Development and characterization of bael (Aegle marmelos) leaf extract incorporated chitosan-based functional edible coating and its application on stored tomatoes
Received
25th May 2024
, Accepted 11th September 2024
First published on 23rd September 2024
Abstract
A bael (Aegle marmelos) leaf extract (BLE) incorporated chitosan-based functional edible coating was developed in this study. The incorporated functional extract exhibited high 2,2-diphenyl-1-picrylhydrazyl (DPPH) radical scavenging antioxidant activity amounting up to 74.35 ± 0.21%, and impressive antimicrobial properties as high as 5 mg mL−1. As a functional extract, BLE contributed excellently by controlling the ripening of the coated tomatoes stored at ambient temperature. This was evidenced by the recorded patterns of the respiration rate (RR), ΔE color, weight loss, total soluble solids (TSS), titratable acidity (TA), pH, and firmness. The inhibition of mesophilic bacterial and fungal growth contributed remarkably to the enhanced shelf-life of the coated tomatoes. A moderate 1% BLE in the coating (coded BLCT-1) resulted in up to a 250% increase in shelf-life. Scanning Electron Microscopy (SEM) revealed appropriate gelling, coating homogeneity, interblending and continuous surface morphology. Such an excellent texture could be related to the lowered crystallinity of BLCT-1. The characteristic X-ray diffraction peaks suggested the occurrence of chitosan crystal forms I and II in the control as well as BLE incorporated films. Infrared spectra confirmed specific chemical interactions between BLE compounds and chitosan, including the stretching of OH, NH and CO (3360 cm−1 and 967–1195 cm−1 respectively), bending of NH2 (1600 cm−1), and the evidence of residual acetic acid at around 1700 cm−1. With suitable thickness (0.08 ± 0.001 mm), water vapor permeability (WVP, 0.065 ± 0.002 × 10−11 g cm−1 s−1 Pa−1), percentage solubility (PS, 11.889 ± 0.04%) and optical parameters (ΔE: 1.06 ± 0.01), BLCT-1 could be considered as the most ideal edible coating for tomatoes with possible applicability in other perishable fruits and vegetables.
Sustainability spotlight
Functionally enhanced edible food coatings and films minimize the need for chemical preservatives and synthetic plastic, offering an eco-friendly packaging alternative. This promotes food safety and non-exposure to harmful chemicals, thereby supporting SDG 3 (Good Health and Well-being). Through enhancing food security and minimizing post-harvest losses, it also contributes to SDG 2 (Zero Hunger). The reduction in plastic use also addresses SDG 12 (Responsible Consumption and Production). Currently, functional edible coatings are of special importance to the climacteric fruit industry, enabling sustainable post-harvest management. By limiting energy consumption in climate-controlled storage facilities, it contributes to SDG 7 (Affordable and Clean Energy). Bael leaf extract is known for its antimicrobial and antioxidant properties, which, when combined with chitosan, enhances the shelf life and quality of tomatoes by reducing the respiration and ripening rates, spoilage and microbial growth. Overall, the developed functional edible coating offers a holistic solution that addresses the post-harvest storage and management of tomatoes and other perishable fresh produce, also significantly contributing to the management of critical environmental and health sustainability issues.
|
1. Introduction
The development of edible films and coatings for raw and processed foods has made significant advancements in recent decades. While conventional synthetic packaging remains dominant due to consumer and industry demands for high-quality, cost-effective, and long-shelf-life packaging, edible packaging has emerged as a sustainable solution to the environmental and health concerns associated with synthetic materials.1,2 Furthermore, existing food preservation technologies, such as modified atmosphere packaging (MAP), chemical preservatives like sodium benzoate, and wax coatings like shellac, have been shown to possess potential drawbacks that limit their applicability. MAP can be costly and may not provide regulated approaches for adequate protection against moisture and oxygen. Chemical preservatives have been observed to be harmful to human health and affect the flavor and texture of foods. Wax coatings are also non-biodegradable and contain potentially harmful chemicals. In contrast, natural edible coatings like chitosan and alginate offer a superior alternative, providing a biodegradable, non-toxic, and sustainable solution that extends shelf life, improves food safety, and enhances its nutritional value. Natural edible coatings also offer economic and environmental benefits, making them a more appealing option for food preservation.1–5 However, edible packaging itself has limitations, including poor barrier properties, susceptibility to microbial contamination, sensory alterations, and limited shelf life. These challenges can be addressed by incorporating functional components into the packaging materials, which complement the edible coating and enhance its functionality. Functional coatings, developed by incorporating antimicrobials, antioxidants, and nutraceuticals into the coating matrix, can inhibit microbial growth, reduce oxidative damage, and preserve or enhance the nutritional value of the coated food.3–6 The use of functional edible coatings is particularly relevant in the fresh fruit industry, given the perishable nature of fruits, their storage and transportation requirements, and the need for effective farm-to-fork solutions.7
All the major polysaccharides, namely cellulose, starch, chitosan, carrageenan, pectin, alginate and pullulan, have been popular choices for developing edible films.8 Chitosan is the second most abundant natural and non-toxic polymer after cellulose. It has a major advantage of possessing antimicrobial efficacy against various algae, fungi, and bacteria, which makes it highly desirable for application in antimicrobial coatings and films.6,9–11 Studies have established the bacteriostatic properties of chitosan.10 Besides, the ability of chitosan to form smooth, transparent films and cohesive coatings makes it an ideal material for food packaging usage. For casting films, solutions are prepared by dissolving chitosan in acidic solutions, filtered to eliminate impurities and poured over surfaces or molds followed by a drying process. For coating, the solution is applied as a dip or spray over the food surface. In our previously conducted study, 2.15% chitosan was mixed with 0.05% glycerol as the plasticizer, pH was adjusted to 5.6, and it was sieved (50 μm) to obtain a coating solution.12 Disinfected tomato samples were dip coated by immersing in the solution for 1 min followed by 30 s of air-drying.
The functional properties of chitosan films can be significantly enhanced through strategic combinations with other substances. For example, incorporating essential oils, preservatives, or diluted organic or inorganic acid solutions (such as acetic, sorbic, propionic, lactic, glutamic, formic, citric, malic, hydrochloric, gallic, tannic, or polyacid solutions) into chitosan or its derivatives has been shown to improve the antimicrobial effects of the films and coatings.13–15 Moreover, the addition of natural extracts to chitosan-based edible coatings or films can enhance their barrier properties, improve their sensory quality, and complement their shelf-life stability. The ease of impregnating natural extracts into the molecular arrangements of the polymer base of edible coatings at the liquid stage facilitates the formation of functional films and enhances their application on fruit surfaces.9–13 Chitosan-based functional coatings have been successfully applied to various fruits, including papaya (Carica papaya L.),13 strawberry and banana,10Feijoa sellowiana,9 blueberry (Vaccinium myrtillus),16 apples (Malus domestica cv Florina),17 and others, demonstrating their potential to extend shelf life while maintaining quality during storage. Furthermore, the use of natural extracts in chitosan-based edible films can also provide additional health benefits, such as antioxidant and anti-inflammatory effects.10
Natural extracts are also suitable for application in edible coatings, as they can contribute to eliminating the use of hazardous chemicals, serving as green alternatives. The fruit, bark, leaf and roots of the bael (Aegle marmelos) plant have ethnomedicinal usage, especially in the Indian ayurvedic remedies dating back to 1500 BC. The abundantly available bael leaves are rich in various phytochemicals such as alkaloids (aegeline and marmeline), terpenoids (limonene and β-caryophyllene) and flavonoids (kaempferol and rutin).18 Studies have also shown that the extract exhibits antimicrobial properties, inhibiting the growth of foodborne pathogens like E. coli and Staphylococcus aureus. It also possesses the potential to extend the shelf life of fruits and vegetables by reducing microbial growth and spoilage. The extract has also been found to possess high antioxidant activity and potential health benefits, making it a valuable ingredient in nutraceuticals. Additionally, it has been effectively used to improve the quality and safety of meat products and as a natural flavoring agent and antioxidant in tea beverages.19 In edible coating, bael leaf extract (BLE) could induce lengthened and sustained efficacy due to its localization inside the base matrix of the coating. Different edible oils, tea extracts, etc., have been effectively used in coatings for extending shelf life and the retention of quality parameters in model fruits.20–24 The natural abundance, broader growing season, easy harvesting, and low cost create opportunities to utilize the potentiality of BLE in edible coatings. However, the physical and physicochemical characteristics of the coating formulations need careful consideration before application.
Tomatoes (Lycopersicon esculentum Mill.) are commercially significant, widely cultivated climacteric fruits meant for staple consumption and processing into value added products. India ranks third in annual tomato production in the world after China and the USA, wherein its postharvest loss is estimated at 30–40% in the world. High perishability makes it a fruit requiring high technological inputs and hence an ideal model for storage studies. In this research, a functional edible coating was intended to be developed by incorporating BLE in a chitosan base and the material properties of the developed formula were studied. Further, the developed coating formula was applied on tomatoes stored at room temperature and its effects on the physiological ripening and shelf-life of the fruits were elucidated.
2. Materials and methods
2.1. Materials and sampling
Tomato was selected as an ideal representative of climacteric fruits being highly perishable in nature, low in price, widely available and nutritionally significant. Medium molecular weight analytical grade chitosan (Himedia, India) was the coating base and glycerol (Merck, Germany) was used as the plasticizing agent. Fresh bael leaves were collected from a single tree located near the Assam University campus in Silchar, Assam, India. Healthy leaves were sorted and thoroughly washed with distilled water. Enzymatic conversions were ceased by drying the leaves in an oven at 60 °C for 5 min.25 The leaves were further hot air dried at 40 °C till about 4% moisture content and slowly ground in a Philips mixer grinder (HL1632). The obtained powder was sieved to obtain standard particle sizes (100–200 μm) and stored in a sealed container at 4 °C for further experimental use.
2.2. Characterization of uncoated tomatoes
2.2.1. Physical and optical characteristics.
Five fruits were randomly selected and their average weights, locules number, pericarp thickness, fruit density and firmness were measured. Tomato weight was measured by using a digital balance (accuracy = 0.01 g). Pericarp thickness and fruit diameters were measured using a Vernier caliper along the cross-sectional (transverse diameter) and longitudinal (stem to blossom end) axes. Volume occupied by the test fruit was measured as water displaced by the fruit upon immersion. The seed-skin-fiber content in tomato fruits was determined by separating them and the pulp yield was measured. Fruit firmness was measured using a TA.XT. Plus texture analyzer (Stable Micro Systems, SMSP) fitted with a 35 mm aluminum probe under conditions detailed by Purakayastha and Mahanta (2011).26 The surface color of the fruits was determined by using a Hunter Lab Color Quest (Model Ultrascan Vis-Model, USA) and expressed in the L, a, b system. The Hunter Lab scale was used to determine L (lightness–darkness), a (chromaticity parameters, red–green) and b (yellow–blue) values of test samples. Hue angle (H) and chroma (C) values were evaluated using the following formulae.
2.2.2. Chemical characteristics.
The latest standard gravimetric protocols (AOAC, 2019) were used for the estimation of moisture content, total solids (TS), volatile solids (VS), lycopene and ash content.27 Methods described by Rangana (2017) were used for measuring titratable acidity (TA), ascorbic acid, total sugar and reducing sugar.28 The pH of uniformly ground tomato paste was determined with the help of a pH meter (Sartorius, PB-11, Germany). Total carotenoids in the sample were estimated by a method reported by de Carvalho et al. (2012) and the result was reported as μg g−1.29
2.3. Extraction of functional extracts from bael leaves
Bael leaf powder was extracted using a 60% ethanol solution as the extraction medium.30,31 The powder sample (250 g) was wrapped in Whatman no-1 filter paper and placed in the thimbles of the holding chamber of a Soxhlet extractor (SOCSPLUS, Pelican Equipments, India). About 500 ml of the extraction medium was used for carrying out the exhaustive extraction at 90 °C for a period of 48 h using the reflux method. The BLE was then concentrated by evaporating to dryness using a rotary evaporator (Roteva, Equitron, India) at 40 °C. The obtained dark-green colored concentrated BLE was then stored in an airtight dark bottle at 4 °C for further use.
2.3.1.
In vitro antioxidant activity of the extract.
The 2,2-diphenyl-1-picrylhydrazyl (DPPH) radical scavenging capacity of variable concentrated (20%, 40%, 60%, 80% and 100%) BLE in water was assessed according to the method of Brand-Williams et al. (1995) and Anosike et al. (2015) with slight modification.32,33 The DPPH method was considered in this study, as this is one of the best methods for antioxidant determination, and involves the reaction of DPPH radicals with antioxidants, causing a color change from purple to yellow. This color change, measured spectrophotometrically, reflects the antioxidant capacity of the sample, providing sensitive and quantifiable results. The method produces consistent and reproducible results due to the stable nature of the DPPH radical. The decrease in absorbance correlates directly with antioxidant activity, allowing for accurate comparison across samples.
The activity was expressed as the %DPPH scavenging effect, as calculated using the following equation. Distilled water was taken as a control for the test.
2.3.2. Minimum inhibitory concentration (MIC) of the extract against potential spoilage microbes.
A method of Kothari et al. (2011) was used with slight modification to determine the MIC of the extract against specific isolated microbes present in ordinarily spoiled tomato samples.34 Microorganisms grown in culture plates inoculated with spoiled tomatoes were isolated and pure cultures of all bacteria and fungi were subsequently prepared. Nutrient agar and potato dextrose agar were used as growth media for the culture of bacteria and fungi, respectively. Then, using serial dilution and streak plate techniques, a pure culture of individual microorganisms was prepared.35 All bacterial and fungal isolates were characterized by considering their colonial, morphological, microscopic examination and preliminary biochemical characteristics.36 The same types of microbial colonies and growth were observed in triplicate analysis ensuring accuracy.
On preliminary analysis, a total of six bacterial strains (four Gram positive and two Gram negative) and five fungal (two Candida, one Penicillium, one Mucor and one Aspergillus) genera were identified. For experimental ease, the Gram-positive bacteria (4 numbers), Gram negative bacteria (2 numbers) and fungi (5 numbers) were coded as GPB-1, GPB-2, GPB-3, GPB-4, GNB-1, GNB-2, FC-1, FC-2, FP-1, FM-1 and FA-1, respectively. The bacterial isolates were first sub-cultured in a nutrient broth medium and incubated at 37 °C for 18 h, while Sabouraud dextrose agar (SDA) was used as a medium for sub-culturing the fungal isolates for a period of 72 h at 25 °C. Subsequently, these microbes were standardized to 0.5 McFarland standards (1.5 × 108 cfu ml−1).
The minimal inhibitory concentration (MIC) of the extract was determined by a two-fold serial micro-dilution method using sterile 96 well microtitre plates.37,38 Hundred microliters of test extract at final concentrations ranging from 10 to 0.0049 mg ml−1 were poured over 100 μl of standardized cell suspensions taken in each well. Microbial suspensions were used as positive controls and the extract in broth was used as a negative control. Resazurin indication solution was prepared by dissolving 270 mg tablet in 40 ml of sterile distilled water. Qualitatively, the purple colour of the resazurin indicator faded, turned pink or turned colourless in the presence of living microorganisms. The lowest concentration at which colour change occurred was taken as the MIC of the extract for the specific microbe type.
2.4. Preparation of an optimized coating formulation and application on tomatoes
The chitosan + glycerol (C + G) control coating formulation was prepared with chitosan as the base polymer, glycerol as the plasticizer and acetic acid as the solvent, following an optimized and validated method.12 2.15% chitosan and 0.50% glycerol were dissolved in 100 ml of 0.5% glacial acetic acid. The pH of the solutions was adjusted to 5.6 with 0.1 M NaOH and the formulation was sieved through a mesh of 50 μm pore size to obtain the optimized control formulation. BLE concentration showing the highest MIC was taken as the lowest limit of inclusion range in the coating. The upper limit was decided after several pre-experimental trials, so that the visible color and coating properties do not deviate beyond the statistical significance level (p < 0.05) as compared with the control (C + G) coating. Functional coating formulations were hence prepared by adding 0.5%, 1% and 1.5% (w/v) BLE to the optimized control (C + G) formulation. BLE was added dropwise 10 minutes prior to the completion of the stirring process. Sample tomatoes were dipped into the different prepared coating formulations for a period of 1 min with an air-drying time of 30 s repeating for twenty continuous cycles in a programmable dip coater (Xdip-MV1, Apex Instrument, Kolkata). The coated tomato samples were dried under ambient conditions (25 ± 4 °C and 90 ± 4% RH) for 24 h and were then stored without any additional covering or wrap for further analysis. Based on the level of incorporation, BLE incorporated functional-edible coated tomatoes were coded as BLCT-0.5, BLCT-1 and BLCT-1.5, respectively.
2.4.1. Effect of coating on the storage quality of tomatoes and antimicrobial efficacy.
The uncoated and control (C + G), BLCT-0.5, BLCT-1 and BLCT-1.5 coated tomatoes were stored under ambient conditions and analyzed for changes in their weight, pH, TSS, TA, ΔE, firmness and respiration rate (RR). Analyses were performed every 5 days up to 25 days to evaluate the effects of the functional coatings on comparative changes in the physicochemical properties of the coated tomatoes. The storage period was extended until visible spoilage and mold growth were observed on the surface.39,40 Simultaneously, for measuring antimicrobial efficacy of the coatings, tomato samples with each coating type were homogenized for 2 minutes with 200 ml tryptone phosphate water in a table top blender (Philips HL1632) under sterile conditions. The obtained tomato homogenates were serially diluted and poured into plate count agar (PCA) and chloramphenicol glucose agar (CGA) to enumerate mesophilic aerobic bacteria, yeasts and molds, respectively. The inoculated PCA and CGA plates were incubated at 30 °C for 48 h and at 25 °C for 5 days respectively and observed for microbial colony forming units.
2.4.2. Identification of the most effective coating.
Comparative analysis of changes in various physicochemical (weight, TSS, TA, ΔE, firmness, RR and pH) and microbiological (total aerobic mesophilic microorganisms, yeast and mold populations) parameters was carried out for the uncoated and coated tomatoes. Comparisons amongst samples for individual parameters were carried out every 5 days (starting from the 0th day till the 25th day). Duncan's multiple range test was adopted to compare the output of the same parameter obtained from each sample type. The most effective coating was identified based on the significance of differences between the other samples towards desirability throughout the storage period.
2.5. Physical, physicochemical, optical, structural and spectral analysis
The most effective edible coating identified was further assessed for physical, physicochemical, optical, structural and spectral properties and an attempt was made to draw a comparative statement with the control (C + G) coating.
2.5.1. Coating thickness, water vapor permeability and percentage solubility.
The coating thickness was measured using a digital micrometer (Mitutoyo, Japan) with an instrument sensitivity of 0.001 mm. Ten measurements of properly marked cross sectional diameters of each tomato sample were taken before and after coating. The mean values of the difference in readings were expressed as coating thickness. The water vapor permeability (WVP) and percentage solubility (PS) of the coating were obtained following the method previously described in Paul et al. (2018).12
2.5.2. Optical parameters.
The optical parameters of coatings applied on tomatoes were analyzed by determining the overall color difference (ΔE), coating opacity and transparency. The colors of the coated films were measured with a Hunter Lab Color analyzer (Ultrascan Vis-Model, USA) calibrated by using a standard white plate (Y = 93.5, x = 0.3114, and y = 0.3190). Measurements were carried out by placing peeled coated films over the standard. Four measurements were recorded for each sample and average values were reported to get homogeneous data. The overall color difference (ΔE) of the tomato peel on application of coatings was determined from root mean square differences in L, a, and b color values before and after coating. The differential (Δ) values were recorded on day 10 and day 0, thus reporting the changes with time.41 The following formula was used.
ΔE = (ΔL2 + Δa2 + Δb2)0.5 |
Opacity was determined as the relationship between the opacity of each sample on a black standard (Yb) and the opacity of each sample on the white standard (Yw) using the following equation.42
where
Y is the CIE tri-stimulus value.
Film transparency was measured as per Gasti et al., 2020, using a spectrophotometer (Spectronic Genesis 2, Thermo Spectronic, Rochester, USA).42 This method was slightly modified from the ASTM method D1746-92 for measuring the transparency of plastic sheets. The transparency of the films was determined from the following equation:
where
A600 is the absorbance,
T600 is the transmittance at 600 nm, and
b is the film thickness.
2.5.3. Microscopy.
A Scanning Electron Microscope (SEM, JEOL 6993 V) operating at an acceleration voltage of 15 kV was used to observe the surface integrity and microstructure of the control (C + G) and most effective coating (BLCT-1). About a 3 cm2 portion of the coating film was carefully removed with a scalpel and forceps, vacuum dried overnight and assembled on metallic stubs with double-sided tape before coating with a thin layer of gold. The morphological and surface characteristics of the coatings were observed at 1500–4000× magnification and changes in the features due to the incorporation of BLE were analyzed.
2.5.4. X-ray diffractometry.
An X-ray diffractometer (Rigaku Miniflex) was used with a λ value of 1.54040 operating at 30 kV acceleration potential and 15 mA current with a copper target to obtain wide angle X-ray diffractograms (XRDs) of the samples. The scanning range and scan speed of the analysis were 2–70° 2θ and 8° 2θ per min, respectively. The XRD patterns were analyzed and reported in line with already established methods.43 For the incident optics, an X-ray mirror was interposed in the incident beam to increase its intensity and parallelism. A Soller slit of 0.04 rad, a 0.5° divergence slit and a mask of 10 mm were used. A Pixel (2.5°) ultrafast X-ray detector was employed for the diffracted optics, to enhance the quality of the diffraction pattern. Measurements were conducted from 3 to 35° at steps of 0.01° and a time step of 100 s in Bragg–Bretano (θ–2θ) symmetric geometry, and 45 kV and 40 mA were used for the tube power.44 Parameters related to crystal characteristics, including percentage crystallinity, were assessed by the method as described by Singh et al. (2006) to evaluate changes in crystalline behaviors and ordered crystalline orientation due to the incorporation of BLE.45 The formula for the determination of % crystallinity is given below.
2.5.5. Infrared spectroscopy.
The Fourier Transform Infrared Spectra (FTIR) of both coating samples, i.e., control (C + G) and most effective coatings (BLCT-1), were obtained using a spectrometer (Nicolet Instruments 410 FT-IR) equipped with a DTGS detector and KBr optics. The equipment was operated in a scanning range of 4000–370 cm−1 with a resolution of 2.0 cm−1. The detector was purged carefully using clean dry nitrogen gas to reduce moisture and increase the signal level. Vacuum dried film samples (2.0 mg) were mixed with 100 mg KBr and compressed for 1 minute to form a transparent pallet. The pallet was then introduced into the machine, and infrared spectra were recorded. The vibrational spectra of the test samples were interpreted and analyzed using the inbuilt software.
2.6. Statistical analysis
Statistical analysis of the obtained data was performed with the assistance of Statistical Package for the Social Sciences (SPSS 21, IBM) software. The mean values of the different replicated data obtained were represented with ± standard error. Duncan's multiple range test (p < 0.05) at the 95% confidence level (P = 0.05) and Analysis of Variance (ANOVA) were performed to compare the means of different data sets and in making statistically accurate judgments and interpretations.
3. Results and discussion
3.1. Physical, optical and chemical attributes of tomatoes
The values of the physical, optical and chemical properties of randomly selected experimental tomato samples are presented in Table 1. All the physical parameters were found within the range as previously reported for different tomato varieties.26 The varieties having a larger number of locules are considered juicier and more suitable for table use. Tomato fruits with a shape index of 1 are round, values between 1.1 and 1.2 are oval and those having a shape index of 1.4 or more are pear shaped. The shape index of the test sample indicated that the analyzed tomatoes were in the shape range of round to oval.46 The firmness of tomatoes is the most relevant property related to the ripening rate and its susceptibility to damage during harvesting and processing. The firmness and L, a, and b color values of the test tomatoes were found to be in accordance with previously reported findings, indicating their ripeness. Optimally ripe tomatoes are characterized by a firm fruit texture and good finger feel, which has a direct correlation with consumer acceptability. Furthermore, harvesting tomatoes at the optimal ripening stage determines the shelf life of the tomatoes on storage.47 The red color of tomatoes appears when lycopene is formed in the fibers during the yellow ripening stage and continues until the red ripening stage, at which the pigment concentration is highest.48 The lycopene content of the experimental tomato samples was recorded as 2.73 ± 0.05 mg/100 g. The chemical properties of the sample tomatoes were found to be most similar to those of the VR-415 variety of tomatoes.26 The acid content of a tomato variety should range from 0.35 to 0.55% to make it suitable for processing.49,50
Table 1 Physical, optical and chemical properties of fresh tomato samplesa
Sl. No. |
Parameter(s) |
Values |
(Mean ± SE and n = 3).
|
Physical attributes
|
1 |
Average fruit weight (g) |
62.0 ± 1.3 |
2 |
Locule number |
2.6 ± 0.23 |
3 |
Shape index (L/T) |
0.986 ± 0.03 |
4 |
Pericarp thickness (mm) |
7.12 ± 0.21 |
5 |
Fruit density (g cm−3) |
0.991 ± 0.001 |
![[thin space (1/6-em)]](https://www.rsc.org/images/entities/char_2009.gif) |
Fruit texture
|
6 |
Firmness (kg mm−2) |
3.701 ± 0.012 |
![[thin space (1/6-em)]](https://www.rsc.org/images/entities/char_2009.gif) |
Optical parameters
|
7 |
L
|
43.33 ± 0.11 |
8 |
a
|
15.21 ± 0.09 |
9 |
b
|
20.03 ± 0.13 |
10 |
Hue angle (H) |
52.79 ± 0.14 |
11 |
Chroma (C) |
25.15 ± 0.10 |
![[thin space (1/6-em)]](https://www.rsc.org/images/entities/char_2009.gif) |
Physicochemical attributes
|
12 |
Moisture content (% w.b.) |
93.90 ± 0.14 |
13 |
TSS (°B) |
3.90 ± 0.06 |
14 |
TA (%) |
0.38 ± 0.01 |
15 |
pH |
4.06 ± 0.01 |
16 |
Ascorbic acid (mg/100 g) |
17 ± 0.04 |
17 |
TS (%) |
6.44 ± 0.06 |
18 |
VS (g/100 g TS) |
82.35 ± 0.13 |
19 |
Ash content (g/100 g TS) |
17.65 ± 0.09 |
20 |
Reducing sugar (%) |
3.24 ± 0.01 |
21 |
Total sugar (%) |
3.62 ± 0.02 |
22 |
Sucrose (%) |
2.13 ± 0.01 |
23 |
Total carotenoid (mg/100 g) |
3.14 ± 0.08 |
24 |
Lycopene content (mg/100 g) |
2.73 ± 0.05 |
3.2. Analysis of the leaf extract
3.2.1. Antioxidant activity.
The results of the DPPH scavenging antioxidant activity of BLEs having different concentrations are presented in Table 2. The results indicated progressive increase of hydrogen ion donating ability with increased concentration of BLE.51 The high antioxidant activity of the leaf extracts is attributed to the presence of various flavonoid compounds in the leaves. The outcomes of the assays were consistent with the findings of Shafique et al. (2011) on Ocimum basilicum essential oil.52 The hydrodistillates of its leaves at higher concentrations exhibited increased hydrogen donors, leading to higher DPPH scavenging activities. The increased scavenging activity observed in this study directly correlated with the increased BLE concentrations. This was evident from the statistically significant superiority of values observed for the concentrations used. Specifically, the concentrations of BLE ranging from 20% to 100% exhibited a substantial increase in antioxidant activity, demonstrating an enhancement of up to approximately 35%.
Table 2
In vitro antioxidant activity of the bael leaf extract (BLE)a
In vitro antioxidant activity |
(Mean ± SE and n = 3).
|
BLE concentration (%) |
20% |
40% |
60% |
80% |
100% |
DPPH scavenging capacity (%) |
56.54 ± 1.61a |
60.89 ± 0.87b |
63.31 ± 0.33c |
69.5 ± 2.05d |
74.35 ± 0.84e |
3.2.2. Minimum inhibitory concentration.
The MIC values of BLE against each bacterium (GPB-1, GPB-2, GPB-3, GPB-4, GNB-1 and GNB-2) and fungi (FC-1, FC-2, FP-1, FM-1 and FA-1) isolated from spoiled experimental tomatoes are presented in Table 3. The results were in accordance with those in a previous study by Kothari et al. (2011).34 The MIC values were found to range between 1.25 and 5 mg ml−1. The highest inhibition efficacy was exhibited against GPB-3, FC-1 and FP-1 with the lowest MIC value of 1.25 mg ml−1 for each. The lowest efficacy was seen against GPB-2, GNB-1 and FA-1 (MIC = 5 mg ml−1). Statistically, higher effectiveness was observed against the fungal species than the bacteria, indicating its potential application as an antifungal substance. This can be evidenced by the fungal species demonstrating lower overall MIC values compared to the bacterial species. The difference in inhibitory effects could be due to differences in the structural make-up of the cell wall, as well as their absorption affinity towards the extracts by the cell wall components or metabolic pathways and intra-cellular interactions inside the pathogenic cells.
Table 3 Minimal inhibitory concentrations (MICs) of bael leaf extracts against spoilage microbes of tomatoesa
Isolated microorganisms in coded names |
MIC (mg ml−1) |
Superscript with the same letter indicates no difference at the significance level of p ≤ 0.05.
|
Bacterial species |
GPB-1 |
2.5b |
GPB-2 |
5
c
|
GPB-3 |
1.25a |
GPB-4 |
2.5b |
GNB-1 |
5
c
|
GNB-2 |
2.5b |
Fungal species |
FC-1 |
1.25a |
FC-2 |
2.5b |
FP-1 |
1.25a |
FM-1 |
2.5b |
FA-1 |
5
c
|
3.2.3. Effect of functional-edible coating on the storage quality of tomatoes.
The values of storage quality parameters, namely total color difference (ΔE), respiration rate, weight loss (%), total soluble solid (TSS), titratable acidity (TA), pH, firmness and antimicrobial efficiency, during the complete storage period of 25 days are graphically presented in Fig. 1a–i.
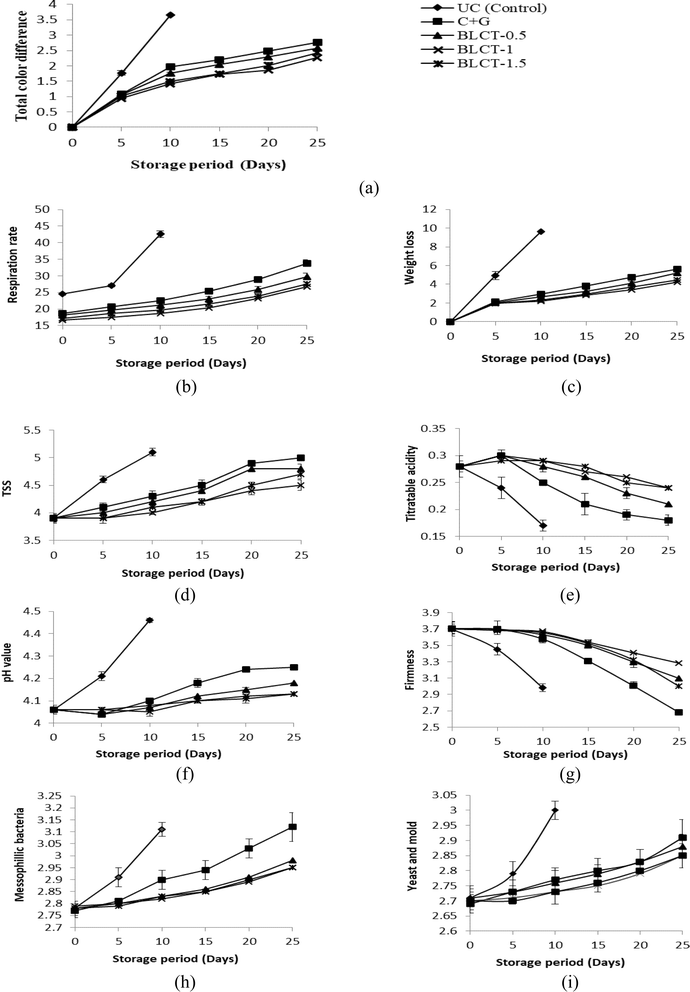 |
| Fig. 1 Effect of a bael leaf extract incorporated functional-edible coating on the (a) total color difference, (b) respiration rate, (c) % weight loss, (d) total soluble solids (TSS), (e) titratable acidity (TA), (f) pH, (g) textural firmness, (h) mesophilic bacterial count, and (i) yeast and mold count of tomatoes during storage. | |
3.2.3.1. Total color difference (ΔE).
Change in fruit surface color is one of the reliable parameters to assess the extent of ripening and storage life including senescence. Prominent yet distinctly different patterns of increase in ΔE were observed in the coated and uncoated tomatoes (Fig. 1a). The incorporation of 1% (g ml−1) BLE significantly controlled surface color deviations during storage. It was observed that beyond the concentration of 1% (g ml−1), the functional extracts showed significant (p ≤ 0.05) elevation in the ΔE values of coated tomatoes in most of the assessment points. Various extracts, like tea leaf extract, ascorbic acid, cysteine and 4-hexylresorcinol, in edible coating have also been reported to reduce the surface color deviation and browning of various fruits, including tomatoes and fresh-cut apples.20 The ΔE value of the coated samples exhibited a slower rate of increase during the initial stages of the storage period, followed by a pronounced acceleration after the 10th day (Fig. 1a). This can be ascribed to the intrinsic fruit physiology and the effects of the coating on the fruit's metabolic and senescence processes. Moreover, the lycopene content in tomato skin may decrease due to various factors. These factors include degradation caused by exposure to heat, light, and oxygen, as documented in previous studies.53 Enzymatic processes, such as increased activity of enzymes like polygalacturonase and pectin methyl esterase during tomato ripening, also contribute to lycopene breakdown.54 Additionally, structural changes occurring during overripening, such as cell wall degradation, can disrupt the distribution of lycopene within the fruit.55 Moreover, overripe tomatoes exhibit higher respiration rates, accelerating metabolic processes that can impact lycopene stability.56 A similar trend in surface color variation was also reported for cherries, grapes, strawberries and fresh-cut apples during their storage after different post-harvest treatments.39,57
3.2.3.2. Respiration rate.
The respiration rate increases over the storage period due to post-harvest stress physiology and ripening. The respiration rate of uncoated samples was 42.6 ± 0.97 mg CO2 kg−1 h−1 on the 10th day of storage (Fig. 1b). The application of the coating reduced the respiration rate and contributed to the extension of shelf-life, suggesting probable suppression of ACC synthase at the RNA level, resulting in the inhibition of ethylene biosynthesis.58 The incorporation of BLE in the optimized coating formulation remarkably exhibited effectiveness in terms of reduction in the respiration rate. The addition of functional extracts above 1% (g ml−1) showed significantly a slowed down increase of the respiration rate, as statistically compared with other samples at p ≤ 0.05 (Fig. 1b). It is also worthy of note that BLCT-1 achieved a significant reduction of over 37% in the total respiration rate compared to the uncoated control tomatoes, as observed at the maximum storage periods (25 days for BLCT-1 and 10 days for the control). Compared to the control (C + G) coating, BLCT-1 and BLCT-1.5 showed a significant reduction in the respiration rate over the storage period by ∼25% and ∼15%, respectively. The BLE might have contributed to filling up of void spaces present in the inter-molecular networks of chitosan gel, thereby reducing the gas permeability of the film. The high antioxidative activity of the extract might have also contributed to the efficacy of coating in controlling the respiration of tomatoes. However, a higher concentration of functional extracts created interference in the intermolecular linkages between the coating compositions, resulting in more permeability.
3.2.3.3. Weight loss.
There was a continuous increase in the cumulative weight loss of both coated and uncoated tomatoes during storage (Fig. 1c). Cumulative weight loss was observed to be significantly higher for the uncoated ones on the 10th day coupled with visible degradation in fruit turgor and firmness. This could be related to the lowering of metabolic activity and associated tissue senescence of fruits over the storage time after coating application.59 The coating provided a barrier for water movement, protected fruit skin from mechanical and microbial injuries, and sealed the small wounds and stomatal openings leading to reduced dehydration.39,60 The effectiveness of various edible coatings and some other post-harvest treatments in reducing the weight loss of other perishable fruits has been reported. 20,59,61,62 The rate of increased weight loss followed an exponential trend during the initial phase of storage, but a significant resistance was observed later in the tomatoes coated with the functional edible coating (Fig. 1c). The addition of BLE at 1% (g ml−1) in the optimized C-G coating showed a significant effect of preventing weight loss (Fig. 1c). However, beyond this concentration, a significant increase in weight loss (p ≤ 0.05) was recorded at most of the assessment points during the storage period. A weight loss reduction as high as 56% was recorded for BLCT-1 compared to UC (control) at their maximum storage periods (25th day and 10th day, respectively). Additionally, BLCT-1.5 showed 25% lower weight loss% compared to the control (C + G). The anti-senescent action of BLE probably caused the triggering effect in reducing the weight loss of the coated tomatoes by filling the spaces inside the inter-molecular networks, leading to better structural continuity, reduced respiration rate and oxidative damage. Several researchers have reported the reduction in the rate of weight loss of various fruits (pomegranate, citrus, Valencia oranges, grape, and tomato) by introducing different functional agents, like tea leaf extract, edible coconut oil, and various other edible lipids, in the coating base of different biopolymers.20,59,63
3.2.3.4. Total soluble solids.
The total soluble solids of all categories of tomato samples increased with storage time and a comparatively higher rate of increase was observed for uncoated tomatoes (Fig. 1d). The application of coating slowed down the respiration and metabolic activities as also evidenced by the results of the respiration rate, weight loss and ΔE. Such a strongly inter-related pattern of variation retarded the ripening process and led to the slower conversion of complex carbohydrates to sugars.50,64 The incorporation of BLE into the coating formulation resulted in the reduction of the rate of change in stored fruit TSS as compared to BLE-free coating. The patterns were also similar to the other parameters. Tomatoes coated with BLCT-0.5, BLCT-1 and BLCT-1.5 showed 8%, 15% and 10% reduction in TSS respectively as compared to C-G coated tomatoes after the 25th day of storage.
3.2.3.5. Titratable acidity.
The trend of change in the titratable acidity of the test tomatoes was observed to be significantly different from that of other parameters (Fig. 1e). The TA of coated tomatoes slightly increased during the initial storage period (till the 5th day), following which the TA of the coated fruits decreased irrespective of the presence or absence of functional extracts. This could be attributed to the higher production of organic acids during this phase in comparison to degradation and metabolic utilization. Possible higher water loss from the coated fruit during the initial storage period as compared to the late storage period might have also triggered the concentration of acids in the fruit tissue.39,65 The conversion of different organic acids to sugars during the process of ripening after maturity resulted in decreased TA after 5 days of storage for all samples.66 The rate of change of TA reduced with the percent incorporation of BLE in the coating formulation. Relative to the control (C + G) coating, the BLCT-0.5, BLCT-1, and BLCT-1.5 coatings exhibited 15%, 35% and 35% increased TTA values respectively over the storage period. The uncoated tomatoes showed logarithmic decline in TA values throughout the storage period till the 10th day as also evidenced by other researchers for tomatoes.20,63,65
3.2.3.6. pH.
The trend of variation of pH was opposite to that of TA (Fig. 1f). The pH of the coated and uncoated tomatoes increased over the period of storage. Changes in TA in the tomato flesh due to enzymatic conversion, metabolic consumption and degradation of various acids (mainly citric acid) in different metabolic processes during storage can be considered as the major cause of variation in the pH of the fruit pulp.67,68 Excessive weight loss from the fruit tissue can also intensify the declining trend of the pH value by concentrating the organic acids.39 Uncoated tomatoes reflected a significantly higher pH value in comparison to the coated samples till the end of their shelf life. The BLE-coated samples exhibited a 6–7% reduction in pH values relative to the uncoated control (UC), and a 1.5–2% reduction compared to the control (C + G) coating. The addition of a higher percentage of functional BLE (beyond 1% g ml−1) did not significantly help in minimizing the rate of change in pH over the period of storage. It may be due to the differences in the modified atmosphere created by different coatings and their interactions. The prevention of weight loss and the antioxidant efficacy of BLE could have also contributed some additional resistance in controlling the deviation of pH in fruit flesh. A similar pH range and pattern of variation have been reported for various fruits including tomatoes and strawberries with different post-harvest treatments.20,39,50
3.2.3.7. Tomato firmness.
The firmness or textural rigidity of tomatoes decreased with storage time. The rate of reduction was higher in the case of uncoated tomatoes compared to the coated ones (Fig. 1g). The application of coating on the tomato surface slowed down the respiration and metabolic activities. There was a sharp decrease in the firmness of the coated tomatoes during the mid-stages of storage. This may be due to the higher ripening rate and subsequent enzymatic breakdown of storage and structural polysaccharides. The rapid decrease of firmness can additionally be attributed to the dehydration and physiological breakdown in the fruit during this storage period.69 The addition of BLE to the coating formulation resulted in the reduction of the rate of decrease in firmness with storage time as compared with the coating without the functional extract. The effectiveness of the functional extracts in maintaining firmness demonstrated a similar pattern to that of the other parameters. Additionally, it is noteworthy that tomatoes coated with BLCT-1 maintained firmness, with a reduction of only 22% compared to C + G coated tomatoes (control) after 25 days of storage, while BLCT-0.5 and BLCT-1.5 coatings resulted in firmness reductions of 12% and 15%, respectively.
3.2.3.8. Antimicrobial efficiency of the coatings.
Changes in the microbial community (mesophilic bacteria, yeast and mold) in both coated and uncoated tomatoes with storage time are shown in Fig. 1h and j. It can be clearly observed that the rapid rise of bacterial (mesophile) and fungal (yeast and mold) populations was retarded by the application of coating. The desirable effect of the coating is due to the atmospheric modification by the applied coating that decreased the O2 concentration with a parallel increase in CO2 concentration in the fruit tissue. The addition of functional extracts further improved the microbe barrier properties of basic C-G coating by reducing the growth of both bacterial and fungal populations.
The addition of BLE resulted in a significant reduction in bacterial (mesophile) and fungal (yeast and mold) growth during the storage as compared to the coating without the functional extract. The antimicrobial efficacy of the functional coatings in controlling bacterial (mesophile) and fungal (yeast and mold) populations followed the pattern of BLCT-1.5 > BLCT-1 > BLCT-0.5 > C + G (for mesophiles) and BLCT-1.5 = BLCT-1 > BLCT-0.5 > C-G (for yeast and mold). It is clearly observed that the coatings with higher proportions of leaf extract showed better and significantly higher antimicrobial efficacy (p ≤ 0.05). These extracts could have reduced the surface micro-floral community and also prevented the entry of microorganisms from the storage environment. Previous research also reported a reduction in the microbial colony count in coated fruits like grapes and tomatoes.20,59
3.3. Most effective concentration of the functional extract
The effect of BLE embedded in the optimized coating revealed that at 1% concentration the extract produced the highest efficiency in almost all the physical, physicochemical and optical parameters. The antimicrobial efficacy of functional edible coatings increased with the increase in the proportion of the extract in the coating base (p ≤ 0.05). However, the efficacy of BLCT-1.5 and BLCT-1 was found to be equal against yeast and mold (p ≤ 0.05). Analyzing the performance of the extract concentrations in the coating with respect to the retention of the quality attributes and extension of the shelf-life of tomatoes for most of the studied parameters, BLCT-1 was found to be the best among the three functional coating formulations and subjected to further analysis.
3.3.1. Physical, physicochemical, optical, structural and spectral analysis of the selected coating.
The most effective functional edible coating BLCT-1 was further analyzed for its various physical, physicochemical, optical, structural and spectral properties and compared with the basic C-G coating to identify a significant intervention in the coating due to the incorporation of BLE into the base (Table 4).
Table 4 Physical, physicochemical and optical parameters of C-G and BLCT-1 coatingsa
Parameter(s) |
C-G |
BLCT-1 |
Superscript with the same letter indicates no difference at the significance level of p ≤ 0.05 (mean ± SE and n = 3).
|
Physical and physicochemical properties
|
Coating thickness (mm) |
0.09 ± 0.002a |
0.08 ± 0.001b |
Water vapor permeability (10−11 g cm−1 s−1 Pa−1) |
0.066 ± 0.001a |
0.065 ± 0.002a |
Percentage solubility (%) |
11.539 ± 0.05a |
11.889 ± 0.04a |
![[thin space (1/6-em)]](https://www.rsc.org/images/entities/char_2009.gif) |
Optical parameters
|
L (black–white) |
94.52 ± 1.08a |
88.4 ± 0.98b |
a (green–red) |
3.26 ± 0.03a |
3.03 ± 0.07b |
b (blue–yellow) |
4.25 ± 0.01a |
4.14 ± 0.06a |
H
|
52.51 ± 1.66a |
53.8 ± 1.43a |
C
|
5.36 ± 0.08a |
5.13 ± 0.06a |
Opacity (%) |
14.26 ± 0.15a |
16.25 ± 0.11a |
Transparency |
21.44 ± 0.23a |
20.12 ± 0.16b |
Overall color difference (ΔE) |
0.85 ± 0.01a |
1.06 ± 0.01b |
3.3.2. Coating thickness, water vapor permeability and percentage solubility.
It was observed that both the coatings had almost the same WVP and water solubility with no significant difference at p ≤ 0.05 (Table 4). However, the thickness of the C-G coating was ∼15% higher than that of BLCT-1. It can be due to the incorporation of extracts and filling up of the void spaces inside the polysaccharide network, as well as on the surface.20,70
3.3.3. Optical parameters.
The b, H and C color values of both coatings were observed to be almost the same without any significant difference at p ≤ 0.05 (Table 4). However, the L, a, opacity (%), and transparency of C-G and BLCT-1 showed significant differences. Compared to C-G, BLCT-1 showed 15% higher opacity, 5% lower transparency and 20% higher ΔE. These could be attributed to the contribution of the green-red color coordinates of the color profile by the BLE of the C-G coating. Siripatrawan and Harte (2010) also observed a decrease in film lightness after the incorporation of aqueous green tea extract in chitosan films.71 This may help to avoid photo-oxidative deterioration of coated fruits. These current results are also supported by a number of previous findings on different optical parameters including opacity, transparency and overall color difference for coatings with starch and other complex polysaccharides.20,39,70
3.3.4. Coating morphology and microstructure.
The SEM images of both coatings indicated sufficient uniformity and homogeneous distribution of chitosan and glycerol blends (Fig. 2). Any distinct separation of coating constituents was not observed, which confirms their proper and uniform blending. The absence of ghost granules suggested that appropriate gel formation was achieved with adequate mixing and deaeration of the coating formulation, leading to a smoother surface with the absence of any cracks at the microstructure level of the coating. Continuity and integrity observed in the surface microstructure of both types of coatings (C-G and BLCT-1) suggested their uniform blocking of the epidermal apertures of the tomato peel that efficiently controlled the water vapor and gas exchange. This further contributed to the reduction of the respiration rate, the rate of color change, weight loss and TSS during storage as compared to the control sample.72 The development of coatings with a compact structural orientation achieved by incorporating different natural extracts represents remarkable progress towards the improvement of different properties of edible coatings and films for the post-harvest storage and management of fruits and vegetables.
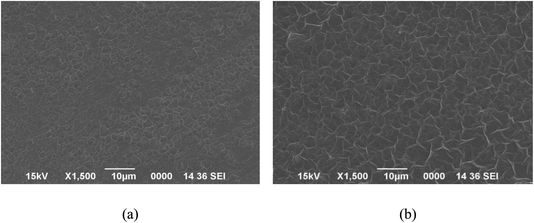 |
| Fig. 2 Scanning electron microstructure (SEM) of the coatings: (a) C-G and (b) BLCT-1. | |
3.3.5. X-ray diffractometry.
Fig. 3 shows the XRD spectra of both coatings. The presence of residual reflection at 2θ near 10–15° and the strongest reflection at 2θ = 20–25° indicated the presence of crystal form I and crystal form II, respectively. This aligns with the findings reported by Souza et al. (2010).73 It is known that even under maximum dried conditions, chitosan contains bound water (at least 5%). The confirmation about the presence of chain segments of chitin and the hydrated crystalline structure of chitosan was given by the crystalline peak centered at around 10° (peak I). The XRD graphs of the experimental coatings revealed that the overall crystalline patterns of both coatings were similar, but the crystallinity of the chitosan films slightly decreased with the incorporation of BLE. Hence, the crystallinity of the C-G coating was higher than that of BLCT-1. It is also reported that the crystallinity of the coating material has a direct impact on the flexibility of the coated film.73 Hence, these structural variations could be further linked to the changes in the water solubility, WVP, gas permeability and various other functional parameters of the coatings.
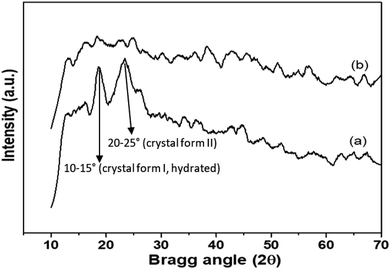 |
| Fig. 3 X-ray diffraction (XRD) pattern of (a) C-G and (b) BLCT-1. | |
3.3.6. Fourier transmission infrared spectroscopy (FTIR).
FTIR spectroscopy was used to assess the possible chemical interactions between the film polymer and the functional agents introduced into it. Fig. 4 presents the FTIR spectra obtained for the chitosan coating (C-G) and functional edible coating (BLCT-1). Both spectra showed similar patterns with absorption peaks at 3360 cm−1 and 967–1195 cm−1, corresponding to the stretching of –OH and –NH bonds and to the stretching vibration of C–O bonds, respectively.74 Absorption peaks at 2926 cm−1 and 2877 cm−1 (C–H stretching) and a band at 1600 cm−1 (–NH2 bending) also confirmed the presence of water. Bands at 1480–1380 cm−1 (asymmetric C–H bending and C–H stretching of the CH2 group) and 1087 cm−1 (skeletal vibration involving the bridge C–O stretching) of glucosamine residue were also observed. In addition, the vibration of residual acetic acid was evidenced near 1700 cm−1, corresponding to the carbonyl vibration of –COOH. The change in the intensity of this peak for coatings incorporated with functional extracts indicated the interaction of acetic acid with compounds in BLE present in the coating matrix.
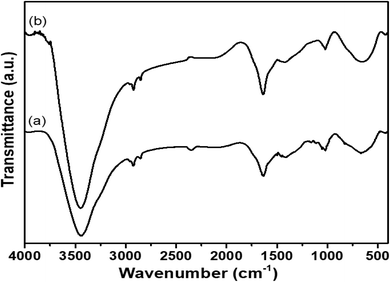 |
| Fig. 4 Fourier transmission infrared spectroscopy (FTIR) spectra of (a) C-G and (b) BLCT-1. | |
Shifting of a few peaks in BLCT-1 suggested specific interactions occurring between the functional groups of bioactive compounds introduced through BLE and the active groups of the chitosan coating. The interactions between functional extracts and the chitosan base were also reflected in the area of the bands, which is a measure of the extent of such interactions. In all cases, the introduction of bioactive compounds led to changes in that area, indicating different intensities of the chemical bonds established in these materials. These differences could influence different physical and physicochemical properties of the coating. Similar findings were also observed by Siripatrawan and Harte (2010), who probed the formation of covalent bonds between chitosan and green tea extract (2%, 5%, 10% and 20%).71
4. Conclusions
This study revealed impressive antioxidant and antimicrobial properties of BLE. Incorporating BLE into the coating significantly slowed down ripening parameters, thereby subsequently extending the shelf life. A moderately higher concentration of the extract, i.e. 1%, can be recommended for maximum control over the incremental elevation of RR, ΔE, weight loss, TSS, TA, pH and spoilage microorganisms. The functional edible coatings have surprisingly increased the shelf life of tomatoes by up to 250% as compared with uncoated tomatoes.
This work aimed to comprehensively cover multiple possible aspects, to confirm our pre-experimental hypothesis and reach a conclusion through detailed experimental analysis. Scientific research is never-ending and every vertical research study opens a new scope for future researchers. Hence, further opportunities of new research in the domain of edible coating interaction, functional mechanisms, consumer safety, establishment of this technology with other produce, marketing trials, etc. are opened with the findings of this work for the welfare of science and society.
Data availability
All the data underlying the study are available in the published article itself. The data are represented in the form of tables, figures or within the text of the publication. If additional data are available and not included with the article, the authors may be contacted at E-mail: sankumarpaul@gmail.com; or duttahimjyoti@gmail.com to obtain the data free of cost.
Conflicts of interest
There are no conflicts to declare.
References
- V. Siracusa, P. Rocculi, S. Romani and M. D. Rosa, Biodegradable polymers for food packaging: a review, Trends Food Sci. Technol., 2008, 19(12), 634–643, DOI:10.1016/j.tifs.2008.07.003.
- A. Petraru and S. Amariei, A novel approach about edible packaging materials based on oilcakes-A review, Polymers, 2023, 15(16) DOI:10.3390/polym15163431.
- M. Vargas, C. Pastor, A. Chiralt, D. J. McClements and C. González-Martínez, Recent advances in edible coatings for fresh and minimally processed fruits, Crit. Rev. Food Sci. Nutr., 2008, 48(6), 496–511, DOI:10.1080/10408390701537344.
- A. Sirelkhatim, S. Mahmud, A. Seeni, N. H. M. Kaus, L. C. Ann and S. K. M. Bakhori,
et al., Review on zinc oxide nanoparticles: Antibacterial activity and toxicity mechanism, Nano-Micro Lett., 2015, 7(3), 219–242, DOI:10.1007/s40820-015-0040-x.
- A. Pavinatto, M. A. V. de Almeida, A. C. G. Malpass, M. H. Okura, D. T. Balogh and R. C. Sanfelice, Coating with chitosan-based edible films for mechanical/biological protection of strawberries, Int. J. Biol. Macromol., 2020, 151, 1004–1011, DOI:10.1016/j.ijbiomac.2019.11.076.
- J. Jovanović, J. Ćirković, A. Radojković, D. Mutavdžić, G. Tanasijević and K. Joksimović,
et al., Chitosan and pectin-based films and coatings with active components for application in antimicrobial food packaging, Prog. Org. Coat., 2021, 158(106349), 106349, DOI:10.1016/j.porgcoat.2021.106349.
- X. Sun, J. Wang, M. Dong, H. Zhang, L. Li and L. Wang, Food spoilage, bioactive food fresh-keeping films and functional edible coatings: Research status, existing problems and development trend, Trends Food Sci. Technol., 2022, 119, 122–132, DOI:10.1016/j.tifs.2021.12.004.
- A. Liyanapathiranage, R. S. Dassanayake, A. Gamage, R. R. Karri, A. Manamperi and P. Evon,
et al., Recent developments in edible films and coatings for fruits and vegetables, Coatings, 2023, 13(7), 1177, DOI:10.3390/coatings13071177.
- J. C. Zárate-Moreno, D. M. Escobar-Sierra and R. Ríos-Estepa, Development and evaluation of chitosan-based food coatings for exotic fruit preservation, BioTech, 2023, 12(1), 20, DOI:10.3390/biotech12010020.
- C. Zhou, J. Bai, F. Zhang, R. Zhang, X. Zhang and K. Zhong,
et al., Development of mussel-inspired chitosan-derived edible coating for fruit preservation, Carbohydr. Polym., 2023, 321(121293), 121293, DOI:10.1016/j.carbpol.2023.121293.
- X. Liu, W. Liao and W. Xia, Recent advances in chitosan based bioactive materials for food preservation, Food Hydrocolloids, 2023, 140(108612), 108612, DOI:10.1016/j.foodhyd.2023.108612.
- S. K. Paul, S. Sarkar, L. N. Sethi and S. K. Ghosh, Development of chitosan based optimized edible coating for tomato (Solanum lycopersicum) and its characterization, J. Food Sci. Technol., 2018, 55(7), 2446–2456, DOI:10.1007/s13197-018-3162-6.
- S. dos Passos Braga, G. A. Lundgren, S. A. Macedo, J. F. Tavares, W. A. dos Santos Vieira and M. P. S. Câmara,
et al., Application of coatings formed by chitosan and Mentha essential oils to control anthracnose caused by Colletotrichum gloesporioides and C. brevisporum in papaya (Carica papaya L.) fruit, Int. J. Biol. Macromol., 2019, 139, 631–639, DOI:10.1016/j.ijbiomac.2019.08.010.
- E. Melro, F. E. Antunes, G. J. da Silva, I. Cruz, P. E. Ramos and F. Carvalho,
et al., Chitosan films in food applications. Tuning film properties by changing acidic dissolution conditions, Polymers, 2020, 13(1), 1, DOI:10.3390/polym13010001.
- S. J. Lee, M. A. Gwak, K. Chathuranga, J. S. Lee, J. Koo and W. H. Park, Multifunctional chitosan/tannic acid composite films
with improved anti-UV, antioxidant, and antimicrobial properties for active food packaging, Food Hydrocolloids, 2023, 136(108249), 108249, DOI:10.1016/j.foodhyd.2022.108249.
- R. Eldib, E. Khojah, A. Elhakem, N. Benajiba and M. Helal, Chitosan, Nisin, silicon dioxide nanoparticles coating films effects on blueberry (Vaccinium myrtillus) quality, Coatings, 2020, 10(10), 962, DOI:10.3390/coatings10100962.
- P.-A. Popescu, L. M. Palade, I.-C. Nicolae, E. E. Popa, A. C. Miteluţ and M. C. Drăghici,
et al., Chitosan-based edible coatings containing essential oils to preserve the shelf life and postharvest quality parameters of organic strawberries and apples during cold storage, Foods, 2022, 11(21), 3317, DOI:10.3390/foods11213317.
- A. K. Sahu, B. R. Kar, K. Deepthi, S. K. Pallath, S. J. Dakni and P. Samal,
et al., Gas chromatography and mass spectroscopy analysis and phytochemical characterization of Aegle marmelos (Bael) leaf, Stem and its screening of antimicrobial activity Primary tabs, GSC Biol. Pharm. Sci., 2019, 8(3), 122–130, DOI:10.30574/gscbps.2019.8.3.0159.
- A. Venthodika, N. Chhikara, S. Mann, M. K. Garg, S. A. Sofi and A. Panghal, Bioactive compounds of Aegle marmelos L., medicinal values and its food applications: A critical review, Phytother. Res., 2021, 35(4), 1887–1907, DOI:10.1002/ptr.6934.
- D. K. Das, H. Dutta and C. L. Mahanta, Development of a rice starch-based coating with antioxidant and microbe-barrier properties and study of its effect on tomatoes stored at room temperature, Lebenson. Wiss. Technol., 2013, 50(1), 272–278, DOI:10.1016/j.lwt.2012.05.018.
- M. Radi, E. Firouzi, H. Akhavan and S. Amiri, Effect of gelatin-based edible coatings incorporated with Aloe vera and black and green tea extracts on the shelf life of fresh-cut oranges, J. Food Qual., 2017, 2017, 1–10, DOI:10.1155/2017/9764650.
- A. Sathiyaseelan, K. Saravanakumar, A. V. A. Mariadoss, C. Ramachandran, X. Hu and D.-H. Oh,
et al., Chitosan-tea tree oil nanoemulsion and calcium chloride tailored edible coating increase the shelf life of fresh cut red bell pepper, Prog. Org. Coat., 2021, 151(106010), 106010, DOI:10.1016/j.porgcoat.2020.106010.
- K. Prasad, G. Singh, S. K. Singh, J. Pradhan, U. Kumar and H. Singh, Plant extract and essential oil coating prolongs shelf life and maintains keeping quality of papaya fruit during storage, J. Food Process. Preserv., 2022, 46(11) DOI:10.1111/jfpp.17015.
- A. S. Sekarina, M. H. S. H. Supriyadi, E. Susanto, P. L. Show and A. Ningrum, Effects of edible coatings of chitosan - fish skin gelatine containing black tea extract on quality of minimally processed papaya during refrigerated storage, Carbohydr. Polym. Technol. Appl., 2023, 5(100287), 100287, DOI:10.1016/j.carpta.2023.100287.
- K. D. Effraim, H. A. Salami and T. S. Osewa, The effects of aqueous leaf extract of Ocimum gratissium on haematological and biochemical parameters in rabbits, Afr. J. Biomed. Res., 2000, 3(3), 175–179 Search PubMed.
- M. D. Purkayastha and C. L. Mahanta, Physicochemical properties of five different tomato cultivars of Meghalaya and their suitability in food processing, Afr. J. Food Sci., 2011, 5(12), 657–667 Search PubMed.
-
Official Methods of Analysis of the Association of Official Analytical Chemists: Official Methods of Analysis of AOAC International, AOAC, 2019 Search PubMed.
-
S. Ranganna, Handbook of Analysis and Quality Control for Fruit and Vegetable Products, Tata McGraw-Hill Education, 2017 Search PubMed.
- L. M. J. de Carvalho, P. B. Gomes, R. L. O. de Godoy, S. Pacheco, P. H. F. do Monte and J. L. V. de Carvalho,
et al., Total carotenoid content, α-carotene and β-carotene, of landrace pumpkins (Cucurbita moschata Duch): A preliminary study, Food Res. Int., 2012, 47(2), 337–340, DOI:10.1016/j.foodres.2011.07.040.
- O. C. Nwinyi, N. C. Salom, O. O. Ajani, C. O. Ikpo and K. O. Ogunniran, Antibacterial effects of extracts of Ocimumgratissimum and piper guineense on Escherichia coli and Staphylococcus aureus, Afr. J. Food Sci., 2009, 3, 22–25 Search PubMed.
- E. Fachriyah, D. Kusrini, I. B. Haryanto, S. M. B. Wulandari, W. I. Lestari and S. Sumariyah, Phytochemical test, determination of total phenol, total flavonoids and antioxidant activity of ethanol extract of Moringa leaves (Moringa oleifera lam), J. Kim. Sains Apl., 2020, 23(8), 290–294, DOI:10.14710/jksa.23.8.290-294.
- W. Brand-Williams, M. E. Cuvelier and C. Berset, Use of a free radical method to evaluate antioxidant activity, Lebenson Wiss Technol., 1995, 28(1), 25–30, DOI:10.1016/s0023-6438(95)80008-5.
- C. A. Anosike, N. E. Ogbodo, A. L. Ezugwu, R. I. Uroko, C. C. Ani and O. Abonyi, DPPH (1,1-Diphenyl-2-Picrylhydrazyl) radical scavenging activity of some ethnomedicinal plants in Nigeria, Am.-Eurasian J. Toxicol. Sci., 2015, 7(2), 104–109, DOI:10.1248/bpb.24.1202.
- S. Kothari, V. Mishra, S. Bharat and S. D. Tonpay, Antimicrobial activity and phytochemical screening of serial extracts from leaves of Aegle marmelos (Linn.), Acta Pol. Pharm., 2011, 68(5), 687–692 CAS.
- S. Mbajiuka and E. Enya, Isolation of Microorganisms associated with Deterioration of Tomato (Lycopersicon esculentum) and Pawpaw (Carica papaya) Fruits, Int. J. Curr. Microbiol. Appl. Sci., 2014, 3(5), 501–512 Search PubMed.
- A. Tang, A. O. Haruna, N. M. A. Majid and M. B. Jalloh, Effects of selected functional bacteria on maize growth and nutrient use efficiency, Microorganisms, 2020, 8(6), 854, DOI:10.3390/microorganisms8060854.
-
A. L. R. Spencer and J. F. T. Spencer, Public Health Microbiology: Methods and Protocols, Humana Press Inc, 2004 Search PubMed.
- T. Prapaiwong, W. Srakaew, C. Wachirapakorn and C. Jarassaeng, Effects of hydrolyzable tannin extract obtained from sweet chestnut wood (Castanea sativa Mill.) against bacteria causing subclinical mastitis in Thai Friesian dairy cows, Vet. World, 2021, 2427–2433, DOI:10.14202/vetworld.2021.2427-2433.
- P. Hernández-Muñoz, E. Almenar, V. D. Valle, D. Velez and R. Gavara, Effect of chitosan coating combined with postharvest calcium treatment on strawberry (Fragaria×ananassa) quality during refrigerated storage, Food Chem., 2008, 110(2), 428–435, DOI:10.1016/j.foodchem.2008.02.020.
- M. M. Rahman, M. N. Islam, M. E. Islam, M. A. Wazed and A. S. M. Kamruzzaman, Determination of post-harvest losses and shelf life of tomato as influenced by different types of polythene at room temperature (28°C), Int. J. Sustain. Agric. Res., 2010, 5, 28–31 Search PubMed.
- N. Maftoonazad and H. S. Ramaswamy, Postharvest shelf-life extension of avocados using methyl cellulose-based coating, Lebenson. Wiss. Technol., 2005, 38(6), 617–624, DOI:10.1016/j.lwt.2004.08.007.
- T. Gasti, S. Dixit, S. P. Sataraddi, V. D. Hiremani, S. P. Masti and R. B. Chougale,
et al., Physicochemical and biological evaluation of different extracts of edible Solanum nigrum L. leaves incorporated chitosan/poly (vinyl alcohol) composite films, J. Polym. Environ., 2020, 28(11), 2918–2930, DOI:10.1007/s10924-020-01832-6.
- R. Sindhu, A. Devi and B. S. Khatkar, Morphology, structure and functionality of acetylated, oxidized and heat moisture treated amaranth starches, Food Hydrocolloids, 2021, 118(106800), 106800, DOI:10.1016/j.foodhyd.2021.106800.
- H. Zhang, B. Chen, H. Jiang, C. Wang, H. Wang and X. Wang, A strategy for ZnO nanorod mediated multi-mode cancer treatment, Biomaterials, 2011, 32(7), 1906–1914, DOI:10.1016/j.biomaterials.2010.11.027.
- V. Singh, S. Z. Ali, R. Somashekar and P. S. Mukherjee, Nature of crystallinity in native and acid modified starches, Int. J. Food Prop., 2006, 9(4), 845–854, DOI:10.1080/10942910600698922.
- O. P. Meena and V. Bahadur, Genetic associations analysis for fruit yield and its contributing traits of indeterminate tomato (Solanum lycopersicum L.) germplasm under open field condition, J. Agric. Sci., 2015, 7(3) DOI:10.5539/jas.v7n3p148.
- F. A. Elsayed, H. Abeer and A. Asma, Biologically-based strategies to reduce postharvest losses of tomato, Afr. J. Biotechnol., 2011, 10(32), 6040–6044 Search PubMed.
- R. Ilahy, I. Tlili, M. W. Siddiqui, C. Hdider and M. S. Lenucci, Inside and beyond color: Comparative overview of functional quality of tomato and watermelon fruits, Front. Plant Sci., 2019, 10, 769, DOI:10.3389/fpls.2019.00769.
-
W. A. Guold, Berry S. Tomatoes for canning. Autdoor Crop Res, Agric Develop Centre, Ohio, 1972 Search PubMed.
- N. Pila, N. B. Gol and T. V. R. Rao, Effect of post harvest treatments on physicochemical characteristics and shelf life of tomato (Lycopersiconesculentum Mill.) fruits during storage, Am.-Eurasian J. Agric. Environ. Sci., 2010, 9(5), 470–479 CAS.
- F. Abdoul-Latif, P. Edou, F. Eba, N. Mohamed, A. Ali and S. Djama,
et al., Antimicrobial and antioxidant activities of essential oil and methanol extract of Jasminumsambac from Djibouti, Afr. J. Plant Sci., 2010, 4, 38–43 Search PubMed.
- M. Shafique, S. J. Khan and N. H. Khan, Study of antioxidant and antimicrobial activity of sweet basil (Ocimum basilicum) essential oil, Pharmacology Online, 2011, 1, 105–111 Search PubMed.
- M. N. Bin-Jumah, M. S. Nadeem, S. J. Gilani, B. Mubeen, I. Ullah and S. I. Alzarea,
et al., Lycopene: A natural arsenal in the war against oxidative stress and cardiovascular diseases, Antioxidants, 2022, 11(2), 232, DOI:10.3390/antiox11020232.
- E. E. Badin, R. Quevedo-Leon, A. Ibarz, P. D. Ribotta and A. R. Lespinard, Kinetic modeling of thermal degradation of color, lycopene, and ascorbic acid in crushed tomato, Food Bioproc. Tech., 2021, 14(2), 324–333, DOI:10.1007/s11947-021-02579-1.
- V. Nour, I. Trandafir and M. E. Ionica, Evolution of antioxidant activity and bioactive compounds in tomato (Lycopersicon esculentum Mill.) Fruits during Growth and Ripening, J. Appl. Bot. Food Qual., 2014, 87, 97–103, DOI:10.5073/JABFQ.2014.087.015.
- S. Forlani, S. Masiero and C. Mizzotti, Fruit ripening: the role of hormones, cell wall modifications, and their relationship with pathogens, J. Exp. Bot., 2019, 70(11), 2993–3006, DOI:10.1093/jxb/erz112.
- X. Meng, B. Li, J. Liu and S. Tian, Physiological responses and quality attributes of table grape fruit to chitosan preharvest spray and postharvest coating during storage, Food Chem., 2008, 106(2), 501–508, DOI:10.1016/j.foodchem.2007.06.012.
- S.-K. Jung and H.-S. Choi, Assessing the effectiveness of natural coating application in prolonging shelf-life in plumcot fruits, Sustainability, 2021, 13(19), 10737, DOI:10.3390/su131910737.
- L. Sánchez-González, C. Pastor, M. Vargas, A. Chiralt, C. González-Martínez and M. Cháfer, Effect of hydroxypropylmethylcellulose and chitosan coatings with and without bergamot essential oil on quality and safety of cold-stored grapes, Postharvest Biol. Technol., 2011, 60(1), 57–63, DOI:10.1016/j.postharvbio.2010.11.004.
- G. Y. Cortez-Mazatán, L. A. Valdez-Aguilar, R. H. Lira-Saldivar and R. D. Peralta-Rodríguez, Polyvinyl acetate as an edible coating for fruits. Effect on selected physiological and quality characteristics of tomato, Rev. Chapingo Ser. Hortic., 2011, XVII(1), 15–22, DOI:10.5154/r.rchsh.2011.17.003.
- E. Ochoa, S. Saucedo-Pompa, R. Rojas-Molina, H. de la Garza, A. V. Charles-Rodríguez and C. N. Aguilar, Evaluation of a candelilla wax-based edible coating to prolong the shelf-life quality and safety of apples, Am. J. Agric. Biol. Sci., 2011, 6(1), 92–98, DOI:10.3844/ajabssp.2011.92.98.
- B. G. S. de Medeiros, A. C. Pinheiro, J. A. Teixeira, A. A. Vicente and M. G. Carneiro-da-Cunha, Polysaccharide/protein nanomultilayer coatings: Construction, characterization and evaluation of their effect on ‘Rocha’ pear (Pyrus communis L.) shelf-life, Food Bioproc. Tech., 2012, 5(6), 2435–2445, DOI:10.1007/s11947-010-0508-0.
- A. T. Oz and Z. Ulukanli, Application of edible starch-based coating including glycerol plus Oleum Nigella on arils from long-stored whole pomegranate fruits: Oleum Nigella on arils pomegranate fruits, J. Food Process. Preserv., 2012, 36(1), 81–95, DOI:10.1111/j.1745-4549.2011.00599.x.
- M. Tolasa, F. Gedamu and K. Woldetsadik, Impacts of harvesting stages and pre-storage treatments on shelf life and quality of tomato (Solanum lycopersicum L.), Cogent Food Agric., 2021, 7(1) DOI:10.1080/23311932.2020.1863620.
- N. Kumar, P. Neeraj and A. Trajkovska Petkoska, Improved shelf life and quality of tomato (Solanum lycopersicum L.) by using chitosan-pullulan composite edible coating enriched with pomegranate peel extract, ACS Food Sci. Technol., 2021, 1(4), 500–510, DOI:10.1021/acsfoodscitech.0c00076.
- H. Toğrul and N. Arslan, Extending shelf-life of peach and pear by using CMC from sugar beet pulp cellulose as a hydrophilic polymer in emulsions, Food Hydrocolloids, 2004, 18(2), 215–226, DOI:10.1016/s0268-005x(03)00066-3.
- H. A. Rathore, T. Masud, S. Sammi and A. H. Soomro, Effect of storage on physico-chemical composition and sensory properties of Mango (Mangifera indica L.) variety Dosehari, Pak. J. Nutr., 2007, 6, 143–148 CrossRef.
- G. E. Anthon, M. LeStrange and D. M. Barrett, Changes in pH, acids, sugars and other quality parameters during extended vine holding of ripe processing tomatoes, J. Sci. Food Agric., 2011, 91(7), 1175–1181, DOI:10.1002/jsfa.4312.
- M. L. Zambrano-Zaragoza, E. Mercado-Silva, P. Ramirez-Zamorano, M. A. Cornejo-Villegas, E. Gutiérrez-Cortez and D. Quintanar-Guerrero, Use of solid lipid nanoparticles (SLNs) in edible coatings to increase guava (Psidium guajava L.) shelf-life, Food Res. Int., 2013, 51(2), 946–953, DOI:10.1016/j.foodres.2013.02.012.
- M. G. Carneiro-da-Cunha, M. A. Cerqueira, B. W. S. Souza, M. P. Souza, J. A. Teixeira and A. A. Vicente, Physical properties of edible coatings and films made with a polysaccharide from Anacardium occidentale L, J. Food Eng., 2009, 95(3), 379–385, DOI:10.1016/j.jfoodeng.2009.05.020.
- U. Siripatrawan and B. R. Harte, Physical properties and antioxidant activity of an active film from chitosan incorporated with green tea extract, Food Hydrocolloids, 2010, 24(8), 770–775, DOI:10.1016/j.foodhyd.2010.04.003.
- M. H. Jafarizadeh, A. Osman, C. P. Tan and R. R. Abdul, Development of an edible coating based on chitosan-glycerol to delay ‘Berangan’ banana (Musa sapientum cv. Berangan) ripening process, Int. Food Res. J., 2011, 18(3), 989–997 Search PubMed.
- B. W. S. Souza, M. A. Cerqueira, J. T. Martins, A. Casariego, J. A. Teixeira and A. A. Vicente, Influence of electric fields on the structure of chitosan edible coatings, Food Hydrocolloids, 2010, 24(4), 330–335, DOI:10.1016/j.foodhyd.2009.10.011.
- Y. Pranoto, S. K. Rakshit and V. M. Salokhe, Enhancing antimicrobial activity of chitosan films by incorporating garlic oil, potassium sorbate and nisin, Lebenson. Wiss. Technol., 2005, 38(8), 859–865, DOI:10.1016/j.lwt.2004.09.014.
|
This journal is © The Royal Society of Chemistry 2024 |
Click here to see how this site uses Cookies. View our privacy policy here.