Effect of processing Verdejo grape must by UHPH using non-Saccharomyces yeasts in the absence of SO2†
Received
29th November 2023
, Accepted 8th January 2024
First published on 16th January 2024
Abstract
Ultra-High Pressure Homogenization (UHPH) is an emerging non-thermal technology that can eliminate wild microorganisms from grape juice facilitating the use of non-competitive non-Saccharomyces yeast in fermentation to modulate the sensory profile. The use of UHPH processing in must from Verdejo variety grapes (Vitis vinifera L.) produces a more varietal profile reducing the contents of fermentative fruity varietal esters (−25–50%) and enhancing the release of volatile thiols (+25–75%). The aromatic profile of UHPH wines is clearly separated of controls by the aroma PCA. Additionally, the inactivation of oxidative enzymes by UHPH preserves a better color in wines with a paler yellow color and lower b* values. A better implantation of some non-Saccharomyces yeasts such as Lachancea thermotolerans can help to reduce the pH in wines from warm areas. Improved varietal aroma, paler wine color and, depending on the strain fermented with, lower pH help control the impact of global warming on wines.
Sustainability spotlight
UHPH is a sustainable and environmentally friendly technology with very low power consumption (50% lower than HHP), even lower compared with conventional thermal treatments. The small system volume demands very moderate requirements of the process and cleaning water. Additionally, the use of steam to sanitize or sterilize the system is unnecessary because it can be done by pressure, and just hot water at 70–80 °C as technical fluid is necessary. Furthermore, the low impact of this technology in sensory and nutritional quality favors the production of healthier and less processed foods. This technology can support the United Nations' Sustainable Development Goals (SDGs), especially Goal 2 (zero hunger) and Goal 13 (climate action).
|
1. Introduction
Ultra-High Pressure Homogenization (UHPH) is an emerging technology that can be considered non-thermal because of the gentle effect on sensitive molecules with impact on the sensory profile and micronutrients.1–4 The UHPH process is done by ultra-high pressure pumping at more than 200 MPa (normally 300 MPa) followed by subsequent instantaneous depressurization (<0.2 seconds) across a highly resistant valve.1,4 It has shown a protective effect on terpenes and other aroma compounds,5,6 anthocyanins7 and vitamins.8 Due to the extreme impact and shear forces in the valve the temperature can be very high (75–150 °C), but with low thermal impact because of the very short residence time, and there is a very scarce formation of thermal markers such as HMF,5 furosine,9 or carcinogenic compounds such as ethyl carbamate, the formation of which may be enhanced by temperature.10
UHPH can control easily high microbial loads in liquid foods, and the inactivation of yeast populations of 6–4
log
CFU mL−1 belonging to the Saccharomyces cerevisiae (Sc) species but also to other non-Saccharomyces yeasts7,11 and 4–3
log of aerobic bacteria in grape juices7,11 has been reported. Depending on the in-valve temperature also sporulate bacteria can be eliminated and it has been observed that under mild conditions (80–90 °C of in-valve temperature) the off-flavor producer, acidophilic and thermoresistant Alicyclobacillus acidoterrestris can be eliminated. Additionally, UHPH produces an effective control of enzymes,10,12 especially oxidative enzymes (PPOs), with inactivation higher than 90% and a control of browning.5,11,13 Therefore this technology can be used to produce wines with a low or null content of SO2.4,5,7
The use of non-Saccharomyces is a current hot topic in wine biotechnology because of their ability to modify and improve the sensory profile of wines enhancing wine quality, aroma, flavor, structure, freshness and color.14–18 Several species of non-Saccharomyces have deserved special attention because of their impact on wine quality among them: Torulaspora delbrueckii (Td) by its impact on aroma, foaming properties, and recently in bioprotection,19,20Metschnikowia pulcherrima (Mp) by the expression of enzymatic activities, capacity to lower the ethanol, and bioprotective effect,21,22Lachancea termotolerans (Lt) by acidification and aroma improvement,23–25 and Hanseniaspora vineae (Hv) by aroma and impact on the body and structure.26,27 A major problem of many non-Saccharomyces yeasts is that they are weaker fermenters compared to Sc, and they have problems to compete and be prevalent in must fermentation. The use of UHPH is a way of eliminating all the competitive wild yeasts in grape juice promoting a good implantation of starters from non-Saccharomyces yeasts. Additionally, it can be done at low levels of SO2 that is harmful for many non-Saccharomyces.
Furthermore, UHPH is a sustainable and environmentally friendly technology because of the low power consumption (50% lower than HHP) and the small system volume with low requirements of the process and cleaning water (https://www.ypsicon.com/). Additionally, the use of steam to sanitize or sterilize the system is unnecessary because it can be done by pressure, and just hot water at 70–80 °C as technical fluid is necessary.
The aim of this research is to study the effect of UHPH processing on grape must of Verdejo variety and the impact on the quality of the wines produced after fermentation by several non-Saccharomyces species.
2. Materials and methods
2.1. Grape juice
The must was obtained from Verdejo blanco grapes (Vitis vinifera L.; VIVC variety number: 12
949) from the DO Rueda in Valladolid, Spain. Grapes were mechanically harvested and pressed using a pneumatic press model Diemme 150 (Lugo, Ravenna, Italy) at less than 2 bar, and the yield was 60% of grape juice; after that the juice obtained was settled at low temperature (4 °C) for 2 days and processed by UHPH. No sulphites were added to the juice. The settling was performed without the use of pectolytic enzymes.
2.2. UHPH treatment
After settling the must was processed with a 60 L h−1 UHPH device (Ypsicon Technologies, Barcelona, Spain) at 300 ± 3 MPa with an inlet temperature of 8 °C, reaching 92 °C in the UHPH valve and being refrigerated after valve depressurization and by additional continuous cooling at 15 °C. Processed juice was aseptically packed in sterilized 2 L plastic bags in the absence of O2 and stored refrigerated at 4 °C until fermentation. Control unprocessed juice was also kept at 4 °C until fermentation in 5 L plastic bottles.
2.3. Fermentation and inoculation
UHPH juices and unprocessed controls were dosed at 200 mL in 250 mL ISO flasks and kept isothermally at 20 °C during fermentation. Inocula of Saccharomyces and non-Saccharomyces yeasts were grown in YPD liquid media, and the populations were synchronized by two successive passes of 2% v/v in 24 h before the final inoculation in the fermenters. Inoculation volume was 2% v/v (4 mL), producing a final population of 7
log
CFU mL−1. The inocula were grown at 20 °C. Fermentation was performed in triplicate. All fermentation samples of non-Saccharomyces were inoculated again on the 8th day of fermentation with S. cerevisiae (Sc7VA) until total sugar depletion to ensure the dryness of wines.
The Sc and non-Saccharomyces yeasts used were Saccharomyces cerevisiae strain 7VA (Sc7VA) selected at the enotecUPM lab (Madrid, Spain), Lachancea thermotolerans L31 (L31) selected at the enotecUPM lab, Torulaspora delbrueckii BIODIVA™ (Td) Lallemand, Hanseniaspora vineae 205 (Hv) selected by the Professor Carrau team at the Universidad de la República (Uruguay), and Metschnikowia pulcherrima M29 (M29) selected at the enotecUPM lab. All of them were kept in YPD agar and refreshed by a pass in solid YPD agar and growth at 20 °C for 48 h previously to be synchronized in the liquid YPD.
All fermentation samples from days 2, 4 and 6 were monitored for inoculated and wild yeast populations by plate seeding according to Section 2.5.
2.4. Optical microscopy of the juice
Optical microscopy was performed on a drop of sediment of the control and UHPH juices using a BA310 LED microscope (Motic, San Antonio, USA) with a 60× achromatic objective and a digital camera FULLHD Moticam 1080INT. The sediment was obtained after centrifugation at 5000 rpm for 5 minutes of 3 mL of juice in an Eppendorf tube.
2.5. Yeast counts
Yeast counts were determined by plating in YPD agar for total yeasts and in lysine agar and CHROMagar™ Candida (Conda, Barcelona, Spain) for non-Saccharomyces counts. The identification was done according to the color and colony appearance in the selective and differential media. Plates were counted after 48 h at 25 °C in the dilution that had a count number in the range of 30–300 colonies.
2.6. Lactic acid and enological parameters
L-Lactic acid has been analyzed at the end of fermentation by enzymatic analysis using an automatic enzymatic analyzer Y25 (BioSystems, Barcelona, Spain). Previously samples were filtered with 0.45 μm membrane filters.
The major compounds at the end of fermentation, such as residual sugars, organic acids, and total acidity, were measured with a FTIR OenoFoss equipment (FOSS Iberia, Barcelona, Spain). The pH was measured using a CRISON micropH 2000 pHmeter (HACH LANGE, Barcelona, Spain).
2.7. Fermentative volatiles quantified by GC-FID
Fermentative volatiles were quantified by gas chromatography with a flame ionization detector (GC-FID) using an Agilent Technologies 6850 gas chromatograph. The injector temperature was set at 250 °C, and the FID was at 300 °C. Compounds were separated on a DB-624 column (60 m × 250 μm × 1.40 μm) with a 1
:
10 split ratio. The quantification was performed using GC quality standards: acetaldehyde, methanol, 1-propanol, 1-butanol, 2-butanol, isobutanol, 2-methyl-1-butanol, 3-methyl-1-butanol, 2-phenylethyl acetate, 2-phenylethyl alcohol, diacetyl, ethyl acetate, isoamyl acetate, isobutyl acetate, ethyl butyrate, 3-ethoxy-1-propanol, ethyl lactate, and hexanol (Fluka, Sigma-Aldrich Corp., Buchs SG, Switzerland). The compound 4-methyl-2-pentanol was added as the internal standard, also from Fluka. The temperature program for the oven was 40 °C for five minutes, and then an increase of 10 °C per minute up to 250 °C, which was kept for five minutes, with a total running and conditioning time of 40 minutes. The carrier gas was hydrogen with a column flow of 2.2 mL min−1. One mL of sample was filtered with a 0.45 μm membrane and spiked with 100 μL of IS. The injection volume was 1 μL. The detection limit was set at 0.1 mg L−1. The volatile compounds analyzed were calibrated using a five-point calibration curve with r2 > 0.999, except 2,3-butanediol (0.991) and 2-phenylethyl alcohol (0.994).
2.8. Volatile compounds quantified by HS-SPME-GC-MS
Volatile compounds (VOCs) were determined by SPME-GC-MS by slightly modifying the method published by Tat et al.28 The following compounds were analysed: ethyl hexanoate, hexyl acetate, ethyl-3-hexenoate, 3-hexen-1-ol acetate, 3-hexen-1-ol, (E)-, 3-ethoxy-1-propanol, 3-hexen-1-ol, (Z)-, ethyl octanoate, acetic acid, 3-methylbutyl hexanoate, ethyl-3-octenoate, 2-ethyl-1-hexanol, benzaldehyde, linalool, 1-octanol, 2-methylpropanoic acid, γ-butyrolactone, butanoic acid, ethyl decanoate, 3-methylbutanoic acid, diethyl succinate, ethyl 9-decenoate, 3-methylthio-1-propanol, β-citronellol, ethyl phenylacetate, ethyl 4-hydroxybutanoate, β-damascenone, hexanoic acid, phenyl methanol (benzyl alcohol), octanoic acid, 4-vinylguaiacol, ethyl hexadecanoate, decanoic acid, ethyl 9-hexadecenoate, ethyl octadecanoate, and ethyl 9-octadecenoate. The instrument used was a GCMS-QP2020 NX GC-MS system (Shimadzu, Kyoto, Japan), equipped with a 2800T autosampler (HTA S.r.l., Brescia Italy). Samples were prepared as follows: 10 mL of filtered wine were introduced into 20 mL glass vials and mixed with 3 g of NaCl; 100 μL of ethyl heptanoate (0.106 g L−1 in ethanol) was added as the internal standard and vials were sealed with PTFE/silicon septa. SPME was carried out using a 2 cm long 50/30 μm DVB/Carboxen/PDMS fiber (Supelco, Bellefonte, PA, USA), at 40 °C for 15 min. Vials were pre-conditioned in an autosampler for 15 min before microextraction, to allow the thermal equilibration of the samples. Injections were performed in splitless mode, with a splitless time of 60 s. The temperatures of the injector and the transfer line were 250 °C and 240 °C respectively, while the ion source was set at 200 °C. The carrier gas was helium at a linear flow rate of 36 cm s−1. Compounds were separated on a J&W DB-Wax capillary column, with 30 m × 0.25 mm i.d. and 0.25 μm film thickness (Agilent Technologies Inc., Santa Clara, CA, USA), according to the gradient reported by Comuzzo et al. (2018).29 Electron impact mass spectra were recorded at 70 eV and the identification of volatile compounds was carried out by comparison of their mass spectra and retention times with those of standard compounds, or by comparison of the mass spectrum with those reported in the mass spectrum libraries Wiley 6 and NIST 107. Linear retention indices were also calculated on the basis of the retention times of n-alkanes and compared with those reported in the literature. Semi-quantitative analysis was performed by the internal standard method, considering a response factor equal to 1.00.
2.9. Thiols quantified by LC-MS/MS
The volatile thiols 3-mercaptohexan-1-ol (3 MH), 4-mercaptopentan-2-one (4MMP), 4-mercapto-4-methylpentan-2-ol (4MMPOH), and 3-mercaptohexyl acetate (3MHA) were analysed by LC-MS/MS. Wines were extracted with modified Quechers using a mixture of ethyl acetate/acetonitrile (1
:
1) without the clean-up step. An aliquot of this extract was analyzed by liquid chromatography coupled to mass spectrometry (LC-MS/MS). The equipment used was an Agilent 1290 Chromatograph coupled to a triple quadrupole mass spectrometer (model 6460) also from Agilent. The ionization source was electrospray type (ESI) with a gas temperature of 300 °C at a flow of 5 L min−1. The separation was carried out on a Phenomenex C18 column with the following dimensions (100 × 3 mm, 250 A, and 2.6 μm) at a temperature of 25 °C and an injection volume of 5 μL. The mobile phases were as follows: (A) 0.1% formic acid in water and (B) acetonitrile at a flow rate of 0.5 mL min−1. Detection was performed in MRM mode for each of the thiols.
2.10. Color
The DNA Phone Smart Analysis – Wine device (Parma, Italy) was used to determine the color parameters. The parameters included the CIELChuv coordinates for chroma (C) and hue (h), as well as the CIELab coordinates for lightness (L*), green-red (a*), and blue-yellow (b*). The samples were put into polymethyl methacrylate cuvettes with a 1 mm path length. The samples were filtered using 0.45 μm methyl-cellulose membranes before analysis.
2.11. Statistical analysis
Statistical analysis was performed using Statgraphics Centurion v19 software (Graphics Software Systems, Rockville, MD, United States) to calculate the mean, standard deviation, analysis of variance (ANOVA), and least significant difference (LSD). Significance was set at p < 0.05.
SPME-GC-MS data were elaborated by Principal Component Analysis (PCA) and One-Way ANOVA, using Statistica for Windows (version 8.0). Regarding PCA, factor loadings (FLs) were calculated by factor analysis, and the most relevant variables were selected for PCA, when marked FLs were higher than 0.7. Concerning One Way ANOVA, means and standard deviations were calculated, and significant differences were assessed by a Tukey HSD test at p < 0.05. Variances were homogeneous according to the Brown–Forsythe test.
3. Results and discussion
3.1. Results
3.1.1. Micro- and macroscopic effects of UHPH.
The raw grape juice after settling shows a low colloidal turbidity with a yellow pale color that starts to show some browning in the beaker (Fig. 1A). The microscopic preparation shows abundant crystal fragments of tartrates together with colloidal particles from the cell walls of the grape pulp. The fragments were irregular in size and shape. The UHPH processed juice showed a thin colloidal structure with a more turbid appearance in the beaker and paler color (Fig. 1B). The microscopic aspect was thinner with practically not observable crystals or other big colloidal fragments. The size was much more regular with a homogeneous granulometry.
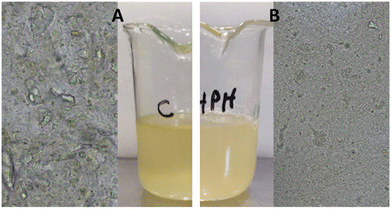 |
| Fig. 1 Optical microscopic and macroscopic appearance of the unprocessed control must made from Verdejo grapes (Vitis vinifera L.) (A) and the UHPH processed (B). | |
3.1.3. Lactic acid and enological parameters.
Lachancea thermotolerans has been included because of its ability to transform sugars into lactic acid with high efficiency in some strains facilitating a good control of the pH. As can be observed the fermentative production of lactic acid is very scarce for Sc or most of the non-Saccharomyces ranging below 100 mg L−1 (Fig. 2). However, Lt is able to produce contents of several g L−1. In the must processed by UHPH and the control, the differences were non-significant with a value ranging from 1.7 to 1.9 g L−1 with a clear impact on pH. The average pH of the fermentation samples with all the strains except Lt is 3.7, while fermentation managed with Lt produced a pH reduction of 0.2 units (Table 2), which means an approximate decrease of 0.1 pH units per 0.8–1.0 g L−1 of lactic acid.
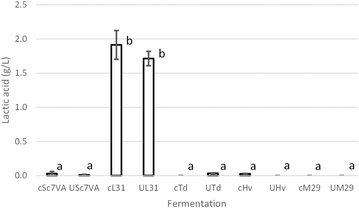 |
| Fig. 2 Lactic acid production in control (c) and UHPH (U) must fermented by Saccharomyces cerevisiae (Sc7VA), Lachancea thermotolerans (L31), Torulaspora delbrueckii (Td), Hanseniaspora vineae (Hv), and Metschnikowia pulcherrima (M29). Lactic acid determined by enzymatic analysis. Values are means ± sd (fermentation in triplicate) in g L−1. Different letters mean significant differences (p < 0.05). | |
Table 2 Enological parameters in controls and UHPH must fermented by Saccharomyces cerevisiae (Sc7VA), Lachancea thermotolerans (L31), Torulaspora delbrueckii (Td), Hanseniaspora vineae (Hv), and Metschnikowia pulcherrima (M29). Compounds analyzed by FTIR, and values are means ± sd (fermentation in triplicate) in g L−1. Different letters mean significant differences (p < 0.05)
|
Ethanol (% v/v) |
Total acidity (g L−1)a |
pH |
Volatile acidity (g L−1)b |
Residual sugars (g L−1) |
Malic acid (g L−1) |
TPIc |
Glucose (g L−1) |
Fructose (g L−1) |
Total acidity expressed in tartaric acid.
Volatile acidity in acetic acid.
TPI (total polyphenol index).
|
cSc7VA Control |
12.9 ± 0.0bc |
4.2 ± 0.1ab |
3.7 ± 0.0c |
0.2 ± 0.0a |
1.5 ± 0.2d |
1.3 ± 0.0 cd |
12.7 ± 1.8d |
0.8 ± 0.1a |
0.9 ± 0.3bcd |
USc7VA UHPH |
12.4 ± 0.3ab |
4.1 ± 0.1ab |
3.7 ± 0.0bc |
0.4 ± 0.0c |
1.5 ± 0.1d |
1.3 ± 0.1bc |
11.7 ± 2.0cd |
0.9 ± 0.1a |
0.8 ± 0.1bc |
cL31 Control |
12.8 ± 0.1bc |
6.3 ± 0.2f |
3.5 ± 0.0a |
0.3 ± 0.0b |
1.1 ± 0.1ab |
1.1 ± 0.1b |
11.2 ± 1.3bcd |
0.8 ± 0.1a |
0.4 ± 0.1a |
UL31 UHPH |
12.5 ± 0.1ab |
5.5 ± 0.2e |
3.5 ± 0.0a |
0.2 ± 0.0a |
1.0 ± 0.3a |
0.8 ± 0.1a |
5.4 ± 3.7a |
1.5 ± 0.1d |
0.4 ± 0.3a |
cTd Control |
13.1 ± 0.0c |
4.4 ± 0.1cd |
3.7 ± 0.0bc |
0.3 ± 0.0b |
1.2 ± 0.1abc |
1.5 ± 0.0e |
9.8 ± 1.5bcd |
1.0 ± 0.1a |
0.8 ± 0.1b |
Utd UHPH |
12.5 ± 0.0ab |
4.2 ± 0.1abc |
3.6 ± 0.0b |
0.2 ± 0.0a |
1.3 ± 0.3bcd |
1.3 ± 0.1bc |
8.5 ± 2.4abc |
1.4 ± 0.2cd |
1.0 ± 0.2bcd |
cHv Control |
12.8 ± 0.0bc |
4.6 ± 0.2d |
3.7 ± 0.0bc |
0.4 ± 0.0c |
1.7 ± 0.1e |
1.3 ± 0.1cd |
9.8 ± 1.7bcd |
1.2 ± 0.1b |
1.1 ± 0.1d |
UHv UHPH |
12.1 ± 0.4a |
4.0 ± 0.1a |
3.7 ± 0.0b |
0.4 ± 0.0c |
1.4 ± 0.1cd |
1.2 ± 0.1bc |
7.5 ± 2.6ab |
1.3 ± 0.2bc |
1.0 ± 0.1bcd |
cM29 Control |
13.1 ± 0.1c |
4.5 ± 0.2cd |
3.7 ± 0.0bc |
0.3 ± 0.0b |
1.2 ± 0.1abcd |
1.4 ± 0.1de |
12.1 ± 0.2cd |
0.9 ± 0.1a |
0.8 ± 0.2bc |
UM29 UHPH |
12.1 ± 0.6a |
4.3 ± 0.1bc |
3.7 ± 0.0c |
0.5 ± 0.0d |
1.3 ± 0.1bcd |
1.3 ± 0.1bc |
13.7 ± 3.5d |
1.3 ± 0.1bc |
1.1 ± 0.1cd |
3.1.4. Fermentative volatiles by GC-FID.
UHPH fermentation had less higher alcohols than controls, on average 374.23 mg L−1 in controls and 260.53 in UHPH (data obtained from Table 3), which means a 30% lower content. Similarly, the UHPH fermentation showed lower contents of esters than controls, on average 36.79 mg L−1 for control fermentation and 26.99 mg L−1 for UHPH and this difference is 27% of reduction. The carbonyl compounds acetoin and diacetyl showed similar concentrations in control and UHPH wines, with a difference of just 3% on average (Table 3). However, the contents of acetaldehyde were much higher in controls than in UHPH fermentation (2–4×) except for Sc.
Table 3 Fermentative volatiles in control and UHPH must fermented by Saccharomyces cerevisiae (Sc7VA), Lachancea thermotolerans (L31), Torulaspora delbrueckii (Td), Hanseniaspora vineae (Hv), and Metschnikowia pulcherrima (M29). Compounds analyzed by GC-FID, and values are means ± sd (fermentation in triplicate) in mg L−1. Different letters mean significant differences (p < 0.05)
|
Sc7VA |
USc7VA |
L31 |
UL31 |
Td |
UTd |
Hv |
UHv |
M29 |
UM29 |
Control |
UHPH |
Control |
UHPH |
Control |
UHPH |
Control |
UHPH |
Control |
UHPH |
Acetaldehyde |
101.90 ± 2.43c |
102.88 ± 7.23c |
113.14 ± 8.85cd |
29.59 ± 2.27ab |
226.25 ± 23.02f |
46.88 ± 4.59b |
97.84 ± 9.43c |
26.50 ± 5.98a |
182.49 ± 18.25e |
124.47 ± 3.52d |
Methanol |
43.49 ± 6.21d |
30.66 ± 3.62bc |
29.29 ± 3.29abc |
23.35 ± 0.66a |
61.06 ± 6.73e |
25.22 ± 4.14ab |
56.79 ± 3.33e |
31.16 ± 1.87bc |
44.83 ± 1.82d |
35.49 ± 5.16c |
1-Propanol |
24.76 ± 1.75a |
33.01 ± 1.08b |
50.43 ± 1.82d |
50.66 ± 5.34d |
30.30 ± 2.71b |
62.16 ± 2.94e |
32.06 ± 1.38b |
42.08 ± 2.10c |
29.36 ± 1.40b |
45.45 ± 3.49c |
Diacetyl |
0.00 ± 0.00a |
0.00 ± 0.00a |
0.00 ± 0.00a |
1.41 ± 0.10b |
1.71 ± 0.16b |
1.64 ± 0.14b |
0.00 ± 0.00a |
0.00 ± 0.00a |
2.67 ± 0.51c |
0.00 ± 0.00a |
Ethyl acetate |
18.02 ± 1.15a |
19.06 ± 2.04a |
37.19 ± 1.90bc |
32.50 ± 0.69bc |
40.86 ± 0.22cd |
30.86 ± 1.87b |
49.67 ± 9.55d |
85.39 ± 13.49e |
35.60 ± 1.99bc |
48.41 ± 5.86d |
2-Butanol |
0.00 ± 0.00a |
0.00 ± 0.00a |
0.00 ± 0.00a |
0.00 ± 0.00a |
2.89 ± 0.35b |
0.00 ± 0.00a |
0.00 ± 0.00a |
0.87 ± 1.50a |
3.36 ± 0.71b |
0.00 ± 0.00a |
Iso-butanol |
26.42 ± 1.23bc |
22.53 ± 2.25ab |
51.22 ± 1.38f |
28.14 ± 1.94c |
49.45 ± 4.24f |
34.16 ± 1.90d |
40.11 ± 1.87e |
20.07 ± 0.81a |
65.41 ± 6.93g |
47.22 ± 2.75f |
1-Butanol |
3.90 ± 0.05b |
0.00 ± 0.00a |
4.68 ± 0.17bcd |
5.58 ± 1.40d |
0.00 ± 0.00a |
5.13 ± 0.22cd |
0.00 ± 0.00a |
5.55 ± 0.80d |
5.53 ± 0.69d |
4.19 ± 0.18bc |
Acetoin |
11.18 ± 1.31b |
11.26 ± 0.82b |
10.61 ± 0.42ab |
10.81 ± 0.99ab |
8.91 ± 1.19a |
11.21 ± 0.70b |
11.25 ± 0.61b |
11.74 ± 0.31b |
12.27 ± 1.43b |
12.00 ± 2.51b |
2-Methyl-1-butanol |
58.62 ± 2.89de |
37.20 ± 4.13a |
82.81 ± 5.49g |
55.15 ± 2.34cd |
64.24 ± 6.84ef |
62.10 ± 1.65def |
66.89 ± 4.69f |
44.01 ± 0.54ab |
68.88 ± 7.62f |
48.23 ± 0.96bc |
3-Methy-1-butanol |
141.50 ± 6.16c |
78.25 ± 6.27a |
187.56 ± 4.47e |
109.60 ± 1.99b |
163.37 ± 8.32d |
133.54 ± 6.30c |
144.13 ± 9.97c |
81.48 ± 1.72a |
176.88 ± 17.89de |
91.39 ± 4.96a |
Isobutyl acetate |
0.00 ± 0.00a |
0.00 ± 0.00a |
0.00 ± 0.00a |
3.10 ± 0.26b |
10.55 ± 0.26d |
0.00 ± 0.00a |
3.54 ± 1.14b |
3.27 ± 0.41b |
4.83 ± 0.86c |
3.63 ± 0.40b |
Ethyl butyrate |
3.44 ± 0.53b |
4.68 ± 0.31c |
2.95 ± 0.19b |
0.00 ± 0.00a |
4.60 ± 0.82c |
4.31 ± 0.67c |
0.00 ± 0.00a |
0.00 ± 0.00a |
4.83 ± 0.40c |
0.00 ± 0.00a |
Ethyl lactate |
15.17 ± 1.20c |
14.52 ± 0.84c |
60.60 ± 2.78e |
26.37 ± 2.92d |
10.00 ± 0.68b |
14.83 ± 0.98c |
5.53 ± 0.86a |
15.69 ± 4.91c |
12.28 ± 2.91bc |
9.32 ± 1.58ab |
2–3 butanediol |
484.44 ± 19.15bc |
565.98 ± 52.62cde |
362.72 ± 25.92a |
444.27 ± 34.85 ab |
370.25 ± 29.25a |
673.93 ± 29.92f |
512.73 ± 65.93bcd |
593.12 ± 25.27def |
435.27 ± 60.09 ab |
643.34 ± 86.96ef |
Isoamyl acetate |
2.42 ± 0.67b |
0.00 ± 0.00a |
2.14 ± 0.30b |
0.00 ± 0.00a |
2.31 ± 0.34b |
0.00 ± 0.00a |
2.37 ± 0.12b |
1.90 ± 0.06b |
4.72 ± 1.06c |
2.05 ± 0.29b |
Hexanol |
0.00 ± 0.00a |
0.00 ± 0.00a |
3.84 ± 0.12c |
3.62 ± 0.12b |
3.87 ± 0.15c |
3.88 ± 0.06c |
0.00 ± 0.00a |
3.64 ± 0.15b |
0.00 ± 0.00a |
0.00 ± 0.00a |
2-Phenylethanol |
47.18 ± 1.31d |
23.81 ± 1.93a |
73.39 ± 9.42e |
30.37 ± 2.88 ab |
69.35 ± 3.08e |
42.77 ± 6.10cd |
38.60 ± 4.46bcd |
23.44 ± 1.02a |
67.82 ± 8.16e |
34.52 ± 4.30bc |
2-Phenylethyl acetate |
5.38 ± 0.05a |
5.36 ± 0.12a |
5.51 ± 0.10a |
5.77 ± 0.03a |
7.70 ± 0.46b |
7.34 ± 0.64b |
5.43 ± 0.20a |
5.56 ± 0.34a |
7.67 ± 0.52b |
7.23 ± 0.25b |
Higher alcohols
|
302.37 ± 12.97bc
|
194.79 ± 13.06a
|
450.09 ± 17.99g
|
279.50 ± 13.31b
|
379.61 ± 18.46e
|
339.86 ± 17.72d
|
321.81 ± 19.68bc
|
217.51 ± 4.31a
|
417.25 ± 39.37f
|
271.01 ± 9.22b
|
Carbonyl compounds
|
11.18 ± 1.31ab
|
11.26 ± 0.82ab
|
10.61 ± 0.42a
|
12.22 ± 1.05ab
|
10.62 ± 1.04a
|
12.86 ± 0.83bc
|
11.25 ± 0.61ab
|
11.74 ± 0.31ab
|
14.94 ± 1.86c
|
12.00 ± 2.51ab
|
Esters
|
44.44 ± 0.33a
|
43.63 ± 2.65a
|
108.38 ± 0.65e
|
67.74 ± 3.33cd
|
76.02 ± 1.63d
|
57.34 ± 1.16b
|
66.54 ± 9.59bc
|
111.80 ± 11.66e
|
69.94 ± 5.52cd
|
70.63 ± 4.82cd
|
Floral and fruity esters
|
26.42 ± 0.90b
|
24.57 ± 1.06b
|
71.19 ± 2.53d
|
35.25 ± 3.17c
|
35.16 ± 1.86c
|
26.48 ± 1.24b
|
16.87 ± 1.42a
|
26.42 ± 5.41b
|
34.33 ± 3.82c
|
22.22 ± 1.82b
|
3.1.5. Other volatile compounds by HS-SPME-GC-MS.
The use of a PCA biplot with the aroma compounds analyzed by HS-SPME-GC-MS allows a clear separation of the wines produced from must processed by UHPH (Fig. 3, red cluster) from the unprocessed controls (Fig. 3, black cluster). In general, higher concentrations of several esters are formed in unprocessed controls (Table S1†). UHPH can be a useful tool to produce cleaner wines, in which the variety can be less hidden by the fermentation biotechnology. Additionally, β-damascenone, decanoic acid and 2-ethyl-1-hexanol clearly differentiate the composition of UHPH wines when fermented by H. vineae (Fig. 3, red cluster, Table S1†). Moreover, 2-ethyl-1-hexanol shows similar concentrations in both UHPH and controls when fermented by S. cerevisiae; however, higher concentrations were found in UHPH wines when they were fermented by whatever non-Saccharomyces (Table S1†).
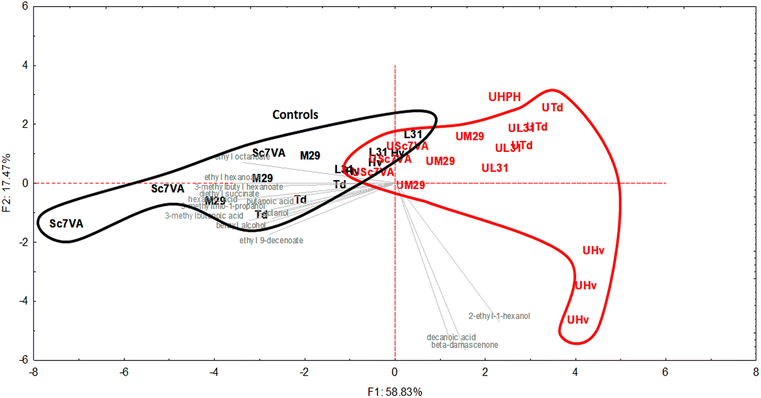 |
| Fig. 3 PCA biplot of values and means in controls and UHPH (U) must fermented by Saccharomyces cerevisiae (Sc7VA), Lachancea thermotolerans (L31), Torulaspora delbrueckii (Td), Hanseniaspora vineae (Hv), and Metschnikowia pulcherrima (M29). Compounds analyzed by HS-SPME-GC-MS. | |
3.1.6. Thiols by GC-MS.
The content of 3MH for Lt L31 fermentation samples has values higher than 400 ng L−1 in all the fermentation processes and on average close to 600 ng L−1 in control fermentation and higher than 800 ng L−1 in UHPH fermentation by Lt (Fig. 4). In general, for all UHPH juices the contents of 3MH were on average higher than in controls except for Hv, although with significant differences just for Td.
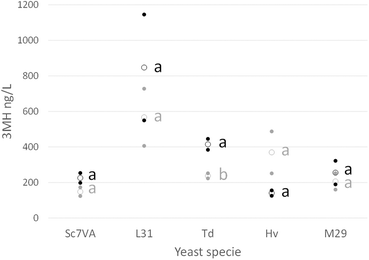 |
| Fig. 4 Content of 3-mercaptohexanol (3 MH) (ng L−1) in the fermentation of several yeast strains: Saccharomyces cerevisiae 7VA (Sc7VA), Lachancea thermotolerans L31 (L31), Torulaspora delbrueckii (Td), Hanseniaspora vineae (Hv), and Metschnikowia pulcherrima (M29). Black dots show fermentation of UHPH processed grape juice (black circles are the average value) and grey dots show fermentation of control unprocessed juice (grey circles are the average value). Fermentation was performed in duplicate. Different letters mean significant differences (p < 0.05). | |
Other thiols such as 4MMP and 4MMPOH were not detected in most of the samples and concerning the 3MHA the concentrations were quite variable and did not follow a clear pattern.
3.1.7. Color.
As per the color analyses results, the values of chroma, which refers to saturation, are consistently higher for wines produced with untreated must; nonetheless, only the fermentation samples produced with Sc, Td, and Hv are significantly different. The average values obtained for these parameters are between 10.4% higher, for the least different (Lt), and 36.7% higher for Sc (see Table 4).
Table 4 Color parameters chroma and hue for all wines produced from untreated and treated Verdejo must. Values are means with standard deviations for n = 3. Values for each pair of yeast species that share the same letter do not differ significantly (p < 0.05)
Wine |
Chroma |
Hue (°) |
Sc7VA |
14.4 ± 2.7a |
91.1 ± 4.3a |
USc7VA |
9.7 ± 1.2b |
95.5 ± 6.9a |
L31 |
11.4 ± 0.2a |
97.8 ± 3.3a |
UL31 |
10.2 ± 1.1a |
98.0 ± 4.0a |
Td |
12.6 ± 1.4a |
97.4 ± 2.1a |
UTd |
9.9 ± 0.6b |
97.6 ± 5.8a |
Hv |
11.4 ± 1.0a |
93.4 ± 4.0a |
UHv |
9.3 ± 1.0a |
98.0 ± 2.6a |
M29 |
12.5 ± 0.4a |
95.5 ± 0.7a |
UM29 |
9.3 ± 2.6b |
94.4 ± 0.3b |
With regards to the hue (Table 4), this parameter had no significant differences between pairs of must, and the media values were slightly higher for the UHPH treated must fermented with Sc and Hv. The results observed for this parameter are consistent with the observations got for the a* axis and b* axis shown in Fig. 5, where Sc is completely isolated from the rest of the wines having the highest b* values (more yellow) and the lowest a* values (less green).
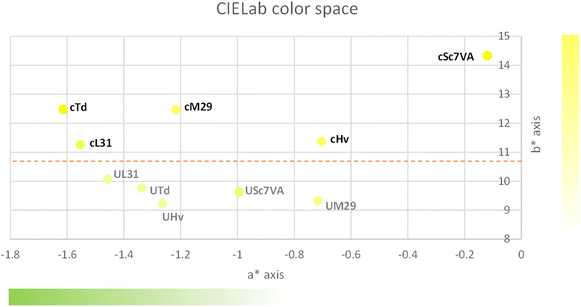 |
| Fig. 5 CIELab parameters for control (c) and UHPH (U) must fermented by Saccharomyces cerevisiae (Sc7VA), Lachancea thermotolerans (L31), Torulaspora delbrueckii (Td), Hanseniaspora vineae (Hv), and Metschnikowia pulcherrima (M29). Color of wines expressed as the contribution of the a* axis and b* axis from the CIELab color space coordinates. Negative values of the a* axis correspond to green, and positive values of the b* axis correspond to yellow. | |
As observed in Fig. 5, the values for wines produced with must without UHPH treatment were larger on the b* axis, above the dotted line and above 11 units, which is translated into wines having more yellow appearance associated with higher levels of oxidation. On the other hand, all wines from UHPH-treated must and the wines from untreated must fermented by non-Saccharomyces yeasts had a* values larger than −0.6 in comparison to Sc. In this case, all wines except for Sc had a larger contribution of green.
3.2. Discussion
3.2.1. Micro- and macroscopic effects of UHPH.
The beginning of browning in the must poured into the beaker just after the settling is a typical effect of oxidation by the grape polyphenol oxidase enzymes (PPOs) working on juice o-diphenols; the process is very fast and clearly observable under exposition to air in the absence of reducing agents such as SO2. This slightly higher hue can be observed in the control beaker (Fig. 1A) but not in the UHPH processed must (Fig. 1B), thanks to the effective inactivation of PPOs by UHPH as it has been previously reported.5,7,11,30 Concerning the microscopic aspect, the thin regular structure in the sediment of the UHPH juice is due to the intense and regular nanofragmentation produced by the mechanical effects on colloidal particles and microorganisms in the UHPH valve.5,11 This microscopic observation agrees with the size range of 235–744 nm previously measured by AFM in grape juice.7
3.2.2. Yeast counts.
The resistance of yeasts to UHPH is not very high and inactivation levels of 6
log have been observed for Saccharomyces and non-Saccharomyces in grape juice.11 The absence of yeasts in the must after the 6th day of fermentation guarantees a better expression and prevalence of the inoculated species during the fermentation. We have observed the absence of wild yeasts in several trials during full fermentation,5,7 and it is possible to maintain unfermented musts for months or even years when sterilized by UHPH.
We have observed a slightly lower fermentation speed in UHPH processed must inoculated with the different species than in controls [data not shown]. This can be due to the lower loads of wild yeasts or the lower availability of thiamine or some nitrogen compounds that can be nano-encapsulated and less available, but more research is necessary to support these tentative explanations.
3.2.3. Lactic acid and enological parameters.
Recently the use of acidifying yeasts to reduce pH is a hot topic in the wine industry to improve the freshness and long-term stability of wines.17 Lt is the most important species from this point of view, due to its ability to reduce significantly wine pH by producing lactic acid from sugars.23–25 It also provides advantages for the consequent increase of molecular SO2 obtained at lower pH, also allowing total SO2 reduction with the same stability influencing the production of off-flavors31 and having a bioprotective effect.32 Although the implantation of Lt has been good in controls and UHPH processed must, many times at the industrial level acidification fails because of a low implantation of the yeast. Wild yeast elimination by UHPH is a guarantee for good implantation for Lt starters promoting good acidification even in juices with high loads of competitive wild yeasts.
3.2.4. Volatile compounds by GC-FID and SPME-GC-MS.
The lower contents of higher alcohols in UHPH wines (30% less, Table 3) have a positive impact on wine aroma because they give a winey and weighty sensory perception,33,34 which reduces the freshness and gives the impression of a flat and simple wine. The reduction of higher alcohols in the fermentation of UHPH wines has been already reported in white wines.11 Additionally, a low content of fermentative esters is also positive in a variety such as Verdejo with a clear thiol profile,35 in which excessive fermentative esters can mask this sensory quality. Previously, high production of esters has been observed in must processed by UHPH;11 however that wine was processed without settling and had more precursors after the nanofragmentation of the colloidal particles by UHPH. The degree of settling may be one way to modulate ester production during fermentation of UHPH-processed juices as indeed is nitrogen nutrition during fermentation.36,37 No significant differences have been found in the concentration of 2-phenylethyl acetate, an ester with a clear floral impact on wine (Table 3).
Concerning carbonyl compounds, the excessive amount of acetoin and diacetyl can be correlated with excessive dairy notes in wines, but in this case, both compounds were at a moderate amount and without significant differences between controls and UHPH wines (3%, Table 3). Finally, the low content of acetaldehyde in UHPH wines is positive, because of the impact of such a compound on the development of flat and oxidized aromatic characters.38 Indeed, in high amounts, this compound can confer to the wine nutty aromas, which turn to green/grassy or apple-like off-flavors at even higher concentrations; in contrast, if the levels are low, it can be connected with fruity notes.38 The lower acetaldehyde content in UHPH wines may be reasonably explained due to a higher presence of wild non-Saccharomyces yeasts in controls. However, it is also reported that acetaldehyde formation during alcoholic fermentation can be negatively affected by a deficiency of thiamine, because this vitamin is a cofactor of pyruvate decarboxylase.39 When heated in water at 140 °C under pressure, thiamine may be degraded40 and, due to the in-valve temperature reached during the experiment, UHPH processing might have reduced its natural content in the grape juice. The low concentrations of acetaldehyde in UHPH wines can be complementary to the suppressive effect of thiols in the oxidative profile produced by this molecule in wines.41
The same trend highlighted by GC-FID analysis for fermentative volatile compounds was also found for the other VOCs analyzed by SPME-GC-MS (although not always in a statistically significant way); indeed many of such compounds also have a fermentative origin. The slight decrease of different fatty acids in UHPH wines may also have a positive role on wine freshness, because of the consequent reduction of their typical pungent notes. Finally, limited to the samples fermented with H. vineae, the lower concentration of β-damascenone found in the samples processed by UHPH is an interesting feature, because this compound can modify the sensory perception of some esters. Indeed, some researchers observed that the addition of low levels of β-damascenone (0.85 μg L−1) to a model solution containing esters increased the fruity notes of the mixture, while when the concentration was higher (3.5 μg L−1), it determined the development of intense notes of raisins and dried plums.42
3.2.5. Thiols by GC-MS.
Thiols are the main aroma compounds in the sensory profile of the Verdejo variety with herbal like box tree nuances. The highly intense effect of Lt L31 in the formation of 3 MH is very significant with average values of 567–847 ng L−1 compared with the other species 140–415 ng L−1. This fact agrees with the positive effect of Lt in the release of thiols.43 It is also noticeable that most of the fermentation samples of the UHPH must had higher contents of 3 MH than the control must even when just the differences were significant in Td probably because of the high dispersion among replicates (Fig. 4). The non-detection or variable behavior in other thiols such as 4MMP, 4MMPOH, A3MH and benzene methanethiol indicates a low influence in the general profile of these wines.
3.2.6. Color.
Regarding the color of the wines, two parameters reflect the differences observed between wines from untreated must and those from UHPH-treated must. These two parameters are chroma and the b* axis. In this way, the only outstanding value was observed for untreated Sc wine with the largest difference in chroma from its treated counterpart, and the highest yellow fraction from the b* axis. The higher the value on the b* axis, the higher the expression of the yellow fraction in wines. The yellow color of wines is associated with oxidation. This phenomenon was observed in wines from untreated must, and this is also related to the inhibition of PPO enzymatic activity in treated must as it has been shown that UHPH has the ability of inactivating these enzymes.5,11,13 The color of wines from treated must had a less yellow fraction as observed in the Vitis vinifera L. variety “Hondarribi zuri” inoculated with 7VA.11 It is also interesting to observe that all wines produced from treated must and the wines produced from untreated must, but fermented with non-Saccharomyces yeast, retain the green color potentially coming from the Verdejo variety. From this observation, the species Lachancea thermotolerans and Torulaspora delbrueckii preserve the green color to a larger extent. Biological acidification of wines may play an important role in avoiding oxidation in the first case as it has been described that Lt reduces the evolution of color in Airén wines with lower chroma and hue values.44
4. Conclusions
UHPH is a promising emerging non-thermal technology environmentally friendly with very low consumption of energy and water resources able to control microbial loads and oxidation in grape juices. The effect, when associated with the use of non-Saccharomyces yeasts, is an effective implantation of the yeast inocula and a positive metabolomic impact facilitating the preservation of varietal aroma from grapes and the improvement of the freshness if acidifying species such as L. thermotolerans are used. Some of the aroma effects of the association of UHPH and specific non-Saccharomyces species might occur only in Verdejo or maybe just in other thiolic varieties. Additionally, lower pH values together with the control of the full wild microbiome of grapes, and the inactivation of oxidative enzymes, facilitate the production of wines with very low levels of SO2. A new enology is possible with cleaner labels and a more stable sensory quality even with grapes from regions affected by global warming.
Author contributions
C. Escott (investigation and methodology), C. Vaquero (investigation and methodology), J. M. del Fresno (investigation, methodology, and writing – original draft), A. Topo (investigation), P. Comuzzo (methodology, supervision, data curation, writing – original draft, and writing – review & editing), C. Gonzalez (methodology, supervision, and writing – review & editing), and A. Morata (conceptualization, funding acquisition, methodology, project administration, supervision, writing – original draft, and writing – review & editing).
Conflicts of interest
There are no conflicts to declare.
Acknowledgements
This research was funded by MINISTERIO DE CIENCIA E INNOVACIÓN, project ENOINNOVAPRESS—PID2021-124250OB-I00 Ministerio de Ciencia e Innovación. This work was partially supported by the State Program to Promote Scientific-Technical Research and its Transfer of the Spanish Ministry of Science and Innovation through the MALTA CONSOLIDER TEAM Research Network (RED2022-134388-T).
References
- A. Zamora and B. Guamis, Food Eng. Rev., 2015, 7, 130–142, DOI:10.1007/s12393-014-9097-4.
- F. Patrignani and R. Lanciotti, Front. Microbiol., 2016, 7, 1132, DOI:10.3389/fmicb.2016.01132.
- P. Comuzzo and S. Calligaris, Beverages, 2019, 5, 56, DOI:10.3390/beverages5030056.
- A. Morata and B. Guamis, Front. Nutr., 2020, 7, 598286, DOI:10.3389/fnut.2020.598286.
- M. A. Bañuelos, I. Loira, B. Guamis, C. Escott, J. M. Del Fresno, I. Codina-Torrella, J. M. Quevedo, R. Gervilla, J. M. Rodríguez Chavarría, S. de Lamo, R. Ferrer-Gallego, R. Álvarez, C. González, J. A. Suárez-Lepe and A. Morata, Food Chem., 2020, 332, 127417, DOI:10.1016/j.foodchem.2020.127417.
- I. Codina-Torrella, J. J. Gallardo-Chacón, B. Juan, B. Guamis and A. J. Trujillo, Foods, 2023, 12, 683, DOI:10.3390/foods12040683.
- C. Vaquero, C. Escott, I. Loira, B. Guamis, J. M. del Fresno, J. M. Quevedo, R. Gervilla, S. de Lamo, R. Ferrer-Gallego, C. González, M. A. Bañuelos, J. A. Suárez-Lepe and A. Morata, Food Bioprocess Technol., 2022, 15, 620–634, DOI:10.1007/s11947-022-02766-8.
- Á. Suárez-Jacobo, C. E. Rüfer, R. Gervilla, B. Guamis, A. X. Roig-Sagués and J. Saldo, Food Chem., 2011, 127, 447–454, DOI:10.1016/j.foodchem.2010.12.152.
- V. Ferragut, M. Hernández-Herrero, M. T. Veciana-Nogués, M. Borras-Suarez, J. González-Linares, M. C. Vidal-Carou and B. Guamis, J. Sci. Food Agric., 2015, 95, 953–961, DOI:10.1002/jsfa.6769.
- G.-y. Arakawa and K.-j. Yokoi, J. Biosci. Bioeng., 2023, 136, 117–122, DOI:10.1016/j.jbiosc.2023.05.003.
- I. Loira, A. Morata, M. A. Bañuelos, A. Puig-Pujol, B. Guamis, C. González and J. A. Suárez-Lepe, Innovative Food Sci. Emerging Technol., 2018, 50, 50–56, DOI:10.1016/j.ifset.2018.10.005.
- J. Pereda, V. Ferragut, J. M. Quevedo, B. Guamis and A. J. Trujillo, Effects of Ultra-High Pressure Homogenization on microbial and physicochemical shelf life of milk, J. Dairy Sci., 2007, 90, 1081–1093, DOI:10.3168/jds.S0022-0302(07)71595-3.
- R.-M. Velázquez-Estrada, M.-M. Hernández-Herrero, B. Guamis-López and A.-X. Roig-Saguès, Int. J. Food Sci. Technol., 2019, 54, 1858–1864, DOI:10.1111/ijfs.14089.
- M. Ciani and F. Comitini, Ann. Microbiol., 2011, 61, 25–32, DOI:10.1007/s13213-010-0069-5.
- N. P. Jolly, C. Varela and I. S. Pretorius, FEMS Yeast Res., 2014, 14, 215–237, DOI:10.1111/1567-1364.12111.
- B. Padilla, J. V. Gil and P. Manzanares, Front. Microbiol., 2016, 7, 411, DOI:10.3389/fmicb.2016.00411.
- A. Morata, C. Escott, M. A. Bañuelos, I. Loira, J. M. del Fresno, C. González and J. A. Suárez-Lepe, Biomolecules, 2020, 10, 34, DOI:10.3390/biom10010034.
- L. Roudil, P. Russo, C. Berbegal, W. Albertin, G. Spano and V. Capozzi, Recent Pat. Food, Nutr. Agric., 2020, 11(1), 27–39, DOI:10.2174/2212798410666190131103713.
- M. Ramírez and R. Velázquez, Fermentation, 2018, 4, 94, DOI:10.3390/fermentation4040094.
- S. Simonin, H. Alexandre, M. Nikolantonaki, C. Coelho and R. Tourdot-Maréchal, Food Res. Int., 2018, 107, 451–461, DOI:10.1016/j.foodres.2018.02.034.
- M. Sipiczki, Appl. Environ. Microbiol., 2006, 72, 6716–6724, DOI:10.1128/AEM.01275-06.
- A. Morata, I. Loira, C. Escott, J. M. del Fresno, M. A. Bañuelos and J. A. Suárez-Lepe, Fermentation, 2019, 5, 63, DOI:10.3390/fermentation5030063.
- M. Gobbi, F. Comitini, P. Domizio, C. Romani, L. Lencioni, I. Mannazzu and M. Ciani, Food Microbiol., 2013, 33, 271–281, DOI:10.1016/j.fm.2012.10.004.
- A. Hranilovic, M. Bely, I. Masneuf-Pomarede, V. Jiranek and W. Albertin, PLoS One, 2017, 12, e0184652, DOI:10.1371/journal.pone.0184652.
- A. Morata, I. Loira, W. Tesfaye, M. A. Bañuelos, C. González and J. A. Suárez Lepe, Fermentation, 2018, 4, 53, DOI:10.3390/fermentation4030053.
- F. Viana, C. Belloch, S. Vallés and P. Manzanares, Int. J. Food Microbiol., 2011, 151, 235–240, DOI:10.1016/j.ijfoodmicro.2011.09.005.
- V. Martin, M. J. Valera, K. Medina, E. Boido and F. Carrau, Fermentation, 2018, 4, 76, DOI:10.3390/fermentation4030076.
- L. Tat, P. Comuzzo, I. Stolfo and F. Battistutta, Food Chem., 2005, 93, 361–369 CrossRef CAS.
- P. Comuzzo, M. Marconi, G. Zanella and M. Querzè, Food Chem., 2018, 264, 16–23 CrossRef CAS PubMed.
- J. N. Sauceda-Gálvez, I. Codina-Torrella, M. Martinez-Garcia, M. M. Hernández-Herrero, R. Gervilla and A. X. Roig-Sagués, LWT, 2021, 136, 110286, DOI:10.1016/j.lwt.2020.110286.
- A. Morata, I. Loira, C. González and C. Escott, Molecules, 2021, 26, 4571, DOI:10.3390/molecules26154571.
- R. Escribano-Viana, L. González-Arenzana, P. Garijo, L. Fernández, R. López, P. Santamaría and A. R. Gutiérrez, Fermentation, 2022, 8, 337, DOI:10.3390/fermentation8070337.
- A. Rapp and G. Versini, Dev. Food Sci., 1995, 37, 1659–1694, DOI:10.1016/S0167-4501(06)80257-8.
- S. Saberi, M. Cliff and H. Van Vuuren, Food Res. Int., 2012, 48, 725–735, DOI:10.1016/j.foodres.2012.06.012.
- D. Elpa, E. Durán-Guerrero, R. Castro, R. Natera and C. G. Barroso, J. Sep. Sci., 2014, 37, 1867–1872, DOI:10.1002/jssc.201400308.
- F. M. Carrau, K. Medina, L. Fariña, E. Boido, P. A. Henschke and E. Dellacassa, FEMS Yeast Res., 2008, 8, 1196–1207, DOI:10.1111/j.1567-1364.2008.00412.x.
- F. Carrau, K. Medina, L. Fariña, E. Boido and E. Dellacassa, Int. J. Food Microbiol., 2010, 143, 81–85, DOI:10.1016/j.ijfoodmicro.2010.07.024.
- I. Arias-Pérez, M. P. Sáenz-Navajas, A. de-la-Fuente-Blanco, V. Ferreira and A. Escudero, Food Chem., 2021, 361, 130081 CrossRef PubMed.
-
M. Ugliano and P. A. Henschke, Yeasts and Wine Flavour, in Wine Chemistry and Biochemistry, ed. Moreno-Arribas M. V. and Polo M. C., Springer, 2009 Search PubMed.
-
F. J. Francis, Wiley Encyclopedia of Food Science and Technology, John Wiley & Sons, 2nd edn, 1999 Search PubMed.
- C. Coetzee, J. Brand, D. Jacobson and W. J. Du Toit, Aust. J. Grape Wine Res., 2016, 22, 197–204, DOI:10.1111/ajgw.12206.
- A. Escudero, E. Campo, L. Fariña, J. Cacho and V. Ferreira, J. Agric. Food Chem., 2007, 55, 4501–4510 CrossRef CAS PubMed.
- K. Zott, C. Thibon, M. Bely, A. Lonvaud-Funel and D. Dubourdieu, I. Masneuf-Pomarede, Int. J. Food Microbiol., 2011, 151, 210–215, DOI:10.1016/j.ijfoodmicro.2011.08.026.
- C. Escott, C. Vaquero, I. Loira, C. López, C. González and A. Morata, Foods, 2022, 11, 3734, DOI:10.3390/foods11223734.
Footnote |
† Electronic supplementary information (ESI) available. Discussion, limited experimental and spectral data, and crystallographic data. See DOI: https://doi.org/10.1039/d3fb00226h |
|
This journal is © The Royal Society of Chemistry 2024 |