DOI:
10.1039/D4FB00126E
(Review Article)
Sustainable Food Technol., 2024,
2, 1456-1468
Exploring natural chalcones: innovative extraction techniques, bioactivities, and health potential
Received
25th April 2024
, Accepted 2nd July 2024
First published on 14th August 2024
Abstract
Chalcones, a class of secondary metabolites within the flavonoid family, are characterized by a distinct C6-C3-C6 structure. They are prevalent in both edible and medicinal plants and serve as critical precursors in the flavonoid biosynthesis pathway. Structurally, chalcones are α,β-unsaturated ketones known for their significant bioactive properties which lend them therapeutic potential for a variety of bioactivities. Research into the bioavailability of chalcones and their derivatives has revealed both potential barriers and enhancements regarding their use in nutraceutical and pharmaceutical contexts. The current review provides a comprehensive discussion on the biosynthesis, chemistry, natural occurrence—especially in medicinal and dietary plants, bioavailability, and important roles of chalcones and their derivatives. Moreover, it delves into the toxicological and pharmacological properties of chalcone derivatives, covering their antioxidant, anticancer, antidiabetic, anti-inflammatory, antimalarial, antimicrobial, immunosuppressive, and neuroprotective effects. There is a call for further research to clarify their structure–activity relationships, address toxicity concerns, understand the cellular mechanisms behind their actions, and explore their interactions with other molecules.
Sustainability spotlight
The chalcone concentration in natural sources like tomatoes, apples, licorice and fingerroot is high to exert sustainable effects on its pharmacological properties. Dealing with the significant role of extraction techniques, this study highlights the ethnopharmacological applications of chalcones, with a focus on their critical biological activities both in vitro and in vivo, including antibacterial, antioxidant, antitumor, antimalarial, and anti-inflammatory effects. Chalcones offer a sustainable solution to health problems, contribute to the food industry and environmental wellbeing and achieve improved nutrition and sustainable agriculture.
|
1 Introduction
Chalcones represent a significant group of flavonoid substances, which are widely found in consumable plants such as vegetables and fruits, including apples, tomatoes, fingerroot, licorice, ashitaba leaves, wax jambu, and kava.1,2 Chalcones are synthesized from two precursors, malonyl-CoA (three molecules) and 4-coumaroyl-CoA (one molecule), through the phenylpropanoid and flavonoid biosynthesis pathways. They are recognized as the biological precursors to open-chain flavonoids and isoflavonoids and significantly contribute to the flavor, pigmentation, and health benefits of vegetables and fruits. Their radical scavenging and therapeutic attributes have recently reignited scientific interest, particularly in the exploration of plant extracts rich in chalcones for their potential health benefits.3,4
Chalcones have been found to be distributed in various portions of a plant, especially in seeds, rhizomes, leaves, and roots as well. Due to their conjugated bond structure, they exist as yellow- or orange-coloured pigments.5,6 Chalcones and their derivatives have been linked to a wide range of biological activities, and their chemistry is really fascinating and attracts researchers for exploiting their potential and role as health beneficial compounds.7–10 Published studies suggest that chalcones and their derivatives have a potential role in a diverse array of biological effects, such as antifungal, anticancer, antimalarial, antibacterial, antioxidant, antidiabetic, antihypertensive, neuroprotective, antiobesity, and anti-inflammatory effects, and they have been used in traditional medicine.11–16 The effectiveness of naringenin chalcones in addressing obesity is evident through their ability to enhance metabolic functions in adipocytes.
Chalcones are multifunctional molecules that display a range of biological activities through their single structural framework Fig. 1. For example, licochalcone A enhances the potent antioxidant effects of licorice and also acts as a bactericidal, antileishmanial, antimalarial, and anti-inflammatory agent and as a cell cycle inhibitor.17,18 Chalcones are frequently used as food additives in the food industry because of their stability, colorlessness, safety for eating, and ease of production through catalytic hydrogenation of flavanones and glucosides. Additionally, several studies5,19,20 have shown that both natural and synthetic chalcones are non-toxic to normal cells and have significant pharmacological capabilities, and chalcone derivatives with slight chemical modifications could provide a solid foundation for drug discovery based on natural products, leading to promising drug lead candidates.
 |
| Fig. 1 General framework of chalcone derivatives. | |
Chalcones have been considered as an integral part of traditional herbal medicine, and they have been utilized in treating important disorders like cancer, viral and cardiovascular diseases for a long time. The extraction of chalcones and their compounds from natural sources typically employs various solvents, so choosing an appropriate solvent and the amount of solvent are crucial for efficient extraction. The current review aims to offer an extensive overview of the natural sources of chalcones, the methods employed for their extraction, and the key mechanisms behind their pharmacological actions. The goal is to illuminate the multifaceted nature of chalcones as promising compounds in the realm of drug development and natural health solutions.
2 Sources of chalcones
It has been reported that many plants are natural sources of chalcones such as tomato, apple, licorice and fingerroot.
2.1 Tomatoes
Chalcones, particularly naringenin chalcone and its conjugates, are frequently found in tomatoes (Solanum lycopersicum) and thus possess various health benefits. In fruits and vegetables, chalcones can be found in the form of naringenin chalcone, which is only present in tomato skin (5–10 mg kg−1 fresh weight). Their occurrence in tomatoes depends upon the stage of ripening of fruits. The highest concentrations are found at the turning stage, and a sharp decline occurs during ripening. To put it another way, chalcones are not visible in the green peel, but their concentration increases sharply when tomatoes turn red. In addition to naringenin, typical flavonoids like quercetin, kaempferol, naringenin, and rutin are also found in tomatoes. Furthermore, eriodictyol chalcone and dihydroxy chalcones21 are chalcone conjugates that have been discovered in tomatoes. Tomatoes have been shown to improve persistent allergic rhinitis, and lower the prevalence of cancer, diabetes, cardiovascular disease, and diabetes. Naringenin chalcone, extracted from tomatoes, has demonstrated potent antiallergic properties by inhibiting histamine release. Notably, among all the polyphenols identified in tomato skin extracts, this chalcone has been the most effective. Other flavonoids in the extract did not show similar inhibitory activities.22 Research studies on chemical structure alterations to develop naringenin chalcone derivatives have been taken advantage of due to the intriguing biological profile of this chalcone. Additionally, this component can reduce allergic asthmatic airway inflammation by preventing the spleen's CD4 T cells from producing the Th2 cytokine.
2.2 Apples
Apples contain a lot of polyphenols, including dihydrochalcones, hydroxycinnamates, anthocyanins, and flavonoids.23 A number of good antioxidants are found in apples which are effective in preventing several cardiovascular diseases.24 Dihydrochalcones (DHCs), a type of polyphenol, predominate among them and accumulate in apple roots, shoots, leaves, and fruits. DHCs are uncommon in edible plants and are frequently found in various medicinal plants across different botanical families, showing a diverse distribution within the plant kingdom. The presence of DHCs in plants has been extensively analyzed in a recent review by Riviere.25 Dihydrochalcones accumulated in apple tissues, such as the skin, pulp, and in particular the core and seeds that are typically destroyed when the apple is ingested fresh, make them toxic to humans. Due to the processing of the entire apple fruit, it has been suggested that the overall intake of these chalcones is higher for products made from apples like juice, cider, and pomace. Phloretin and its conjugates, phloridzin and phlorizin, are the primary dihydrochalcones present and make up to 90% of the soluble polyphenols discovered in apple tree leaves.26 The cultivar affects the amount of phloridzin in apples significantly. Phloridzin, a glucoside of phloretin, is also known as phloretin-2′-β-D-glucopyranoside. These components have demonstrated a range of biological activities, including antioxidants, anti-inflammatory agents,27 antimicrobials,28 anticancer agents,29 and the prevention of cardiovascular diseases.
2.3 Licorice
One species of legume that is abundant in bioactive chalcones is licorice (Glycyrrhiza glabra), often known as the liquorice plant. This plant is frequently used to make medicinal items like hematinic tablets and cough expectorants to cover up the unpleasant taste of other medications. Additionally, Chinese traditional medicine frequently prescribes licorice roots for a number of diseases, including gastric ulcer, pneumonia, fever, and sore throat. As of now, approximately 56 chalcones have been isolated and identified from licorice, with 14 exhibiting antitumor properties. Despite this, the precise molecular mechanisms underlying the anticancer effects of chalcones remain unclear.30 Triterpene saponins, flavanones, and chalcones with a variety of conjugates are the main metabolites found in licorice roots.31 Licorice roots contain a sizable amount of the triterpene saponin glycyrrhizin which is responsible for a variety of pharmacological actions, including hepatoprotective, antiviral, anticancer, and anti-inflammatory activities.32 A recent study highlighted that chalcones effectively inhibit h3β-HSD2 and r3β-HSD1, suggesting their potential as therapeutic agents for conditions such as polycystic ovary syndrome or Cushing's syndrome. The primary components of licorice are the glucosides of flavanone (liquiritigenin), 2′-hydroxychalcone (iso-liquiritigenin), and its isomer. Iso-liquiritigenin has demonstrated promising chemopreventive properties in anticancer trials, including the capacity to control cell growth and block ErbB3 signaling in DU145 prostate cancer cells. This compound has also shown significant antioxidant benefits by lowering myocardial reactive levels of reactive oxygen species (ROS) in ischemia damage via activation of AMPK and pathways for ERK signaling.33 Examples of licochalcones, which make up the majority of the chalcones found in the licorice plant, are licochalcones A, B, C, D, and E. These chalcones, particularly licochalcone A, have been shown to have a range of biological activities, including anti-inflammatory, antileishmanial, antibacterial, anticancer, antidiabetic, antiviral, and antileishmanial properties.34
2.4 Fingerroot
Chinese keys and Chinese ginger are other names for the tropical plant known as fingerroot (Boesenbergia rotunda). In Thailand, it is known as Krachai and is frequently used as a spice in Thai foods. Species from this family's rhizomes and leaves have long been utilized in traditional medicine and are frequently linked to antioxidant capabilities. There have been numerous studies reporting on their pharmacological activities, including their anticancer, anti-inflammatory, antiallergic, and antibacterial effects.35 Boesenbergin A, cardamonin, 4-hydroxypanduratin A, panduratin A, pinocembrin chalcone, and pinostrobin chalcone are the bioactive chalcones discovered in fingerroot. Traditional users of the fingerroot plant have identified its aphrodisiac effects to be mediated through the chalcones of boesenbergin A, pinostrobin, and panduratin.35 In fingerroot, panduratin A and its 4-hydroxypanduratin A derivative with a cyclohexene moiety are present in comparatively large proportions. These chalcones have been linked to a number of biological activities, including anti-inflammatory, antioxidant, antibacterial, and antiobesity characteristics. Furthermore, via inhibiting COX-2 enzymes, these chalcones are also thought to have anti-inflammatory properties.36 Due to its anticancer, antipyretic, and protease inhibitory properties, another chalcone, cardamonin, has received a lot of interest. Recently, in vitro research has confirmed the anticancer properties of B. rotunda, demonstrating that extracts from the plant are highly cytotoxic and can induce apoptosis in breast cancer cell lines.37
3 Extraction of chalcones
3.1 Conventional methods
The classic and conventional methods of extraction of chalcones are totally based on solvents. The most effective and robust methods to extract chalcones are liquid–liquid extraction methods which are still being used in the extraction and isolation of chalcones.38 The fundamental processes include infusion, decoction, maceration, percolation, Soxhlet, and reflux extraction. Traditionally, methanol enriched solvents were used to soak the plant material overnight, the obtained crude extract was loaded onto the silica gel filled column and then the column was eluted with a series of solvents and solvent combinations, viz., acetone, ethanol, methanol, chloroform, dichloromethane, ethyl acetate, and hexane based on the polarity of the interested chalcones and their related compounds.39 During the elution process, similar color fractions were mixed and allowed to reconstitute, and then thin layer chromatography (TLC) and cellulose paper chromatography were used for the characterization and confirmation of chalcone compounds.40 In most of the cited literature studies, the presence of yellowish colored fractions was considered a sign for the existence of chalcone related compounds in those fractions,41 so those fractions were then used for purification through the recrystallization process, and reconfirmation was done by checking melting points and other physical properties like texture, color, solubility, etc.42 In general, the traditional extraction methods for natural plant products are still being used and these techniques cannot be replaced or removed completely. Some of the old methods are presented in Table 1.
Table 1 Common traditional methods/techniques used in extraction of chalcones
Compounds |
Method of extraction |
Solvents used |
Sources |
Characterization technique |
References |
Marein 1 |
Hot extraction |
Ethanol–EtOAC |
Ray flowers of Coreopsis tinctoria |
Recrystallisation and paper chromatography |
Shimokoriyama43 1957 |
Chalcone (4,2′,4′-trihydroxy) (I) |
Hot extraction |
Butanol–H2O–ethanol–phenol |
Flowers of gorse, Ulex europaeus |
Paper chromatography |
Harborne41 1962 |
Flavan-3,4-diol |
Paper ionophoresis |
Butan-2-ol and acetic acid |
Acacia auriculiformis
|
2D chromatography, color reactions, NMR |
Drewes and Roux44 1966 |
Triangularin |
Polyamide TLC |
CHCl3–MeOH–MeCOEt |
Exudate of Pityrogramma triangularis |
UV light and UV spectroscopy |
Star et al.,40 1978 |
2′-3-Dihydroxy-4-methoxy-4′-glucosyl chalcone |
Co-chromatography |
MeOH, CHCl3, and EtOAc |
Megaoldonta beckii
|
UV spectroscopy and Rf values |
Roberts45 1980 |
Boesenbergin A |
Column chromatography |
CHCl3 |
Boesenbergia pandurata
|
Infrared spectroscopy and NMR |
Isa et al.,36 2012 |
Quinoidal |
Cellulose anion exchange |
MeOH–hexane |
Aqueous extracts of Dyer's saffron flowers |
UV spectroscopy |
Saito and Fukushima46 1989 |
Helichrysetin |
Percolation and Si gel column chromatography |
Petroleum ether, CHCl3, EtOAc, hexane, H2O, and MeOH |
Dried flowers of Helichrysum odoratissimum |
1H NMR |
Van et al.,47 1989 |
4′,6′-Dihydroxy-2′-methoxy-3′,5′-dimethyl chalcone |
Si gel chromatography |
MeOH, CHCl3, BuOH, and H2O |
Syzygium samarangense
|
1H NMR, IR, and ESI-MS |
Srivastava et al.,48 1995 |
3.2 Advanced methods
The most updated and new methods being used in extraction of natural products including flavonoids are microwave assisted, accelerated solvent, ultrasound assisted, ionic liquid, solid phase, and supercritical fluid extraction. Some of the methods and techniques are depicted in Table 2.
Table 2 New methods/techniques used in extraction of chalcones
Compounds |
Method of extraction |
Solvents used |
Sources |
Characterization technique |
References |
Chalcones |
Solid phase microextraction |
Solution of aqueous NaCl and acetic acid |
Honey samples |
GC-MS |
Daher and Gulacar49 2008 |
(E)-1-(2′,4′-Dihydroxy, 5′-methoxy, 3′-methylphenyl)-3-phenylprop-2-en-1-one |
Silica gel column chromatography |
Hexane, dichloromethane, ethyl acetate and methanol |
Leaves of Heteropyxis natalensis |
IR, NMR, HR-ESI-MS |
Adesanwo et al.,50 2009 |
Bioactive compounds |
Liquid–liquid extraction, Sephadex LH-20 column |
Ethanol, hexane, and CHCl3 |
Brazilian propolis
|
1H NMR, 13C NMR, UV, and mass spectrometry |
Oldoni et al.,51 2011 |
Chalcone fractions |
Silica gel column chromatography |
Chloroform–acetone gradient |
Roots of Codonopsis cordifolioidea |
HPLC |
Meng et al.,52 2013 |
Chalcones |
Supercritical CO2 fluid extraction |
Dichloromethane and liquid CO2 |
Citri Reticulatae pericarpium |
Ultra-high-performance liquid chromatography–Q Exactive Orbitrap tandem mass spectrometry |
Zheng et al.,53 2020 |
Chalcone-rich extracts |
Silica gel column chromatography |
Hexane, dichloromethane and methanol |
Roots of Lonchocarpus cultratus |
1H NMR |
Staffen et al.,54 2022 |
Flavonoid group |
QuEChERS method |
Acetonitrile–acetic acid |
Apple and grape juice |
UHPLC/Q Orbitrap ESI-MS |
Wang and Chow55 2022 |
Dietary polyphenols |
Sorbent-based micro-extraction technique |
Solvent free |
Food samples |
UHPLC-IT-TOF-MS/MS |
Casado et al.,56 2020 |
3.2.1 Ultrasound assisted extraction.
Ultrasound assisted extraction (UAE) is a good technique to extract highly valuable compounds of natural products. It has advantages like usage of less energy and being operable at low to medium temperature which is good for heat sensitive molecules. To get a higher efficiency of UAE, some key points need to be considered, including the frequency, the reactor characteristics, ultrasonic power, the extraction temperature, and the solvent–sample interaction. The extraction efficiency can be increased in UAE by using 40% ethanol as the solvent, and it is more efficient than enzyme assisted extraction, use of microwave and heating.57
3.2.2 Microwave assisted extraction.
The basic principle in the microwave heating process is induction of dipole rotation in organic molecules that cause hydrogen bonding; this causes the traffic of ions which results in a heating effect due to increased kinetic energies of ions as well as the friction between ions due to their continuous movements and changes in direction. The efficiency of microwave assisted phytochemical extraction could be dependent on several factors like the frequency and power of microwave, moisture content, microwave radiation exposure time, sample type, particle size, behavior and amount of solvent used, proportion of solute to solvent, extraction pressure, extraction temperature, and number of extraction cycles.58
3.2.3 Ionic liquid assisted extraction.
The use of ionic liquids (ILs) as diluents or extractants in solvent extraction is basically referred to as IL assisted extraction. It is the latest and novel technique in separation science. The unique properties of ILs are low combustibility, high thermal stability, low vapor pressure, and wide liquid range, which make them a better substitute for volatile compounds in solvent extraction.59 Recently, imidazolium based ILs were applied as new solvents to extract bioactive glabridin from licorice and flavonoids from grape skin.60,61
3.2.4 Accelerated solvent extraction.
Accelerated solvent extraction (ASE) is known as green technology of extraction, and basically it is based on the use of pressurized hot water in the extraction process for bioactive compounds from the plants. Several reports are available in which ASE is applied to extract phenolic compounds.62,63 Very recently, the accelerated solvent extraction (ASE) method was compared to conventional ultrasonic-assisted extraction (UAE) and solvent extraction (CSE) methods, and interestingly the ASE was found to be more appropriate and efficient among all three methods for the extraction of anthocyanin and total phenolic compounds.64
3.2.5 Supercritical fluid extraction.
Supercritical fluid extraction (SFE) operates based on the concept of dissolving target compounds in the solvent's liquid phase and then transitioning the solvent to a gaseous state to facilitate component separation. SFE is particularly useful in the pharmaceutical industry for isolating specific phytochemicals from plants, such as volatile oils. Recently, SFE was used to extract the chalcone pinostrobin, along with combinations of volatile and non-volatile compounds.65
3.2.6 Solid phase micro-extraction.
Solid phase micro-extraction (SPME) is a sophisticated extraction technique. It was designed to address certain limitations of solid phase extraction (SPE), such as reducing the extraction time and the consumption of organic solvents. SPME is a solvent-free sample preparation method that utilizes a fused silica fiber coated with a suitable stationary phase, which is connected to a modified micro-syringe.56 The use of SPME to determine aromatic compounds and other phenolics is well known and getting more popular day-by-day.49
4 Biological activities of chalcones
Chalcones are a superabundance of biologically active compounds in natural products. Synthetic modifications and natural isolates of chalcones are being explored globally for the development of more potent molecules for various diseases. Chalcones, having an α, β-unsaturated carbonyl moiety, exhibited various pharmacological activities due to their multiple mechanisms.66 Chalcones, which belong to the flavonoid family, broadly occur in nature in the form of pigments and are phenolic group compounds. These are one of the dominant categories of natural products and are extensively distributed in vegetables, fruits, tea and spices. Chalcones, presumed to be precursors of flavonoids as well as isoflavonoids, are present in ample amounts in edible plants and exhibit multiple arrays of pharmacological activities.67 Chalcones have demonstrated remarkable biological characteristics and a low toxicity profile. According to several researchers, the removal of the keto-ethylenic group reduces biological activities, which supports the theory that the presence of this group is the primary contributor to these biological activities. In the absence of nerve growth factor chalcones like helichrysetin, flavokawain-C and xanthohumol alone had no effect on neurite outgrowth. In the presence of nerve growth factor (5 ng mL−1), helichrysetin and xanthohumol at the concentration of 10 μg mL−1 have effect on neurite outgrowth and neurite bearing cells growth was found 30.2 ± 5.9% and 44.3 ± 3.2% respectively.68
Chalcones have aroused interest due to their radical properties suppression of phenolic groups which is used in food preservation and in the development of drugs.69 There are several reports available which indicate that chalcones have various useful properties such as antifungal, anti-inflammatory, cytotoxic, antioxidant, antitumor and anticancer properties (Table 3).14,69,70 A phenolic substance called isoliquiritigenin, which is present in liquorice, has been used in a number of illnesses. Liquorice (Glycyrrhiza glabra) is enriched in natural chalcones and has been widely used in China for curing various diseases such as gastric problems, duodenal ulcer, bronchial asthma, Addison's disease, and skin problems like eczema.80 The benefits of natural dietary chalcone butein in nutraceuticals are widely recognized.81 In Eastern countries, for instance, Japan, China and Korea, butein has been conventionally used for curing stomach cancer, gastritis, pain and various parasitic infections.82 Chalcones are presumed to be the precursors of flavonoids in the biosynthetic pathways (Fig. 2).
Table 3 Biological activity of chalcones from natural sources
Chalcones |
Source |
Biological activities |
Reference |
Xanthohumols and dihydroxanthohumol |
Mallotus philippinensis (fruit) |
Anti-inflammatory |
Zhao et al.,71 2005 |
Licochalcone A |
Liquorice root |
Multiple pharmacological activities |
Chen et al.,72 1994 |
Prenylbutein and homobutein |
|
Antiplasmodial |
Yenesew et al.,73 2004 |
Crotaorixin |
Crotalaria orixensis
|
Antimalarial |
Narender et al.,74 2005 |
Medicagenin |
C. medicagenia root |
Multiple pharmacological activities |
Narender et al.,74 2005 |
Xanthohumol |
Humulus lupulus
|
Multiple pharmacological activities |
Frolich et al.,75 2005 |
Isobavachalcone |
Psoralea corylifolia Linn (seed) |
Multiple pharmacological activities |
Wang et al.,76 2021 |
Aureusidin |
Soybean |
Antioxidant |
Detsi et al.,77 2009 |
Licochalcone B and D |
Roots of Chinese licorice |
Antioxidant and chemopreventive activity |
Pastorino et al.,78 2018 |
Garcinol |
Garcinia indica
|
Antioxidant |
Padhye et al.,79 2009 |
Isoliquiritigenin |
Glycyrrhiza glabra
|
Anticancer properties |
Wang et al.,80 2020 |
Butein |
Rhus verniciflua
|
Anticancer properties |
Semwal et al.,81 2015 |
 |
| Fig. 2 Flowchart depicting the biosynthetic pathway of chalcone, isoflavonoids and anthocyanidins. | |
4.1 Antioxidant properties
Most of the naturally occurring chalcones are polyhydroxylated in the phenyl ring. Due to the presence of phenolic moieties in chalcones, chalcone enriched plant extracts have been of interest as antioxidants for drugs or food preservatives.83,84 For the protection of human health, antioxidants are essential. The relationship between chalcones' antioxidant activity, cytotoxicity against tumour cells, and chemopreventive characteristics is well known. Vanadium complexes with 1-(1-hydroxy-naphthalen-2-yl)-3-phenyl-propenone and 2′-hydroxychalcone have been synthesized and assessed for their antiradical properties.85 Among these, the vanadium oxychloride complex with 2′-hydroxychalcone demonstrated the highest efficacy in scavenging DPPH free radicals, with an IC50 of 0.03 μg mL−1. Licochalcone B and D, isolated from roots of Chinese licorice, exhibited excellent antioxidant activity.78 According to Padhye et al.,79 garcinol is a powerful antioxidant; however, new data suggest that garcinol might be effective as a cancer preventative agent.
4.2 Antimicrobial properties
The antibacterial properties of chalcones are currently being gradually documented. Several researchers have not only isolated but also recognized the structures of various chalcones and/or modified natural chalcones having antibacterial properties.86,87 The antibacterial properties of these compounds are attributed to the α, β-unsaturated ketone group, which causes toxicity through interaction with cellular nucleophiles, such as the thiol groups in proteins, and forms adducts with proteins that play important roles in cellular processes.88 For instance, liquorice is used in the pharmaceutical, cigarette, and confectionery sectors. Among different retro-chalcones, licochalcone A and licochalcone C, isolated from Glycyrrhiza inflata, exhibited excellent antibacterial activity, particularly against Staphylococcus aureus, Bacillus subtilis and Micrococcus luteus89 (Fig. 3A). Recently, the antibacterial activity of isoliquiritigenin was reported against Staphylococcus epidermidis and S. aureus (MIC = 250 μg mL−1).90 Pinocembrin chalcone also exhibited good activity against S. aureus.91 Asebogenin (dihydrochalcone), which is present in the ethanolic fractions of Piper longicaudatum leaves and twigs, inhibited the growth of S. aureus (IC50, 10 μg mL−1).92 The chalcone isolated from Dalea versicolor showed efficacy against the human pathogen S. aureus both on its own and in combination with other antibiotics.86 Fluorinated chalcone-1,2,3-triazole was synthesized and tested against S. epidermidis, E.coli, B. subtilis and P. aeruginosa. The results revealed that it is better than the standard drug ciprofloxacin (MIC: 0.0047 μmol mL−1) against E. coli and S. epidermidis with a minimum inhibitory concentration (MIC) of 0.0032 μmol mL−1.93 Recently, triazolyl bearing chalcones were synthesized and tested against S. aureus, M. luteus, B. typhi, B. subtilis, P. aeruginosa, C. mycoderma and B. proteus. They displayed maximum activity against C. mycoderma (MIC: 8 μg mL−1) and M. luteus (MIC: 4 μg mL−1), which are comparable to or better than the control drugs such as chloromycin and norfloxacin.94
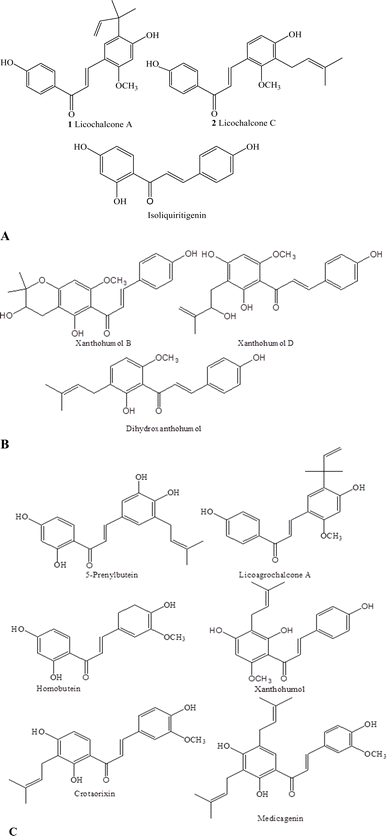 |
| Fig. 3 Natural chalcones possessing (A) antibacterial properties, (B) anti-inflammatory activities and (C) antimalarial activities. | |
4.3 Anti-inflammatory activity
Prostaglandin E2 (PGE2) and nitric oxide (NO) suppression is a potential treatment for a variety of inflammatory disorders. The tissues are damaged due to an excessive amount of NO production. Numerous chalcone compounds showed NO and PGE2 inhibitory effects.71,95 Mallotophilippens C, D, and E, as well as xanthohumols and dihydroxanthohumol, which were extracted from Mallotus philippinensis fruits, were novel chalcone derivatives that inhibited NO formation (Fig. 3B). Also, nuclear factor κB (NF-κB) was suppressed by these compounds.71 Additional insights into how natural chalcones suppress NF-κB-mediated cancer and inflammation are discussed in various reviews on the subject.66,88 A range of 2′-hydroxy- and 5′-dihydroxychalcones were synthesized and evaluated in vitro for their capacity to inhibit the activation of various immune cells, including microglial cells, macrophages, mast cells and neutrophils. The compound 2,2′-dihydroxychalcone was reported as an effective agent in suppressing the release of β-glucuronidase and lysozyme from rat neutrophils. Additionally, 2′,5′-dialkoxychalcones demonstrated significant inhibitory effects on the production of nitric oxide by LPS-stimulated murine microglial cells of the N9 cell line.96
4.4 Antimalarial activity
Chinese liquorice roots contain licochalcone A which has exhibited excellent activity against P. falciparum strains.72 Prenylbutein, licoagrochalcone A and homobutein exhibited antiplasmodial activity (IC50: 10.3–16.1 μM) against P. falciparum73 (Fig. 3C). The aerial portion of the Crotalaria orixensis plant contains crotaorixin, which showed an outstanding inhibitory effect against P. falciparum strain. Medicagenin, a diprenylated compound, isolated from C. medicagenia roots, showed 100% inhibition at 2 μg mL−1, while the chromeno-dihydrochalcones, from C. ramosissima, showed lower activity at the same concentration.74 The Humulus lupulus (hops; Family: Cannabaceae) plant has prenylated chalcone derivatives and exhibits antiplasmodial activity. Xanthohumol, a main hop chalcone, exhibits activity against P. falciparum.75
4.5 Antitumor activity
Chalcones are one of the precursors of all flavonoids and have many biological activities, including antitumor,11,97 antimalarial and anti-inflammatory activities. Research on the structure–activity relationship (SAR) indicates that the inclusion of methoxy and hydroxy groups on rings A and B of chalcones is crucial for their antioxidant properties.77,98 Natural chalcones, such as xanthohumol, loncocarpine, licochalcones, and panduretin, have been comprehensively studied and moderated.99 Some prime examples of compounds isolated from natural sources include isoliquiritigenin, licochalcone A, isobavachalcone and butein known for their potential anticancer activity. Isoliquiritigenin, a bioactive compound with a chalcone structure, is extracted from the roots of the licorice plant and has shown promising inhibitory activity against various cancers such as colon, breast, stomach, lung, and ovarian cancers, leukemia, and melanoma. Butein obtained from the bark of Rhus verniciflua contains a physiologically active flavonoid that exhibits anticancer properties in a variety of cancers.100 Different studies show that licochalcone A induces the apoptosis of epithelial ovarian carcinoma cells, U87 glioma cells, bladder cancer cells and nasopharyngeal cancer cells. Also, chalcones have the ability to enhance autophagy and in breast cancer cells block the cell cycle. Furthermore, they suppress the specific protein 1 in breast cancer and induce apoptosis.101 By generating Reactive Oxygen Species (ROS) that are dependent on endoplasmic reticulum stress, an apoptotic pathway can be activated both in vivo and in vitro.4
4.6 Neuroprotective effect
Dopamine is degraded in the presence of a Mg2+ cofactor by the catechol-O-methyltransferase (COMT) protein, and the catalytic site of the enzyme is completed with Asp141, Lys144, Asp169, Asn179, Glu199, and the SAM (S-adenosylmethionine) fragment.102 A study revealed that the chalcone derivatives through metal–donor interactions can bind to Mg2+ and by non-covalent bonding stabilize the chalcone–COMT complexes. In this adduct, several conventional H-bonds are also found with key residues (Lys144, Asn169, and Asp170) in the catalytic site and some alkyl and π-bonds also contribute to the stabilization of complexes. The coordination bond between chalcone derivatives and Mg2+ significantly affects the binding energy, and this conformation is most stabilized. Also, other studies have found that the chalcone derivatives have a stronger interaction with COMT than dopamine and levodopa. Therefore, the chalcone derivatives can both potentially protect them from COMT induced degradation and prevent their depletion. Monoamine oxidase B (MAO-B) enzyme is associated with deamination of alkylamines, and malfunction of this enzyme has been associated with depression conditions. Its malfunction also causes ADHD (attention deficit hyperactivity disorder). The chalcone derivative dCHA-553 through the π-forces presents the strongest interaction with the MAO-B enzyme, and shows inhibitor activity than the parent molecule for the MAO-B enzyme.103 In Parkinson's disease, the role of the MAO-B enzyme is not clear, but it is well known that the enzyme inhibitors help nerve cells make better use of the neurotransmitters, and chalcones being the inhibitor of MAO-B may help in better use of neurotransmitters in Parkinson's disease. In Alzheimer's disease, the loss of cholinergic neurons occurs in the brain, and AChE is responsible for the hydrolysis of acetylcholine neurotransmitters. The inhibitor of AChE downregulates the acetylcholine degradation in the synaptic clefts. The chalcone derivatives form adducts with the AChE via intermolecular forces to form the stable adducts and lead to the loss of esterase activity.104 The chalcones have high inhibition potential because with this enzyme they present high stabilization energy and at a nanomolar range have an estimated inhibition constant. Nevertheless, the best estimated inhibition effect was shown by donepezil. Thus, the results show that the chalcone might function as an acetylcholine protector and inhibit the action of AChE. 1-Hydroxynaphthalene-4-trifluoromethylphenyl chalcone has been synthesized and evaluated for its inhibitory activity against acetylcholinesterase (49.76 μg mL−1) and butyrylcholinesterase (77.82 μg mL−1). Docking studies have shown that this chalcone binds to the catalytic active site of acetylcholinesterase105 and inhibits the cholinesterase activity, which may help in the prevention of neurodegenerative damage. In beta-amyloid plaques, for confirmation of the binding affinity in the mouse brain, a fluorescence study was carried out. Intense staining of beta-plaques by the compounds 5-iodo-2-thienyl and 4-dimethylaminophenyl was observed. Also, in cerebrovascular amyloids, clear staining was observed. These suggest that these compounds detect beta-plaques in the brain. Furthermore, an inhibition study was conducted to know the information of the binding site of the chalcones and chalcone like compounds. Congo red (CR) and thioflavin T (ThT) were used for the inhibition study, and these are prototypes of amyloid imaging probes. 5-Iodo-2-thienyl and 4-dimethylaminophenyl compounds competes to 4-dimethylamino-40-iodo-chalcone for binding to Ab aggregates. This study suggests that chalcone like compounds show antiamyloid activity but with different binding sites compared to CR and ThT on Ab aggregates.106
5 Toxicity of chalcones
Results showed that embryos treated with 3′-hydroxychalcone (3 ppm) displayed a high percentage of muscle defects (96.6%), especially myofibril misalignment.107 In addition, the preferential reactivity with thiols in contrast to amino and hydroxyl groups makes these molecules less toxic than other therapeutic cancer drugs.108 At very low and non-toxic concentrations, tube formation and human brain endothelial cell migration were inhibited by flavokawain B chalcone. Moreover, at a concentration of 2.5 μg mL−1, it did not show any toxic effect, but in a dose dependent manner it blocked the angiogenesis process in zebrafish and reduced the subintestinal vein formation.109 Synthetic chalcones like (E)-2-(40-methoxybenzylidene)-1-benzosuberone in non-toxic concentrations inhibited VEGF induced migration of HUVECs. Also, they decreased the secretion of VEGF and MMP-9 at the same time.110 Similarly, 4-hydroxychalcone (22 mg mL−1), without showing any sign of cytotoxicity, suppressed the angiogenesis steps like proliferation, migration, and tube formation. Further, in vivo studies showed that at concentrations of 25 mg per kg per day and 50 mg per kg per day for 25 days it could inhibit breast cancer and neo-angiogenesis.111
6 Conclusion
Chalcone scaffolds are vital bioactive precursors to plant flavonoids and possess significant chemical and biological potential, which are essential in the fields of medicinal chemistry and biology. The chemical and biological relevance of naturally occurring chalcones is yet to be fully explored. While these compounds have widespread medicinal uses, comprehending their pharmacokinetics poses a considerable challenge. But there is limited preclinical or clinical research on naturally occurring chalcones in the existing literature. This study highlights the applications of chalcones, with a focus on their critical biological activities both in vitro and in vivo, including antibacterial, antioxidant, antitumor, antimalarial, and anti-inflammatory effects. It calls for more thorough research to tackle the biological activities and toxicological challenges associated with naturally occurring chalcones and their flavonoid derivatives. Additionally, further clinical investigations are essential to decipher their action mechanisms at the cellular level and link their structural features to biological effects, particularly regarding anticancer activity. The identification of lead molecules or drug candidates from bioactive chalcones found in nature holds great promise. Therefore, strategic chemical modifications of natural chalcones are essential to create new flavonoid molecules, potentially making a significant impact on drug discovery utilizing chalcone frameworks.
Data availability
The data that support the findings of this study are available from the corresponding author upon reasonable request.
Author contributions
MKS contributed to the organization, writing, and editing of the manuscript. DKY, PK, MK and MK wrote sections of the manuscript. PM, KT, SSS, HR, and SK reviewed the manuscript. All authors contributed to the article and approved the submitted version.
Conflicts of interest
The authors declare that the research was conducted in the absence of any commercial or financial relationships that could be construed as a potential conflict of interest.
References
- M. Chatzopoulou, K. Pegklidou, N. Papastavrou and V. J. Demopoulos, Development of aldose reductase inhibitors for the treatment of inflammatory disorders, Expet Opin. Drug Discov., 2013, 8, 1365–1380 CrossRef CAS PubMed.
- R. Maccari and R. Ottana, Targeting aldose reductase for the treatment of diabetes complications and inflammatory diseases: new insights and future directions, J. Med. Chem., 2015, 58, 2047–2067 CrossRef CAS PubMed.
- M. N. Gomes, E. N. Muratov, M. Pereira, J. C. Peixoto, L. P. Rosseto, P. V. Cravo, C. H. Andrade and B. J. Neves, Chalcone derivatives: Promising starting points for drug design, Molecules, 2017, 22, 1210 CrossRef PubMed.
- Y. Ouyang, J. Li, X. Chen, X. Fu, S. Sun and Q. Wu, Chalcone Derivatives: Role in Anticancer Therapy, Biomolecules, 2021, 11, 894 CrossRef CAS PubMed.
- N. K. Sahu, S. S. Balbhadra, J. Choudhary and D. V. Kohli, Exploring pharmacological significance of chalcones scaffold: A review, Curr. Med. Chem., 2012, 19, 209–225 CrossRef CAS PubMed.
- B. Evranos Aksöz and R. Ertan, Chemical and structural properties of chalcones I, Fabad J. Pharm. Sci., 2011, 36, 223–242 Search PubMed.
- N. G. Marcelo, N. M. Eugene, P. Maristela, C. P. Josana, P. R. Lucimar, V. L. C. Pedro, H. A. Carolina and J. N. Bruno, Chalcone Derivatives: Promising Starting Points for Drug Design, Molecules, 2017, 22, 1210 CrossRef.
- R. M. Martinez, F. A. Pinho-Ribeiro, V. S. Steffen, C. V. Caviglione, V. Fattori, A. J. Bussmann, C. Bottura, M. J. Fonseca, J. A. Vignoli and M. M. Baracat,
et al., Trans-Chalcone, a flavonoid precursor, inhibits UV-induced skin inflammation and oxidative stress in mice by targeting NADPH oxidase and cytokine production, Photochem. Photobiol. Sci., 2017, 16, 1162–1173 CrossRef CAS PubMed.
- J. Khan, P. K. Deb, S. Piya, K. D. Medina, R. Devi, S. G. Walode and M. Rudrapal, Dietary Flavonoids: Cardioprotective potential with antioxidant effects and their pharmacokinetic/therapeutic concerns, Molecules, 2021, 26, 4021 CrossRef CAS PubMed.
- S. Singh, A. Sharma, R. Reddy and M. K. Samota, Eco-Friendly Processing of Momordica Charantia L. Based Chemical Free Functionally Enriched Nectar And Evaluation of Its Nutritional Profile, Bangladesh J. Bot., 2022, 51(3), 445–453 CrossRef.
- A. Sharma, B. Chakravarti and M. P. Gupt, Synthesis and anti-breast cancer activity of biphenyl based chalcones, Bioorg. Med. Chem., 2010, 18, 4711–4720 CrossRef CAS PubMed.
- Z. H. Zhang, L. J. Yu and X. C. Hui,
et al., Hydroxy-safflor yellow A attenuates Abeta(1)(_)(4)(2)-induced inflammation by modulating the JAK2/STAT3/NF-kappa B pathway, Brain Res., 2014, 1563, 72–80 CrossRef CAS PubMed.
- K. Zhou, S. Yang and S. M. Li, Naturally occurring prenylated chalcones from plants: Structural diversity, distribution, activities and biosynthesis, Nat. Prod. Rep., 2021, 38, 2236–2260 RSC.
- B. Salehi, C. Quispe, I. Chamkhi, N. El Omari, A. Balahbib, J. Sharifi-Rad and F. Les, Pharmacological properties of chalcones: a review of preclinical including molecular mechanisms and clinical evidence, Front. Pharmacol, 2021, 11, 592654 CrossRef.
- Y. Tak, M. Kaur, M. C. Jain, M. K. Samota, N. K. Meena, G. Kaur and R. Amarowicz, Jamun Seed: A Review on Bioactive Constituents, Nutritional Value and Health Benefits, Pol. J. Food Nutr. Sci., 2022, 72(3), 211–228 CrossRef CAS.
-
S. Tekale, S. Mashele, O. Pooe, S. Thore, P. Kendrekar and R. Pawar, Biological Role of Chalcones in Medicinal Chemistry, Intech Open, 2020, DOI:10.5772/intechopen.91626.
- A. Friis-Møller, M. Chen, K. Fuursted, S. B. Christensen and A. Kharazmi, In vitro antimycobacterial and antilegionella activity of licochalcone A from Chinese licorice roots, Planta Med., 2002, 68(05), 416–419 CrossRef PubMed.
- J. I. Furusawa, M. Funakoshi-Tago, T. Mashino, K. Tago, H. Inoue, Y. Sonoda and T. Kasahara, Glycyrrhiza inflata-derived chalcones, Licochalcone A, Licochalcone B and Licochalcone D, inhibit phosphorylation of NF-κB p65 in LPS signaling pathway, Int. Immunopharm., 2009, 9(4), 499–507 CrossRef CAS.
- M. Utsintong, A. Massarotti and A. Caldarelli,
et al., Parallel synthesis of “click” chalcones as antitubulin agents, Med. Chem., 2013, 9, 510–516 CrossRef CAS PubMed.
- M. K. Samota, M. Sharma, K. Kaur, Sarita, D. K. Yadav, A. K. Pandey, Y. Tak, M. Rawat, J. Thakur and H. Rani, Onion anthocyanins: Extraction, stability, bioavailability, dietary effect, and health implications, Front. Nutr., 2022, 9, 917617 CrossRef PubMed.
- A. Vallverdú-Queralt, O. Jáuregui and G. Di Lecce,
et al., Screening of the polyphenol content of tomato based products through accurate-mass spectrometry (HPLC–ESI-QTOF), Food Chem., 2011, 129, 877–883 CrossRef PubMed.
- T. Yamamoto, M. Yoshimura, F. Yamaguchi, T. Kouchi, R. Tsuji, M. Saito, A. Obata and M. Kikuchi, Anti-allergic activity of naringenin chalcone from a tomato skin extract, Biosci. Biotechnol. Biochem., 2004, 68(8), 1706–1711 CrossRef CAS PubMed.
- M. Ramirez-Ambrosi, B. Abad-Garcia and M. Viloria-Bernal, A new ultrahigh performance liquid chromatography with diode array detection coupled to electrospray ionization and quadrupole time-of-flight mass spectrometry analytical strategy for fast analysis and improved characterization of phenolic compounds in apple products, J. Chromatogr. A, 2013, 1316, 78–91 CrossRef CAS PubMed.
- S. Reagan-Shaw, D. Eggert and H. Mukhtar, Antiproliferative effects of apple peel extract against cancer cells, Nutr. Cancer, 2010, 62, 517e24 Search PubMed.
- C. Riviere, Dihydrochalcones: occurrence in the plant kingdom, chemistry and biological activities, Stud. Nat. Prod. Chem., 2016, 51, 253–381 CAS.
- C. Gosch, H. Halbwirth and K. Stich, Phloridzin: biosynthesis, distribution and physiological relevance in plants, Phytochemistry, 2010, 71, 838–843 CrossRef CAS PubMed.
- J. H. Lee, S. C. Regmi and J. A. Kim,
et al., Apple flavonoid phloretin inhibits Escherichia coli O157: H7 biofilm formation and ameliorates colon inflammation in rats, Infect. Immun., 2011, 79, 4819–4827 CrossRef CAS PubMed.
- A. Vikram, G. K. Jayaprakasha and P. R. Jesudhasan, Suppression of bacterial cell-cell signalling, biofilm formation and type III secretion system by citrus flavonoids, J. Appl. Microbiol., 2010, 109, 515–527 CrossRef CAS PubMed.
- S. T. Lin, S. H. Tu and P. S. Yang,
et al., Apple polyphenol phloretin inhibits colorectal cancer cell growth via inhibition of the type 2 glucose transporter and activation of p53-mediated signaling, J. Agric. Food Chem., 2016, 64, 6826e37 Search PubMed.
- Y. Wang, W. Xia, M. Tao and X. Fu, Oncopreventive and oncotherapeutic potential of licorice chalcone compounds: Molecular insights, Mini Rev. Med. Chem., 2023, 23(6), 662–699 CrossRef CAS PubMed.
- C. Simmler, A. Hajirahimkhan and D. C. Lankin,
et al., Dynamic residual complexity of the isoliquiritigenin- liquiritigenin interconversion during bioassay, J. Agric. Food Chem., 2013, 61, 2146–2157 CrossRef CAS PubMed.
- U. A. Ashfaq, M. S. Masoud and Z. Nawaz,
et al., Glycyrrhizin as antiviral agent against hepatitis C virus, J. Transl. Med., 2011, 9, 112 CrossRef CAS.
- X. Zhang, P. Zhu and X. Zhang,
et al., Natural antioxidant-isoliquiritigenin ameliorates contractile dysfunction of hypoxic cardiomyocytes via AMPK signaling pathway, Mediat. Inflamm., 2013, 2013, 390890 Search PubMed.
- R. Yang, B. C. Yuan and Y. S. Ma,
et al., The anti-inflammatory activity of licorice, a widely used Chinese herb, Pharm. Biol., 2017, 55, 5–18 CrossRef CAS PubMed.
- O. Ongwisespaiboon and W. Jiraungkoorskul, Fingerroot, Boesenbergia rotunda and its aphrodisiac activity, Pharmacogn. Rev., 2017, 11, 27–30 CrossRef CAS PubMed.
- N. M. Isa, S. I. Abdelwahab and S. Mohan,
et al., In vitro anti-inflammatory, cytotoxic and antioxidant activities of boesenbergin A, a chalcone isolated from Boesenbergia rotunda (L.) (fingerroot), Braz. J. Med. Biol. Res., 2012, 45, 524–530 CrossRef CAS PubMed.
- M. H. Widyananda, S. T. Wicaksono, K. Rahmawati, S. Puspitarini, S. M. Ulfa, Y. D. Jatmiko and N. Widodo, A potential anticancer mechanism of finger root (Boesenbergia rotunda) extracts against a breast cancer cell line, Scientifica, 2022, 5, 9130252 Search PubMed.
- J. Y. Park, J. A. Ko, D. W. Kim, Y. M. Kim, H. J. Kwon, H. J. Jeong and Y. B. Ryu, Chalcones isolated from Angelica keiskei inhibit cysteine proteases of SARS-CoV, J. Enzym. Inhib. Med. Chem., 2016, 31(1), 23–30 CrossRef CAS PubMed.
-
W. P. Jones and A. D. Kinghorn, Extraction of plant secondary metabolites, Natural products isolation, 2012, pp. 341–366 Search PubMed.
- A. E. Star, T. J. Mabry and D. M. Smith, Triangularin, a new chalcone from Pityrogramma triangularis, Phytochemistry, 1978, 17(3), 586–587 CrossRef CAS.
- J. B. Harborne, Plant polyphenols. VIII. Chalcone and flavonol glycosides of gorse flowers, Phytochemistry, 1962, 1(3), 203–207 CrossRef CAS.
-
J. Leonard, B. Lygo and G. Procter, Advanced Practical Organic Chemistry, CRC press, 2013 Search PubMed.
- M. Shimokoriyama, Anthochlor pigments of Coreopsis tinctoria, J. Am. Chem. Soc., 1957, 79(1), 214–220 CrossRef CAS.
- S. E. Drewes and D. G. Roux, A new flavan-3, 4-diol from Acacia auriculiformis by paper ionophoresis, Biochem. J., 1966, 98(2), 493 CrossRef CAS PubMed.
- M. L. Roberts, The flavonoids of Megalodonta beckii and isolation of 2′-3-dihydroxy-4-methoxy-4′-glucosyl chalcone, Biochem. Syst. Ecol., 1980, 8(2), 115–118 CrossRef CAS.
- K. Saito and A. Fukushima, A new affinity-trapping method for isolation of quinoidal chalcone pigments from aqueous extracts of dyer's saffron flowers, Biochem. Physiol. Pflanz., 1989, 184(1–2), 145–153 CrossRef CAS.
- L. Van Puyvelde, N. De Kimpe, J. Costa, V. Munyjabo, S. Nyirankuliza, E. Hakizamungu and N. Schamp, Isolation of flavonoids and a chalcone from Helichrysum odoratissimum and synthesis of helichrysetin, J. Nat. Prod., 1989, 52(3), 629–633 CrossRef CAS PubMed.
- R. Srivastava, A. K. Shaw and D. K. Kulshreshtha, Triterpenoids and chalcone from Syzygium samarangense, Phytochemistry, 1995, 38(3), 687–689 CrossRef CAS.
- S. Daher and F. O. Gülaçar, Analysis of phenolic and other aromatic compounds in honeys by solid-phase microextraction followed by gas chromatography mass spectrometry, J. Agric. Food Chem., 2008, 56(14), 5775–5780 CrossRef CAS PubMed.
- J. K. Adesanwo, F. O. Shode, O. Aiyelaagbe, R. T. Oyede and H. Baijnath, Isolation and characterization of a new chalcone from the leaves of Heteropyxis natalensis, Int. J. Med. Med. Sci., 2009, 1(2), 023–032 Search PubMed.
- T. L. C. Oldoni, I. S. Cabral, M. A. R. d'Arce, P. L. Rosalen, M. Ikegaki, A. M. Nascimento and S. M. Alencar, Isolation and analysis of bioactive isoflavonoids and chalcone from a new type of Brazilian propolis, Separ. Purif. Technol., 2011, 77(2), 208–213 CrossRef CAS.
- C. Y. Meng, Y. Han, Y. X. Duan, J. X. Chen, Q. F. Hu and X. M. Gao, Isolation of Chalcones from the Root of Codonopsis cordifolioidea and Their Antitobacco Mosaic Virus Activities, Asian J. Chem., 2013, 25(17), 9517–9519 CrossRef CAS.
- G. Zheng, M. Liu, Y. Chao, Y. Yang, D. Zhang, Y. Tao and M. Wei, Identification of lipophilic components in Citri Reticulatae Pericarpium cultivars by supercritical CO2 fluid extraction with ultra-high-performance liquid chromatography–Q Exactive Orbitrap tandem mass spectrometry, J. Sep. Sci., 2020, 43(17), 3421–3440 CrossRef CAS.
- I. V. Staffen, F. W. Banhuk, F. Tomiotto-Pellissier, B. T. da Silva Bortoleti, W. R. Pavanelli, T. S. Ayala and R. A. Menolli, Chalcone-rich extracts from Lonchocarpus cultratus roots present in vitro leishmanicidal and immunomodulatory activity, J. Pharm. Pharmacol., 2022, 74(1), 77–87 CrossRef PubMed.
- J. Wang and W. Chow, Study of Ultrahigh-Performance Liquid Chromatography Electrospray Ionization Q-Orbitrap Mass Spectrometry and Various Extraction Methods for Fingerprinting and Identification of Molecular Authenticity Markers in Apple and Grape Juices, ACS Food Sci. Technol., 2022, 2, 1326–1338 CrossRef CAS.
- N. Casado, S. Morante-Zarcero, D. Pérez-Quintanilla, J. S. Câmara and I. Sierra, Two novel strategies in food sample preparation for the analysis of dietary polyphenols: Micro-extraction techniques and new silica-based sorbent materials, Trends Food Sci. Technol., 2020, 98, 167–180 CrossRef CAS.
- W. Huang, A. Xue, H. Niu, Z. Jia and J. Wang, Optimised ultrasonic-assisted extraction of flavonoids from Folium eucommiae and evaluation of antioxidant activity in multi-test systems in vitro, Food Chem., 2009, 114(3), 1147–1154 CrossRef CAS.
- H. F. Zhang, X. H. Yang and Y. Wang, Microwave assisted extraction of secondary metabolites from plants: Current status and future directions, Trends Food Sci. Technol., 2011, 22(12), 672–688 CrossRef CAS.
-
D. Qi, Extractants used in solvent extraction-separation of rare earths: extraction mechanism, properties, and features, Hydrometallurgy of rare earths, 2018, 204, pp. 187–389 Search PubMed.
- N. Curko, M. Tomašević, M. C. Bubalo, L. Gracin, I. R. Redovniković and K. K. Ganić, Extraction of proanthocyanidins and anthocyanins from grape skin by using ionic liquids, Food Technol. Biotechnol., 2017, 55(3), 429 CAS.
- X. Li, R. Guo, X. Zhang and X. Li, Extraction of glabridin using imidazolium-based ionic liquids, Separ. Purif. Technol., 2012, 88, 146–150 CrossRef CAS.
- E. Brglez Mojzer, M. Knez Hrnčič, M. Škerget, Z. Knez and U. Bren, Polyphenols: Extraction methods, antioxidative action, bioavailability and anticarcinogenic effects, Molecules, 2016, 21(7), 901 CrossRef PubMed.
- N. Baharuddin, M. F. M. Nordin, N. A. Morad and N. A. Rasidek, Pressurized hot water extraction of phenolic and antioxidant activity of Clinacanthus nutan leaves using accelerated solvent extractor, Aust. J. Basic Appl. Sci., 2017, 11, 56–63 CAS.
- S. Sirichokworrakit, H. Rimkeeree, W. Chantrapornchai and U. Sukatta, Comparative study on conventional, accelerated solvent extraction and ultrasonic-assisted extraction of total phenolic and anthocyanin contents and antioxidant activities from Riceberry bran, Agric. Nat. Resour., 2022, 56(2), 243–254 Search PubMed.
- P. M. Kus, P. Okińczyc, M. Jakovljević, S. Jokić and I. Jerković, Development of supercritical CO2 extraction of bioactive phytochemicals from black poplar (Populus nigra L.) buds followed by GC–MS and UHPLC-DAD-QqTOF-MS, J. Pharm. Biomed. Anal., 2018, 158, 15–27 CrossRef CAS PubMed.
- D. K. Yadav, P. Kaushik, Pankaj, V. S. Rana, D. Kamil, D. Khatri and N. A. Shakil, Microwave assisted synthesis, characterization and biological activities of ferrocenyl chalcones and their QSAR analysis, Front. Chem., 2019, 7, 1–15 CrossRef PubMed.
- S. L. Gaonkar and U. N. Vignesh, Synthesis and pharmacological properties of chalcones: a review, Res. Chem. Intermed., 2017, 43(11), 6043–6077 CrossRef CAS.
- C. W. Phan, V. Sabaratnam, W. K. Yong and S. N. A. Malek, The role of chalcones: Helichrysetin, xanthohumol, and flavokawin-C in promoting neurite outgrowth in PC12 Adh cells, Nat. Prod. Res., 2017, 32, 1229–1233 CrossRef PubMed.
- P. Singh, A. Anand and V. Kumar, Recent developments in biological activities of chalcones: A mini review, Eur. J. Med. Chem., 2014, 85, 758–777 CrossRef CAS PubMed.
- H. L. Qin, Z. W. Zhang, R. Lekkala, H. Alsulami and K. P. Rakesh, Chalcone hybrids as privileged scaffolds in antimalarial drug discovery: A key review, Eur. J. Med. Chem., 2020, 193, 112215 CrossRef CAS.
- F. Zhao, Y. Watanabe, H. Nozawa, A. Daikonnya, K. Kondo and S. Kitanaka, Prenylflavonoids and phloroglucinol derivatives from hops (Humulus lupulus), J. Nat. Prod., 2005, 68(1), 43–49 CrossRef CAS PubMed.
- M. Chen, T. G. Theander, S. B. Christensen, L. Hviid, L. Zhai and A. Kharazmi, Licochalcone A, a new antimalarial agent, inhibits in vitro growth of the human malaria parasite Plasmodium falciparum and protects mice from P. yoelii infection, Antimicrob. Agents Chemother., 1994, 38(7), 1470–1475 CrossRef CAS PubMed.
- A. Yenesew, M. Induli, S. Derese, J. O. Midiwo, M. Heydenreich, M. G. Peter, H. Akala, J. Wangui, P. Liyala and N. C. Waters, Anti-plasmodial flavonoids from the stem bark of Erythrina abyssinica, Phytochemistry, 2004, 65(22), 3029–3032 CrossRef CAS PubMed.
- T. Narender, K. Tanvir, M. S. Rao, K. Srivastava and S. K. Puri, Prenylated chalcones isolated from Crotalaria genus inhibits in vitro growth of the human malaria parasite Plasmodium falciparum, Bioorg. Med. Chem. Lett., 2005, 15(10), 2453–2455 CrossRef CAS PubMed.
- S. Frolich, C. Schubert, U. Bienzle and K. Jenett-Siems,
In vitro antiplasmodial activity of prenylated chalcone derivatives of hops (Humulus lupulus) and their interaction with haemin, J. Antimicrob. Chemother., 2005, 55(6), 883–887 CrossRef PubMed.
- M. Wang, L. Lin, J. J. Lu and X. Chen, Pharmacological review of isobavachalcone, a naturally occurring chalcone, Pharmacol. Res., 2021, 165, 105483 CrossRef CAS PubMed.
- A. Detsi, M. Majdalani, C. A. Kontogiorgis, D. Hadjipavlou-Litina and P. Kefalas, Natural and synthetic 2′-hydroxy-chalcones and aurones: synthesis, characterization and evaluation of the antioxidant and soybean lipoxygenase inhibitory activity, Bioorg. Med. Chem., 2009, 17(23), 8073–8085 CrossRef CAS PubMed.
- G. Pastorino, L. Cornara, S. Soares, F. Rodrigues and M. B. P. Oliveira, Liquorice (Glycyrrhiza glabra): A phytochemical and pharmacological review, Phytother Res., 2018, 32(12), 2323–2339 CrossRef CAS PubMed.
- S. Padhye, A. Ahmad, N. Oswal and F. H. Sarkar, Emerging role of Garcinol, the antioxidant chalcone from Garcinia indica Choisy and its synthetic analogs, J. Hematol. Oncol., 2009, 2(1), 1–13 CrossRef PubMed.
- D. Wang, J. Liang, J. Zhang, Y. Wang and X. Chai, Natural Chalcones in Chinese Materia Medica: Licorice, Evid. base Compl. Alternative Med., 2020, 2020, 3821248 CrossRef PubMed.
- R. B. Semwal, D. K. Semwal, S. Combrinck and A. Viljoen, Butein: From ancient traditional remedy to modern nutraceutical, Phytochem. Lett., 2015, 11, 188–201 CrossRef CAS.
- J. C. Lee, K. Y. Lee, J. Kim, C. S. Na, N. C. Jung, G. H. Chung and Y. S. Jang, Extract from Rhus verniciflua Stokes is capable of inhibiting the growth of human lymphoma cells, Food Chem. Toxicol., 2004, 42(9), 1383–1388 CrossRef CAS PubMed.
- G. Di Carlo, N. Mascolo, A. A. Izzo and F. Capasso, Flavonoids: old and new aspects of a class of natural therapeutic drugs, Life Sci., 1999, 65(4), 337–353 CrossRef CAS PubMed.
- E. Hofmann, J. Webster, T. Do, R. Kline, L. Snider, Q. Hauser and S. Paula, Hydroxylated chalcones with dual properties: Xanthine oxidase inhibitors and radical scavengers, Bioorg. Med. Chem., 2016, 24(4), 578–587 CrossRef CAS PubMed.
- M. Kaur and R. Kaushal, Spectroscopic investigations, ab initio DFT calculations, molecular docking and in vitro assay studies of novel oxovanadium (V) chalcone complexes as potential antidiabetic agents, J. Mol. Struct., 2023, 1271, 133994 CrossRef CAS.
- G. Belofsky, D. Percivill, K. Lewis, G. P. Tegos and J. Ekart, Phenolic metabolites of Dalea versicolor that enhance antibiotic activity against model pathogenic bacteria, J. Nat. Prod., 2004, 67(3), 481–484 CrossRef CAS PubMed.
- H. Kromann, M. Larsen, T. Boesen, K. Schonning and S. F. Nielsen, Synthesis of prenylated benzaldehydes and their use in the synthesis of analogues of licochalcone A, Eur. J. Med. Chem., 2004, 39(11), 993–1000 CrossRef CAS PubMed.
- Z. Nowakowska, A review of anti-infective and antiinflammatory chalcones, Eur. J. Med. Chem., 2007, 42, 125–137 CrossRef CAS PubMed.
- R. I. Tsukiyama, H. Katsura, N. Tokuriki and M. Kobayashi, Antibacterial activity of licochalcone A against spore-forming bacteria, Antimicrob. Agents Chemother., 2002, 46(5), 1226–1230 CrossRef CAS PubMed.
- S. Varsha, R. C. Agrawal and P. Sonam, Phytochemical screening and determination of anti-bacterial and anti-oxidant potential of Glycyrrhiza glabra root extracts, Int. J. Environ. Res. Dev., 2013, 7(4A), 1552 Search PubMed.
- P. D. Bremner and J. M. Meyer, Pinocembrin chalcone: an antibacterial compound from Helichrysum trilineatum, Planta Med., 1998, 64(8), 777 CrossRef CAS PubMed.
- A. S. Joshi, X. C. Li, A. C. Nimrod, H. N. Elsohly, L. A. Walker and A. M. Clark, Dihydrochalcones from Piper longicaudatum, Planta Med., 2001, 67(2), 186–188 CrossRef CAS PubMed.
- P. Yadav, K. Lal, L. Kumar, A. Kumar, A. Kumar, A. K. Paul and R. Kumare, Synthesis, crystal structure and antimicrobial potential of some fluorinated chalcone-1,2,3- triazole conjugates, Eur. J. Med. Chem., 2018, 155, 263–274 CrossRef CAS PubMed.
- B. T. Yin, C. Y. Yan, X. M. Peng, S. L. Zhang, S. Rasheed, R. X. Geng and C. H. Zhou, Synthesis and biological evaluation of α-triazolyl chalcones as a new type of potential antimicrobial agents and their interaction with calf thymus DNA and human serum albumin, Eur. J. Med. Chem., 2014, 71, 148–159 CrossRef CAS PubMed.
- S. J. Won, C. T. Liu, L. T. Tsao, J. R. Weng, H. H. Ko, J. P. Wang and C. N. Lin, Synthetic chalcones as potential anti-inflammatory and cancer chemopreventive agents, Eur. J. Med. Chem., 2005, 40(1), 103–112 CrossRef CAS PubMed.
- M. L. Birsa and L. G. Sarbu, Hydroxy chalcones and analogs with chemopreventive properties, Int. J. Mol. Sci., 2023, 24(13), 10667 CrossRef CAS PubMed.
- A. Sharma, R. Sharma, M. Sharma, M. Kumar, M. D. Barbhai, J. M. Lorenzo, S. Sharma, M. K. Samota, M. Atanassova, G. Caruso, M. Naushad and Radha,
et al., Carica papaya L. Leaves: Deciphering Its Antioxidant Bioactives, Biological Activities, Innovative Products, and Safety Aspects, Oxid. Med. Cell. Longevity, 2022, 2451733 CAS.
- R. J. Anto, K. Sukumaran, G. Kuttan, M. N. A. Rao, V. Subbaraju and R. Kuttan, Anticancer and antioxidant activity of synthetic chalcones and related compounds, Cancer Lett., 1995, 97, 33–37 CrossRef CAS PubMed.
- T. Constantinescu and C. N. Lungu, Anticancer activity of natural and synthetic chalcones, Int. J. Mol. Sci., 2021, 22(21), 11306 CrossRef CAS PubMed.
- D. Shi, J. Yang, Y. Jiang, L. Wen, Z. Wang and B. Yang, The antioxidant activity and neuroprotective mechanism of isoliquiritigenin, Free Radic. Biol. Med., 2020, 152, 207–215 CrossRef CAS PubMed.
- W. C. Huang, H. H. Su, L. W. Fang, S. J. Wu and C. J. Liou, Licochalcone A Inhibits Cellular Motility by Suppressing E-cadherin and MAPK Signaling in Breast Cancer, Cells, 2019, 8, 218 CrossRef CAS PubMed.
- D. A. Learmonth, M. J. Bonifácio and P. Soares-da-Silva, Synthesis and biological evaluation of a novel series of “orthonitrated” inhibitors of catechol-o-methyltransferase, J. Med. Chem., 2005, 48, 8070–8078 CrossRef CAS PubMed.
- J. P. Finberg and J. M. Rabey, Inhibitors of mao-a and mao-b in psychiatry and neurology, Front. Pharmacol, 2016, 7, 340 CAS.
- E. H. Moscato, A. Jain, X. Peng, E. G. Hughes, J. Dalmau and R. J. Balice-Gordon, Mechanisms underlying autoimmune synaptic encephalitis leading to disorders of memory, behavior and cognition: Insights from molecular, cellular and synaptic studies, Eur. J. Neurosci., 2010, 32, 298–309 CrossRef PubMed.
- M. Mehreen Ghias, M. N. Ahmed, B. Sajjad, M. A. A. Ibrahim, U. Rashid, S. W. A. Shah, M. Shoaib, M. Madni, M. N. Muhammad Nawaz Tahir and A. Mario,
et al., 1-Hydroxynaphthalene-4-trifluoromethylphenyl chalcone and 3-hydroxy-4-trifluoromethylphenyl flavone: A combined experimental, structural, in vitro AChE, BChE and in silico studies, J. Mol. Struct., 2022, 1253, 132253 CrossRef.
- M. Ono, M. Haratake, H. Mori and M. Nakayama, Novel chalcones as probes for in vivo imaging of beta-amyloid plaques in Alzheimer's brains, Bioorg. Med. Chem., 2007, 15, 6802–6809 CrossRef CAS PubMed.
- Y. T. Lee, T. H. Fong, H. M. Chen, C. Y. Chang, Y. H. Wang, C. Y. Chern and Y. H. Chen, Toxicity assessments of chalcone and some synthetic chalcone analogues in a zebrafish model, Molecules, 2014, 19(1), 641–650 CrossRef PubMed.
- J. R. Dimmock, D. W. Elias, M. A. Beazely and N. M. Kandepu, Bioactivities of chalcones, Curr. Med. Chem., 1999, 8, 1125–1149 CrossRef.
- M. C. Rossette, D. C. Moraes, E. K. Sacramento, M. A. Romano-Silva, J. L. Carvalho, D. A. Gomes, H. Caldas, E. Friedman, L. Bastos-Rodrigues and L. De Marco, The In Vitro and In Vivo Antiangiogenic Effects of Flavokawain B, Phytother Res., 2017, 31, 1607–1613 CrossRef CAS PubMed.
- M. Pilatova, L. Varinska, P. Perjesi, M. Sarissky, L. Mirossay, P. Solar, A. Ostro and J. Mojzis,
In vitro antiproliferative and antiangiogenic effects of synthetic chalcone analogues, Toxicol. In Vitro, 2010, 24, 1347–1355 CrossRef CAS PubMed.
- Z. Wang, N. Wang, S. Han, D. Wang, S. Mo, L. Yu, H. Huang, K. Tsui, J. Shen and J. Chen, Dietary compound isoliquiritigenin inhibits breast cancer neoangiogenesis via VEGF/VEGFR-2 signaling pathway, PLoS One, 2013, 8, e68566 CrossRef CAS PubMed.
|
This journal is © The Royal Society of Chemistry 2024 |
Click here to see how this site uses Cookies. View our privacy policy here.