Extractability of oleuropein, hydroxytyrosol, tyrosol, verbascoside and flavonoid-derivatives from olive leaves using ohmic heating (a green process for value addition)
Received
23rd December 2023
, Accepted 3rd February 2024
First published on 26th February 2024
Abstract
The aim of this study was to determine the influence of ohmic heating (OH) as a green processing approach on the extraction of principal polar phenols from olive leaves, with special attention on oleuropein content. The concentrations were quantified using ultra-high-performance liquid chromatography (UHPLC). Other target analytes including total flavonoid content (TFC) and total condensed tannins (TCT) were also assessed as secondary response variables. The key predictor variables were (i) extraction method: ohmic heating (OH) and conventional heating (conven), (ii) solvent ratio (40–80% aqueous ethanol), and (iii) extraction temperature: 45–75 °C. In addition, control samples were prepared through solvent extraction at room temperature using the same solvent ratios applied for OH and conven. The length of processing time (15 min) was the same for all extraction methods. Overall, the data showed that intensified extractability of major polyphenols from olive leaves is readily achievable by using ohmic heating rather than the other aforementioned methods (p < 0.05). Importantly, the recovery of oleuropein through the ohmic system, that reached up to 26.18 mg per g extract at 75 °C with 80% ethanol was significantly higher than other examined approaches (7.98–14.55 mg per g in conven groups, and 8.64–10.81 mg per g in control groups). Other major polyphenols that reached the maximum levels via the ohmic approach, were as follows: (i) luteolin 7-O-glucoside (4.12 mg per g extract), apigenin 7-O-glucoside (3.47 mg per g extract), rutin (3.78 mg per g extract), and tyrosol (0.34 mg per g extract) using 60% ethanol at 55 °C, (ii) verbascoside (1.04 mg per g extract) using 80% ethanol at 75 °C, and (iii) hydroxytyrosol (1.38 mg per g extract) using 80% ethanol at 55 °C. The findings of this study demonstratethat ohmic heating is potentially a method of preference for efficient recovery of representative phenols of olive leaves.
Sustainability spotlight
This investigation showed that the choice of the extraction method is of great importance as the use of ohmic heating (an emerging mechanical technique), compared to the conventional heating, significantly intensified the recovery of target polar phenols of olive leaves particularly oleuropein, hydroxytyrosol, and verbascoside which are among the highly valued natural antioxidants. The data from this investigation highlight that ohmic heating (with fast/uniform heat transfer, energy-saving, and cost-effective advantages) has great potential for optimal valorization of olive leaf biomass. With reference to the United Nations Sustainable Development Goals, the results presented in this study correspond to the following goals. Goal 2 – “End hunger, achieve food security and improved nutrition and promote sustainable agriculture”. Goal 12 – “Ensure sustainable consumption and production patterns”.
|
Introduction
Olive mill leaves (the biomass residues of the olive oil industry) contain significant levels of polyphenols, some of which are prized for their distinctive antioxidant properties. These include oleuropein (a secoiridoid glycoside, exclusively intrinsic to Oleaceae plants), verbascoside, hydroxytyrosol, and flavonoids.1 In this regard, sustainable recovery of such valuable compounds from olive leaf residues (presently underexploited) may find high-value applications in food and pharmaceutical products; which in part meets the current market requirements (the growing demands for bio-based and health-promoting food ingredients). In food applications, the leaf extracts rich in the abovementioned polyphenols have great potential to be incorporated into a diverse range of food products to (i) enhance their oxidative stability and shelf-life and (ii) promote their nutritional and health benefits. In particular, oleuropein-rich extracts have additional benefits as, beyond having potential for food formulation, they can act as natural substrates for hydroxytyrosol formation (upon successful hydrolysis from which a phenolic alcohol such as hydroxytyrosol can be generated). Development of this application can be of value for the industry to produce natural hydroxytyrosol in place of the costly synthetic ones.
At present, the existing extraction system is commonly based on conventional/classic modes which represent weak efficiency (high consumption of fossil fuel energy/solvents for a prolonged extraction time). This has prompted scientists to investigate innovative mechanical approaches to address the downsides inherent in the conventional/current system. In effect, numerous emerging techniques have been researched through a broad range of operating conditions/processing designs. Typical examples are microwave-assisted extraction, ultrasound-assisted extraction (UAE),2 supercritical fluid extraction (SFE),3 high-voltage electric discharges (HVED),4 and pulsed electric field (PEF).5
Another green solution is making use of ohmic heating (OH) which is primarily based on an inside-out thermal flow system, enabling an instantly uniform heat supply within the food with minimum energy use. This particular method was indeed studied in our previous research6 for the extraction of olive leaves, through a series of temperatures (45, 55, and 75 °C) and solvent ratios (using aqueous ethanol). As a result, the ohmic system, compared to the conventional approach, enabled significantly higher (i) extraction yield (up to 34.53%) at 75 °C with 80% ethanol, (ii) total phenolic content (TPC) up to 42.53 mg GAE per g extract at 55 °C with 60% ethanol, and (iii) antiradical capacity at 75 °C with 80% ethanol (1.21 mM TE per g by DPPH and 0.62 mM TE per g extract by ABTS). Above all, the data on the total phenols across the extraction methods showed relatively similar trends of changes to those observed in radical scavenging activities, which can in part annotate the roles of polyphenol concentrations in dictating antioxidant activities of the resulting extracts.
However, limitations exist in our previous work as it was a preliminary study. For example, the experiment was limitted to the evaluation of total polyphenols, and the assays were based on spectrophotometric methods. Hence, there needs to be further investigation, particularly, on the quantities of the individual/principal polyphenols of the extracts via instrumental analyses such as high-performance liquid chromatography (HPLC). This is especially important due to the fact that there are variations in phenolic structures and biochemical routes, and thus the performance of each phenolic group may be markedly different from other phenolic group(s) under various processing conditions. Moreover, taking a cue from the information stated above, data are particularly needed on the extractability of oleuropein from leaves. In this line, to further evaluate the extraction efficacy of ohmic heating, the present study extended the previous research to examine the olive leaf extracts for the content of the chief polyphenols comprising oleuropein, hydroxytyrosol, verbascoside, tyrosol, and flavonoid-derivatives (luteolin 7-O-glucoside, apigenin 7-O-glucoside, and rutin). In addition, the concentrations of total flavonoids and total condensed tannins were assessed as the secondary outcome variables. In terms of tannins which are among the most notable active compounds in olive leaves, their recoveries have shown complications in different studies and reportedly, the extraction method (operational input/parameters) and solvent nature/ratio substantially affect their diffusions from the cell tissues.7–10 Therefore, their quantitative variations were examined in this study to provide further insights into the extraction ability of ohmic heating.
Materials and methods
Plant materials and chemicals
Olive mill leaves, from the same cultivar and growing site as those used in our earlier work,6 were kindly provided by the “Center for Advanced Studies in Energy and Environment”, University of Jaén, Campus of Las Lagunillas, Jaén, Spain. All chemicals used in this study were identical to those used in our earlier work.6 Additionally the following chemicals (Sigma-Aldrich, Saint Louis, MO, USA) were used for the current study: (i) analytical-grade chemicals – aluminum chloride, sodium nitrite, 2,4,6-tris(2-pyridyl)-s-triazine (TPTZ), sodium hydroxide, methanol, quercetin, vanillin, and catechin, (ii) HPLC-grade standards/reagents – oleuropein, hydroxytyrosol, verbascoside, tyrosol, luteolin 7-O-glucoside, apigenin 7-O-glucoside, rutin, formic acid, and acetonitrile (≥99.9%).
Study design
Prior to samples being extracted, olive leaves were pre-processed following precisely the same procedure as that used previously.6 Briefly, the leaves upon arrival were cleaned, and dried at 37 °C for 48 h. The dried leaves were ground to 0.3 mm, vacuum packed in polypropylene bags and refrigerated (0–4 °C) for a maximum of two weeks prior to extraction experiments. The extraction of dry ground olive leaves was conducted (solid-solvent ratio of 1
:
10) via the following techniques. The extraction time (15 min) was the same for all three extraction methods.
Ohmic heating.
Ohmic extraction was performed using a bench-scale ohmic heater following the same procedure previously reported.6 Prior to the extraction, the electrical conductivity of the extraction solvents was intensified, using sodium chloride, to obtain 4.2 mS cm−1, 3.1 mS cm−1, and 3.4 mS cm−1 for 80%, 60%, and 40% aqueous ethanol, respectively. The frequency and electrical voltage range remained constant, 25 kHz and 1–10 V cm−1, respectively. The main extraction parameters were the (i) solvent ratio (in the range of 40–80% ethanol–water v/v), and (ii) extraction temperature (in the range of 45–75 °C).
Conventional heating.
Conventional (conven) heating extraction of dry ground leaves was performed following the same process design used earlier.6 The extraction process was under the same solvent ratio/temperature conditions using the same operation components as those used for the ohmic system, but in the absence of electrodes in the food chamber.
Solvent extraction (control).
As the control samples, the dry leaves were extracted through constant agitation at room temperature (without heating) using the same aforementioned solvent ratios applied in the OH and conven methods.
Colorimetric analyses – determination of the recovery of total flavonoids and tannins from olive leaf extracts
Total flavonoid content (TFC).
Total flavonoid content was measured using aluminum trichloride assay as described by Qawasmeh et al.11 In a 5 mL volumetric flask, 500 μL of extract was mixed with 150 μL of 5% sodium nitrite solution and incubated at room temperature for 5 min prior to adding 150 μL of 10% aluminum trichloride solution. The solution was vortex mixed and allowed to stand for 1 min. After adding 1 mL of 1 M sodium hydroxide, the mixture was made up to a final volume of 5 mL with distilled water and vortex mixed for 1 min. The absorbance readings were measured at 510 nm and the concentrations of total flavonoids were calculated against the quercetin standard curve. The results were expressed as mg of quercetin equivalents per gram of extract (mg QE per g extract).
Total condensed tannins (TCT).
The content of total condensed tannins in olive leaf extracts was quantified using the method of Julkunen-Tiitto12 with minor modifications. Briefly, an aliquot (50 μL) of the extract or standard solution was added to 1 mL of reagent solution (containing 4% vanillin and 8% hydrochloric acid mixed with methanol, 1
:
1 v/v). After 20-min incubation at room temperature, the absorbance readings were measured at 500 nm. Total tannins were calculated against the standard curve, and the results were expressed as mg catechin equivalents per gram of extract (mg CE per g extract).
HPLC analyses – determination of the recovery of principal polyphenols from olive leaf extracts
The target/individual polyphenols were separated using an ultra-high-performance liquid chromatograph (UHPLC) (Shimadzu Nexera X2 UHPLC) connected to a diode array detector (Shimadzu SPD-M20A), and an integration system (Shimadzu LabSolutions software, Kyoto, Japan). A reverse-phase Aquity UPLC BEH C-18 column (100 mm × 2.1 mm, with 1.7 μm particle size, from Waters Corporation) was applied for the separation of analytes within the gradient elution program detailed previously.13,14 The temperature remained constant at 45 °C. Eluent A (water/formic acid, 99.9/0.1 v/v) and eluent B (acetonitrile) were used as mobile phases at a flow rate of 0.3 mL min−1. The injection volume was 5 μL, and the chromatograms were obtained at 280 nm.
Statistical analysis
The significant differences (p < 0.05) between the mean values (±SD) of all determinations were statistically assessed via analysis of variance (ANOVA) by means of SPSS software (version 27.0). The dependent variables of the extracts were individually analyzed using two-way ANOVA to assess the interactive effects of the factors (independent variables) on the mean values of each dependent (response) variable individually.
Results and discussion
Total flavonoids (TFC) and total condensed tannins (TCT)
Regardless of the extraction technique, between groups for the same extraction method, the use of 60% ethanol led to the highest extractability of total flavonoids (Fig. 1). However, the values varied largely between OH and conven/control groups. For the same solvent ratio at the same extraction temperature, the OH extracts significantly improved the recoveries compared to other extraction methods. Indeed, the OH extract with 60% ethanol at 55 °C enabled the maximum yield of TFC which reached 20.45 mg QE per g extract.
 |
| Fig. 1 Effect of extraction methods on the recovery of (a) total flavonoid content TFC (mg QE per g leaf extract) and (b) total condensed tannins (mg CE per g leaf extract). Extraction methods: ohmic heating (OH), conventional heating (conven), and solvent extraction without heating (control). The results are presented as mean values with standard deviation error bars. | |
Flavonoids constitute a significant proportion of polyphenols in olive leaves, predominately in the glycosidic form rather than in the aglycon (free) form. The variations in flavonoid content reported in different studies confirm that the extractability of bio-phenols is heavily affected by the parameters involved in the processing systems.
In the study of Lee et al.,15 through 80% ethanol solvent extraction, the total flavonoids yielded 58 mg naringin equivalents per g extract. Ghelichkhani et al.,16 detected 396.4 mg QE per g and 298.16 mg QE per g in spray dried and freeze dried olive leaf extracts, respectively. In their research, Abaza et al.,17 observed the highest recovery of flavonoids from olive leaves extracted using 50% acetone through an ultrasound-assisted system for 10 min at 60 °C. Agatonovic-Kustrin et al.,18 described the effectiveness of fermentation extraction with lactic acid-forming bacteria using ethyl acetate in the improved yield of flavonoids that reached up to 238 μg rutin equivalents/20 μL of olive leaf extract. Also, in the study of Lins et al.,19 the commercial micronized dry olive leaf powders (superfine-ground) when extracted with 80% methanol produced 19.4 mg quercetin equivalents per g olive leaves.
The mean values of the total condensed tannins (TCT) obtained by different extraction methods are shown in Fig. 1(b). Similar to those observed for TFC, the OH extract with 60% ethanol at 55 °C produced the highest recovery of TCT (10.92 mg CE per g extract).
Tannins, complex polymeric groups of phenolics, possess a range of bio-functional properties, and are known to have antioxidant, antimicrobial, and anticarcinogenic activities. In the literature, the amount of total condensed tannins (the catechin-class tannins) in olive leaves is variable. Examples are 8.30 g per kg leaves (d.w.), 6.24–9.92 g per kg leaves (d.w.), 0.46 g per 100 g leaves (d.w.), and 1.07 g per 100 g leaves (fresh weight).20–23 In their research, Mansour-Gueddes et al.24 using maceration at room temperature for two days, reported variations in condensed tannins of the aqueous extracts of olive leaves from three growing sites in Tunisia (0.24, 0.74, and 0.84 mg CE per g leaves of Northern, Central, and Southern areas, respectively). In a recent paper, Guebebia et al.25 highlighted the significant effects of genotypes and the extraction approach as reportedly, the leaves (Chemlali variety), processed by maceration, yielded a greater amount of condensed tannins (23.03 mg CE/100 g d.w.) compared to the leaves (Zarrazi variety) extracted by using an ultrasonic assisted system (8.32 mg CE/100 g d.w.).
Beyond the key factors such as cultivars, climate, leaf life-time, and handling/storage conditions, other factors including operations/parameters of pre-processing can also be influential in the recovery of condensed tannins. Molina-Alcaide & Yáñez-Ruiz21 through their research on comparing various drying methods/temperatures found 10.0, 9.92, 7.90, 6.24, and 9.57 g kg−1 total condensed tannins in fresh, freeze dried, air-dried, and forced convection oven dried (60 and 100 °C) samples, respectively.
Recovery of principal polar phenols from olive leaves – chromatographic analysis
The concentrations of the target polyphenols in olive leaf extracts (mg per g extract) were determined through chromatographic analysis.
Oleuropein content.
Compared to the conven and control extracts, the OH groups contained significantly higher content of oleuropein at the same extraction temperature/solvent ratio (p < 0.05) (Fig. 2). The maximum level of oleuropein (26.18 mg oleuropein per g extract) belonged to the OH extracts at 75 °C with 80% ethanol. In our previous study, this particular approach (OH 75 °C, 80% ethanol) also produced maximum values of radical scavenging activities detected by DPPH and ABTS. Given the fact that antiradical activities in the plant matrices are heavily influenced by the content of certain bio-phenols, and the fact that oleuropein has substantial antioxidant potential, the data here may suggest that oleuropein in part acted, dose-dependently, as a radical scavenger for each extract.
 |
| Fig. 2 Effect of extraction methods on the recovery of oleuropein from olive leaves (mg per g leaf extract). Extraction methods: ohmic heating (OH), conventional heating (conven), and solvent extraction without heating (control). The results are presented as mean values with standard deviation error bars. | |
The data on oleuropein content of olive leaves differ noticeably among studies depending on growing sites, seasonal/collection times, and processing strategies. Having said that, despite the lack of consistency of the results, the majority of studies described oleuropein to be among the chief phenolic secoiridoids present in olive leaves. The research performed by Contreras et al.,26 (using the same variety and growing region as those used in the present study) demonstrated that oleuropein levels varied largely in response to three sequent extraction steps, from which those extracted with UAE prior to alkaline extraction presented the highest values (12
694 and 1790 mg per 100 g extracts from tree-picked olive leaves and olive mill leaves, respectively). Şahin et al.,27 reported that Arbequina olive leaves cultivated in various regions in Texas (sampled in February) presented different proportions, among which those grown in Santa Fe yielded the highest amount of oleuropein (71.53 μg per mg fresh leaves).
In another study,28 steam-blanched olive leaves following an optimized multiple extraction system (85 °C for 30 min) contained significantly greater proportions of oleuropein (103.1 mg per g olive leaves d.w.) when compared to those extracted by the conventional method (4.6 mg g−1, at 40 °C for 48 h). Xie et al.,29 observed an increased amount of oleuropein when the leaves (Frantoio cultivar) were subjected to an ultrasound-assisted system combined with reduced pressure extraction that enabled 92.3% efficiency through a single attempt of the extraction. Irakli et al.,30 reported improved concentration of oleuropein (10.65%) in tree-picked olive leaves (north Greece) having been extracted by UAE optimized with 50% acetone at 60 °C for 10 min.
In a recent paper, examining olive mill leaves (Arbequina variety), Márquez et al.,31 found substantial effectiveness of the homogenizer-assisted extraction approach in the recovery of oleuropein (4345 mg/100 g). Moreover, Ahmad-Qasem et al.,32 compared different drying and freezing methods used before the extraction process and detected the highest recovery of oleuropein (108.6 mg per g extract, d.w.) when the leaves, prior to being extracted in a shaking water bath (22 °C for 24 h), were initially hot air dried at 120 °C for 12 min.
Hydroxytyrosol content.
Hydroxytyrosol, a phenolic alcohol in olive leaves, is typically produced from the ring cleavage of oleuropein in the course of acidic or enzymatic (endogenous and exogenous) hydrolysis. This compound is valued as being one of the many powerful antioxidants present in plant species. The production of its synthetic counterpart is generally costly and/or intricate. In effect, researchers have resorted to the investigation of efficient solutions to isolate natural hydroxytyrosol from plant-based materials. Among them, olive leaves can be of interest, provided that they are rich in oleuropein compounds and not being oxidized under severe processing conditions. If that is the case, through various bioconversion strategies, hydroxytyrosol may be freed during the decomposition of oleuropein.
The mean values of hydroxytyrosol obtained with different extraction approaches are highlighted in Fig. 3. Examining the OH groups here, it is clear that the use of ohmic heating with 80% ethanol (for the same extraction temperature) shows great potential for the diffusion of hydroxytyrosol from the cell walls, in particular, at 55 °C which produced the highest extraction (1.38 mg per g extract d.w.). On the other hand, comparably, the use of 40% ethanol in OH groups enabled the least amount of recovery; particularly at 45 °C (0.77 mg per g extract). Furthermore, no significant difference was found between conven 45 °C and conven 55 °C for the same solvent ratio (p > 0.05).
 |
| Fig. 3 Effect of extraction methods on the recovery of hydroxytyrosol from olive leaves (mg per g leaf extract). Extraction methods: ohmic heating (OH), conventional heating (conven), and solvent extraction without heating (control). The results are presented as mean values with standard deviation error bars. | |
In previous studies, the results of hydroxytyrosol concentrations tend to vary largely among different experiments through various approaches/conditions. In the research of Ghomari et al.,33 using 80% ethanol and distilled water, the concentrations of hydroxytyrosol were 15.17 and 27.20 mg g−1 (through sonication), and 0.02 and 0.25 mg g−1 (through a single-run maceration). Ortega-García & Peragón34 through their investigation on the effect of Spanish cultivars (including Picual), detected that there was a correlation between catalytic activities of regulatory enzymes [phenylalanine ammonia lyase (PAL) and polyphenol oxidase (PPO)] and endogenous phenolic content as it was found that each cultivar responded differently. Among them, the Picual cultivar exhibited low hydroxytyrosol content in response to the low activity of PAL and high activity of PPO. In another research study conducted by Orak et al.,35 using different genotypes of olive leaves from Izmir, Turkey, the hydro-methanolic extracts (65 °C, 15 min) exhibited variations in the range of 1.33–4.03 mg g−1 with “Uslu” and “Esek Zeytini” cultivars representing the lowest and the highest yields, respectively. Caballero et al.,36 using supercritical fluid extraction of olive leaves, observed the highest recovery of hydroxytyrosol (1.35 mg per g extract) with the pressure at 300 bar. Furthermore, a recent study37 demonstrated the significant effectiveness of ultrasound-assisted extraction using natural deep eutectic solvent (citric acid/glycine/water) in the extractability of hydroxytyrosol (87 ppm); this was over a four-fold increase compared to that provided by a conventional method with water.
Tyrosol content.
As illustrated in Fig. 4, the maximum content of tyrosol observed in this study belonged to the OH 75 °C with 80% ethanol (0.39 mg per g extract). Tyrosol is a phenylethanoid (a phenolic alcohol) with relatively the same molecular structure as that of hydroxytyrosol but with one less hydroxyl group. Although present at a low concentration in olive leaves, tyrosol is known to have substantially great stability against auto-oxidation (its antioxidant potential remains stable longer than that of other major phenolic compounds).38
 |
| Fig. 4 Effect of extraction methods on the recovery of tyrosol from olive leaves (mg per g leaf extract). Extraction methods: ohmic heating (OH), conventional heating (conven), and solvent extraction without heating (control). The results are presented as mean values with standard deviation error bars. | |
The diffusivity of tyrosol from olive leaf tissues is partially reliant on certain conditions of the extraction system. A recent report by Akli et al.,39 proposed an optimized extraction protocol using deep eutectic solvents mixed with different amino acids including lysine which represented 53
420.23 μg per g tyrosol in the extracts, that was significantly higher than that obtained by using a conventional solvent with 70% ethanol (19
398.64 μg g−1). These authors observed that the content of tyrosol was greater than that of hydroxytyrosol and oleuropein (this is typically different from what can be expected) and explained that the probable reasons can be the high stability of tyrosol and solvent suitability for tyrosol recovery.
In their research Ghomari et al.,33 through a single run maceration, found that distilled water yielded tyrosol of around 0.2 mg g−1 while other types of solvents (80% ethanol and 20% acetonitrile) yielded no traces of this compound. These authors, through a two-step maceration, obtained 0.49 and 1.95 mg g−1, using ethanol/methanol and ethanol/distilled water, respectively. Also, in the study of Benincasa et al.40 the leaf extracts, obtained by maceration using three types of water, contained minute amounts of tyrosol: 3.4 mg kg−1 (microfilter water), 0.1 mg kg−1 (ultrapure water) and 0.2 mg kg−1 (osmosis-treated water).
Martín-Vertedor et al.41 through their investigation using ultrapure water, 3 h, and 60–65 °C detected 1681 mg per kg of aqueous leaf extract. In another research study, through hydro-distillation followed by extraction with ethyl acetate, the leaf extracts yielded around 0.0359 (g L−1).42 Moreover, Martín-García et al.,43 examined several cultivars (Spain) through an optimized sonotrode ultrasound-assisted system (55% ethanol, 100% amplitude, 8 min) and reported 0.0016 mg g−1 (Picual), 0.007 mg g−1 (Koroneiki), 0.005 mg g−1 (Frantoio and Changlot Real), 0.014 (Sikitita), 0.012 (Arbequina), and 0.00439 mg g−1 (Arbosana).
Verbascoside content.
As a phenylethanoid glycoside, this compound is among the valuable bioactive molecules with strong antioxidant, anti-carcinogenic, anti-inflammatory, and anti-microbial effects.44 It is structurally characterized by an ester of caffeic acid (attached to sugar) and hydroxytyrosol. As shown in Fig. 5, the highest level of verbascoside (1.04 mg per g extract) belongs to the one extracted by using an ohmic heater at 75 °C with 80% ethanol.
 |
| Fig. 5 Effect of extraction methods on the recovery of verbascoside from olive leaves (mg per g leaf extract). Extraction methods: ohmic heating (OH), conventional heating (conven), and solvent extraction without heating (control). The results are presented as mean values with standard deviation error bars. | |
Intriguingly, the abovementioned extraction approach (ohmic at 75 °C, 80% ethanol) proved useful for oleuropein content, through which its concentration rose to the highest level (see Fig. 2). Moreover, in our previous study,6 the antiradical activities reached the maximum in the OH extract at the same temperature/solvent ratio. This may further indicate that both oleuropein and verbascoside dose-dependently contributed to the rise in antioxidant capacity.
The extractability of verbascoside from olive leaves partly depends on the type of cultivar and collection time. In a recent report45 on the characterization of phenolic compounds in different varieties of olive leaves grown in Italy (collection time: April and November), the greatest amount of verbascoside was detected in samples from Coratina cultivar (142.2 mg/100 g extract). In another research study performed by Orak et al.,35 the quantified verbascoside showed significantly large variations across nine different genotypes (range: 0.45–21.07 mg per g leaf extract).
Another influential factor in the recovery of verbascoside from olive leaves evidently is the processing approach. Particular examples of the values reported in previous studies are: 18.5 mg g−1 using ultrasound-assisted extraction; 22.9 ppm using cloud point extraction based on the salting-out phenomenon; 3.90 mg g−1 in Picual leaf extracts using microwave extraction with water.46–48
Flavonoid derivatives.
Flavonoids in olive leaves are predominately in the glycosidic form and are clustered into different sub-classes. The following are among the most typical flavonoid-derivatives in olive leaves:
Luteolin 7-O-glucoside content.
Luteolin belongs to flavones, a representative sub-class of flavonoids in olive leaves. This phenol is mostly in the glycosidic linkage form in olive leaves; and includes luteolin 7-O-glucoside that has been regarded as one of the principal polyphenols in olive leaves.49,50 The values observed in this study (Fig. 6) revealed that ohmic heating, despite variations, exhibited significantly higher concentrations, in the range of 3.02–4.17 mg g−1, particularly using ohmic heating at 55 °C with 60% ethanol being the most effective for the liberation of this compound.
 |
| Fig. 6 Effect of extraction methods on the recovery of luteolin 7-O-glucoside from olive leaves (mg per g leaf extract). Extraction methods: ohmic heating (OH), conventional heating (conven), and solvent extraction without heating (control). The results are presented as mean values with standard deviation error bars. | |
The data from previous studies show large variations in the content of luteolin 7-O-glucoside which partly explains the contributing effects of processing/extraction methods. Contreras et al.,26 reported 237–338 mg per 100 g of extracts from olive mill leaves. Olive leaves extracted by ultrasound-assisted and conventional (with agitation) methods presented around 11.0 and 9.7 mg luteolin-7-O-glucoside per gram, respectively.46 In another study,50 an optimized design of pressurized liquid extraction (using 80% aqueous ethanol, 190 °C, 5 min) has been shown to improve the recovery of this compound (2.71 g per kg dry olive leaves). Moreover, Kashaninejad et al.,51 through their research on UAE and conventional methods, found that freeze-dried extracts using 80% ethanol led to an increase in the content of bio-phenols including luteolin 7-O-glucoside (1.4% w/w).
Apigenin 7-O-glucoside content.
Another major flavone-derived phenol is apigenin that is typically in the glycosidic bond form in olive leaves. Of all the constituents, apigenin 7-O-glucoside is among the prominent glycosides of apigenin in olive leaves. As shown in Fig. 7, the proportion of this compound reached up to 3.47 mg per g extract using ohmic heating at 55 °C, 60% ethanol.
 |
| Fig. 7 Effect of extraction methods on the recovery of apigenin 7-O-glucoside from olive leaves (mg per g leaf extract). Extraction methods: ohmic heating (OH), conventional heating (conven), and solvent extraction without heating (control). The results are presented as mean values with standard deviation error bars. | |
Together with others, the extractability of this particular phenol from olive leaves may highly depend on the selected cultivar. In the study of Lukić et al.,52 the cultivar type factor notably became influential in phenolic concentrations including that of apigenin 7-O-glucoside and showed large variations across six selected cultivars of olive leaves (27.79–91.75 mg/100 g).
Rutin content.
Similar to other flavonoid phenols examined thus far, the maximum rutin content (3.78 mg per g extract) was detected in the ohmic extract at 55 °C and 60% ethanol (Fig. 8). The extractability of rutin is reportedly influenced by the nature and/or proportion of the extraction solvent(s). A typical example is the research of Akli et al.,39 wherein olive leaves exhibited discrepancies in rutin proportions depending on the extraction solvents, as the extraction by using a conventional solvent (70% ethanol) presented 714.63 μg rutin per g, while the extraction by using eutectic solvents enabled recoveries in the range of 268.59–463.06 μg g−1.
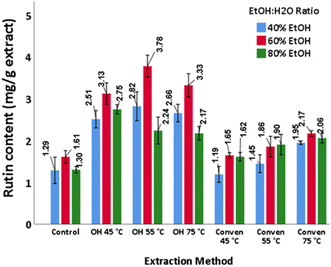 |
| Fig. 8 Effect of extraction methods on the recovery of rutin components from olive leaves (mg per g leaf extract). Extraction methods: ohmic heating (OH), conventional heating (conven), and solvent extraction without heating (control). The results are presented as mean values with standard deviation error bars. | |
Furthermore, in another research study51 olive leaves from the same cultivar as used in the current study (Picual) have showed dissimilarities in the rutin content when different solvent ratios were examined. The results ranged from 0.026 (100% ethanol) to 0.5 mg g−1 (50% ethanol). Talhaoui et al.,53 observed variations in the concentrations of rutin from Picual, Sikitita, and Arbequina, which were 0.289, 0.319, and 0.651 mg per g leaves (dw), respectively.
Overall the findings of the present study demonstrate that the use of ohmic extraction significantly favored the highest recovery of individual phenols in this study, when compared to the other two methods with p < 0.05. It is also noteworthy that the extractability of each phenolic group differed with solvents/temperatures as the data showed that ohmic heating at 55 °C with 60% ethanol produced the highest levels of flavonoid phenols (rutin, apigenin 7-O-glucoside, and luteolin 7-O-glucoside), while ohmic heating at 75 °C with 80% ethanol exhibited maximum values of oleuropein and verbascoside. These discrepancies can be explained by their inherently diverse structural features in terms of (i) chemical composition, (ii) locations within the cells, and (iii) molecular bindings.
Given the above, using an ohmic heater that proved useful for the extractability of the bio-phenols of this study, potentially entails appropriate customization of the solvent ratio and/or extraction temperature when the isolation of specific polyphenol(s) is of importance.
Conclusions
The present study showed that ohmic heating, compared to conventional heating and solvent/non-heating extraction (control), was significantly effective in the recoveries of all groups of phenols examined in this study, particularly oleuropein, verbascoside, and hydroxytyrosol (p < 0.05). By comparison, the magnitude of differences between ohmic groups and conventional groups (at the same extraction temperature) was significantly high. The interactive effects of ohmic heating and the solvent ratio showed that the use of ohmic heating with 80% ethanol enabled the highest recoveries of oleuropein, hydroxytyrosol, tyrosol, and verbascoside, while 60% ethanol represented optimum values of luteolin 7-O-glucoside, apigenin 7-O-glucoside, and rutin.
The data obtained in this study in part shed light on the significant roles of ohmic heating in the extraction of the aforementioned phenolic groups that characteristically are among the major antioxidants in olive leaves. This study can be further extended in future research work to evaluate an up-scalable process benchmark for the application of an ohmic heater to optimally extract the desired bio-phenol(s) from olive leaf residues. More research work is also needed to ascertain adaptations of the extraction parameters/conditions, particularly in terms of the solvent ratio and extraction temperature when the liberation of certain types of phenols is of particular interest.
Author contributions
Fereshteh Safarzadeh Markhali: investigation; methodology; writing the manuscript; conceptualization; data analysis; resources. José A. Teixeira: conceptualization; supervision; methodology; review and editing; resources.
Conflicts of interest
There are no conflicts to declare.
Acknowledgements
The authors wish to thank Eulogio Castro, Center for Advanced Studies in Energy and Environment, University of Jaén, Campus of Las Lagunillas, Jaén, Spain, for providing the olive leaves. This study was supported by the Portuguese Foundation for Science and Technology (FCT), under the scope of the strategic funding of the UID/BIO/04469/2020 unit.
References
- F. S. Markhali, J. A. Teixeira and C. M. Rocha, Processes, 2020, 8(9), 1177 CrossRef CAS.
- G. S. da Rosa, S. K. Vanga, Y. Gariepy and V. Raghavan, Innovative Food Sci. Emerging Technol., 2019, 58, 102234 CrossRef.
- F. Le Floch, M. T. Tena, A. Rıos and M. Valcarcel, Talanta, 1998, 46, 1123–1130 CrossRef CAS PubMed.
- I. Žuntar, P. Putnik, D. B. Kovačević, M. Nutrizio, F. Šupljika, A. Poljanec, I. Dubrović, F. J. Barba and A. R. Jambrak, Foods, 2019, 8(7), 248 CrossRef PubMed.
- V. M. Pappas, A. Lakka, D. Palaiogiannis, E. Bozinou, G. Ntourtoglou, G. Batra, V. Athanasiadis, D. P. Makris, V. G. Dourtoglou and S. I. Lalas, Beverages, 2021, 7(3), 45 CrossRef CAS.
- F. S. Markhali, J. A. Teixeira and C. M. Rocha, Clean Technol., 2022, 4(2), 512–528 CrossRef.
- M. Fraga-Corral, P. García-Oliveira, A. G. Pereira, C. Lourenço-Lopes, C. Jimenez-Lopez, M. A. Prieto and J. Simal-Gandara, Molecules, 2020, 25(3), 614 CrossRef CAS PubMed.
- A. K. Das, M. N. Islam, M. O. Faruk, M. Ashaduzzaman and R. Dungani, S. Afr. J. Bot., 2020, 135, 58–70 CrossRef CAS.
- M. T. P. Dentinho, K. Paulos, A. Francisco, A. T. Belo, E. Jerónimo, J. Almeida, R. J. B. Bessa and J. Santos-Silva, Livest. Sci., 2020, 236, 104021 CrossRef.
- R. O. Martins, I. C. Gomes, A. D. M. Telles, L. Kato, P. S. Souza and A. R. Chaves, J. Chromatogr. A, 2020, 1620, 460977 CrossRef CAS PubMed.
- A. Qawasmeh, H. K. Obied, A. Raman and W. Wheatley, J. Agric. Food Chem., 2012, 60(13), 3381–3388 CrossRef CAS PubMed.
- R. Julkunen-Tiitto, J. Agric. Food Chem., 1985, 33(2), 213–217 CrossRef CAS.
- J. Quero, L. F. Ballesteros, P. Ferreira-Santos, G. R. Velderrain-Rodriguez, C. M. Rocha, R. N. Pereira, J. A. Teixeira, O. Martin-Belloso, J. Osada and M. J. Rodríguez-Yoldi, Antioxidants, 2022, 11(5), 828 CrossRef CAS PubMed.
- F. S. Markhali and J. A. Teixeira, Sustain. Food Technol., 2023, 1(6), 896–905 RSC.
- O. H. Lee, B. Y. Lee, J. Lee, H. B. Lee, J. Y. Son, C. S. Park, K. Shetty and Y. C. Kim, Bioresour. Technol., 2009, 100(23), 6107–6113 CrossRef CAS PubMed.
- G. Ghelichkhani, M. H. Modaresi, L. Rashidi, N. Shariatifar, M. Homapour and M. Arabameri, J. Food Meas. Char., 2019, 13, 2751–2763 CrossRef.
- L. Abaza, A. Taamalli, H. Nsir and M. Zarrouk, Antioxidants, 2015, 4, 682–698 CrossRef CAS PubMed.
- S. Agatonovic-Kustrin, V. Gegechkori, D. S. Petrovich, K. T. Ilinichna and D. W. Morton, Molecules, 2021, 26(22), 6892 CrossRef CAS PubMed.
- P. G. Lins, S. M. P. Pugine, A. M. Scatolini and M. P. de Melo, Heliyon, 2018, 4(e00805) Search PubMed.
- A. M. García, A. Moumen, D. Y. Ruiz and E. M. Alcaide, Anim. Feed Sci. Technol., 2003, 107, 61–74 CrossRef.
- E. Molina-Alcaide and D. R. Yáñez-Ruiz, Anim. Feed Sci. Technol., 2008, 147, 247–264 CrossRef CAS.
- M. Olfaz, U. Kilic, M. Boga and A. M. Abdi, Open Life Sci., 2018, 13(1), 269–278 CAS.
- K. Fegeros, G. Zervas, F. Apsokardos, J. Vastardis and E. Apostolaki, Small Rumin. Res., 1995, 17(1), 9–15 CrossRef.
- S. B. Mansour-Gueddes, D. Saidana-Naija, A. Bchir and M. Braham, Not. Bot. Horti Agrobot. Cluj-Napoca, 2020, 48(1), 436–455 CrossRef.
- S. Guebebia, K. B. Othman, Y. Yahia, M. Romdhane, W. Elfalleh and H. Hannachi, Int. J. Plant Bas. Pharm., 2022, 2(1), 17–24 CrossRef.
- M. D. M. Contreras, A. Lama-Muñoz, J. M. Gutiérrez-Pérez, F. Espínola, M. Moya, I. Romero and E. Castro, Foods, 2019, 8(11), 531 CrossRef PubMed.
- S. Şahin, N. S. A. Malik, J. L. Perez and J. E. Brockington, J. Agric. Sci. Technol., 2012, 242–247 Search PubMed.
- K. Stamatopoulos, A. Chatzilazarou and E. Katsoyannos, Foods, 2014, 3(1), 66–81 CrossRef PubMed.
- P. J. Xie, L. X. Huang, C. H. Zhang, F. You and Y. L. Zhang, Food Bioprod. Process., 2015, 93, 29–38 CrossRef CAS.
- M. Irakli, P. Chatzopoulou and L. Ekateriniadou, Ind. Crops Prod., 2018, 124, 382–388 CrossRef CAS.
- K. Márquez, N. Márquez, F. Ávila, N. Cruz, A. Burgos-Edwards, X. Pardo and B. Carrasco, Front. Nutr., 2022, 9, 1–14 Search PubMed.
- M. H. Ahmad-Qasem, E. Barrajón-Catalán, V. Micol, A. Mulet and J. V. García-Pérez, Food Res. Int., 2013, 50, 189–196 CrossRef CAS.
- O. Ghomari, F. Sounni, Y. Massaoudi, J. Ghanam, L. B. D. Kaitouni, M. Merzouki and M. Benlemlih, Biotechnol. Rep., 2019, 23, e00347 CrossRef PubMed.
- F. Ortega-García and J. Peragón, J. Agric. Food Chem., 2010, 58(23), 12440–12448 CrossRef PubMed.
- H. H. Orak, M. Karamać, R. Amarowicz, A. Orak and K. Penkacik, Molecules, 2019, 24, 1130 CrossRef CAS PubMed.
- A. S. Caballero, J. M. Romero-García, E. Castro and C. A. Cardona, J. Chem. Technol. Biotechnol., 2020, 95(2), 356–362 CrossRef CAS.
- E. Zurob, R. Cabezas, E. Villarroel, N. Rosas, G. Merlet, E. Quijada-Maldonado, J. Romero and A. Plaza, Sep. Purif. Technol., 2020, 248, 117054 CrossRef CAS.
- A. K. Marković, J. Torić, M. Barbarić and C. Jakobušić Brala, Molecules, 2019, 24, 1–39 Search PubMed.
- H. Akli, S. Grigorakis, A. Kellil, S. Loupassaki, D. P. Makris, A. Calokerinos, A. Mati and N. Lydakis-Simantiris, Appl. Sci., 2022, 12(2), 831 CrossRef CAS.
- C. Benincasa, I. Santoro, M. Nardi, A. Cassano and G. Sindona, Molecules, 2019, 24(19), 3481 CrossRef CAS PubMed.
- D. Martín-Vertedor, M. Garrido, J. A. Pariente, J. Espino and J. Delgado-Adámez, Phytother. Res., 2016, 30(7), 1172–1179 CrossRef PubMed.
- A. Issaoui, A. Mahfoudh, H. Ksibi and M. Ksibi, Trends Chem. Eng., 2012, 14, 65–69 CAS.
- B. Martín-García, S. De Montijo-Prieto, M. Jiménez-Valera, A. Carrasco-Pancorbo, A. Ruiz-Bravo, V. Verardo and A. M. Gómez-Caravaca, Antioxidants, 2022, 11(3), 558 CrossRef PubMed.
- K. Alipieva, L. Korkina, I. E. Orhan and M. I. Georgiev, Biotechnol. Adv., 2014, 32(6), 1065–1076 CrossRef CAS PubMed.
- G. Difonzo, M. A. Crescenzi, S. Piacente, G. Altamura, F. Caponio and P. Montoro, Plants, 2022, 11(23), 3321 CrossRef CAS PubMed.
- M. H. Ahmad-Qasem, J. Cánovas, E. Barrajón-Catalán, V. Micol, J. A. Cárcel and J. V. García-Pérez, Innovative Food Sci. Emerging Technol., 2013, 17, 120–129 CrossRef CAS.
- K. Stamatopoulos, E. Katsoyannos and A. Chatzilazarou, Antioxidants, 2014, 3(2), 229–244 CrossRef PubMed.
- M. E. Martínez-Navarro, C. Cebrián-Tarancón, J. Oliva, M. R. Salinas and G. L. Alonso, Antioxidants, 2021, 10(12), 1963 CrossRef PubMed.
- K. Kiritsakis, A. M. Goula, K. G. Adamopoulos and D. Gerasopoulos, Waste Biomass Valorization, 2018, 9, 619–633 CrossRef CAS.
- A. Lama-Muñoz, M. D. M. Contreras, F. Espínola, M. Moya, A. de Torres, I. Romero and E. Castro, Food Chem., 2019, 293, 161–168 CrossRef PubMed.
- M. Kashaninejad, M. T. Sanz, B. Blanco, S. Beltrán and S. M. Niknam, Food Bioprod. Process., 2020, 124, 196–207 CrossRef CAS.
- I. Lukić, I. Pasković, P. Žurga, V. M. Germek, M. Brkljača, Š. Marcelić, D. Ban, K. Grozić, M. Lukić, Z. Užila and S. Goreta Ban, Plants, 2020, 9(12), 1667 CrossRef PubMed.
- N. Talhaoui, A. M. Gómez-Caravaca, L. León, R. De la Rosa, A. Segura-Carretero and A. Fernández-Gutiérrez, LWT--Food Sci. Technol., 2014, 58(1), 28–34 CrossRef CAS.
|
This journal is © The Royal Society of Chemistry 2024 |
Click here to see how this site uses Cookies. View our privacy policy here.