Millets as supergrains: a holistic approach for sustainable and healthy food product development
Received
8th February 2024
, Accepted 10th April 2024
First published on 10th April 2024
Abstract
The Global Report on Food Crises underscores the urgent need for sustainable food solutions as the world faces a 34% increase in acute food insecurity. Millets, which are ancient grains abundant in proteins with essential amino acids, dietary fiber and some essential minerals, are resistant to climate change and thus have become an important tool in the fight against this issue. A comprehensive analysis of millets is presented in this review, covering their historical relevance, sustainable production, nutritional value, novel sustainable processing methods, product development and digestibility for health benefits. In terms of agriculture, millets provide a viable choice as they are resilient to climate change and boost productivity. Because millets have a high quantity of vital amino acids and antioxidants, their nutritional composition makes them an important tool in the fight against malnutrition and the prevention of chronic illnesses. Furthermore, eco-friendly millet processing technologies are discussed, highlighting the contribution of both low-temperature and green processes as sustainable approaches to improve their nutritional value and reduce detrimental environmental effects. Millets demonstrate versatility in food production, ranging from classic recipes to cutting-edge items such as extruded snacks and 3D-printed meat substitutes. However, nutrient-dense millets encounter challenges from anti-nutritional elements. Their digestibility is affected by elements such as the starch structure, poor protein solubility, tannins and processing techniques. Important roles are played by processing techniques such as soaking, germination, cooking, and fermentation. Protein digestion is improved by soaking, but the content of tannins, phytic acid, oxalates and other antinutrient compounds may decrease by germination. Alternatively, fermentation increases protein and starch digestibility and reduces anti-nutrients. Thus, to create a more resilient and sustainable future, millets have enormous potential to help achieve a number of Sustainable Development Goals (SDGs), presenting a strong argument for their inclusion in global food systems.
Sustainability spotlight
Millets as supergrains present solutions with wide-ranging advantages, including economic efficiency, climate resilience, and alignment with important Sustainable Development Goals (SDGs) of the UN. Innovative processing methods that preserve nutritional value, while minimizing environmental impact and waste generation, including low-temperature methods such as microwave and infrared processing and non-thermal methods such as cold plasma and high-pressure processing, further improve their sustainability. The potential for commercialization and broad public acceptance of millet-based products, which include baked foods, beverages, and even 3D-printed alternatives, increases as they gain popularity and provide a variety of sustainable and nutrient-dense options.
|
1. Introduction
Critical global challenges such as climate change, natural resource depletion, and hunger are compounded by the rapid population growth, which is forecasted to reach 9.7 billion by 2050.1 The food chain has emerged as a pivotal path towards overcoming these sustainability challenges, in which case the increased demand for food production must not further compromise the environment or contribute to climate change. It is projected that this food demand will increase in 2050 by 60–100%,2 which is further complicated by the inability to meet current demands. The Global Report on Food Crises3 demonstrated a 34% increase in acute food insecurity with more than 65 million people facing the issue currently, illustrating the inherent strain an increasing population will place on an already insufficient system.
Current food systems account for more than a quarter of total greenhouse gas emissions, with livestock and fisheries accounting for the largest portion. This is increased when factoring in land-use where livestock production accounts for two-thirds of emissions compared to one-third from crop production.4 Consequently, as protein demands are increasing globally, the current production systems for animal protein account for the most significant environmental impacts. Alternatively, crop production generally demonstrates less environmental emissions than livestock production.4 However, it is often limited by the climate, and high protein crops often lack essential amino acids critical to a diet. Accordingly, environmental changes cyclically impact overall food production. Climate change has direct impacts on total food yield due to its morbidity, mortality, and reproduction in livestock5 and on crop yield due to increased pest activity, lower rainfall, or increased CO2 levels.6
Innovative and modern solutions including plant-based protein production, insect proteins, cellular agriculture, and precision fermentation offer potential green and nutritional alternatives to diminish the costs of traditional food production. A key factor in many of these alternatives is the production of protein; however, it is essential that new food production strategies focus on comprehensive nutritional values considering fiber, vitamins, and nutrients. Cellular agriculture potentiates diminished resource utilization, particularly land, water, and less methane production,7 while generating the key proteins and nutrients associated with a meat diet. However, current methods use higher energy, the production scale of this field of study is still far away from providing food for large populations and consumer acceptance is currently lower than other strategies. Precision fermentation operates similarly to cell agriculture in reducing land use but has high energy while utilizing microbial production food ingredients or flavors.8 Insect meal is another potential sector, which also demonstrates minimal consumer acceptance, particularly in higher income countries. Thus, plant-based sustainable strategies offer the solution for the most immediate sustainable impact. However, some plant sources are insufficient in certain essential amino acids particularly methionine, cysteine, and lysine.9 Therefore, sustainable food solutions centered on crop production require products that provide key proteins with many of the essential amino acids, limited resource utilization for production, and resiliency to various climates and environments.
Various potential innovations have been introduced that may offer solutions to generate more sustainable food systems, and among them, millets, which are grains that have been utilized for centuries, presents a key resource towards attaining this. As a cereal crop, millets exhibit the ability to grow in various climates, have low water utilization, and require minimal labor,10 while also providing comparable or higher proteins and fiber to other cereal grains.11 The world may make great strides towards accomplishing several SDGs by incorporating millets in agricultural methods and the diet. This review establishes the value of millets as a sustainable alternative from production through processing.
Millets were domesticated and cultivated for 8700–10
300 years as both human and animal nourishment across the continents of Asia, Africa, and a small portion of Europe; however, they have been replaced by wheat and rice due to irrigation, open markets, and urbanization. Specifically, archeological records indicate that millet has been grown in India for thousands of years,12 which can be categorized into major and minor millets. Pearl millet (Pennisetum typhoides L.) and sorghum (Sorghum bicolor L.) are major millets, whereas Kodo millet, barnyard millet (Echinochloa frumentacea L.), little millet (Panicum sumatrense Roth), and foxtail millet (Setaria italica L.) are minor millets.13 In these two categories, although their nutrient composition varies, a key component of these crops is their climate resilience, with the ability to grow in drier conditions with limited labor requirements and growth inputs.
Millets offer extensive potential to improve food security and diet diversification; however, their current global implementation is limited by their economic viability and consumer knowledge compared to other global staple crops. The economic viability of millets is often constrained by their reduced overall yield through current production methods.14 Conversely, Padulosi et al.15 asserted that the limitations in their yield are correlated with their cultivation methods, which have drastic room for improvement and further supported by Grovermann et al.,16 demonstrating a reduction in yield gap when integrating irrigation. Thus, considering the present difficulties of reducing land availability, limited food supply, and inadequate nutrition, it is crucial to incorporate millet as a fundamental crop in addition to wheat and rice. The recognition of the significance of millets has been acknowledged by several organizations and researchers in enhancing food and nutrition security, particularly in areas facing high rates of malnutrition and poverty, as emphasized by the Food and Agriculture Organization of the United Nations (FAO).1,17 Further, expanding and improving processing methods may increase the value of the product by reducing resource inputs, extending its shelf life, and increasing its nutritional components. This review establishes the value of millets as a sustainable alternative from their production to processing.
2. Nutritional value of millets
Environmental sustainability is not the only factor to consider in food production to realize SDGs 2 and 3 of food security and overall health, which also requires the production and availability of more nutrient-dense foods.18 It is critical to consider that eating habits have a big influence on diabetes, in addition to obesity and a sedentary lifestyle. Thus, diversifying diets is essential to control and avoid diabetes and other diseases. Conversely, approximately 80% of the energy eaten in developing countries is derived from staple foods such as refined rice, wheat, and maize.19 In this case, traditional, nutrient-dense and less glucose-forming staple grains such as millet offer a crucial alternative.20
Millets are rich in protein, fatty acids, and dietary fiber, while also contributing key vitamins, minerals, and polyphenols. However, their overall composition varies between species, as depicted in Table 1. Dietary fiber is typically greater in millets than rice or wheat, especially species such as barnyard millets, which contain approximately 14.7 g of dietary fiber per 100 g. This table also reveals that millets have more protein than rice and are often comparable to other cereals such as wheat and maize. Further, the main characteristic of millet protein is its high concentration of important amino acids including the sulfur-containing cysteine and methionine, as well as most of the other essential amino acids.21Table 2 highlights the amino acid composition of several millet strains and compares them with the most common cereal grains of rice and wheat. Nearly all the essential acids are present in each millet, and some species may be key sources of specific essential amino acids that are low in cereal grains. Therefore, adding these neglected millets to the diet will help people across the world overcome their hidden hunger.
Table 1 Nutritional properties of millets and other cereals per 100 g of samplea
Nutrient |
Maize |
Rice |
Wheat |
Barnyard millet |
Finger millet |
Foxtail millet |
Kodo millet |
Little millet |
Pearl millet |
Proso millet |
Sorghum |
Source: Dayakar et al.22
|
Protein (g) |
11.5 |
6.8 |
11.8 |
11.6 |
7.3 |
12.3 |
8.3 |
8.7 |
11.4 |
12.5 |
10.4 |
Crude fiber (g) |
2.7 |
0.2 |
1.2 |
14.7 |
3.6 |
8 |
9 |
8.6 |
1.2 |
2.2 |
1.6 |
Carbohydrate (g) |
66.2 |
78.2 |
71.2 |
74.3 |
72 |
60.9 |
65.9 |
75.7 |
67.5 |
70.4 |
72.6 |
Ca (mg) |
20 |
10 |
41 |
14 |
344 |
31 |
27 |
17 |
42 |
14 |
25 |
Fe (mg) |
2.3 |
0.7 |
5.2 |
15.2 |
3.9 |
5 |
1.7 |
9.3 |
16 |
10 |
4.1 |
P (mg) |
348 |
160 |
306 |
121 |
283 |
290 |
188 |
220 |
296 |
206 |
222 |
Table 2 Amino acid composition of millets per 100 g proteina
Amino acid |
Finger millet |
Barnyard millet (K141285) |
Foxtail millet |
Proso millet |
Wheat |
Rice |
Source: Chandra et al.;23 Kim et al.;24 Wiedemair et al.;25 Yang et al.26
|
Histidine (g) |
0.13 |
0.31 |
0.38 |
0.25 |
0.32 |
0.16 |
Lysine (g) |
0.22 |
0.23 |
0.41 |
0.18 |
0.27 |
0.24 |
Phenyl (g) |
0.31 |
0.83 |
0.95 |
1.04 |
0.68 |
0.35 |
Tryptophan (g) |
0.22 |
0.28 |
0.54 |
1.04 |
0.36 |
0.22 |
Methionine (g) |
0.21 |
0.25 |
× |
0.18 |
0.22 |
0.16 |
Threonine (g) |
0.24 |
0.47 |
0.56 |
0.49 |
0.37 |
0.24 |
Leucine (g) |
0.69 |
1.51 |
2.15 |
1.35 |
0.93 |
0.55 |
Isoleucine (g) |
0.40 |
0.70 |
0.66 |
0.43 |
0.53 |
0.55 |
Valine (g) |
0.48 |
0.78 |
0.89 |
0.54 |
0.59 |
0.40 |
The quality nutrients found in the various millet species also have direct positive impacts on human health. Millets act as anti-diabetic due to the gradual digestion of their carbohydrates, which do not generate the blood glucose spike that is common with other grains, especially white rice. Further, due to their high fiber content, the consistent consumption of millets promotes gut health and ensures adequate colon hydration, preventing constipation. Tryptophan in millets leads to increased serotonin levels, promoting a calming effect on the mind. Furthermore, the presence of magnesium (Mg) in millets is beneficial for mitigating the impact of cardiac attacks and migraines, while blood cholesterol levels are reduced in the presence of vitamin B3. Additionally, millets contribute to a decrease in C-reactive proteins and triglycerides, acting as a preventive measure against coronary diseases. Each type of millet is devoid of gluten and exhibits notable antioxidant properties, together with being non-allergenic.22 The seed coat of millets contains many phenolic acids, acting as antioxidants, with finger millets containing a high phenolic content, which exhibit greater radical scavenging ability compared to wheat, rice, and even other millets.27 These phenolic properties also contribute to the effective preservative qualities of millets given that the total and compositions of phenolic compounds in millet grains demonstrated an inverse relationship to fungal load.27,28 Considering their protein and fiber content, as well as anti-diabetic, antioxidant, and antimicrobial capacity, millets present a stable, healthy crop alternative to traditional cereal grains, which can also help meet the growing demands for protein globally.
3. Sustainable production of millet
Millets have potential to grow in adverse condition of high temperatures, low rainfall, and poor soil quality such as alkaline soil with a pH as high as 11, at altitudes of 2500 meters above sea level, and coping with variables in rainfall ranging from 800 to 1200 mm. Furthermore, they are resilient to drought, diseases and pests in a variety of climates and ecosystems.29 This resilience is due to their physiological characteristics such as deep root systems, allowing these plants to reach moisture found deep within the soil, effective water usage, enhanced water-use efficiency and C4 photosynthesis, and C4 plants can limit leaf moisture loss by closing their stomata for long periods. Consequently, they effectively utilize the inadequate moisture found in semi-arid areas.30,31 Millets have a smaller environmental impact than other cereal crop such as paddy and wheat because they frequently require fewer inputs, such as less water, pesticides and fertilizers.32,33 Research has shown that millet cultivation has a comparatively smaller carbon footprint and water footprint compared to rice and wheat production. This is mostly because millet requires less artificial fertilizers and irrigation.34 Also, the maturity period of various millet varieties is shorter, which helps them avoid drought conditions.35
Pearl millet production is primarily concentrated in arid regions of Asia and Africa, where rainfall is limited and unpredictable.36 Sorghum, covering 42.1 million hectares in 105 countries, is the most ubiquitous millet in farming operations, while pearl and other minor millets are dispersed throughout 93 countries, but 97% is grown and consumed in developing nations, mainly in Africa and Asia. India grows the most millet, 26.6% of the global total and 83% of that in Asia.37,38 It grows well at temperatures as high as 42 °C, having originated in the hot and arid Sahelian region of Africa. Alternatively, finger millet can withstand lower temperatures, making it appropriate for farming at high altitudes.31 Because pearl millet uses moisture well and can withstand high temperatures and moisture stress, it is the main crop in northern Namibia. This area is affected by issues such as poor water-holding capacity, erratic rainfall, and barren sandy soils. Growing pearl millet on marginal and unstable land is a major barrier to productivity growth and maintenance in settings with poor potential for production.39 The inclusion of millets in intercropping systems offers soil health, resource use efficiency, and agricultural sustainability. Finger millet benefits from intercropping with pigeon pea, field bean, soybean, and black gram. Foxtail millet benefits from intercropping with groundnut, cotton, and pigeon pea. Little millet benefits from intercropping with black gram, sesamum/soybean/pigeon pea, and pigeon pea. Proso, barnyard, and Kodo millet should be intercropped with green gram, rice bean, pigeon pea, and other legumes to boost production and economic returns.40Fig. 1 illustrates the various types of millets cultivated across the globe.
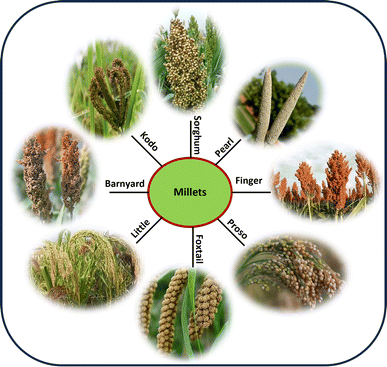 |
| Fig. 1 Millet types cultivated around the world. | |
Millets are cultivated and consumed in a way that helps the UN achieve several Sustainable Development Goals (SDGs). Due to their low input requirements and environmentally favorable qualities, millets help achieve SDG 13 (climate action) and SDG 2 (zero hunger).41,42 Consistent with SDGs 1 (no poverty) and 12 (responsible consumption and production), they also assist in lowering the demand for synthetic fertilizers.43 Furthermore, millets help with the difficulties of water scarcity in agriculture and contribute to the creation of rural income (SDG 1).44 They are sustainable substitutes for conventional cereals because of their climate resistance, which advances SDG 13 (climate action).45 To stimulate local millet production, India declared 2018 the national year of millets. Also, the UN Food and Agriculture Organization (FAO) proclaimed 2023 the international year of millets at India's request.46
4. Sustainable millet processing techniques
4.1 Thermal processing of millets
Thermal processing, which is the application of heat (dry heat, hydrothermal or both) alone or together with novel low thermal heating techniques (microwave, infrared, and ohmic heating) to the grains and food for preservation, modification, and shelf-life extension, has emerged as potential technology for a sustainable future.47 However, most of the commonly used methods for the thermal processing of millets, such as steaming, extrusion, roasting, popping, flaking and high-temperature treatments, influence the texture, flavor, nutritional composition, and shelf-life of the final millet product.48
Studies have indicated that applying heat can reduce the activity of enzymes such as lipoxygenase and lipase, thereby extending the shelf life of grains.49 According to Gomez and Martinez,50 thermal processing of grains can also lead to changes in the structure of the starch granules, reduction in the microbial load, flavor and fragrance modifications, and denaturation of proteins. Thermal treatments change the color of flour and reduce its nutrients. A color change can enable consumer acceptance.51 Thermal processing of millets is associated with various chemical reactions, including starch gelatinization, protein denaturation, modification of structure, improvements in flavour, aroma, and microbial reduction. Thus, although thermal processing offers numerous benefits, a critical question arises: is thermal processing a sustainable approach? Also, sustainability does not only involve mono-dimensional methods such as carbon footprints, energy consumption and nutritional indices but also lies in the ratio of energy output to input and contribution of renewable energy to the energy input.52 Conventional food processing methods (dry heating) are responsible for the loss of some nutrients, low productivity, high energy consumption, high water usage, and prolonged heating and stirring. Therefore, novel heating methods aimed at reducing the energy consumption, production cost, time and improving the sustainability through energy input–output balance are required.
4.1.1 Heat treatment processing.
Heat treatments such as boiling, puffing, parboiling and roasting improve the eating quality of millets but their effect on the nutritional attributes of millets varies with the heating method applied.47 The physicochemical characteristics and milling yield of millet grains are enhanced by parboiling. A study on decorticated pearl and proso millets showed an increase in yield by 37% and 28%, respectively, significantly increasing the phenolic content in the millet product, i.e., porridge and couscous.53 Dry heating demonstrates significant potential in deactivating microbes responsible for spoilage and reducing free fatty acids (FFA), which is crucial for prolonged storage. Heat treatment is effective in decreasing the FFA levels in flour by deactivating lipase enzymes, especially at elevated temperatures. A study showed that dry heat treatment at 100 °C for 120 min resulted in a notable 63.6% reduction in FFA in pearl millet flour.54 Moreover, this treatment resulted in an increase in the in vitro iron content. Singh Scholar et al.55 observed a reduction in crude fat from 1.5% to 1.3% upon roasting at 97 °C, indicating an extended shelf life. Furthermore, composite bread made from the extrusion of finger millet flour demonstrated superior sensory attributes, scoring higher than control bread.56 Accordingly, dry heat treatment improves the nutritional value and storage capacity, encouraging long-term usage.
4.2 Low thermal processing
4.2.1 Microwave processing.
Microwaves have frequencies in the range of 300 MHz to 300 GHz. Through molecular interaction with an electromagnetic and electric field, microwave energy is provided directly to the product. Microwave processing is a suitable method given that it leads to a faster drying rate, uniform and rapid heating, reduction in processing time, precise control of the process and low operational cost.57,58 Venkateswara Rao et al.59 reported enhanced functional properties (WAC, solubility) with the effective use of microwaves as pretreatment in millet flour. Applying a microwave (MW) treatment for 40 to 100 s at 900 W resulted in a 45.53%, 45.18%, and 77.54% reduction in the tannin levels in millet varieties, including finger millet, pearl millet, and sorghum, respectively.60 Microwaves have been applied in various processes including thawing, sterilization, baking, and drying, which have shown in a reduction in processing time, while no influence on quality indices, and economy in term of energy. In the case of pearl millet, microwave treatment at 900 W for 100 s, starting with a moisture content of 18%, led to decreased lipase activity. This treated flour exhibited an insignificant increase in free fatty acids (FFA) over 30 days, while the untreated flour remained acceptable for only 10 days.61 Correspondingly, Kashaninejad62 noted the highest lipase inactivation at 90 s for 900 W in millet grains. Furthermore, Kumar et al.63 reported a reduction in fat content from 3.24% to 3.05% for microwaved cooked proso millet and from 1.91% to 1.79% for little millet. This reduction suggests a potential extension in the shelf life of millet flour, thereby promoting enhanced food utilization and availability. Kodo millet was studied by Gopal and Bhuvana.64 They evaluated the phytochemical, functional, and rheological aspects after microwave treatment. The color (L* value reduced from 75.22 to 67.93), total phenolic content (2.64 to 4.67 mg GAE/100 g), and free radical scavenging activity (91.12% to 87.94%) changed significantly at higher power and time levels. Higher power and time settings increased the water absorption capacity (WAC) from 0.98 to 1.12 g g−1 and lowered the oil absorption capacity (OAC) from 0.84 to 0.78. The treated Kodo millet flour showed improved functional, rheological, and antioxidant qualities, making it appropriate for bakery extrusion, functional food development, and other goods. The application of microwaves in the food industry improves consistency, saves time and money, and reduces post-treatment waste. Microwave usage optimizes resource utilization and minimizes energy consumption, supporting sustainable food production.
4.2.2 Ohmic heating.
Ohmic heating (OH) uses an alternating electrical current across a conductor to increase the temperature according to Joule's law. This method heats the substance volumetrically, accelerating the temperature rise and enables rapid and uniform heating with minimal loss of the structure, nutritional and sensory properties of a food product.65 Ohmic heating is an effective way to cook pasta, rice and maize due to its energy efficiency, speed, low energy consumption, and sustainability.66,67 Also, the low thermal process offers numerous advantages compared to traditional heating methods, such as preserving nutritional value, deactivating microbes and enzymes and effectively used in baking, roasting, and frying process.68 However, its use in millet processing is underexplored. Its potential in cooking pearl millet was highlighted by its yield, texture, color, water solubility index and pasting properties. Ohmic heating has emerged as promising technology. It exhibits no adverse effects on the quality attributes of the grain and it stands out as eco-friendly and highly efficient cooking technology.69 Peroxidase action on C-glycosylflavones gives pearl millet flour a mousy smell when processed at high moisture. Also, the storage conditions greatly affect triglyceride hydrolysis and peroxidation, causing off-flavors in millet flour. Ohmic heating, which affects lipase activity non-thermally, enables the better storage of pearl millet flour.70 Dias-Martins et al.71 explored ohmic heating (OH) and conventional open-pan (CONV) cooking of pearl millet and compared them. The cooking yield was lower for OH than CONV. OH softened the grains slower than CONV, but it changed the decorticated grain texture and color with higher L* values. The WAI and WSI were similar for the OH and CONV techniques. Both methods produced identical paste viscosity profiles, indicating equivalent starch conversion.
4.2.3 Infrared heating.
Infrared (IR) heating radiation lies between visible light and microwaves with wavelengths in the range of 0.78 to 1000 µm and changes the atomic and molecular states of the surface of foods. Electronic and rotational changes occur with UV/visible rays and microwaves, while vibrational changes occur with IR radiation. IR vibrates food molecules at 60
000–150
000 MHz, heating them quickly without heating the air.72 Microbial cells are damaged by this energy both internally and externally, leading to internal content leakage, membrane contraction, mesosome disintegration, and damage to their cell walls. Additionally, the microbial cells that survive the treatment suffer sub-lethal damage, making their survival difficult.73 Infrared stabilization controls hydrolytic rancidity in many foods, and adjusting the operational settings can lengthen the storage life and improve the acceptability of food. In a study on improving infrared heating parameters for sorghum flour, 120 °C for 8.5 min reduced its free fatty acid (FFA) and lipase activity (LA) content.74 Due to the positive association between enzyme activity and water activity, water desorption causes a decrease in lipase activity and free fatty acid content. Similarly, Rousta et al.75 observed that infrared irradiation caused substantial moisture loss in sorghum samples and significant reduction in anti-nutritional component, namely phytate and tannins, and increase in total phenolic component, indicating an increase in the nutritional value of the IR-treated sorghum samples. Increasing the infrared heating intensity decreased the viscosity of sorghum flour according to Swaminathan et al.74 The treatment resulted in substantial changes in the flour moisture content but not whiteness compared to the control. Infrared radiation improved the physicochemical and storage properties of millet flour without affecting its nutritional value. However, research on the microbiological stability of millet flour after infrared heating is scarce.
Traditional thermal treatments are inferior to novel infrared heating methods. Their fast-heating rate lowers the processing time, exposure, and quality losses. This can significantly reduce the energy use, heat load, and heating footprint. These methods improve the thermal efficiency and process management (Sruthi & Rao, 2021).51 The uniform temperature rise from selective heating is another benefit. Also, infrared heating methods are better than heat treatments for millet flour stability because they retain its nutrients, flavor, color, aroma, and texture.
4.3 Green processing techniques of millets
Heat treatment alters food chemically and physically, reducing its organoleptic characteristics and consumer acceptance. Thus, producing a high-quality product without bacterial contamination, with superior storage and functionality becomes the objective. In this case, green processing techniques such as ultrasound processing, high-pressure processing (HPP) and cold plasma, which is also known as non-thermal cereal processing, have become widespread. The above-mentioned non-conventional food processing practices improve food processing sustainability. Unlike typical processing procedures, they work at low temperatures due to physical or chemical restrictions. It has been found that millets undergo structural and compositional changes during the use of thermal processing techniques. As a result, researchers are trying to investigate the applicability of green and non-thermal technologies. These techniques have been shown to reduce the percentage of antinutrients and improve the shelf stability of the products.76,77
There are distinct alterations arising from thermal processing in relation to the choice of green processing method. Millets have the ability to undergo different alterations when subjected to thermal processing procedures such as heat treatment, microwave heating, ohmic heating and infrared heating.
Changes in enzyme activity, structural modifications, and alterations in nutritional characteristics are all included in these transitions. Heat treatment can modify the enzymatic activity of millets, affecting their bioaccessibility, antioxidant capabilities, and anti-proliferative effects. Microwave and infrared heating have the potential to induce alterations in the composition and structure of millets throughout the processing stage.78,79 Green millet processing includes ultrasonic, high pressure, and cold plasma treatment. Non-thermal approaches can reduce the processing time, energy use, and bioactive component retention in millets.47,80 Ultrasound can improve the nutritional content of processed millets by lowering their glycemic index.80 High-pressure processing is another green method for improving the starch functionality of millets.47 The sustainable millet processing techniques are illustrated in Fig. 3.
4.3.1 Ultrasound processing.
Ultrasound (US) is green technology that reduces the processing and maximizes the quality and safety of food. Waves with a frequency in the range of 20–100 kHz are used in this technique.81 High-energy waves propagating across the food medium cause rarefaction and compression cycles, perturbing liquid molecules and altering the structure of food. Acoustic cavitation forms, expands, and collapses gas bubbles to transmit energy, increasing the heat and mass exchange through micro-streaming. The sudden and forceful collapse of the cavitation bubbles causes high pressure of more than 1000 atm and temperature fluctuations up to 500 °C. Thus, cell membranes break, enzymes deactivate, microchannels develop, and free radicals arise.82 Usually, US for food processing involves the use of low frequency US (2–10 MHz), which does not cause any negative alteration in the properties of food.83 Sullivan et al.84 found that ultrasonication for 10 min at 40% amplitude increased the oxygen and nitric oxide radical scavenging and decreased the generation of DPPH in sorghum gluten-like flour. The sonicated samples produced low molecular weight peptides, which improved the antioxidant capabilities against less stable radicals and delayed oxidation. Sonication also improved the hydrolysis, enhancing the digestibility and nutrition. In a related study, Hassan et al.85 determined that ultrasonic processing lowered the content of alkaloids, saponins, tannins, and phytates and increased the content of sterols, flavonoids, phenols, and antioxidants in sorghum sprout-derived flours. It was found that US treatment caused alterations in the structural and physicochemical properties of millet starch, improving its digestibility by 13.3% and a reduction in viscosity.86 Compared with conventional processes, US reduces the processing time and cost, increases the efficiency, eliminates post water treatment, and uses miniscule energy and time needed for processing.83 Because it optimizes the extraction procedures, boosts the energy efficiency, minimizes waste and residues, and processes food products minimally, it is regarded as a sustainable alternative for millet processing.
4.3.2 High pressure processing.
High pressure (HP) processing is a technique in which the products are distributed under equal pressures of 100–800 MPa at normal ambient temperatures (5 °C–40 °C), as shown in Fig. 2. The advantages of using HP over thermal pasteurization is that it can lead to an extension in shelf-life, while preserving thermally labile flavors, nutrients, and color (Ramakrishnan et al., 2023; Tsevdou et al., 2019).87,88 The major advantage of using HP is its accelerated spore inactivation and shorter processing time compared to thermal processing. According to the study by Jwa et al.,89 complete sterilization of foxtail millet takju was obtained at 400 MPa and 66 °C for 60 min. The α-amylase activity was also reduced by 59.7% at 400 MPa for 10 min at 66 °C. Yeast inactivation was achieved at 400 MPa for 10 min under ambient conditions.79,87 The effects of high-pressure soaking on the grains of foxtail millet were investigated by Sharma et al.,90 where in the germinated grains, the gelatinization degree increased significantly from 0.51% to 64.93%, while in the control samples, it increased from 0.32% to 55.21%. In addition, the amount of tannin and phytic acid in the two types of grain samples decreased as a result of the treatment. In the experiment by Gulati et al.,91 proso millet flour was treated with HPP for 5 and 20 min at 200 and 600 MPa, respectively. The results showed that compared to the untreated flour, the HPP-treated flour had less pepsin digestibility. Also, no appreciable variations in pepsin digestibility were noted amongst the treated flour samples.
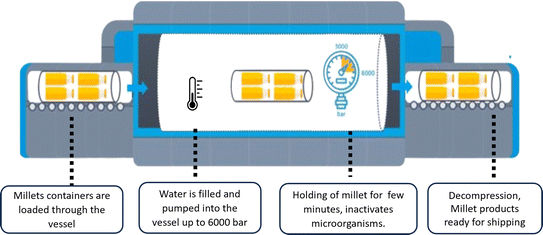 |
| Fig. 2 High-pressure processing of products. | |
Thus, these studies show that besides decreasing the chances of contamination, HP also has minimal effects on the nutritional characteristics of millets. Thus, HP processing can be considered sustainable technology given that it conducts pressure instantly, requires shorter processing times, and consumes lower energy because it is applied at much lower temperatures compared to thermal processing. The reduction in total energy consumption is estimated to decrease by 25–30%. HP processing can significantly reduce microbial activity by inactivation of enzymes and the reduction of spoilage microbes, and hence it extends the shelf life of food. This leads to the decreased disposal of foods, as well as significant reduction in food waste.
4.3.3 Cold plasma.
Cold plasma (CP) is a non-thermal, green processing method that is conducted at room temperature. Because this method is chemical-free and leaves no residue on the sample material, it is considered sustainable. In CP, the sample material is treated with active plasma species generated by electron collision (Joshi et al., 2023).79 It can also be produced at atmospheric and low pressures. Plasma can be produced via microwaves and radio frequencies. However, a major difficulty associated with this method is choosing a plasma source for large millet product batches. Another important factor is consumer acceptance. In the study by Lokeshwari et al.,92 CP technology was carried out with conventional treatment of soaking of pearl millet grains. It was found that applying CP at 180 V with 0.01 m3 h−1 airflow rate reduced the phytic acid concentration by 60.66% (1 h) and 39.27% (2 h). Alternatively, only soaking the grains for 12 h reduced the percentage by only 21.6%.93,94 Owing to its zero production of residues, it can be considered a ‘sustainable’ and ‘clean label’ technique, which can help increase industrial and consumer acceptance.95 Cold plasma, in addition to being a sustainable method, is also effective against microorganisms and helps mitigate pesticide residues in food.95,96
4.4 Enzymatic alterations of millets during sustainable processing
Thermal and green processing technologies affect the enzymatic profile of millets during sustainable processing. Heat treatment such as extrusion, steaming, roasting, and high-temperature treatments can affect the enzymatic activity of millets. Pearl millet extrusion was examined for its effects on lipolytic enzymes and anti-nutritional factors. Under ideal extrusion settings (140 °C/150 rpm/30% moisture), the content of trypsin inhibitors decreased by 100%, phytic acid by 89%, and condensed tannins by 85%. It also inhibited lipase and lipoxygenase by 79.2% and 77.9%, respectively.97 Hydrothermal processing alters the structural and enzymatic characteristics of millets and reduces their rancidity. Hydro treatment (HT), hydrothermal (HTh), and thermal near infrared rays (thNIR) are used to treat pearl millet flour rancidity. The content of lipase (47.8%), lipoxygenase (84.8%), peroxidase (98.1%), and polyphenol oxidase (100%) decreased significantly with HT-HTh-thNIR. The free fatty acid and peroxide levels dropped by 67.84% and 66.4% after 90 days. FTIR confirmed the protein structural changes.98 Microwave, ohmic and infrared heating have the potential to induce alterations in the composition and structure of millets throughout the processing stage.99 Microwave treatment reduced the lipase activity in high-fat pearl millet flour, extending its shelf life.61 In addition, microwave treatment affected the polyphenol concentration of millets differently. Pradeep et al.71 found that microwave treatment dramatically reduced the total phenolic content (TPC) in barnyard millet but increased the TPC, gallon acid, ferulic, gallic, erucic, and cinnamic acid in foxtail and proso millets. Ohmic heating altered the enzyme activities of millets, specifically lipolytic and α-glucosidase. Also, it reduced the lipase activity in millet flour, decreasing its storage stability. Additionally, it induced α-glucosidase inhibitory activity, unlike the control samples. Temperature-treated millet hydrolyzates also blocked angiotensin-converting enzyme (ACE), suggesting antihypertensive effects. These studies show that ohmic heating can improve the functionality and storage stability of millet products.
Ultrasound, high pressure, and plasma can modify the enzymatic properties of millets, changing their nutritional, functional, and technological aspects. The utilization of ultrasound treatment has promise in modulating the enzymatic activity in millets, thereby exerting an influence on many parameters such as lipase activity and protein digestibility.71 Ultrasonication (UA) and enzyme treatment followed by ultrasonication (EUA) were compared to heat reflux (HR) for finger millet seed coat polyphenol extraction. EUA with xylanase (XUA) improved the phenolic yield by 2.3-fold above HR, while UA was equivalent. Also, the content of tannins increased by 1.1-fold and total flavonoids by 1.4-fold in UA and 1.3-fold in XUA. FTIR and ESI-MS research validated the polyphenols in the extracts, including XUA-exclusive substances. This study showed that enzyme treatment and ultrasonication improve FMSC polyphenol extraction, making nutraceutical and functional food production sustainable.100 Mustač et al.101 examined high-intensity ultrasonic pretreatment to boost the bioactives in proso millet bran for functional cereal product development. The optimal treatment (100% amplitude for 9.3 min) boosted the antioxidant activity by 15%, phenolic content by 16%, and dietary fiber by 38%. Ultrasound reduced the browning and increased the water retention; however, polyphenol oxidase activation required tuning. High-pressure processing can impact the functionality, durability, and structural organization of enzymes such as trypsin, pectin methylesterase, and thermolysin.102
Ultra-high-pressure treatment, especially at 600 MPa, increased the enzyme susceptibility in proso millet, improving its starch digestibility and reducing its resistant starch (RS). Under high pressure, starch granule molecules lose hydrogen bonding, causing this result.103 Ultra-high-pressure (UHP) and cold plasma (CP) treatments drastically alter proso millet starch characteristics. UHP at 400 MPa and CP lowered the content of resistant starch (RS), whereas UHP at 600 MPa-CP enhanced the enzyme degradation and molecular chain links, which may promote RS. UHP at 200 MPa-CP for 1 minute resulted in the formation of more RS than native starch, suggesting that it may lower the starch hydrolysis and GI. Higher UHP pressures increased the RS content and diminished the starch digestibility in sorghum starch, lowering its glycemic response.104
5. Product development from various millets
The nutritional benefits and health-enhancing qualities of millet make it an excellent foundation to develop various millet-based products, including vermicelli, extruded flakes, ready-to-eat snacks, instant mixes, baked goods, and more.105 Originally, millets were consumed mostly in the form of cooked rice, but with time, there was a diversification in their consumption patterns. Millet grains that have been partially cooked are crushed into a powdery state, and subsequently used to create round sweets (laddu) and a porridge-like dish made with milk.106 An overview of the commercial millet products available on the market can be seen in Table 3. Furthermore, millets are utilized in the preparation of many local traditional dishes such as porridge, dosa, chapati, bread, pasta, biscuits, and ready-to-eat meals.107Table 4 highlights traditional millet products from around the world, showcasing their significance and diversity in various cultures and regions. Millet-based items and beverages are a fundamental part of the daily diet in many African nations.
Table 3 List of some commercial millet products that are considered supergrains at the global level
Commercial product |
Type of millet used |
Used as supergrains |
References |
Sorghum mini pops, Jowar pops |
Sorghum, Jowar |
Antiproliferative, antioxidant, anti-diabetic, and anti-inflammatory actions as well as reducing plaque deposits |
Saleem et al.108 |
Organic millet flakes |
All millets |
Systolic blood pressure reduction through lowering angiotensin converting enzyme activity and antioxidative and anti-inflammatory effect; processing enhances total flavonoid content (TFC), and gamma aminobutyric acid (GABA) |
Gull et al.109 |
Millet pancake |
Sorghum & foxtail flour |
High density lipoprotein (HDL) increase, and diminished low density lipoproteins (LDL), cholesterol, glycosylated hemoglobin, and serum glucose |
Saleem et al.108 |
Ragi vermicelli |
Ragi + wheat |
Slow aging through inhibition of collagen cross-linking thus keeping flexibility in tissues and skin |
Gull et al.109 |
Ragi cookies |
Ragi flour + wheat refined flour |
Coat of finger millet seeds is higher in polyphenols than flour and possesses potent antifungal and antibacterial qualities |
Mahajan et al.77 |
Instant mix-Ragi idli |
Finger millet |
Helps in lowering glycemic index, and significant reduction in cholesterol |
Saleh et al.;110 Selladurai et al.111 |
Ragi bites – Choco poco |
Ragi + jowar |
Shown to have high antioxidant and anti-inflammatory levels |
Jaybhaye et al.112 |
Millet Chinese poha |
Kodo millet flaxes |
Availability of high polyphenols helps in reducing oxidative stress |
Selladurai et al.111 2023b |
Millet upma mix |
Little mix |
Shown to have high antioxidant and anti-inflammatory levels |
Anandharamakrishnan et al.76 |
Millet kichadi mix |
Foxtail millet |
Helps in reducing serum triglyceride levels, liver lipid accumulation and total cholesterol; ease of digestion |
Saleem et al.108 |
Table 4 List of traditional millet products worldwidea
Regional name |
Source |
Product type |
Region |
Source: Anandharamakrishnan et al.;76 Jaybhaye et al.;112 Kumar et al.113
|
Koko |
Pearl millet |
Porridge |
West Africa |
Fura |
Pearl millet |
Porridge |
Sahel |
Togwa |
Finger millet |
Sweet beer |
Tanzania |
Ogi |
Sorghum |
Porridge |
West Africa |
Malt |
Foxtail/barnyard/finger/sorghum |
Beverage |
India |
Vermicelli |
Finger/pearl millet |
Pasta |
India |
Kola Puttu |
Finger/foxtail |
Breakfast food |
South India |
Researchers have investigated the potential use of millet to develop food products that have enhanced nutritional contents and nutraceutical properties. These products include extruded and expanded products, 3-D printed millet products, bakery products (such as biscuits, breads, muffins, and waffles), porridges, pasta and noodles, and alcoholic and non-alcoholic beverages.
5.1 Millet-based baked products
The low costs and greater variety in textures and tastes of the bakery products of millets have increased their popularity and marketability across the globe.114 Thus, the implementation of millets due to their superiority in fiber and micronutrients creates a valuable addition to the market.115 Millet flour is used in gluten-free baked goods due to the increase in gluten intolerance. Biscuits and pastries are being made with finger millet and foxtail millet instead of wheat flour. Gluten-free bread production relies on minor millets and up to 40% non-wheat flour. Millet composite flour bread is developed chemically using the standard method of 50, 35, and 30 ppm of ascorbic acid, cystine, and potassium bromate, respectively.76,116 However, despite these advances, wheat is still a necessary inclusion in most millets bread. Non-wheat recipes utilizing only millet varieties of sorghum and pearl millet generated cookies; however, this resulted in poor physical qualities such as toughness and grittiness. Overall, these products lacked the necessary desirable traits.112
5.2 Extruded and expanded products of millets
In the food sector, extrusion cooking is a commonly used method that makes it possible to produce a wide range of food products, including wafers and snacks. Its automation, large capacity, user friendliness, energy efficiency, inactivation of anti-nutritional substances, variety in shaping, and structural change by shearing action make it popular.116,117
Millets are now an upcoming raw material source for extrusion. They are rich in almost all nutrients, and additionally they are gluten free. These attributes and health benefits make them a suitable ingredient for the production of RTE snacks, composite flour, flaked products, and other extruded products. Millet pellets have been developed using extrusion. The raw material is dough from millet milk powder, which has been granulated to obtain a uniform texture.33 Various other research has been ongoing to use millet with other grains or cereals to develop a healthy food alternative. However, one of the major challenges is the presence of antinutrients in millets. According to Jaybhaye et al.,112 extrusion increased the iron availability of extruded weaning products based in pearl millet, cowpea, and peanut compared to conventional weaning foods. Various other studies have also concluded that extrusion enhances the nutritional attributes of millet-based composite flours and can be considered as an excellent alternative nutrient-dense product.112,118
5.3 Beverages from millets
Various alcoholic and non-alcoholic beverages have been prepared traditionally across Asia and Africa for a very long time. These products can now be considered novel products because of their commercialization potential and potential health benefits. These products can also be considered sustainable given that they are prepared with minimal processing techniques and processed mostly to remove antinutritional compounds from the product itself. Some alcoholic and non-alcoholic products include koozh, koko, togwa, dambu, chhyang, ogi, uji, and. Mangisi is an alcoholic beverage from Africa, which is prepared through natural fermentation. Malted finger millet is allowed to stand, mixed with water, and allowed cool, where spontaneous fermentation allows the development of mangisi.76 Bushera is another malted, fermented beverage from Uganda, Africa. During the malting process, wood ash is mixed, which enhances the zinc, magnesium, and iron contents in the grains.76 The Nigerian fermented drink known as kunun zaki is non-alcoholic. Mostly, it is drunk as a weaning beverage, appetizer, and morning beverage. However, it has a limited shelf-life owing to its high moisture content.107 Chhyang is another popular fermented drink among the Indian subcontinent, particularly India and Nepal. It is nutritious as well as cheap. Finger millet is mostly used for its production. It is mostly used among the local population as a remedy against the common cold and fever.76,107 Amalgamation of traditional techniques with modern technologies will allow for the greater commercialization as well as availability of these products among consumers.
5.4 3-D printing of millet products
3D printing innovates snack food manufacturing. Three-dimensional printing allows snack food manufacturers to make customized goods, which is a fast-growing market. With this technique, meat-based snacks and analogues of meat-based snacks using millet protein can be made with improved nutritional, physical, and sensory qualities to suit customer demands for convenient and functional food.119,120 Sanuujabertini et al.121 investigated the rheology and 3-D printability of millet-based dough. Despite the presence of xanthan gum, the storage and loss moduli of the dough increased with the angular frequency, suggesting that it had an elastic gel-like structure and potential to correct nutritional deficiencies, providing sustainable solutions to malnutrition. In the case of 3-D edible inks, S. Ghimire122 combined raw and fermented finger millet flour with plant-based proteins such mung bean, pea, and fava bean. The textural qualities of the meat analogue samples were considerably enhanced (p < 0.05) in all protein-based formulations by the inclusion of millet flour, whether it was raw or fermented. Krishnaraj et al.120 developed healthy 3D-printed snacks using composite flour, which included barnyard millet. The snacks demonstrated favorable customer reception, indicating their promising commercial viability. This study contributes to the progress of creating high-fiber foods using a variety of plant sources, with a particular focus on solutions that are appealing to consumers.
6. Digestibility of millets
Although millets are a good source of nutritional value, they are often rich in anti-nutritional factors associated with fiber, which interfere with nutrient absorption in the human body and reduce mineral bioavailability. Also, there are many factors that affect digestibility including the starch structure, amylose to amylopectin ratio, and presence of lipids, proteins, fiber, or antinutrients.63 Plant storage proteins, which are found in cereal grains and legumes, typically exhibit reduced digestion compared to animal proteins.123 This can occur for several reasons, such as the inhibition of digestive enzymes by tannins or protease inhibitors, the limited solubility of proteins, the arrangement of proteins into protein bodies, and the decreased accessibility of enzymes because of rigid seed coverings and/or cell walls.123
Significant research has been done on how processing affects the chemical components of millet. One major drawback is its digestibility, given that the digestibility of starch is inhibited by the lipid and protein content, and protein digestion is disrupted by phytic acid and tannin quantities.124 Conventional and modern processing reduce these nutritional factors but may negatively alter the overall nutritional characteristics.125 Hence, the impact of different food-processing techniques on the digestibility of millet and millet-based products has emerged as a significant field of research. A review of the processing techniques aimed at improving the digestibility of millets is illustrated in Table 5 and Fig. 4. The following section discusses the digestibility of millet-based foods after four processes including soaking, germination, cooking, and fermentation.
Table 5 Summary of processing methods that can improve the digestibility of millets
Method |
Millet |
Result |
Reference |
Soaking |
Foxtail |
The IVPD value increased from 62.3% to 76% |
Pawar and Machewad134 |
Dehulling, soaking, and cooking techniques were applied to foxtail millet, the IVPD rose from 62.3% in untreated millet to 83% |
Germination |
Barnyard |
Tannin content decreased from 1.594 to 0.657 mg/100 g |
Sharma et al.140 |
Phytate content decreased from 0.125 to 0.099 mol kg−1 |
Finger |
51.11% decrease in phytic acid after 48 h of germination at 25 °C |
Azeez et al.132 |
Foxtail |
After 4 days of germination |
Kumari et al.130 |
Tannin content decreased from 0.33 to 0.02 mg g−1 |
Saponin content decreased from 0.39 to 0.02 mg g−1 |
Phytic acid decreased from 6.40 to 2.67 mg g−1 |
Proso |
After 4 days of germination |
Tannin content decreased from 0.51 to 0.05 mg g−1 |
Saponin content decreased from 0.44 to 0.07 mg g−1 |
Phytic acid decreased from 8.09 to 4.86 mg g−1 |
Cooking |
Foxtail |
The IVPD resulted in values exceeding 80% |
Pawar and Machewad134 |
Proso |
Proso millet that had been pan-cooked, microwave-cooked, or uncooked had starch digestibility percentages of 57.18%, 42.20%, and 43.73%, respectively |
Kumar et al.63 |
Little |
The starch digestibility percentages of small millet that was raw, pan-cooked, and microwave-cooked were 41.96%, 29.17%, and 30.09%, respectively |
Fermentation |
Finger |
56.63% PA concentration reduction after fermentation at 27 °C for 16 h |
Azeez et al.132 |
The combined method of germination and fermentation can decrease the phytic acid content by 59.57% |
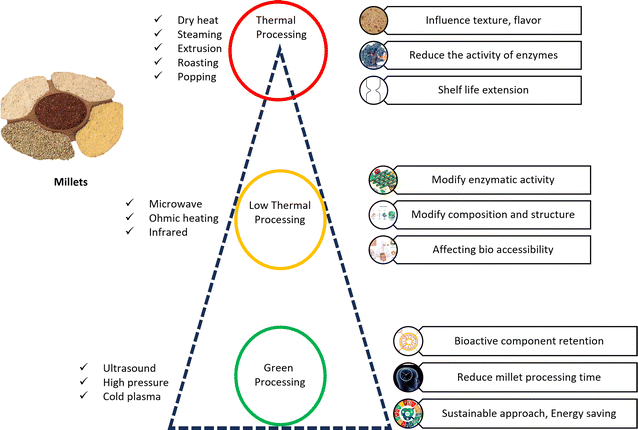 |
| Fig. 3 Sustainable millet processing techniques. | |
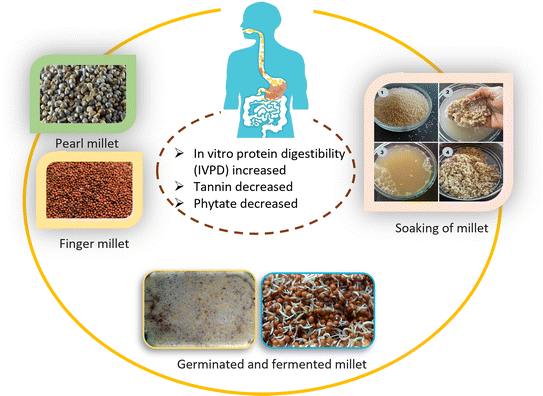 |
| Fig. 4 Digestibility of processed (soaked, germinated and fermented) millets. | |
6.1 Soaking
Immersion in water can reduce the level of the activation of internal phytase and the discharge of phytic acid in the soaking water. Soaking whole rice grains in acidic conditions (45 °C for 48 h) resulted in the removal of approximately 91% of phytic acid. The decrease in phytic acid content was attributed to the stimulation of endogenous phytase and the release of phytic acid in the soaking water. The in vitro protein digestibility (IVPD) value increased from 62.3% to 76% after soaking. The combination of dehulling, soaking, and cooking methods led to an increase in IVPD from 62.3% in untreated foxtail millet to 83%.126 Jha et al.127 soaked bran- and endosperm-rich pearl millet fractions in different pH solutions and germinated and steamed them. Iron, zinc, polyphenols, phytic acid, fiber, and flavonoids were abundant in bran. Short soakings lowered the pH-dependent inhibitory factors. In the bran-rich fraction, alkaline soaking reduced the content of flavonoids, while acidic soaking reduced the phytic acid content. Acids increased the zinc and iron bioavailability. Soaking was examined on little, foxtail, pearl, finger, and Kodo millets. Nutrition was optimized with 24 h of soaking. Longer soaking reduced the tannin content in little millet. Little millet exhibited the greatest phytase activity and pearl millet the most saponin. Long-term soaking increased the antioxidant activity of foxtail millet.128
6.2 Germination/malting
Germination/malting is an easy process that involves the transformation and production of bioactive substances through enzymatic pathways, which begin when grains are soaked, which also improve the grain quality and reduce anti-nutrients.129 During germination, phytase breaks down phytate phosphorus into inositol monophosphate, lowering the content of phytic acid. Furthermore, the tannin levels dropped significantly. Tannin molecules create hydrophobic interactions with the seed proteins and enzymes. The content of tannins may also decrease during germination due to their absorption in water and polyphenol interaction with proteins and carbohydrates.130 Prasad et al.131 examined how germination treatment affected white quinoa, proso, and foxtail millet flour. The total starch, amylose, amylopectin, polyphenols, flavonoids, in vitro protein digestibility, and glycemic potential of the flours changed dramatically. Germination affected the physicochemical, antioxidant, digestibility, functional, and thermal properties of brown finger millet. Germination and fermentation enhanced the protein, crude fiber, minerals, resistant starch, total flavonoid and phenolic content, and antioxidants. They reduced the antinutritional factors, digestible starch, and increased the amino acid profile and in vitro protein digestibility.132
6.3 Cooking
Cereals are primarily composed of three types of starch, i.e., rapid digestive starch, slow digestive starch, and resistant starch. Kumar et al.63 observed that the digestibility of millet starch was higher with pan cooking in comparison to microwave heating. The digestibility of starch in vitro is influenced by several parameters, including the amylose concentration, degree of crystallinity, amylose–lipid complexes, and the molecular structure of amylopectin. Annor et al.133 claimed that in contrast to rice, the hard granule shape of starches derived from finger millet was resist digestive enzymes. The digestibility was observed to increase after cooking.134 According to Roopa and Premavalli,135 roasting or baking of different varieties of finger millets reduced the amount of starch that could be digested compared to boiling in water. The starch digestibility of finger millet was enhanced by either puffing the grains or pressure-cooking the flour, which resulted in more pronounced starch gelatinization.
The protein digestibility of millet was enhanced through the process of cooking. Several processes were suggested by Duodu et al.136 to elucidate the impact of heating on in vitro protein digestibility (IVPD) of sorghum, as follows: (1) the low ability of uncooked materials to be digested is mainly because of the presence of heat-sensitive substances that inhibit protein breakdown; (2) the process of cooking causes proteins to lose their natural structure and become more susceptible to enzyme breakdown; and (3) when proteins are cooked, they can interact with non-protein substances or other proteins, which can impact their ability to be digested. All these factors affect the digestibility of sorghum protein. Multiple factors may work based on the sorghum grain type such as whole grain, endosperm, protein body preparation, high-tannin, and condensed-tannin-free.
6.4 Fermentation
Fermentation, a well-known technique for digesting plant foods that also reduces the presence of anti-nutrient elements, improves the nutritional value, antioxidant, health-promoting, and functional qualities of cereals and legumes.137 Various conventional millet products and beverages undergo fermentation, either alone by lactic acid bacteria or in conjunction with yeasts. Nutritional quality is enhanced by lactic fermentation through the increased digestibility of proteins and starch (Annor et al., 2017).133 The proteolytic activity during fermentation breaks down complex storage proteins into soluble molecules and degrades tannins, polyphenols, and phytic acid, improving the protein digestibility. Enzymatic pretreatment and targeted fermentation with cellulase and hemicellulose speed up the protein accessibility of finger millet. Enzymatic digestion of dietary fibers releases seed protein, lowering the phytate and tannin content and enhancing the digestibility. Microflora may hydrolyze starch during millet fermentation, and a decrease in the content of phytic acid increases the digestibility of starch.138 Jan et al.139 studied finger millet flour fermentation using Lactobacillus strains, yeast, and their combinations across varying periods. Lactobacillus plantarum and yeast + ammonium sulfate fermentation increased the overall phenolic content and antioxidant activity. It increased the crude protein and minerals and decreased the crude fiber, fat, phytic acid, and tannins. Fermentation improved the digestibility and nutritional value of finger millet flour, making it a viable nutritional enhancer.
7. Conclusion and future perspectives
Millets as supergrains present a solution with wide-ranging advantages, including economic efficiency, climate resilience, and alignment with the important Sustainable Development Goals (SDGs) of the UN. Innovative processing methods that preserve nutritional value, while minimizing environmental impact and reducing waste, such as low thermal methods such as microwave and infrared processing and non-thermal methods such as cold plasma and high-pressure processing, further improve the sustainability of millets. The potential for the commercialization and broad public acceptance of millet-based products, which include baked foods, beverages, and even 3D-printed alternatives, increases as they gain popularity and provide a variety of sustainable and nutrient-dense options. However, there are still issues with the digestion of millets that must be resolved for them to be used to their full potential. Anti-nutritional elements can decrease the bioavailability of minerals and obstruct the absorption of nutrients, such as fiber-associated chemicals. There are several factors that have been shown to affect the digestibility of millets, including the structure of their starch, ratio of amylose to amylopectin, and presence of proteins, lipids, and antinutrients. It has been found that soaking, germination/malting, boiling, and fermentation are useful techniques to improve their digestibility. Soaking has been demonstrated to lower the content of phytic acid, whilst germination and malting dramatically lower the levels of anti-nutrient elements. Fermentation increases the protein and starch digestibility due to microbial enzyme activity, while cooking can either slightly decrease or slightly increase the starch digestibility depending on the technique used.
Integrating millets into diets globally will require multidisciplinary cooperation necessitating agricultural expansion, government support and regulations favoring more sustainable products, further investment in research and development, and continued consumer education to reveal the ecological and health benefits associated with millet use. Prospects for the future can include further investigation into the possibilities of millet hulls.
The nutritional profile of millet-based products can be improved and waste can be decreased by investigating novel ways to use millet hulls, such as a source of dietary fiber or in the creation of functional food ingredients. Furthermore, more investigation into refining processing methods and how they affect the digestibility of millets may maximize their nutritional advantages. Thus, to fully realize the potential of millets, which are emerging as key components of future food systems, multidisciplinary cooperation among the fields of agriculture, nutrition, and food technology is needed. Through the resolution of digestibility issues and the optimization of millet by-product applications, we can create a more robust, resilient, and sustainable global food system.
Conflicts of interest
There are no conflicts to report.
References
-
FAO, The Future of Food and Agriculture—Alternative Pathways to 2050, Food and Agriculture Organization of the United Nations, Rome, Italy, 2018 Search PubMed.
- H. Aiking and J. de Boer, The next protein transition, Trends Food Sci. Technol., 2020, 105 Search PubMed.
-
World Food Programme, Global Report on Food Crises, 2022 Search PubMed.
-
H. Ritchie, Food Production Is Responsible for One-Quarter of the World's Greenhouse Gas Emissions, Our World Data, 2019 Search PubMed.
- M. Cheng, B. McCarl and C. Fei, Climate Change and Livestock Production: A Literature Review, Atmosphere, 2022, 13 CAS.
- G. S. Malhi, M. Kaur and P. Kaushik, Impact of climate change on agriculture and its mitigation strategies: A review, Sustain., 2021, 13 Search PubMed.
- H. L. Tuomisto and M. J. Teixeira De Mattos, Environmental impacts of cultured meat production, Environ. Sci. Technol., 2011, 45, 6117–6123 CrossRef CAS PubMed.
- N. R. Rubio, N. Xiang and D. L. Kaplan, Plant-based and cell-based approaches to meat production, Nat. Commun., 2020, 11, 1–11 CrossRef PubMed.
- A. G. A. Sá, Y. M. F. Moreno and B. A. M. Carciofi, Plant proteins as high-quality nutritional source for human diet, Trends Food Sci. Technol., 2020, 97, 170–184 CrossRef.
- M. L. Wilson and R. VanBuren, Leveraging millets for developing climate resilient agriculture, Curr. Opin. Biotechnol., 2022, 75 Search PubMed.
- Z. M. Hassan, N. A. Sebola and M. Mabelebele, The nutritional use of millet grain for food and feed: a review, Agric. Food Secur., 2021, 10 Search PubMed.
- A. Porwal, G. Bhagwat, J. Sawarkar, P. Kamble and M. Rode, An overview of millets-the nutri-cereals: Its nutritional profile, potential health benefits and sustainable cultivation approach, Int. J. Sci. Res. Arch., 2023, 10, 841–859 CrossRef.
- P. Banerjee and S. Maitra, The Role of Small Millets as Functional Food to Combat Malnutrition in Developing Countries, Indian J. Nat. Sci., 2020, 10, 20412–20417 Search PubMed.
- S. A. Kheya, S. K. Talukder, P. Datta, S. Yeasmin, M. H. Rashid, A. K. Hasan, M. P. Anwar, A. K. M. A. Islam and A. K. M. M. Islam, Millets: The future crops for the tropics - Status, challenges and future prospects, Heliyon, 2023, 9, e22123 CrossRef PubMed.
- S. Padulosi, B. Mal, O. I. King and E. Gotor, Minor millets as a central element for sustainably enhanced incomes, empowerment, and nutrition in rural India, Sustain, 2015, 7, 8904–8933 CrossRef.
- C. Grovermann, K. B. Umesh, S. Quiédeville, B. Ganesh Kumar, S. Sakamma and S. Moakes, The economic reality of underutilised crops for climate resilience, food security and nutrition: Assessing finger millet productivity in India, Agric, 2018, 8, 131–142 Search PubMed.
- L. Kumar, R. K. Naresh, H. Tiwari, S. K. Kataria, S. Saharan, B. R. Reddy, O. Singh, S. Qidwai and R. P. Singh, Millets for Food and Nutritional Security in the Context of Climate Resilient Agriculture: A Review, Int. J. Plant Soil Sci., 2022, 34, 939–953 CrossRef.
-
UN Global, UN Climate Change Annual Report, 2022 Search PubMed.
- S. Anitha, D. I. Givens, K. Subramaniam, S. Upadhyay, J. Kane-Potaka, Y. D. Vogtschmidt, R. Botha, T. W. Tsusaka, S. Nedumaran, H. Rajkumar, A. Rajendran, D. J. Parasannanavar, M. Vetriventhan and R. K. Bhandari, Can Feeding a Millet-Based Diet Improve the Growth of Children?—A Systematic Review and Meta-Analysis, Nutrients, 2022, 14(1), 255 CrossRef PubMed.
- S. B. Ahirwar, T. N. Srinatha, T. N. Dhanalakshmi, A. Bharti, G. Patidar, N. Nandeha and D. Mukherjee, Review on Millets: A Sustainable Ancient Superfood for the Modern World, Int. J. Plant Soil Sci., 2023, 35, 278–287 CrossRef.
-
M. K. Tripathi, D. Mohapatra, R. S. Jadam, S. Pandey, V. Singsh, V. Kumar and A. Kumar, Nutritional Composition of Millets, Millets and Millet Technology, 2021, pp. 101–119 Search PubMed.
-
B. Dayakar Rao, K. Bhaskarachary, G. D. Arlene Christina, G. Sudha Devi and A. T. Vilas, Nutritional and Health Benefits of Millets, ICAR_Indian Institute of Millets Research (IIMR), Rajendranagar, Hyderabad, 2017, pp. 1–2 Search PubMed.
- D. Chandra, S. Chandra and A. K. Sharma, Review of Finger millet (Eleusine coracana (L.) Gaertn): A power house of health benefiting nutrients, Food Sci. Hum. Wellness, 2016, 5, 149–155 CrossRef.
- J. Y. Kim, K. C. Jang, B. R. Park, S. I. Han, K. J. Choi, S. Y. Kim, S. H. Oh, J. E. Ra, T. J. Ha, J. H. Lee, J. Hwang, H. W. Kang and W. D. Seo, Physicochemical and antioxidative properties of selected barnyard millet (Echinochloa utilis) species in Korea, Food Sci. Biotechnol., 2011, 20, 461–469 CrossRef CAS.
- V. Wiedemair, S. Scholl-Bürgi, D. Karall and C. W. Huck, Amino Acid Profiles and Compositions of Different Cultivars of Panicum miliaceum L, Chromatographia, 2020, 83, 829–837 CrossRef CAS.
- X. S. Yang, L. L. Wang, X. R. Zhou, S. M. Shuang, Z. H. Zhu, N. Li, Y. Li, F. Liu, S. C. Liu, P. Lu, G. X. Ren and C. Dong, Determination of protein, fat, starch, and amino acids in foxtail millet [Setaria italica (L.) Beauv.] by Fourier transform near-infrared reflectance spectroscopy, Food Sci. Biotechnol., 2013, 22, 1495–1500 CrossRef CAS.
- S. Shobana, Y. N. Sreerama and N. G. Malleshi, Composition and enzyme inhibitory properties of finger millet (Eleusine coracana L.) seed coat phenolics: Mode of inhibition of α-glucosidase and pancreatic amylase, Food Chem., 2009, 115, 1268–1273 CrossRef CAS.
- V. Viswanath, A. Urooj and N. G. Malleshi, Evaluation of antioxidant and antimicrobial properties of finger millet polyphenols (Eleusine coracana), Food Chem., 2009, 114, 340–346 CrossRef CAS.
-
V. C. Verma, S. Agrawal, M. K. Tripathi and A. Kumar, Millet Starch: Current Knowledge and Emerging Insights of Structure, Physiology, Glycaemic Attributes and Uses, Springer, Singapore, 2021 Search PubMed.
- A. Tiwari, K. Kesarwani, A. Sharma, T. Ghosh, N. Bisht and S. Punetha, Drought Stress in Millets and Its Response Mechanism, Adv. Plant Def. Mech., 2022, 1–35 CAS.
- N. B. Mohod, P Ashoka, A. Borah, P. Goswami, A. K. Koshariya, S. Sahoo and N. Prabhavathi, The International Year of Millet 2023: A Global Initiative for Sustainable Food Security and Nutrition, Int. J. Plant Soil Sci., 2023, 35, 1204–1211 CrossRef.
- T. W. Reynolds, S. R. Waddington, C. L. Anderson, A. Chew, Z. True and A. Cullen, Environmental impacts and constraints associated with the production of major food crops in Sub-Saharan Africa and South Asia, Food Secur., 2015, 7, 795–822 CrossRef.
-
R. Ravikesavan, A. P. Sivamurugan, K. Iyanar, J. L. Pramitha and A. Nirmalakumari, in Handbook of Millets - Processing, Quality, and Nutrition Status, 2022, pp. 23–48 Search PubMed.
- A. Kumar, V. Tomer, A. Kaur, V. Kumar and K. Gupta, Millets: A solution to agrarian and nutritional challenges, Agric. Food Secur., 2018, 7, 1–15 CrossRef.
- P. S. J. Michaelraj and a Shanmugam, a Study on Millets Based Cultivation and Consumption in India, Int. J. Mark. Financ. Serv. Manag. Res., 2013, 2, 49–58 Search PubMed.
- A. K. Jukanti, C. L. L. Gowda, K. N. Rai, V. K. Manga and R. K. Bhatt, Crops that feed the world 11. Pearl Millet (Pennisetum glaucum L.): an important source of food security, nutrition and health in the arid and semi-arid tropics, Food Secur., 2016, 8, 307–329 CrossRef.
-
R. P. Meena, D. Joshi, J. K. Bisht and L. Kant, Global Scenario of Millets Cultivation, Millets and Millet Technology, 2021, pp. 33–50 Search PubMed.
- C. T. Satyavathi, S. Ambawat, V. Khandelwal and R. K. Srivastava, Pearl Millet: A Climate-Resilient Nutricereal for Mitigating Hidden Hunger and Provide Nutritional Security, Front. Plant Sci., 2021, 12, 1–18 Search PubMed.
- C. M. Matanyaire, Sustainability of pearl millet (Pennisetum glaucum) productivity in northern Namibia: Current situation and challenges, S. Afr. J. Sci., 1998, 94, 157–166 CAS.
- B. K. Dhaka, P. Dhaka, B. Singh, A. Kamal and A. K. Dhaka, Intercropping Options for Sustainable Minor Millet Production: A Review, Int. J. Environ. Clim. Chang., 2023, 13, 89–100 CrossRef.
- R. Devkota, K. Khadka, H. Gartaula, A. Shrestha, S. Karki, K. Patel and P. Chaudhary, Gender and labour efficiency in finger millet production in Nepal, Transform. Gend. Food Secur. Glob. South, 2016, 76–95 Search PubMed.
- T. L. Goron and M. N. Raizada, Genetic diversity and genomic resources available for the small millet crops to accelerate a New Green Revolution, Front. Plant Sci., 2015, 6, 132042 Search PubMed.
-
U. G. Assembly, in Resolut. Adopt.by Gen. Assem. 25 Sept. 2015 16301, 2016, pp. 2–5 Search PubMed.
- M. J. Feldman, P. Z. Ellsworth, N. Fahlgren, M. A. Gehan, A. B. Cousins and I. Baxter, Components of water use efficiency have unique genetic signatures in the model C4 grass setaria1, Plant Physiol., 2018, 178, 699–715 CrossRef CAS PubMed.
- D. Saha, M. V. C. Gowda, L. Arya, M. Verma and K. C. Bansal, Genetic and Genomic Resources of Small Millets, CRC. Crit. Rev. Plant Sci., 2016, 35, 56–79 CrossRef CAS.
-
B. D. Rao, T. M. Dinesh, Anuradha and D. Nune, Millets Pseudo Cereal Genetic Resources and Breeding Advancements, 2021, pp. 185–201.
- H. Wang, Y. Fu, Q. Zhao, D. Hou, X. Yang, S. Bai, X. Diao, Y. Xue and Q. Shen, Effect of Different Processing Methods on the Millet Polyphenols and Their Anti-diabetic Potential, Front. Nutr., 2022, 9, 1–8 CAS.
- N. Sharma, J. K. Sahu, V. Bansal, O. J. Esua, S. Rana, A. Bhardwaj, S. Punia Bangar and A. A. Adedeji, Trends in millet and pseudomillet proteins - Characterization, processing and food applications, Food Res. Int., 2023, 164, 112310 CrossRef CAS PubMed.
- B. Bucsella, Á. Takács, V. Vizer, U. Schwendener and S. Tömösközi, Comparison of the effects of different heat treatment processes on rheological properties of cake and bread wheat flours, Food Chem., 2016, 190, 990–996 CrossRef CAS PubMed.
- M. Gómez and M. M. Martínez, Changing flour functionality through physical treatments for the production of gluten-free baking goods, J. Cereal Sci., 2016, 67, 68–74 CrossRef.
- N. U. Sruthi and P. S. Rao, Effect of processing on storage stability of millet flour: A review, Trends Food Sci. Technol., 2021, 112, 58–74 CrossRef CAS.
-
B. K. Tiwari, T. Norton, & N. M. Holden, Sustainable Food Processing, 2023 Search PubMed.
- P. Bora, S. Ragaee and M. Marcone, Effect of parboiling on decortication yield of millet grains and phenolic acids and in vitro digestibility of selected millet products, Food Chem., 2019, 274, 718–725 CrossRef CAS PubMed.
- D. Bhati, V. Bhatnagar and V. Acharya, Effect of pre-milling processing techniques on pearl millet grains with special reference to in vitro iron availability, Asian J. Dairy Food Res., 2016, 35, 12–13 Search PubMed.
- N. Singh Scholar, J. David Professor, D. Thompkinson Professor Emeritus, B. Sagar Seelam Scholar, H. Rajput Scholar, S. Morya Scholar, C. Neha Singh Scholar, N. Singh, J. David, D. Thompkinson, B. Sagar Seelam, H. Rajput and S. Morya, Effect of roasting on functional and phytochemical constituents of finger millet (Eleusine coracana L.), Pharma Innov. J., 2018, 7, 414–418 Search PubMed.
- S. S. Patil, S. G. Rudra, E. Varghese and C. Kaur, Effect of extruded finger millet (Eleusine coracan L.) on textural properties and sensory acceptability of composite bread, Food Biosci., 2016, 14, 62–69 CrossRef CAS.
- L. Devraj, V. Natarajan, S. Vadakkeppulpara Ramachandran, L. Manicakam and S. Sarvanan, Influence of microwave heating as accelerated aging on physicochemical, texture, pasting properties, and microstructure in brown rice of selected Indian rice varieties, J. Texture Stud., 2020, 51, 663–679 CrossRef PubMed.
- M. Naik, V. Natarajan, A. Rawson, J. Rangarajan and L. Manickam, Extraction kinetics and quality evaluation of oil extracted from bitter gourd (Momardica charantia L.) seeds using emergent technologies, LWT, 2021, 140, 110714 CrossRef CAS.
- M. V. Rao, K. G. Akhil, C. K. Sunil, N. Venkatachalapathy and R. Jaganmohan, Effect of microwave treatment on physical and functional properties of foxtail millet flour, Int. J. Chem. Stud., 2021, 9, 2762–2767 CrossRef.
- A. Singh, S. Gupta, R. Kaur and H. R. Gupta, Process optimization for anti-nutrient minimization of millets, Asian J. Dairy Food Res., 2017, 011, 2022 Search PubMed.
- D. N. Yadav, T. Anand, J. Kaur and A. K. Singh, Improved Storage Stability of Pearl Millet Flour Through Microwave Treatment, Agric. Res., 2012, 1, 399–404 CrossRef.
- H. S. Tabarestani, S. M. Khorasani, M. Alami and M. Kashaninejad, Increasing the shelf life of millet flour by using heat-moisture and microwave treatments, J. Food Sci. Technol., 2019, 16, 5–8 Search PubMed.
- S. R. Kumar, M. B. Sadiq and A. K. Anal, Comparative study of physicochemical and functional properties of pan and microwave cooked underutilized millets (proso and little), LWT, 2020, 128, 109465 CrossRef CAS.
- D. Gopal and S. Bhuvana, Effect of microwave treatment on phytochemical, functional and rheological property of Kodo millet (Paspalum scrobiculatum), Pharma Innov. J., 2021, 10, 397–401 CrossRef.
-
R. M. Rodrigues, A. A. Vicente, A. J. Teixeira and R. N. Pereira, Ohmic Heating-An Emergent Technology in Innovative Food Processing, Elsevier Inc, 2021 Search PubMed.
- Y. Turgut, S. S. Turgut and E. Karacabey, Use of ohmic heating as an alternative method for cooking pasta, J. Sci. Food Agric., 2021, 101, 5529–5540 CrossRef CAS PubMed.
- W. Jittanit, K. Khuenpet, P. Kaewsri, N. Dumrongpongpaiboon, P. Hayamin and K. Jantarangsri, Ohmic heating for cooking rice: Electrical conductivity measurements, textural quality determination and energy analysis, Innov. Food Sci. Emergy Technol., 2017, 42, 16–24 CrossRef CAS.
- B. Jan, R. Shams, Q. E. H. Rizvi and A. Manzoor, Ohmic heating technology for food processing: a review of recent developments, J. Postharvest Technol., 2021, 9, 20–34 Search PubMed.
- A. M. Dias-Martins, L. P. Cappato, M. da Costa Mattos, F. N. Rodrigues, S. Pacheco and C. W. P. Carvalho, Impacts of ohmic heating on decorticated and whole pearl millet grains compared to open-pan cooking, J. Cereal Sci., 2019, 85, 120–129 CrossRef CAS.
- N. Mishra, Y. Jyoti and S. Jain, Role of ohmic heating in stabilization of pearl millet: An overview, J. Pharm. Innov., 2021, 10, 232–234 Search PubMed.
- P. M. Pradeep and Y. N. Sreerama, Impact of processing on the phenolic profiles of small millets: Evaluation of their antioxidant and enzyme inhibitory properties associated with hyperglycemia, Food Chem., 2015, 169, 455–463 CrossRef CAS PubMed.
- P. Sakare, N. Prasad, N. Thombare, R. Singh and S. C. Sharma, Infrared Drying of Food Materials: Recent Advances, Food Eng. Rev., 2020, 12, 381–398 CrossRef.
-
R. Ramaswamy, K. Krishnamurthy and S. Jun, Microbial Decontamination of Food by Infrared (IR) Heating, Woodhead Publishing Limited, 2012 Search PubMed.
- I. Swaminathan, M. Guha, U. H. Hunglur and D. B. Rao, Optimization of infrared heating conditions of sorghum flour using central composite design, Food Sci. Biotechnol., 2015, 24, 1667–1671 CrossRef CAS.
- E. Beam and I. Radiation, Effect of Gamma, Electron Beam and Infrared Radiation Treatment on the Nutritional Value and Anti-Nutritional Factors of Sorghum Grain, Iran. J. Appl. Anim. Sci., 2014, 4, 723–731 Search PubMed.
-
C. Anandharamakrishnan, A. Rawson and C. K. Sunil, Handbook of Millets- Processing, Quality, and Nutrition Status, Springer Singapore, Singapore, 1st edn, 2022 Search PubMed.
- M. Mahajan, P. Singla and S. Sharma, Sustainable postharvest processing methods for millets: A review on its value-added products, J. Food Process Eng., 2024, 47(1), e14313 CrossRef.
- M. Karaś, A. Jakubczyk, U. Szymanowska, K. Jęderka, S. Lewicki and U. Złotek, Different temperature treatments of millet grains affect the biological activity of protein hydrolyzates and peptide fractions, Nutrients, 2019, 11(3), 550 CrossRef.
- T. J. Joshi, S. M. Singh and P. S. Rao, Novel thermal and non-thermal millet processing technologies: advances and research trends, Eur. Food Res. Technol., 2023, 249, 1149–1160 CrossRef CAS.
- S. R. Kumar, N. Tangsrianugul and M. Suphantharika, A Review on Isolation, Characterization, Modification, and Applications of Proso Millet Starch, Foods, 2023, 12, 1–16 CAS.
-
B. Khadhraoui, A. S. Fabiano-Tixier, P. Robinet, R. Imbert and F. Chemat, in Green Food Processing Techniques: Preservation, Transformation and Extraction, Elsevier, 2019, pp. 23–56 Search PubMed.
- O. P. Stebeleva and A. V. Minakov, Application of Cavitation in Oil Processing: An Overview of Mechanisms and Results of Treatment, ACS Omega, 2021, 6, 31411–31420 CrossRef CAS PubMed.
-
N. Pallarés, H. Berrada, E. Ferrer, J. Zhou, M. Wang, F. J. Barba and M. Brnčić, Effect of high hydrostatic pressure (HPP) and pulsed electric field (PEF) technologies on reduction of aflatoxins in fruit juices, in Sustainable Production Technology in Food, Elsevier, 2021, pp. 155–164 Search PubMed.
- A. C. Sullivan, P. Pangloli and V. P. Dia, Impact of ultrasonication on the physicochemical properties of sorghum kafirin and in vitro pepsin-pancreatin digestibility of sorghum gluten-like flour, Food Chem., 2018, 240, 1121–1130 CrossRef CAS PubMed.
- S. Hassan, M. Imran, M. H. Ahmad, M. I. Khan, C. XU, M. K. Khan and N. Muhammad, Phytochemical characterization of ultrasound-processed sorghum sprouts for the use in functional foods, Int. J. Food Prop., 2020, 23, 853–863 CrossRef CAS.
- A. Hu, Y. Li and J. Zheng, Dual-frequency ultrasonic effect on the structure and properties of starch with different size, LWT, 2019, 106, 254–262 CrossRef CAS.
- S. R. Ramakrishnan, U. Antony and S. J. Kim, Non-thermal process technologies: Influences on nutritional and storage characteristics of millets, J. Food Process Eng., 2023, 46 Search PubMed.
-
M. Tsevdou, E. Gogou and P. Taoukis, High hydrostatic pressure processing of foods, in Green Food Processing Techniques: Preservation, Transformation and Extraction, Elsevier, 2019, pp. 87–137 Search PubMed.
- M. K. Jwa, S. B. Lim, C. K. Mok and Y. S. Park, Inactivation of Microorganisms and Enzymes in Foxtail Millet Takju by High Hydrostatic Pressure Treatment, Korean J. Food Sci. Technol., 2001, 33, 226–230 Search PubMed.
- N. Sharma, S. K. Goyal, T. Alam, S. Fatma, A. Chaoruangrit and K. Niranjan, Effect of high pressure soaking on water absorption, gelatinization, and biochemical properties of germinated and non-germinated foxtail millet grains, J. Cereal Sci., 2018, 83, 162–170 CrossRef CAS.
- P. Gulati, L. Sabillón and D. J. Rose, Effects of processing method and solute interactions on pepsin digestibility of cooked proso millet flour, Food Res. Int., 2018, 109, 583–588 CrossRef CAS.
- L. R, S. P. S and M. R, Improvement in Millet Soaking by Way of Bubbled Cold Plasma Processed Air Exposure; Phytic Acid Reduction Cum Nutrient Analysis Concern, Front. Adv. Mater. Res., 2021, 1–16 Search PubMed.
-
N. N. Misra and M. S. Roopesh, Cold plasma for sustainable food production and processing, in Green Food Processing Techniques: Preservation, Transformation and Extraction, Elsevier, 2019, pp. 431–453 Search PubMed.
- F. L. Pathan, R. R. Deshmukh and U. S. Annapure, Soaking plasma processed chickpea (Cicer arientinum) cultivars, Legume Sci., 2021, 3, 1–6 Search PubMed.
- N. N. Misra, B. Yadav, M. S. Roopesh and C. Jo, Cold Plasma for Effective Fungal and Mycotoxin Control in Foods: Mechanisms, Inactivation Effects, and Applications, Compr. Rev. Food Sci. Food Saf., 2019, 18, 106–120 CrossRef CAS PubMed.
-
D. A. Popović, D. D. Milinčić, M. B. Pešić, A. M. Kalušević, Ž. L. Tešić and V. A. Nedović, Encapsulation technologies for polyphenol-loaded microparticles in food industry, in Green Food Processing Techniques: Preservation, Transformation and Extraction, Elsevier, 2019, pp. 335–367 Search PubMed.
- A. M. Pathare, R. S. Singhal, B. D. Rao and J. S. Gokhale, Extrusion inhibits lipolytic enzymes in pearl millet (Pennisetum glaucum) flour, Innovative Food Sci. Emerging Technol., 2024, 93, 103605 CrossRef CAS.
- T. Vinutha, D. Kumar, N. Bansal, V. Krishnan, S. Goswami, R. R. Kumar, A. Kundu, V. Poondia, S. G. Rudra, V. Muthusamy, G. Rama Prashat, P. Venkatesh, S. Kumari, P. Jaiswal, A. Singh, A. Sachdev, S. P. Singh, T. Satyavathi, S. V. Ramesh and S. Praveen, Thermal treatments reduce rancidity and modulate structural and digestive properties of starch in pearl millet flour, Int. J. Biol. Macromol., 2022, 195, 207–216 CrossRef CAS PubMed.
- S. Goswami, V. T, R. R. Kumar, T. Singh, A. Ali, M. C. Meena, S. P. Singh, K. Dulait and C. T. Satyavathi, Insights into Recent Techniques for Improving Shelf Life and Value Addition in Pearl Millet Flour: A Mini Review on Recent Advances, Ann. Arid Zone, 2023, 62, 103–108 CrossRef.
- V. G. Balasubramaniam, P. Ayyappan, S. Sathvika and U. Antony, Effect of enzyme pretreatment in the ultrasound assisted extraction of finger millet polyphenols, J. Food Sci. Technol., 2019, 56, 1583–1594 CrossRef CAS PubMed.
- N. C. Mustac, B. Voucko, D. Novotni, S. Drakula, A. Gudelj, F. Dujmic and D. Curic, Optimization of high intensity ultrasound treatment of proso millet bran to improve physical and nutritional quality, Food Technol. Biotechnol., 2019, 57, 183–190 CrossRef CAS.
- B. R. de C. Leite Júnior, A. A. L. Tribst and M. Cristianini, Effect of High-Pressure Technologies on Enzymes Applied in Food Processing, Enzyme Inhib. Act., 2017, 49–72 Search PubMed.
- H. Liu, H. Fan, R. Cao, C. Blanchard and M. Wang, Physicochemical properties and in vitro digestibility of sorghum starch altered by high hydrostatic pressure, Int. J. Biol. Macromol., 2016, 92, 753–760 CrossRef CAS PubMed.
- Z. Afrose Subaitha, S. R. Priyadarshini, K. S. Yoha and J. A. Moses, Impact of post-harvest processing techniques on the glycemic index of millets, Food Chem. Adv., 2024, 4, 100636 CrossRef.
-
S. Deshpande, M. K. Tripathi, D. Mohapatra and R. S. Jadam, Product Development from Millets, Millets and Millet Technology, 2021, pp. 143–160 Search PubMed.
- S. Srivastava and A. Bisht, Millet-Based Value-Added Food Products for Diabetics, Millets and Millet Technology, 2021, 321–331 Search PubMed.
-
V. R. Chamarthy and V. A. Tonapi, Millet-Based Traditional Processed Food Beverages, Millets and Millet Technology, 2021, pp. 305–320 Search PubMed.
- S. Saleem, N. U. Mushtaq, W. H. Shah, A. Rasool, K. R. Hakeem, C. S. Seth, I. Tahir and R. U. Rehman, Millets as smart future food with essential phytonutrients for promoting health, J. Food Compos. Anal., 2023, 124, 105669 CrossRef CAS.
- A. Gull, R. Jan, A. Nayik, K. Prasad and P. Kumar, Significance of Finger Millet in Nutrition, Health andValue added Products: A Review, J. Environ. Sci., 2014, 3, 1601–1608 Search PubMed.
- A. S. M. Saleh, Q. Zhang, J. Chen and Q. Shen, Millet grains: Nutritional quality, processing, and potential health benefits, Compr. Rev. Food Sci. Food Saf., 2013, 12, 281–295 CrossRef CAS.
- M. Selladurai, M. K. Pulivarthi, A. S. Raj, M. Iftikhar, P. V. V. Prasad and K. Siliveru, Considerations for gluten free foods - pearl and finger millet processing and market demand, Grain Oil Sci. Technol., 2023, 6, 59–70 CrossRef CAS.
- R. V Jaybhaye, I. L. Pardeshi, P. C. Vengaiah and P. P. Srivastav, Processing and Technology for Millet Based Food Products: A Review, J. Ready Eat Food, 2014, 1(2), 32–48 Search PubMed.
- A. Kumar, A. Kaur, K. Gupta, Y. Gat and V. Kumar, Assessment of germination time of finger millet for value addition in functional foods, Curr. Sci., 2021, 120, 406–413 CrossRef CAS.
- S. Rai, A. Kaur and B. Singh, Quality characteristics of gluten free cookies prepared from different flour combinations, J. Food Sci. Technol., 2014, 51, 785–789 CrossRef CAS PubMed.
- V. Verma and S. Patel, Value added products from nutri-cereals: Finger millet (Eleusine coracana), Emir. J. Food Agric., 2013, 25, 169–176 CrossRef.
- J. M. Harper, Food extrusion, CRC Crit. Rev. Food Sci. Nutr., 1979, 11, 155–215 CrossRef PubMed.
- C. G. Dalbhagat, D. K. Mahato and H. N. Mishra, Effect of extrusion processing on physicochemical, functional and nutritional characteristics of rice and rice-based products: A review, Trends Food Sci. Technol., 2019, 85, 226–240 CrossRef CAS.
- R. Geetha, H. N. Mishra and P. P. Srivastav, Twin screw extrusion of kodo millet-chickpea blend: process parameter optimization, physico-chemical and functional properties, J. Food Sci. Technol., 2014, 51, 3144–3153 CrossRef CAS PubMed.
-
M. A. Alarcón-García, J. A. Perez-Alvarez, J. H. López-Vargas and M. J. Pagán-Moreno, Meat Snacks Consumption: Aspects that the Consumer Looks for to Consider Them a Healthy Food, 2021, p. 82 Search PubMed.
- P. Krishnaraj, T. Anukiruthika, P. Choudhary, J. A. Moses and C. Anandharamakrishnan, 3D Extrusion Printing and Post-Processing of Fibre-Rich Snack from Indigenous Composite Flour, Food Bioprocess Technol., 2019, 12, 1776–1786 CrossRef CAS.
- J. Sanuujabertini, M. Balakrishnan, S. Parveen, R. Ravikumar and A. P. M. Kumar, Studies on Rheological Properties of 3D Printable Millet Based Dough Studies on Rheological Properties of 3D Printable Millet Based Dough, Biol. Forum – Int. J., 2023, 15(7), 10–14 CAS.
-
S. Ghimire, 3D Printing of Finger Millet Incorporated Meat Analogues Using Fava Bean, Pea and Mung Bean Protein Based Ink, Asian Institute of Technology, 2023 Search PubMed.
- P. M. Becker and P. Yu, What makes protein indigestible from tissue-related, cellular, and molecular aspects?, Mol. Nutr. Food Res., 2013, 57, 1695–1707 CrossRef CAS PubMed.
- L. Yousaf, D. Hou, H. Liaqat and Q. Shen, Millet: A review of its nutritional and functional changes during processing, Food Res. Int., 2021, 142, 110197 CrossRef CAS PubMed.
- A. Sarkar, T. Niranjan, G. Patel, A. Kheto, B. K. Tiwari and M. Dwivedi, Impact of cold plasma treatment on nutritional, antinutritional, functional, thermal, rheological, and structural properties of pearl millet flour, J. Food Process Eng., 2023, 46, 1–16 Search PubMed.
- M. Albarracín, R. J. González and S. R. Drago, Effect of soaking process on nutrient bio-accessibility and phytic acid content of brown rice cultivar, Lebensm.-Wiss. Technol., 2013, 53, 76–80 CrossRef.
- N. Jha, R. Krishnan and M. S. Meera, Effect of different soaking conditions on inhibitory factors and bioaccessibility of iron and zinc in pearl millet, J. Cereal Sci., 2015, 66, 46–52 CrossRef CAS.
- G. Bhuvaneshwari, A. Nirmalakumari and S. Kalaiselvi, Impact of soaking, sprouting on antioxidant and anti-nutritional factors in milletgrains, J. Phytol., 2020, 12, 62–66 CrossRef CAS.
- S. Bhinder, S. Kumari, B. Singh, A. Kaur and N. Singh, Impact of germination on phenolic composition, antioxidant properties, antinutritional factors, mineral content and Maillard reaction products of malted quinoa flour, Food Chem., 2021, 346, 128915 CrossRef CAS PubMed.
- K. Kumari, P. Kashyap and P. Chakrabarti, Germination and probiotic fermentation: a way to enhance nutritional and biochemical properties of cereals and millets, Food Sci. Biotechnol., 2023, 33, 505–518 CrossRef PubMed.
- P. Prasad and J. K. Sahu, Effect of soaking and germination on grain matrix and glycaemic potential: A comparative study on white quinoa, proso and foxtail millet flours, Food Biosci., 2023, 56, 103105 CrossRef CAS.
- S. O. Azeez, C. E. Chinma, S. O. Bassey, U. R. Eze, A. F. Makinde, A. A. Sakariyah, S. S. Okubanjo, N. Danbaba and O. A. Adebo, Impact of germination alone or in combination with solid-state fermentation on the physicochemical, antioxidant, in vitro digestibility, functional and thermal properties of brown finger millet flours, Lebensm.-Wiss. Technol., 2022, 154, 112734 CrossRef CAS.
- G. A. Annor, C. Tyl, M. Marcone, S. Ragaee and A. Marti, Why do millets have slower starch and protein digestibility than other cereals?, Trends Food Sci. Technol., 2017, 66, 73–83 CrossRef CAS.
- V. D. Pawar and G. M. Machewad, Processing of foxtail millet for improved nutrient availability, J. Food Process. Preserv., 2006, 3, 269–279 CrossRef.
- S. Roopa and K. S. Premavalli, Effect of processing on starch fractions in different varieties of finger millet, Food Chem., 2008, 106, 875–882 CrossRef CAS.
- K. G. Duodu, J. R. N. Taylor, P. S. Belton and B. R. Hamaker, Factors affecting sorghum protein digestibility, J. Cereal Sci., 2003, 38, 117–131 CrossRef CAS.
- S. O. Azeez, C. E. Chinma, S. O. Bassey, U. R. Eze, A. F. Makinde, A. A. Sakariyah, S. S. Okubanjo, N. Danbaba and O. A. Adebo, Impact of germination alone or in combination with solid-state fermentation on the physicochemical, antioxidant, in vitro digestibility, functional and thermal properties of brown finger millet flours, Lebensm.-Wiss. Technol., 2022, 154, 112734 CrossRef CAS.
- S. P. Bangar, S. Suri, S. Malakar, N. Sharma and W. S. Whiteside, Influence of processing techniques on the protein quality of major and minor millet crops: A review, J. Food Process. Preserv., 2022, 1–23 Search PubMed.
- S. Jan, K. Kumar, A. N. Yadav, N. Ahmed, P. Thakur, D. Chauhan, Q. U. E. H. Rizvi and H. S. Dhaliwal, Effect of diverse fermentation treatments on nutritional composition, bioactive components, and anti-nutritional factors of finger millet (Eleusine coracana L.), J. Appl. Biol. Biotechnol., 2022, 10, 46–52 CrossRef CAS.
- S. Sharma, D. C. Saxena and C. S. Riar, Analysing the effect of germination on phenolics, dietary fibres, minerals and γ-amino butyric acid contents of barnyard millet (Echinochloa frumentaceae), Food Biosci., 2016, 13, 60–68 CrossRef CAS.
|
This journal is © The Royal Society of Chemistry 2024 |
Click here to see how this site uses Cookies. View our privacy policy here.