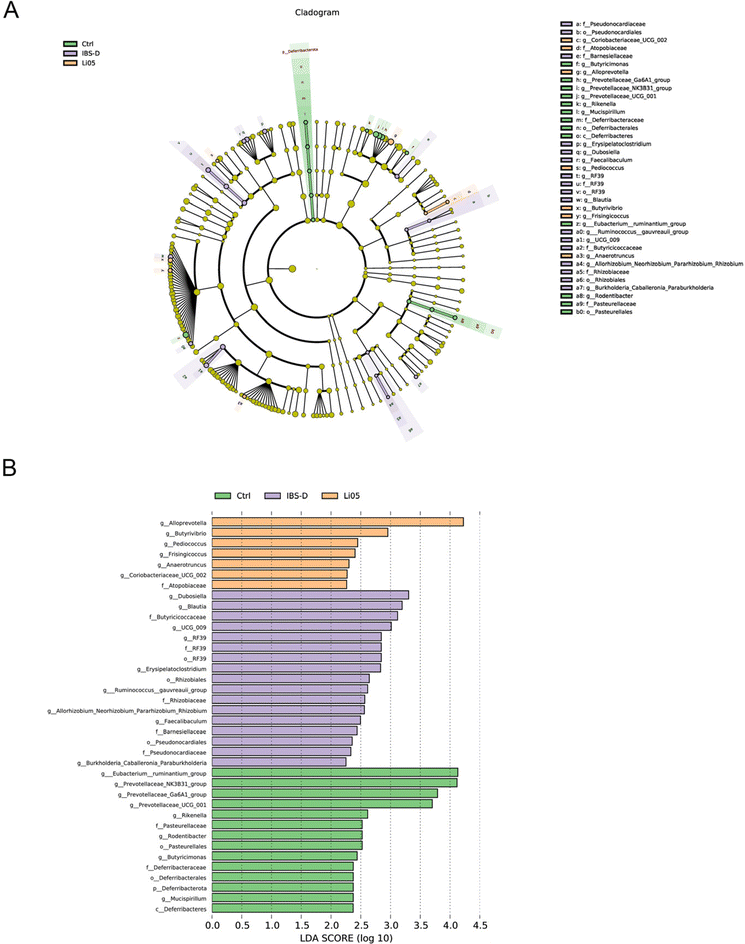 |
| Fig. 6 Changes in the gut microbiota community among the Ctrl, IBS-D, and Li05 groups. (A) LEfSe cladogram illustrating the taxa enriched in the Ctrl group (green), IBS-D group (purple), and Li05 group (yellow). (B) Discriminative biomarkers with an LDA score >2.0 between the Ctrl group (green), IBS-D group (purple), and Li05 group (yellow). | |
Based on our research findings, the key bacteria with significantly increased relative abundance in the IBS-D group mostly belonged to the phylum Firmicutes (Dubosiella, Butyricicoccus UCG-009, and Erysipelatoclostridium), while the relative abundance of bacteria from the phylum Bacteroidota (Prevotellaceae Ga6A1 and Rodentibacter) was significantly decreased. Recent studies have shown an elevated relative abundance of Firmicutes and a reduced abundance of Bacteroidota in IBS, specifically an increase in genera such as Clostridium and Ruminococcus within Firmicutes, and a decrease in genera such as Prevotellaceae, Alloprevotella, and Prevotella within Bacteroidota.42 This is consistent with our research findings. However, there have also been reports of a decreased abundance of Firmicutes and an increased abundance of Bacteroidota in IBS-D patients.16 The characteristic changes in the IBS-D microbiota are still uncertain and require further clinical research evidence.
Mucispirillum utilizes short-chain fatty acids (SCFAs) for energy metabolism in the gut and has been shown to protect mice from colitis by antagonizing Salmonella, exhibiting certain intestinal protective effects.43Blautia and Erysipelatoclostridium are considered to be inflammation-associated microbes. Zhen et al. found that the abundance of Blautia was notably higher in individuals with IBS-D and demonstrated a positive correlation with IL-1β, IL-6, IFN-γ and TNF-α.6Erysipelatoclostridium, identified as a harmful bacterium, showed significantly increased abundance in mice with nonalcoholic fatty liver disease (NAFLD) and was successfully isolated from the intestines of Crohn's disease patients.44–46 After Li05 intervention, the relative abundance of Mucispirillum significantly increased, alongside a significant decrease in the relative abundance of the inflammation-associated microbes Blautia and Erysipelatoclostridium, suggesting its protective effects against IBS-D. Additionally, the relative abundance of Alloprevotella has been positively correlated with the expression levels of intestinal immune-related genes and an increase in inflammatory bowel disease (IBD).47,48 However, our data results showed a decreased relative abundance of Alloprevotella in IBS-D, and additional research is required to clarify the specific role in IBS-D.
Based on our research findings, we propose that Li05 may improve IBS-D by promoting the growth of beneficial bacteria and suppressing the proliferation of harmful bacteria, potentially alleviating mild intestinal inflammation by decreasing the abundance of inflammation-associated microbes.
3.5 Li05 altered the gut metabolism in IBS-D rats
Intestinal microbiota is involved in the metabolism of intestinal components, and the metabolites produced may directly or indirectly contribute to IBS symptoms.49 To explore the metabolic changes in IBS-D and the effect of Li05 on metabolites, we performed an untargeted metabolomics analysis on collected intestinal contents. We identified 1004 different metabolites that were common or unique to each group (Fig. 7A). The results of the orthogonal partial least squares discriminant analysis (OPLS-DA) and partial least squares discrimination analysis (PLS-DA) showed noteworthy differences in metabolites across each group (Fig. 7B). We further analyzed the differential metabolites. The top 50 metabolites showed a predominance of lipids and lipid-like molecules in the differential analysis between the Li05 and IBS-D groups (n = 20) (Fig. 7C), as well as between the IBS-D and Ctrl groups (n = 27) (ESI Fig. S1A†). We also summarized the top 50 significant metabolites in a heatmap between the Li05 and IBS-D groups (Fig. 7D), as well as between the Ctrl and IBS-D groups (ESI Fig. S1B†).
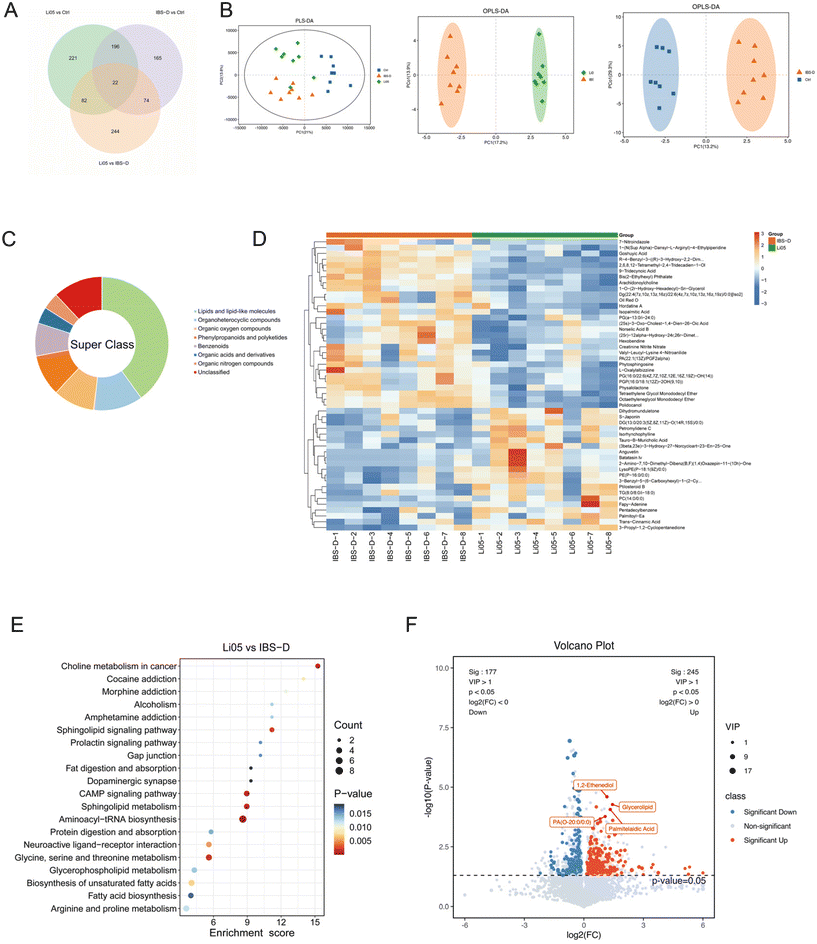 |
| Fig. 7 Changes in the metabolome of colon contents among the Ctrl, IBS-D, and Li05 groups. (A) Number of common or unique different metabolites between the Ctrl, IBS-D, and Li05 groups. (B) Partial least squares discrimination analysis (PLS-DA) and orthogonal partial least squares-discriminant analysis (OPLS-DA) plots depicting the discrimination between the Ctrl, IBS-D, and Li05 groups. (C) Super class classification of the top 50 most importantly altered metabolites. (D) Heatmap displaying hierarchical clustering of the top 50 most significantly altered metabolites between the Li05 and IBS-D groups. (E) The top 20 most significant pathways identified from the KEGG pathway analysis of the significantly altered metabolites between the Li05 and IBS-D groups. (F) Differential metabolites between the Li05 and IBS-D groups. | |
In addition, we performed the KEGG pathway enrichment analysis and found that the main metabolic pathways that differed significantly between the Ctrl and IBS-D groups were primary bile acid biosynthesis, steroid hormone biosynthesis, phenylalanine metabolism, glucagon signaling pathway, and pyruvate metabolism (p < 0.05 for all) (ESI Fig. S1C†). The metabolites involved in primary bile acid biosynthesis, such as cholic acid, deoxycholic acid, 7alpha,25-dihydroxycholesterol and glycochenodeoxycholic acid were significantly increased (ESI Fig. S1D†). In addition, Li05 significantly changed the choline metabolism in cancer, sphingolipid metabolism, cAMP signaling pathway, aminoacyl-tRNA biosynthesis, glycine, serine and threonine metabolism, and biosynthesis of unsaturated fatty acid metabolic pathways (p < 0.015 for all) (Fig. 7E). Significant alterations were observed in the pathways related to lipid metabolism and amino acid metabolism in the Li05 group, with amino acids such as serine, threonine, and tyrosine, and unsaturated fatty acids such as oleic acid, linoleic acid, arachidic acid, docosanoic acid, and tetracosanoic acid significantly increased. In addition, 1,2-ethenediol, glycerolipid, palmitelaidic acid, and PA(O-20:0/0:0) were significantly increased in the Li05 group, which may serve as potential biomarkers for the improvement of IBS-D (Fig. 7F).
The pathophysiology of IBS cannot be explained by obvious structural or tissue abnormalities. Research suggests that colonic exposure to bile acid (BA) malabsorption can stimulate colonic motility and secretion, which may be a mechanism underlying IBS-D.50 IBS-D patients have elevated fecal BA concentrations compared to IBS-C and healthy individuals, with higher levels of BA synthesis and excretion than IBS-C patients. These concentrations are negatively correlated with body weight and fat levels.51 Consistently, our study found a significant upregulation of the primary bile acid biosynthesis pathway in the IBS-D group, primarily involving metabolites such as cholic acid, deoxycholic acid, and glycochenodeoxycholic acid, which can stimulate water secretion, colonic transit and increase stool frequency.52 Based on these findings, we strongly believe that BA malabsorption may be an important pathogenic mechanism in IBS-D. It is crucial to identify the key regulatory molecular mechanisms involved in bile acid breakdown and absorption, which is beneficial for finding effective treatment methods to alleviate symptoms of IBS-D.
Intestinal microbiota is involved in the energy metabolism of host intestinal proteins and peptides, producing various bioactive compounds, among which amino acids and their derivatives play a certain role in IBS.53 Serine plays a role in lipid metabolism and maintaining immune system stability, while threonine can promote growth and enhance immunity.54 In addition, studies suggest that the gut microbiota may play a role in the synthesis and degradation of many neurotransmitters through the production of amino acids and amines, thereby affecting the host's mood and psychological state.55 Based on these findings and our results, we speculate that Li05 may improve energy metabolism and immune function by enhancing mucosal amino acid nutrition, and may also improve IBS-related emotional symptoms by improving neurotransmitter-related metabolism.
Regarding the impact of fatty acids on IBS, a cohort study found an association between polyunsaturated fatty acids (PUFA) and IBS in epidemiology, emphasizing the protective effect of PUFA intake. Supplementation with conjugated linoleic acid can alleviate IBS inflammation by downregulating TNF-α and nuclear factor kappaB (NF-κB) and increasing peroxisome proliferator-activated receptor-gamma (PPAR-γ) and transforming factor beta (TGF-β).56,57 Studies have also found that linoleic acid can inhibit compensatory activation of the Nrf2 pathway, significantly alleviating anxiety and depression symptoms.58 Our results suggested that biosynthesis of unsaturated fatty acid is significantly increased. Therefore, we speculate that Li05 may reduce intestinal inflammation and alleviate clinical symptoms of IBS-D such as anxiety and depression by affecting fatty acid and amino acid metabolism.
3.6 Multiple correlation analysis of IBS-D treated with Li05
In order to further analyze whether Li05 improves IBS-D through gut microbiota and metabolites, we conducted the Pearson correlation analysis. The results showed that the mRNA expression levels of colon chemokine RANTES were negatively correlated with the metabolites MG(0:0/22:1(13Z)/0:0) and 1,2-ethenediol and microbiota Prevotellaceae_NK3B31_group (p < 0.05), and the serum chemokine and pro-inflammatory factors (GRO/KC, IL-6, IL-1β, and IL-18) were closely related to the gut microbiota Prevotella, Bacteroides, Dubosiella, Erysipelatoclostridium, and Ruminococcus, as well as the metabolites Oil Red O, hordatine A, and 9-tridecanoic acid. In addition, IBS-D related symptoms including stool water content, diarrhea index, and loose stool rate were closely related to the metabolites tsuzuic acid, Oil Red O, hordatine A, 9-tridecanoic acid, and the gut microbiota Deferribacterota, Prevotellaceae_NK3B31_group, Dubosiella, and Erysipelatoclostridium (Fig. 8A). Among them, Deferribacterota significantly increased in the Li05 group (p < 0.01) and showed a negative correlation with diarrhea-related indicators (p < 0.05). Dubosiella and Erysipelatoclostridium were positively correlated with pro-inflammatory factors and diarrhea-related indicators, and were significantly reduced in the Li05 group (as shown in Fig. 5). It has also been reported that Erysipelatoclostridium is an inflammation-related microbiota.44 In addition, Oil Red O, hordatine A, and 9-tridecanoic acid, which are positively correlated with inflammatory factors and diarrhea-related indicators (Fig. 7D), were decreased in the Li05 group. These metabolites may be risk factors for IBS-D. 1,2-Ethenediol was significantly increased in the Li05 group, but negatively correlated with RANTES (p < 0.05), which may be a potential protective factor and biomarker for IBS-D. It can be seen that gut microbiota and metabolites are closely related to inflammatory markers and diarrhea in IBS-D. Therefore, Li05 may improve gut inflammation and alleviate symptoms of IBS-D through gut microbiota and metabolites.
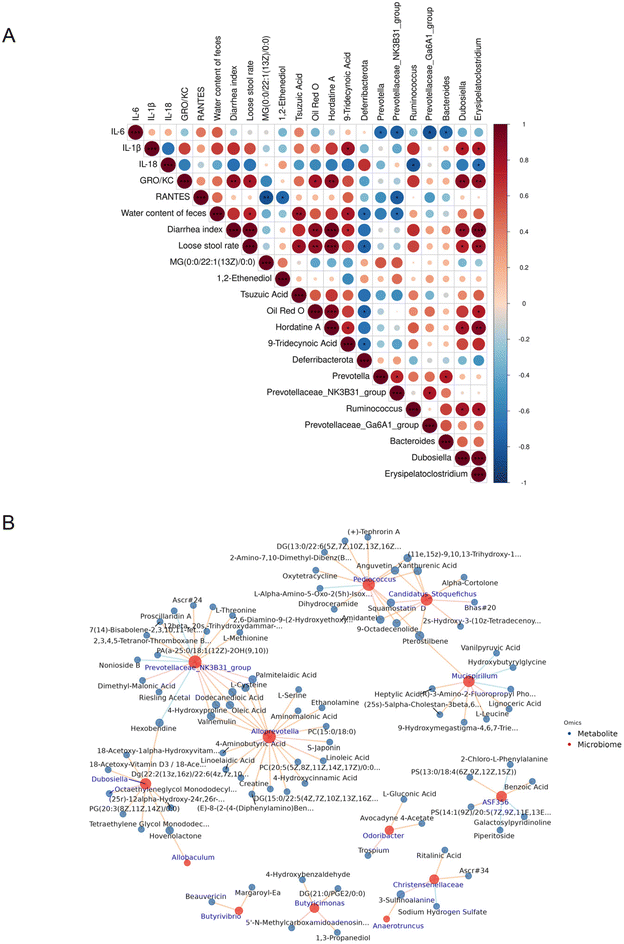 |
| Fig. 8 Multiple correlation analysis of IBS-D treated with Li05. (A) Correlation analysis of chemokines and proinflammatory cytokines, diarrhea related indicators, gut microbiota and metabolites. (B) Correlation analysis of the significantly different microbiota and metabolites between Li05 and IBS-D groups in omics data, and display of data with high correlation coefficients. * (p < 0.05), ** (p < 0.01), and *** (p < 0.001). | |
In addition, in order to further explore which microbiota exert improvement effects on IBS-D by influencing which metabolites, we conducted a further correlation analysis between significantly different genus-level microbiota and metabolites between Li05 and IBS-D groups. As shown in Fig. 8A and B, gut microbiota is also closely related to metabolites. Alloprevotella, Prevotellaceae, and Dubosiella are closely associated with metabolites such as linoelaidic, linoleic acid, L-cysteine, L-threonine, etc. Alloprevotella, Prevotellaceae, and Dubosiella all show significant differences in gut microbiota between the Li05 group and the IBS-D group. Linoleic acid, which is involved in unsaturated fatty acid metabolism, is significantly increased in the Li05 group. Furthermore, metabolites such as L-cysteine and L-threonine are involved in serine, threonine, and tyrosine metabolism, and there are significant differences between the Li05 group and the IBS-D group (as mentioned above). Therefore, the key microbiota of Alloprevotella, Prevotellaceae, and Dubosiella may exert improvement effects on IBS-D by influencing metabolites such as linoleic acid, L-cysteine, L-threonine.
However, our study has some limitations. The correlation analysis between gut microbiota, gut metabolites, inflammatory factors, and diarrhea-related indicators cannot directly prove that Li05 improves inflammation and IBS-D symptoms by changing a certain microbiota or metabolite. Our correlation analysis provides clues for identifying microbiota and metabolites that exert improvement effects. Further experimental studies are needed to confirm the roles and mechanisms of these microbiota and metabolites in IBS-D.
4. Conclusions
In summary, our data demonstrate that Li05 has potential as a probiotic for the treatment of IBS-D. Firstly, Li05 reduces proinflammatory cytokines and chemokines, which can alleviate both intestinal and systemic inflammation. It also modulates the 5-HT signaling pathway, resulting in a reduction in excessive intestinal motility and secretion and a consequent decrease in diarrhea symptoms in IBS-D. Secondly, Li05 regulates the gut microbiota in IBS-D by decreasing the abundance of harmful bacteria such as Dubosiella and Erysipelatoclostridium, which are associated with inflammation, and increasing the abundance of beneficial bacteria such as Mucispirillum. Lastly, Li05 may exert its effects by balancing intestinal immunity and energy metabolism through influencing fatty acid and amino acid metabolism in the gut. However, further research using genomic, transcriptomic, and proteomic techniques, as well as larger clinical research, are necessary to further understand the molecular mechanisms of Li05 and to verify its feasibility and efficacy in clinical applications.
Author contributions
Conceptualization, Lanjuan Li and Youhe Wu; methodology, Youhe Wu; software, Shengjie Li; validation, Longxian Lv and Shiman Jiang; formal analysis, Longxian Lv; investigation, Youhe Wu; resources, Youhe Wu and Lvwan Xu; data curation, Youhe Wu and Shengjie Li; writing – original draft preparation, Youhe Wu and Shengjie Li; writing – review and editing, Hui Chen and Lanjuan Li; visualization, Shengjie Li; supervision, Lanjuan Li; project administration, Lanjuan Li; and funding acquisition, Lanjuan Li. All authors have read and agreed to the published version of the manuscript.
Conflicts of interest
There are no conflicts to declare.
Acknowledgements
This study was supported by the Fundamental Research Funds for the Central Universities (No. 2022ZFJH003), the Shandong Provincial Laboratory Project (SYS202202) and the Research Project of Jinan Micro-ecological Biomedicine Shandong Laboratory (JNL-2022001A, JNL-2022009B, and JNL-2022047D).
References
- W. D. Chey, J. Kurlander and S. Eswaran, Irritable bowel syndrome: a clinical review, J. Am. Med. Assoc., 2015, 313, 949–958 CrossRef CAS PubMed.
- A. C. Ford, A. D. Sperber, M. Corsetti and M. Camilleri, Irritable bowel syndrome, Lancet, 2020, 396, 1675–1688 CrossRef CAS PubMed.
- M. Zhang, Y. Zheng, X. Li, H. Wu, P. Liu, K. Zhang, Z. Shi, M. Lv, F. Wang and X. Tang, Tong-Xie-Yao-Fang alleviates diarrhea-predominant irritable bowel syndrome in rats via the GCN2/PERK-eIF2alpha-ATF4 signaling pathway, Phytomedicine, 2022, 107, 154350 CrossRef CAS PubMed.
- T. Rodrigues, S. Rodrigues Fialho, J. R. Araujo, R. Rocha and A. Moreira-Rosario, Procedures in Fecal Microbiota Transplantation for Treating Irritable Bowel Syndrome: Systematic Review and Meta-Analysis, J. Clin. Med., 2023, 12, 1725 CrossRef PubMed.
- A. Canakis, M. Haroon and H. C. Weber, Irritable bowel syndrome and gut microbiota, Curr. Opin. Endocrinol., Diabetes Obes., 2020, 27, 28–35 CrossRef PubMed.
- Z. Zhen, L. Xia, H. You, Z. Jingwei, Y. Shasha, W. Xinyi, L. Wenjing, Z. Xin and F. Chaomei, An Integrated Gut Microbiota and Network Pharmacology Study on Fuzi-Lizhong Pill for Treating Diarrhea-Predominant Irritable Bowel Syndrome, Front. Pharmacol., 2021, 12, 746923 CrossRef CAS PubMed.
- J. Macsharry, L. O'Mahony, A. Fanning, E. Bairead, G. Sherlock, J. Tiesman, A. Fulmer, B. Kiely, T. G. Dinan, F. Shanahan and E. M. Quigley, Mucosal cytokine imbalance in irritable bowel syndrome, Scand. J. Gastroenterol., 2008, 43, 1467–1476 CrossRef CAS PubMed.
- G. J. Holtmann, A. C. Ford and N. J. Talley, Pathophysiology of irritable bowel syndrome, Lancet Gastroenterol. Hepatol., 2016, 1, 133–146 CrossRef PubMed.
- S. P. Dunlop, D. Jenkins, K. R. Neal and R. C. Spiller, Relative importance of enterochromaffin cell hyperplasia, anxiety, and depression in postinfectious IBS, Gastroenterology, 2003, 125, 1651–1659 CrossRef PubMed.
- J. Gao, T. Xiong, G. Grabauskas and C. Owyang, Mucosal Serotonin Reuptake Transporter Expression in Irritable Bowel Syndrome Is Modulated by Gut Microbiota Via Mast Cell-Prostaglandin E2, Gastroenterology, 2022, 162, 1962–1974 CrossRef CAS PubMed.
- L. A. Houghton, W. Atkinson, R. P. Whitaker, P. J. Whorwell and M. J. Rimmer, Increased platelet depleted plasma 5-hydroxytryptamine concentration following meal ingestion in symptomatic female subjects with diarrhoea predominant irritable bowel syndrome, Gut, 2003, 52, 663–670 CrossRef CAS PubMed.
- M. Simren, L. Simms, D. D'Souza, H. Abrahamsson and E. S. Bjornsson, Lipid-induced colonic hypersensitivity in irritable bowel syndrome: the role of 5-HT3 receptors, Aliment. Pharmacol. Ther., 2003, 17, 279–287 CrossRef CAS PubMed.
- J. M. Yano, K. Yu, G. P. Donaldson, G. G. Shastri, P. Ann, L. Ma, C. R. Nagler, R. F. Ismagilov, S. K. Mazmanian and E. Y. Hsiao, Indigenous bacteria from the gut microbiota regulate host serotonin biosynthesis, Cell, 2015, 161, 264–276 CrossRef CAS PubMed.
- J. R. Marchesi, D. H. Adams, F. Fava, G. D. Hermes, G. M. Hirschfield, G. Hold, M. N. Quraishi, J. Kinross, H. Smidt, K. M. Tuohy, L. V. Thomas, E. G. Zoetendal and A. Hart, The gut microbiota and host health: a new clinical frontier, Gut, 2016, 65, 330–339 CrossRef PubMed.
- L. Zhai, C. Huang, Z. Ning, Y. Zhang, M. Zhuang, W. Yang, X. Wang, J. Wang, L. Zhang, H. Xiao, L. Zhao, P. Asthana, Y. Y. Lam, C. F. W. Chow, J. D. Huang, S. Yuan, K. M. Chan, C. S. Yuan, J. Y. Lau, H. L. X. Wong and Z. X. Bian, Ruminococcus gnavus plays a pathogenic role in diarrhea-predominant irritable bowel syndrome by increasing serotonin biosynthesis, Cell Host Microbe, 2023, 31, 33–44 CrossRef CAS PubMed.
- X. Zhuang, Z. Tian, L. Li, Z. Zeng, M. Chen and L. Xiong, Fecal Microbiota Alterations Associated With Diarrhea-Predominant Irritable Bowel Syndrome, Front. Microbiol., 2018, 9, 1600 CrossRef PubMed.
- I. B. Jeffery, P. W. O'Toole, L. Ohman, M. J. Claesson, J. Deane, E. M. Quigley and M. Simren, An irritable bowel syndrome subtype defined by species-specific alterations in faecal microbiota, Gut, 2012, 61, 997–1006 CrossRef PubMed.
- A. C. Ford, E. M. Quigley, B. E. Lacy, A. J. Lembo, Y. A. Saito, L. R. Schiller, E. E. Soffer, B. M. Spiegel and P. Moayyedi, Efficacy of prebiotics, probiotics, and synbiotics in irritable bowel syndrome and chronic idiopathic constipation: systematic review and meta-analysis, Am. J. Gastroenterol., 2014, 109, 1547–1561 CrossRef PubMed ; quiz 1546, 1562.
- J. Wu, L. Lv and C. Wang, Efficacy of Fecal Microbiota Transplantation in Irritable Bowel Syndrome: A Meta-Analysis of Randomized Controlled Trials, Front. Cell. Infect. Microbiol., 2022, 12, 827395 CrossRef PubMed.
- L. X. Lv, Y. D. Li, X. J. Hu, H. Y. Shi and L. J. Li, Whole-genome sequence assembly of Pediococcus pentosaceus LI05 (CGMCC 7049) from the human gastrointestinal tract and comparative analysis with representative sequences from three food-borne strains, Gut Pathog., 2014, 6, 36 CrossRef PubMed.
- X. Bian, L. Yang, W. Wu, L. Lv, X. Jiang, Q. Wang, J. Wu, Y. Li, J. Ye, D. Fang, D. Shi, K. Wang, Q. Wang, Y. Lu, J. Xie, J. Xia and L. Li, Pediococcus pentosaceus LI05 alleviates DSS-induced colitis by modulating immunological profiles, the gut microbiota and short-chain fatty acid levels in a mouse model, Microb. Biotechnol., 2020, 13, 1228–1244 CrossRef CAS PubMed.
- Q. Xu, S. Gu, Y. Chen, J. Quan, L. Lv, D. Chen, B. Zheng, L. Xu and L. Li, Protective Effect of Pediococcus pentosaceus LI05 Against Clostridium difficile Infection in a Mouse Model, Front. Microbiol., 2018, 9, 2396 CrossRef PubMed.
- D. Shi, L. Lv, D. Fang, W. Wu, C. Hu, L. Xu, Y. Chen, J. Guo, X. Hu, A. Li, F. Guo, J. Ye, Y. Li, D. Andayani and L. Li, Administration of Lactobacillus salivarius LI01 or Pediococcus pentosaceus LI05 prevents CCl(4)-induced liver cirrhosis by protecting the intestinal barrier in rats, Sci. Rep., 2017, 7, 6927 CrossRef PubMed.
- H. Wu, K. Zhan, K. Rao, H. Zheng, S. Qin, X. Tang and S. Huang, Comparison of five diarrhea-predominant irritable bowel syndrome (IBS-D) rat models in the brain-gut-microbiota axis, Biomed. Pharmacother., 2022, 149, 112811 CrossRef CAS PubMed.
- L. Fei and Y. Wang, microRNA-495 reduces visceral sensitivity in mice with diarrhea-predominant irritable bowel syndrome through suppression of the PI3K/AKT signaling pathway via PKIB, IUBMB Life, 2020, 72, 1468–1480 CrossRef CAS PubMed.
- X. Li, K. Ren, X. Hong, S. Guo, S. Yu and S. Yang, Ameliorating effects of electroacupuncture on the low-grade intestinal inflammation in rat model of diarrhea-predominant irritable bowel syndrome, J. Gastroenterol. Hepatol., 2022, 37, 1963–1974 CrossRef PubMed.
- Q. Zhao, W. R. Yang, X. H. Wang, G. Q. Li, L. Q. Xu, X. Cui, Y. Liu and X. L. Zuo, Clostridium butyricum alleviates intestinal low-grade inflammation in TNBS-induced irritable bowel syndrome in mice by regulating functional status of lamina propria dendritic cells, World J. Gastroenterol., 2019, 25, 5469–5482 CrossRef CAS PubMed.
- R. D. Moloney, R. M. Stilling, T. G. Dinan and J. F. Cryan, Early-life stress-induced visceral hypersensitivity and anxiety behavior is reversed by histone deacetylase inhibition, Neurogastroenterol. Motil., 2015, 27, 1831–1836 CrossRef CAS PubMed.
- J. Shen, B. Zhang, J. Chen, J. Cheng, J. Wang, X. Zheng, Y. Lan and X. Zhang, SAHA Alleviates Diarrhea-Predominant Irritable Bowel Syndrome Through Regulation of the p-STAT3/SERT/5-HT Signaling Pathway, J. Inflammation Res., 2022, 15, 1745–1756 CrossRef CAS PubMed.
- Y. D. Choi, T. S. Sung, H. J. Kim, J. H. La, T. W. Kim and I. S. Yang, Increased 5-hydroxytryptamine mediates post-inflammatory visceral hypersensitivity via the 5-hydroxytryptamine 3 receptor in rats, Dig. Dis Sci., 2008, 53, 2909–2916 CrossRef CAS PubMed.
- A. Carrasco-Labra, L. Lytvyn, Y. Falck-Ytter, C. M. Surawicz and W. D. Chey, AGA Technical Review on the Evaluation of Functional Diarrhea and Diarrhea-Predominant Irritable Bowel Syndrome in Adults (IBS-D), Gastroenterology, 2019, 157, 859–880 CrossRef CAS PubMed.
- L. Chang, M. Adeyemo, I. Karagiannides, E. J. Videlock, C. Bowe, W. Shih, A. P. Presson, P. Q. Yuan, G. Cortina, H. Gong, S. Singh, A. Licudine, M. Mayer, Y. Tache, C. Pothoulakis and E. A. Mayer, Serum and colonic mucosal immune markers in irritable bowel syndrome, Am. J. Gastroenterol., 2012, 107, 262–272 CrossRef CAS PubMed.
- Y. Xie, X. Zhan, J. Tu, K. Xu, X. Sun, C. Liu, C. Ke, G. Cao, Z. Zhou and Y. Liu, Atractylodes oil alleviates diarrhea-predominant irritable bowel syndrome by regulating intestinal inflammation and intestinal barrier via SCF/c-kit and MLCK/MLC2 pathways, J. Ethnopharmacol., 2021, 272, 113925 CrossRef CAS PubMed.
- T. Liebregts, B. Adam, C. Bredack, A. Roth, S. Heinzel, S. Lester, S. Downie-Doyle, E. Smith, P. Drew, N. J. Talley and G. Holtmann, Immune activation in patients with irritable bowel syndrome, Gastroenterology, 2007, 132, 913–920 CrossRef CAS PubMed.
- P. A. Hughes, M. Moretta, A. Lim, D. J. Grasby, D. Bird, S. M. Brierley, T. Liebregts, B. Adam, L. A. Blackshaw, G. Holtmann, P. Bampton, P. Hoffmann, J. M. Andrews, H. Zola and D. Krumbiegel, Immune derived opioidergic inhibition of viscerosensory afferents is decreased in Irritable Bowel Syndrome patients, Brain, Behav., Immun., 2014, 42, 191–203 CrossRef CAS PubMed.
- M. D. Gershon and J. Tack, The serotonin signaling system: from basic understanding to drug development for functional GI disorders, Gastroenterology, 2007, 132, 397–414 CrossRef CAS PubMed.
- Z. Chen, J. Luo, J. Li, G. Kim, A. Stewart, J. F. Urban Jr., Y. Huang, S. Chen, L. G. Wu, A. Chesler, G. Trinchieri, W. Li and C. Wu, Interleukin-33 Promotes Serotonin Release from Enterochromaffin Cells for Intestinal Homeostasis, Immunity, 2021, 54, 151–163 CrossRef CAS PubMed.
- M. D. Gershon, Nerves, reflexes, and the enteric nervous system: pathogenesis of the irritable bowel syndrome, J. Clin. Gastroenterol., 2005, 39, S184–S193 CrossRef PubMed.
- R. E. Gregory and D. S. Ettinger, 5-HT3 receptor antagonists for the prevention of chemotherapy-induced nausea and vomiting. A comparison of their pharmacology and clinical efficacy, Drugs, 1998, 55, 173–189 CrossRef CAS PubMed.
- A. Altomare, C. Di Rosa, E. Imperia, S. Emerenziani, M. Cicala and M. P. L. Guarino, Diarrhea Predominant-Irritable Bowel Syndrome (IBS-D): Effects of Different Nutritional Patterns on Intestinal Dysbiosis and Symptoms, Nutrients, 2021, 13, 1506 CrossRef CAS PubMed.
- J. P. Jacobs, V. Lagishetty, M. C. Hauer, J. S. Labus, T. S. Dong, R. Toma, M. Vuyisich, B. D. Naliboff, J. M. Lackner, A. Gupta, K. Tillisch and E. A. Mayer, Multi-omics profiles of the intestinal microbiome in irritable bowel syndrome and its bowel habit subtypes, Microbiome, 2023, 11, 5 CrossRef CAS PubMed.
- M. Rajilic-Stojanovic, D. M. Jonkers, A. Salonen, K. Hanevik, J. Raes, J. Jalanka, W. M. de Vos, C. Manichanh, N. Golic, P. Enck, E. Philippou, F. A. Iraqi, G. Clarke, R. C. Spiller and J. Penders, Intestinal microbiota and diet in IBS: causes, consequences, or epiphenomena?, Am. J. Gastroenterol., 2015, 110, 278–287 CrossRef PubMed.
- S. Herp, S. Brugiroux, D. Garzetti, D. Ring, L. M. Jochum, M. Beutler, C. Eberl, S. Hussain, S. Walter, R. G. Gerlach, H. J. Ruscheweyh, D. Huson, M. E. Sellin, E. Slack, B. Hanson, A. Loy, J. F. Baines, P. Rausch, M. Basic, A. Bleich, D. Berry and B. Stecher, Mucispirillum schaedleri Antagonizes Salmonella Virulence to Protect Mice against Colitis, Cell Host Microbe, 2019, 25, 681–694 CrossRef CAS PubMed.
- M. Nagayama, T. Yano, K. Atarashi, T. Tanoue, M. Sekiya, Y. Kobayashi, H. Sakamoto, K. Miura, K. Sunada, T. Kawaguchi, S. Morita, K. Sugita, S. Narushima, N. Barnich, J. Isayama, Y. Kiridooshi, A. Shiota, W. Suda, M. Hattori, H. Yamamoto and K. Honda, TH1 cell-inducing Escherichia coli strain identified from the small intestinal mucosa of patients with Crohn's disease, Gut Microbes, 2020, 12, 1788898 CrossRef PubMed.
- A. Zhuge, S. Li, P. Lou, W. Wu, K. Wang, Y. Yuan, J. Xia, B. Li and L. Li, Longitudinal 16S rRNA Sequencing Reveals Relationships among Alterations of Gut Microbiota and Nonalcoholic Fatty Liver Disease Progression in Mice, Microbiol. Spectrum, 2022, 10, e0004722 CrossRef PubMed.
- J. K. Jo, S. H. Seo, S. E. Park, H. W. Kim, E. J. Kim, J. S. Kim, J. Y. Pyo, K. M. Cho, S. J. Kwon, D. H. Park and H. S. Son, Gut Microbiome and Metabolome Profiles Associated with High-Fat Diet in Mice, Metabolites, 2021, 11, 482 CrossRef CAS PubMed.
- Y. Fu, H. Gao, X. Hou, Y. Chen and K. Xu, Pretreatment with IPA ameliorates colitis in mice: Colon transcriptome and fecal 16S amplicon profiling, Front. Immunol., 2022, 13, 1014881 CrossRef CAS PubMed.
- L. Huang, J. Zheng, G. Sun, H. Yang, X. Sun, X. Yao, A. Lin and H. Liu, 5-Aminosalicylic acid ameliorates dextran sulfate sodium-induced colitis in mice by modulating gut microbiota and bile acid metabolism, Cell. Mol. Life Sci., 2022, 79, 460 CrossRef CAS PubMed.
- C. Xu, Q. Jia, L. Zhang, Z. Wang, S. Zhu, X. Wang, Y. Liu, M. Li, J. Zhang, X. Wang, J. Zhang, Q. Sun, K. Wang, H. Zhu and L. Duan, Multiomics Study of Gut Bacteria and Host Metabolism in Irritable Bowel Syndrome and Depression Patients, Front. Cell. Infect. Microbiol., 2020, 10, 580980 CrossRef CAS PubMed.
- G. Sciarretta, A. Furno, B. Morrone and P. Malaguti, Absence of histopathological changes of ileum and colon in functional chronic diarrhea associated with bile acid malabsorption, assessed by SeHCAT test: a prospective study, Am. J. Gastroenterol., 1994, 89, 1058–1061 CAS.
- B. S. Wong, M. Camilleri, P. Carlson, S. McKinzie, I. Busciglio, O. Bondar, R. B. Dyer, J. Lamsam and A. R. Zinsmeister, Increased bile acid biosynthesis is associated with irritable bowel syndrome with diarrhea, Clin. Gastroenterol. Hepatol., 2012, 10, 1009–1015 CrossRef CAS PubMed.
- N. M. Sagar, H. Duboc, G. L. Kay, M. T. Alam, A. N. Wicaksono, J. A. Covington, C. Quince, M. Kokkorou, V. Svolos, L. J. Palmieri, K. Gerasimidis, J. R. F. Walters and R. P. Arasaradnam, The pathophysiology of bile acid diarrhoea: differences in the colonic microbiome, metabolome and bile acids, Sci. Rep., 2020, 10, 20436 CrossRef CAS PubMed.
- L. Xiao, Q. Liu, M. Luo and L. Xiong, Gut Microbiota-Derived Metabolites in Irritable Bowel Syndrome, Front. Cell. Infect. Microbiol., 2021, 11, 729346 CrossRef CAS PubMed.
- X. Y. Zhao, J. W. Wang, Y. Yin, K. Li, M. Zhang and F. P. Yan, Effect of Tong Xie Yao Fang on endogenous metabolites in urine of irritable bowel syndrome model rats, World J. Gastroenterol., 2019, 25, 5134–5151 CrossRef CAS PubMed.
- L. Han, L. Zhao, Y. Zhou, C. Yang, T. Xiong, L. Lu, Y. Deng, W. Luo, Y. Chen, Q. Qiu, X. Shang, L. Huang, Z. Mo, S. Huang, S. Huang, Z. Liu, W. Yang, L. Zhai, Z. Ning, C. Lin, T. Huang, C. Cheng, L. L. D. Zhong, S. Li, Z. Bian and X. Fang, Altered metabolome and microbiome features provide clues in understanding irritable bowel syndrome and depression comorbidity, ISME J., 2022, 16, 983–996 CrossRef CAS PubMed.
- O. S. Palsson, W. E. Whitehead, M. A. van Tilburg, L. Chang, W. Chey, M. D. Crowell, L. Keefer, A. J. Lembo, H. P. Parkman, S. S. Rao, A. Sperber, B. Spiegel, J. Tack, S. Vanner, L. S. Walker, P. Whorwell and Y. Yang, Rome IV Diagnostic Questionnaires and Tables for Investigators and Clinicians, Gastroenterology, 2016 DOI:10.1053/j.gastro.2016.02.014.
- H. Stenlund, C. Nilholm, E. Chorell, B. Roth, M. D’Amato and B. Ohlsson, Metabolic Profiling of Plasma in Patients with Irritable Bowel Syndrome after a 4-Week Starch- and Sucrose-Reduced Diet, Metabolites, 2021, 11, 440 CrossRef CAS PubMed.
- L. Cigliano, M. S. Spagnuolo, F. Boscaino, I. Ferrandino, A. Monaco, T. Capriello, E. Cocca, L. Iannotta, L. Treppiccione, D. Luongo, F. Maurano, M. Rossi and P. Bergamo, Dietary Supplementation with Fish Oil or Conjugated Linoleic Acid Relieves Depression Markers in Mice by Modulation of the Nrf2 Pathway, Mol. Nutr. Food Res., 2019, 63, e1900243 CrossRef PubMed.
|
This journal is © The Royal Society of Chemistry 2024 |
Click here to see how this site uses Cookies. View our privacy policy here.