Application of electrolysed water in post-harvest treatment of fruits and vegetables
Received
8th November 2023
, Accepted 20th December 2023
First published on 11th January 2024
Abstract
The increasing concerns of post-harvest losses in fruits and vegetables have triggered the interest of scientists across the globe to look for alternative methods for treatment of horticultural produce after harvest that facilitates inactivation of fungal and bacterial postharvest pathogens without causing any ill effects. Electrolysed water (also known as electrochemically activated water solution) is primarily composed of hypochlorous acid (HOCl) and is produced by passing electric current through a cell submerged in a saturated brine solution made up of high purity sodium chloride salt and deionised water. The solution thus collected at the anode terminal has strong oxidizing properties that have proven its use as a broad-spectrum sanitiser capable of inactivating a wide range of bacteria, viruses, yeast and molds. The ease of production makes electrolysed water a viable on-site generation option for industries that require a huge amount of the solution, thus reducing the strain on the supply chain logistics. The efficacy of the solution is determined by its temperature of use and the exposure time in addition to the active chlorine concentration (ACC), thus offering flexibility in the type of treatment required for different commodities. This chapter highlights the mechanism of action of electrolysed water against pathogenic microbes, its application on different fruits and vegetables post-harvest, its influence on the organoleptic properties of the product and global regulations around its use on fresh produce.
Sustainability spotlight
Post-harvest treatment of fruits and vegetables is imperative to ensure economic sustainability and prevent occurrences of food borne illnesses. The existing techniques and chemicals in the industry do not only pose complex environmental, health and safety risks but also increase challenges in terms of sustainability. There is a dire need to explore environmentally and economically sustainable options that not only have a good efficacy against eliminating microbial contamination but also minimise the risk to the environment and the health of people. Hypochlorous acid (HOCl) is one such potent alternative that is being explored as a post-harvest treatment option for fruit and vegetables due to its potent antimicrobial activity. The on-site generation option for such solution eliminates the use of unnecessary plastic packaging and is in line with the United Nations Sustainable Development Goals (SDG 3, 6, 7, 9, 11, and 13).
|
1. Introduction
Fruits and vegetables constitute a major part of human diet, primarily because they are a good source of several bioactive components including water soluble vitamins, polyphenolic compounds, and dietary fibre. Their marketable life is influenced by their storage conditions such as temperature, relative humidity (RH), air composition and the degree of microbial load on the surface1 that affects properties such as moisture loss, microbial degradation, and subsequent loss of nutritional value.2,3 Of these, microbiological infestation is of critical importance as it is directly linked to the occurrence of food borne illnesses.4 It has been reported that there are approximately 31 known food borne pathogens including Campylobacter spp., Bacillus spp., Brucella spp., and E. coli among others that are known to infect ∼50 million people resulting in more than 128
000 hospitalization cases and several deaths per annum.5 In addition, more than half of these illnesses can be traced back to their origin from fresh produce including fruits, vegetables, and nuts. Thus, increased attention has been placed on optimising the safety of fresh produce for human consumption by ensuring inactivation of spoilage causing pathogens to reduce post-harvest losses as well as their potency as a biological hazard. In addition, concerns have also arisen to tackle the losses that occur due to physiological processes of fresh produce by controlling the respiration and transpiration rate.6
Currently, the main methods employed to ensure the quality and safety of fresh produce are physical treatments like irradiation, magnetic fields, controlled atmosphere storage, edible coatings and cold storage7–9 and chemical treatments like washing (sodium carbonate, chlorinated water, ozonated water, peroxide, etc.), and use of elemental sulphur (sulphur dioxide).10–13 However, the declining effectiveness of the above methods, high cost and adverse impact on the nutritional quality along with their limited pragmatic applications have encouraged the need for a more economical and sustainable alternative to reduce the spoilage in fruits and vegetables after harvest.
Electrolysed water has evolved as one of the safe alternatives for sanitisation in the food industry due to its low cost and convenient application in addition to environmental friendliness.14 Most studies have seen the focus of electrolysed water to demonstrate antimicrobial efficacy in meat and meat products and recent advances have demonstrated the ability of electrolysed water to inactivate pathogens in post-harvest fruits and vegetables along with improving their sensorial attributes.15–17
2. Principle of electro-chemical activation technology
The first occurrence of electrolysed water was centuries ago in Russia with the primary purpose of purifying/decontaminating water for use in medical devices. However, it has since been used by Japan, Korea, USA and other parts of the world in several other applications including food industry, agriculture, livestock management and clinical applications.14,15,18,19 With recent development in science, industries have attempted to improve the electrochemical activation process, and as a result it has gained more attraction as a promising nonthermal technology, particularly for the food industry.
The term ‘electrolysed water’ is a broad term used to define the output generated by the passage of electric current through an electrolytic cell (containing anode and cathode terminals separated by a membrane) immersed in a salt-water solution. A schematic representation of an electrolytic cell used for the generation of electrolysed water is shown in Fig. 1.
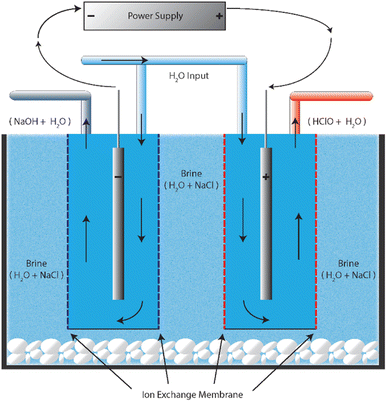 |
| Fig. 1 A schematic representation of the process of electrochemical activation of salt water. | |
Since sodium chloride is a strong electrolyte, the supply of direct current voltages results in the dissociation of salt and water into ions such as Na+, Cl−, H+ and OH− respectively. The negatively charged ions (Cl− and OH−) move towards the anode terminal of the cell to give up electrons resulting in the formation of products like chlorine gas (Cl2), hypochlorous acid (HOCl), hypochlorite ion (OCl−) and hydrochloric acid (HCl), while the positively charged ions (Na+ and H+) move to the cathode to accept electrons and form hydrogen gas and sodium hydroxide,20 thus leading to the generation of two kinds of water simultaneously, and the water at the anode has strong oxidising properties (pH < 7) with an oxidation–reduction potential (ORP) of ∼850–1100 mV required to cause microbial inactivation while the solution at the cathode terminal has strong reducing properties (pH > 7) capable of performing cleaning action. A typical process reaction can be illustrated by the following equations:
At the anode:
2NaCl → Cl2↑ + 2e− + 2Na+ |
At the cathode:
2NaCl + 2OH− → 2NaOH + Cl− |
There is a significant influence of factors like amperage, voltage, and flow rate on the final output from the machine. For instance, the efficiency of the cell is significantly reduced with increase in the water flow rate and the salt concentration in the feed solution.21 Hsu20 also demonstrated that the free chlorine concentration in the output solutions increases with the increase in the salt concentration and reduced flow rate of water. Rahman et al.22 showed that a higher input current resulted in the output solution with high pH, active chlorine concentration and thus a strong antimicrobial activity.
Based on the operating parameters, there are different types of electrolysed water that can be generated at the anode terminal and depending on their pH are called – acidic, slightly acidic, and neutral. Acidic electrolysed water is produced within the pH range of 2–3, slightly acidic electrolysed water has pH within the range of 5–6.5 and neutral electrolysed water pH is between 6.5–7.5.23 The pH of the electrolysed water determines the dominant “active chlorine species” that is available to cause the antimicrobial effect, which subsequently affects the end use of the solution. The different forms of chlorine i.e., Cl2, HOCl and OCl− relative to the pH are shown in Fig. 2. For instance, in slightly acidic electrolysed water, almost all of chlorine is present as HOCl and as the pH increases beyond neutral, OCl− becomes the dominating form of chlorine in the solution. Each chlorine form has its own efficacy and hence, can influence the end-use of the generated solution. For instance, HOCl is 80 times more effective as a sanitizing agent than the hypochlorite ion (OCl−) at an equivalent concentration24 and has been shown to have strong bactericidal effects on many pathogenic bacteria such as E. coli, Listeria monocytogenes, Staphylococcus aureus and Salmonella enterica. HOCl can exhibit strong oxidising power because of its ability to produce hydroxyl radicals after penetrating the cell membranes that simultaneously impact the metabolic processes within a microbial cell25 (discussed in Section 3), thus making slightly acidic electrolysed water a good alternative for applications in the food industry. Having said that, it has also been reported that the other forms of electrolysed water can also generate the antimicrobial effect and have been used for disinfection of fruits and vegetables during processing or after harvest provided it has a sufficiently high available chlorine concentration (ACC).26
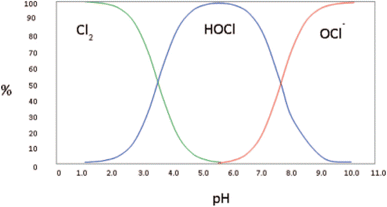 |
| Fig. 2 Relationship between the dominant form of chlorine and the pH of the solution (source: D. Gombas, et al., J. Food Prot., 2017, 80, 2, 312–330). | |
3. Electrolysed water generators and types of electrolysed water
Electrolysed water generators can be categorised on the basis of the cell technology and automation control systems, which ensures the quality of the final product output. For instance, the presence of dual ion exchange membranes in the cell is a patented feature of the PathoSans systems manufactured by Spraying Systems Co. The ion exchange membranes prevent the leaching of the unionized salt into either of the two solutions produced at the cathode and anode parts of the cell, thus ensuring the purity of the solutions. In some systems, there are no or a single ion exchange membrane in place such that either one or both generated solutions contain salt in the final output. Based on automation, there are generators that allow the user to select the brine flow rate while the machines adjust the current accordingly (Amano companies), while the other types, like Hoshizaki models, allow the user to select amperage and voltage while the machine changes the brine flow accordingly.27 In addition, there are generators made by Toyo and Nippon Intek companies that allow the users to pre-feed the desired chlorine concentration in the output while the machine adjusts the current and the flow rate accordingly.
The selection of electrolysed water generators is crucial to the intended use of the final solutions. For instance, there are two kinds of generators – one is used to generate acidic/slightly acidic and alkaline electrolysed water that can be collected in two different collection tanks whereas the other is used to generate a neutral electrolysed water.28 The difference between these two generate is the presence of two chambers in the former that allows generation of two chemically different solutions on either side of the electrode, whereas the latter is a single-chamber generator producing only one solution. Generally, studies use a commercial electrolysed water generator where the information about system fabrication such as electrode type, electrode gap, electrical current or voltage supply among others is only selectively available.
4. Mechanism of antimicrobial activity of electrolysed water
Over the past decade, electrolysed water has gained tremendous importance as a means to achieve sanitation and disinfection of hard surfaces. The antimicrobial mechanism to date has not been fully understood; however attempts have been made to conceptualize its mode of action based on factors such as pH, ORP and the form and concentration of available chlorine.14 The possible mechanism of action of HOCl and OCl− which can be present in electrolysed water, depending on the pH of its production, is illustrated in Fig. 3. It has been identified that the main reason for the antimicrobial efficacy of electrolysed water is the penetration properties of HOCl and OCl−. The penetration power of OCl− through the microbial cell is limited because of the existence of the hydrophobic lipid bilayer and some protective cell wall structures, and the negatively charged nature of the bacterial cell membrane. Due to the innate repulsion offered by the like charges, OCl− can only weakly oxidise the surface of the bacterial cell wall. On the other hand, the neutral HOCl molecule can penetrate the cell wall of the micro-organism relatively easily, thus increasing its efficacy as a disinfectant.14 Thus, pH is a critical factor for the disinfection efficacy of a solution.
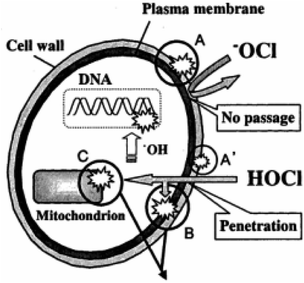 |
| Fig. 3 Difference in the mechanism of action of different chlorine species HOCl and OCl− on a microbial cell (source: https://www.ams.usda.gov/sites/default/files/media/Hypochlorous%20Acid%20Petition.pdf). | |
The growth rate of aerobic bacteria is maximal within the pH range of 4–9 and ORP of +200 to +800 mV, while anerobic bacteria can grow between −700 mV and +200 mV.29 When the electrolysed water falls within the acidic pH region of 2–3, it reduces the bacterial growth by making the bacterial cell hypersensitive to active chlorine by active modifications in its cell membrane;29 however regardless of bacterial membrane sensitisation, the presence of chlorine in one of its forms and the solution ORP are the main factors responsible for the bactericidal activity.29 For instance, high ORP levels in electrolysed water are associated with modification of metabolic fluxes and ATP production and as a result available chlorine can destroy the microbial membrane, cause denaturation of proteinaceous structures, inhibit oxygen utilisation coupled with leakage of some macromolecules, inhibit glucose oxidation by chlorine-oxidizing sulfhydryl groups, form toxic N-chlorine derivatives of cytosine, react with nucleic acids, purines, and pyrimidines, and unbalance the metabolism of key enzymes.29–31 In contrast, some other researchers have reported bacterial inactivation at ORP levels between 500 and 700 mV.22 So it is evident that ORP alone may not be the main factor for bacterial inactivation, especially since the performance of other anti-microbial agents like ozone is significantly lower than electrolysed water despite possessing high ORP.32 Thus, it can be efficiently concluded that the efficacy of electrolysed water in microbial inactivation is influenced by a combination of pH, ACC, ORP, storage conditions, temperature of water, and hardness of water among other factors.27
5. Micro-organisms causing post-harvest losses in fruits and vegetables
The agents associated with the post-harvest losses of some of the major fruits and vegetables are mostly bacterial or fungal in origin. It has been well-established that fungal losses are generally associated with species of Alternaria, Aspergillus, Botrytis, Colletotrichum, Penicillium and Rhizopus, while bacterial losses are confined to species like Erwinia and Pseudomonas. A summary of the diseases associated with some of the major fruits and vegetables grown around the world is shown in Table 1. Most of the listed organisms are classified as “weak pathogens” due to their inability to invade an intact produce. Post-harvest deterioration and subsequent losses are characterised by the physical damage exhibited by the fresh produce, which can be influenced by the injuries sustained during cultivation or harvest. For instance, fruits like peaches, plums and berries can be easily damaged by strong winds, insects, birds, rodents and farming techniques, but this injury may not be noticeable instantly. With the passage of time, bruising may become noticeable externally which may be amplified by overstocking the bulk produce in storehouses or due to the vibration damage that occurs in under-filled packs especially during long distance transportation, thus giving the micro-organisms a chance to proliferate and cause further decay in the quality of the produce during storage.
Table 1 Different diseases associated with selected fruits and vegetables post-harvest
Name of the fruit/vegetable |
Associated disease |
Causative organism |
References |
Apples |
Apple scab |
Venturia inaequalis
|
Dubey and Jalal;72 Wilson et al.73 |
Bitter rot |
Colletotrichum gloeosporioides
|
Apple blotch |
Marssonina coronaria
|
Blue mould |
Pseudomonas syringae, P. cepacia, Cryptococcus spp. |
Gray mould |
Pichia guilliermondii, P. cepacia, C. flavus, C. albidus, Acremonium breve |
Berries |
Black spot |
Colletotrichum acutatum
|
Paulus74 |
Powdery mildew |
Sphaerotheca macularis
|
Grey mould |
Botrytis cinerea
|
Crown rot |
Colletotrichum gloeosporioides and Colletotrichum fragariae |
Rhizopus rot |
Rhizopus nigricans
|
Anthracnose |
Colletotrichum fragariae and other spp. |
Red stele |
Phytophthora fragariae
|
Verticillium wilt |
Verticillium dahliae Kleb. |
Cherries, peaches and other stone fruit |
Bacterial canker |
Pseudomonas syringae pv syringae |
Pusey and Wilson;75 Nam et al.;76 Aysan et al.;77 Mohammadi and Aminifard78 |
Bacterial spot |
Xanthomonas arboricola pv pruni |
Brown rot |
Pseudomonas cepacia, P. fluorescens, B. thuringiensis |
Blossom blight |
Cladosporium cladosporioides and C. tenuissimum |
Crown gall |
Agrobacterium radiobacter var. tumefaciens |
Transit rot |
Agrobacterium tumefaciens
|
Grey mould |
Botrytis cinerea
|
Citrus fruits |
Alternaria rot |
Alternaria citri |
Eckert79 |
Brown rot |
Phytophthora spp. |
Blue mould |
Penicillium italicum |
Green mould |
Penicillum digitatum |
Sour rot |
Geotrichum candidum |
Potatoes |
Bacterial wilt |
Ralstonia solanacearum
|
Secor and Gudmestad80 |
Septoria leaf spot |
Septoria lycopersici
|
Late blight/early blight |
— |
Silver scurf |
Helminthosporium solani
|
Pink rot |
Phytophthora erythroseptica
|
Common scab |
Steptomyces scabies
|
Dry rot |
Fusarium sambucinum
|
Skin spot |
Polyscytalum pustuluns
|
Powdery scab |
Spongospora subterranea
|
Verticillium wilt |
Verticillium dahlia or Verticillium albo-atrum |
Rhizoctonia |
Rhizoctonia solani
|
Onions |
Basal rot |
Fusarium oxysporum
|
Kumar et al.81 |
Black mould |
Aspergillus niger
|
Blue mold rot |
Penicillium species
|
Botrytis Brown stain |
Botrytis cinerea
|
Botrytis leaf blight |
Botrytis squamosa
|
Downy mildew |
Peronospora destructor
|
Powdery mildew |
Leveillula taurica
|
Purple blotch |
Alternaria porri
|
White rot |
Sclerotium cepivorum
|
Yeast soft rot |
Kluyveromyces marxianus var. Marxianus |
Mushrooms |
Dry bubble |
Lecanicillium fungicola, var. fungicola and var. aleophilum |
Gea et al.82 |
Cob web |
Cladobotryum spp |
Wet bubble |
Mycogone perniciosa
|
Green mold |
Trichoderma spp. |
Tomatoes |
Gray mould |
Botrytis cinerea
|
Liu et al.;83 Srivastava et al.84 |
Blue mould |
Penicillium expansum
|
Alternaria rot |
Alternaria tenuis
|
Anthracnose |
Colletotrichum dematium
|
Cladosporium rot |
Cladosporium fulvum
|
Fusarium rot |
Fusarium roseum
|
Malustela rot |
Malustela aeria
|
Myrothecium rot |
Myrothecium roridum
|
Oospora rot |
Oospora lactis var. parasitica |
Phoma rot |
Phoma spp. |
Rhizopus rot |
Rhizopus nigricans
|
6. Post-harvest treatment of fruits and vegetables
It has been well documented that the post-harvest quality of fresh fruits and vegetables is influenced by their physiological processes such as respiration, maturing and senescence. The process of transpiration via the surface of fresh produce allows the water to escape into the ambient atmosphere, thus reducing the freshness in quality.33,34 Often, the quality is also influenced by the presence and the activity of pathogens that make the produce unconsumable and hence, contribute to the economic losses incurred by growers.35 Hence, critical measures are necessary to optimise the use of treatments that can alleviate these problems.
6.1 Disease control in fruits and vegetables using electrolysed water
Fungal infections are the major causes associated with the degradation of quality of fresh produce36 and the use of fungicides is greatly linked to the risks associated with residues in the fresh produce as well as the development of resistance by pathogens by repeated exposure.37 Therefore, electrolysed water is increasingly gaining acceptance as the method to control the expression of these diseases in fresh harvest. For instance, Khayankarn et al.38 studied the effect of electrolysed water (100–300 ppm) on the decay of pineapples during storage and reported the incidence of decay to be 33.3% lower than that of the control. It was suggested that the acidic pH of electrolysed water sensitises the outer cellular membrane of micro-organisms, thus allowing HOCl to enter the microbial cell more efficiently and subsequently oxidizing nucleic acids and proteins causing irreversible damage. In another study, Guentzel et al.39 reported that the use of electrolysed water with pH 6.3–6.5, ACC 250 ppm and ORP 800–900 mV could control 80% of the brown rot in peaches when stored at 25 °C for 16 days, while the efficacy in disease control was only 21% against Botrytis cinerea that causes gray mold in grapes. This could possibly indicate the varied susceptibility of different organisms to the effect of electrolysed water manufactured at pH > 6.2, where a majority of chlorine species in the disinfecting solution are expressed as OCl− (Fig. 2). Okull and Laborde40 demonstrated the efficacy of acidic electrolysed water (pH: 3; ACC: 80 ppm and ORP:1154 mV) against Pencillium expansum that causes decay in apples and made it ineffective for disease control during storage at 25 °C; however it was successful in avoiding cross-contamination of the affected produce with the healthy one. Other studies by Al-Haq et al.41 and Whangchai et al.42 demonstrated 50–90% effectiveness of acidic electrolysed water containing 100–250 ppm in controlling the diseases caused by Botryosphaeria berengeriana in pears and Penicillium digitatum in tangerines, respectively. In a nutshell, varied reductions in microbial growth have been observed in the fruits treated with electrolysed water and the difference in their response may be allocated to a variety of factors – primarily the physicochemical properties of electrolysed water, the exposure time, pathogen resistance and the mode of contact between the disinfectant and the sample.
Therefore, to increase the performance of electrolysed water, it may sometimes be coupled with other techniques to enhance the antimicrobial efficacy. For instance, Tango et al.43 studied the influence of different physical and chemical treatments in combination with slightly acidic electrolysed water against E. coli and Listeria monocytogenes in apples and tomatoes, respectively and reported that while significant reductions were observed by individual applications, a combination of fumaric acid, calcium oxide and ultrasonic treatment resulted in higher decontamination. A similar conclusion was also drawn by Ding et al.44 in their work on cherry tomatoes and strawberries; and Chen et al.45 on apple, mandarin, and tomato at the industrial scale. In another study by Koide et al.,46 mildly heated electrolysed water (pH: 5.5, ACC: 23 ppm) successfully caused >2.2 log reduction in total aerobic bacteria and >1.9 log reduction in molds and yeasts in comparison with tap water treatment.
6.2 Effect of electrolysed water on the physiology of fruits and vegetables
Respiration is a process of breakdown of complex carbohydrates into simple sugars and organic acids to produce energy that is utilised by living cells to maintain a series of anabolic processes that are essential to the integrity of the cell. Fruits and vegetables also undergo respiration after harvest and are broadly classified as climacteric fruits (that can undergo ripening after harvest, e.g. peaches, plums, cantaloupe, bananas, pears and tomatoes) and non-climacteric fruits (that do not ripen after harvest, e.g. berries, cherries, citrus fruits (lemons, limes, oranges, grapefruits, mandarins, and tangerines), cucumber, dates, eggplant, grapes, lychee, okra, peas, peppers, pineapple, pomegranates, strawberry, summer squash, tamarillo and watermelon). Respiration in fruits during post-harvest storage results in the decomposition of proteins and other substances, producing ethylene and carbon dioxide in the process leading to fruit ripening and senescence.47 Electrolysed water has shown variable effects on the respiration rates of fruits and vegetables. For instance, slightly acidic electrolysed water (pH: 5.9, ORP 904 mV) delayed the occurrence of climacteric peak, and hence the senescence, of peaches during 8 days of ripening at 25 °C,48 while similar effects were observed by Rico et al.49 for their application on lettuce. In contrast, no significant impact was observed on the quality of mizuna baby leaves on treatment with acidic and neutral electrolysed water indicating that HOCl may be a more effective form of chlorine to delay ripening in fruits and vegetables after harvest.50 It has also been proposed that pre-treatment with electrolysed water coupled with refrigerated conditions may reduce the respiration rate in shredded cabbages and broccoli.51,52 Guo et al.47 reported a delay in the ethylene production peak of “Hami” melon fruit treated with chlorine dioxide. In plants, ethylene production has been explained by a well defined pathway in which 1-aminocyclopropane-1-carboxylic acid (ACC) synthase (ACS) and ACC oxidase (ACO) catalyse the reactions from S-adenosylmethionine (SAM) to ACC and from ACC to ethylene, respectively. Controlling ethylene production (and thus, respiration) has been achieved in the past by different methods and based on the observations, it is quite plausible that electrolysed water (much like chlorine dioxide) may have the potential to reduce ethylene production by suppressing the expression of transcripts of ACS and ACO genes.
6.3 Effect of electrolysed water on the chemical composition of fruits and vegetables
Fruits and vegetables are a source of multiple nutrients like vitamins and minerals including phenolic compounds that have been associated with several health promoting benefits. Therefore, the study of the impact of electrolysed water treatment on their chemical composition is required. For instance, vitamin C is a known antioxidant that can scavenge free radicals, release oxidative stress and regulate the cancerous cell formation in the body;53 and 11% reductions in its content have been reported in sliced carrots washed with slightly acidic electrolysed water containing 23 ppm ACC at pH 5.5.46 Similarly, neutral electrolysed water at ACC 4.5 ppm decreased the vitamin C content in iceberg lettuce by 0.38 mg/100 g while at ACC 31.7 ppm it reduced the vitamin C content in white cabbage by 0.43 mg/100 g.54 In both these instances the results were not significantly different from tap water washed samples suggesting that electrolysed water does not have any adverse impact on the vitamin C content of the treated fruits and vegetables. Similar to vitamin C, β-carotene is also related with several therapeutic benefits and increases the attractiveness of food by imparting a bright yellow-orange colour.55 Koide et al.46 did not report any significant decreases in the β-carotene content of carrots treated with slightly acidic electrolysed water in comparison to tap water. This was also supported by Vandekinderen et al.54 with washing of iceberg lettuce and white cabbage with neutral electrolysed water. While reduction in vitamins by leaching is an expected scenario especially when it comes to washing, studies have reported increases in certain compounds after treatment with electrolysed water. For instance, Tomas-Callejas et al.50 reported a 33% increase in the total phenolics of mizuna baby leaves when treated with neutral electrolysed water containing 410 ppm ACC during storage at 5 °C for 11 days. In a similar study Navarro-Rico et al.52 reported that the total phenolics in broccoli washed with acidic electrolysed water containing 100 ppm ACC were stable throughout 19 days of storage, higher than sodium hypochlorite treated ones. This observation was supported by Puligundla et al.56 where electrolysed water washing did not affect the functional parameters of the treated product in contrast to other sanitizing solutions.
The effect of electrolysed water treatment on the sugar content of fruits has also been studied. Jemni et al.57 reported a reduction in the total sugar content of date palms by ∼18% and reducing sugar content by ∼5% after storage at 20 °C for 30 days upon washing with electrolysed water. In comparison neutral electrolysed water (pH 7.15) did not cause any significant changes in the sugar content hinting at the ability of compositional variation of electrolyzed water to influence the application outcome.58 In some situations, such outcomes may be desirable; for instance, acidic electrolysed water treatment (100 ppm ACC) of persimmons enhanced the reducing sugar content in wine to almost double that of pure water treatment by suppressing the yeast fermentation activity.59 Results from Solomon and Singh,60 in contrast, state that spraying electrolysed water on sugarcane could prevent the sugar inversion to fructose and glucose.
6.4 Effect of electrolysed water on chemical residues
Chemicals used during growth and cultivation of fruits and vegetables may find their way into the human system and cause several health problems. Post-harvest produce is usually rinsed with water; however in the case of hydrophobic residues, tap water may not be a reliable way of cleaning the fresh produce.61 As a result chlorine based solutions are often used to get rid of the chemical residues in the produce.62 The high ORP and free available chlorine concentrations of electrolyzed water make it eligible for use in fresh produce for removal of pesticide and insecticide residues. However, studies have shown that alkaline electrolyzed water is more effective than acidic electrolyzed water in reducing the pesticide residues by breaking their double bonds and undergo degradation.63 Han et al.64 concluded that by increasing the pH of alkaline electrolysed water by ∼31%, its effectiveness in removing the pesticide residues increased with increase in washing time from 5 to 45 min. Similarly, Qi, Huang and Hung61 demonstrated that when the pH of the acidic electrolysed water is reduced to less than half, residue degradation was significantly higher. However, neutral electrolyzed water did not demonstrate any residual removal ability for pesticides tested on the post-harvest fruit indicating that solution pH and composition are critical to its effectiveness in residual removal.65
Wang et al.63 demonstrated that acidic/slightly acidic electrolysed water reduced pesticide residues of dimethoate from fresh vegetables by 99% in 10 min, whereas the alkaline electrolysed water was only able to achieve 40% pesticide removal in the same time. The difference in the ability of different electrolysed water stems from the structural differences of the pesticides. For instance, acidic/slightly acidic electrolysed water is more equipped to break the P
S double bond present in pesticides such as diazinon and phosmet by oxidizing the double bond while alkaline electrolysed water is more capable of attacking C
O, C
N and N
O double bonds attributed to the fact that they need to be reduced to cause degradation (Qi et al.,61 2018). However, in the presence of both kinds of double bonds, acidic/slightly acidic electrolyzed water demonstrates better efficacy in residue removal.
7. Regulations for the use of electrolysed water on fresh produce
Electrolysed water containing HOCl is a well-established, fast-acting, broad-spectrum antimicrobial compound that is generally utilized for surface disinfection. However, its use in food is generally governed by regulatory authorities such as the United States Food and Drug Administration (USFDA), Food Safety and Standards Authority of India (FSSAI), Food Standards Australia and New Zealand (FSANZ) and Australian Pesticides and Veterinary Medicines Authority (APVMA) among others in order to ensure that the end products for consumption are free of any toxic residues or contaminants that can pose a threat to human health.
Electrolysed water is an FDA-approved substance for use as an antimicrobial wash on fruits and vegetables and is generally used at a concentration lower than 60 ppm of ACC in order to achieve general sanitisation as well as targeted disinfection in fruits and vegetables. The Japanese Ministry of Health and Welfare has approved 20–60 ppm chlorine and slightly acidic electrolysed water with 10–30 ppm chlorine.66 As per Australian regulations, the ACC differs on the basis of the end-product and conditions of use; for instance registrations are available for use of chlorinated water at a concentration of 25–80 ppm for fruits and vegetable washing for a contact time of at least 30 seconds, and ∼20–50 ppm to control bacterial blotch in mushrooms under clean conditions. The concentrations are generally higher in case the use occurs in the presence of soil load as the effectiveness of the solution decreases in the presence of other organic matter. Residues from electrolysed water are not a concern if the concentration of the available chlorine is below 1%; however since the presence of HOCl makes electrolysed water much effective at lower concentrations, it is very rare for the fresh produce to be exposed to such high concentrations of the active ingredient.
The ACC of electrolysed water used for fruits and vegetable washing is lower than that required for surface disinfection to ensure product and consumer safety. Several companies in the world manufacture electrolysed water at separate concentrations to achieve the desired outcome; for instance AquaOx LLC in the United States produces two types of electrolysed water with different HOCl concentrations which have been tested in food and for medical applications. Similarly, Spraying Systems Co. across the globe have diversified their applications of HOCl from hard surface disinfection and odour control to fruits and vegetable washing as well as carcass washes by managing the ACC in the end solution.
8. Recommendations and future scope
The management of postharvest diseases and the quality of fruits and vegetables is a difficult task, and electrolyzed water has shown tremendous scope as an alternative disinfection technology that can be used on fresh produce. Some of the advantages that electrolysed water offers are
• relatively inexpensive on-site generation systems,
• environmentally friendly solutions generated using raw materials like salt and water,
• reduction in supply chain logistics by ready availability of solutions.
• Safe handling due to non-toxic nature of the chemicals.
• Does not lead to the formation of “super” bacteria that are resistant to disinfecting practices unlike others.67
• More effective than bleach and chlorine68
However, certain limitations are associated with the application of electrolysed water and typically revolve around its storage. The sensitivity of HOCl to heat, light and air causes its rapid degradability and hence, a lower shelf life.14,29 It has been reported that while closed storage conditions may prolong the shelf life of the product,22 refrigerated conditions are well suited for the stability of the HOCl molecule compared to 25 °C.69 In addition, agitation may further aggravate the stability issue such that Len et al.70 reported 30 h of agitation was sufficient for removing all chlorine from electrolysed water. Overall, the form of chlorine may have a significant impact on the shelf life of electrolysed water where acidic electrolysed water is the least stable among the other types.71 Therefore, if optimisation can be met between the pros and cons of the generation and application of electrolysed water, the status of this novel technology can attract more attention and gain wider acceptance in the coming years for sanitation and disinfection of food products.
Conflicts of interest
There are no conflicts to declare.
References
-
D. Singh and R. R. Sharma, Postharvest diseases of fruits and vegetables and their management, in Postharvest Disinfection of Fruits and Vegetables, Academic Press, 2018, pp. 1–52 Search PubMed.
- Y. Pan, J. H. Cheng and D. W. Sun, Cold plasma-mediated treatments for shelf life extension of fresh produce: A review of recent research developments, Compr. Rev. Food Sci. Food Saf., 2019, 18(5), 1312–1326 CrossRef PubMed.
- Y. Duan, G. B. Wang, O. A. Fawole, P. Verboven, X. R. Zhang, D. Wu, U. L. Opara, B. Nicolai and K. Chen, Postharvest precooling of fruit and vegetables: A review, Trends Food Sci. Technol., 2020, 100, 278–291 CrossRef CAS.
- S. Sivapalasingam, C. R. Friedman, L. Cohen and R. V. Tauxe, Fresh produce: a growing cause of outbreaks of foodborne illness in the United States, 1973 through 1997, J. Food Prot., 2004, 67(10), 2342–2353 CrossRef PubMed.
-
D. A. Adams, Summary of notifiable infectious diseases and conditions — United States, 2014, Morbidity and mortality weekly report (MMWR), 2016, vol. 63, pp. 1–152 Search PubMed.
- S. Khalid, A. U. Malik, A. S. Khan, M. N. Khan, M. I. Ullah, T. Abbas and M. S. Khalid, Tree age and fruit size in relation to postharvest respiration and quality changes in ‘Kinnow’mandarin fruit under ambient storage, Sci. Hortic., 2017, 220, 183–192 CrossRef CAS.
- E. Dziedzic, J. Błaszczyk, M. Bieniasz, K. Dziadek and A. Kopeć, Effect of modified (MAP) and controlled atmosphere (CA) storage on the quality and bioactive compounds of blue honeysuckle fruits (Lonicera caerulea L.), Sci. Hortic., 2020, 265, 109226 CrossRef CAS.
- M. Ponce-Valadez, H. B. Escalona-Buendía, J. M. Villa-Hernández, F. D. de León-Sánchez, F. Rivera-Cabrera, I. Alia-Tejacal and L. J. Pérez-Flores, Effect of refrigerated storage (12.5 C) on tomato (Solanum lycopersicum) fruit flavor: A biochemical and sensory analysis, Postharvest Biol. Technol., 2016, 111, 6–14 CrossRef CAS.
- W. Zhang, H. Zhao, J. Zhang, Z. Sheng, J. Cao and W. Jiang, Different molecular weights chitosan coatings delay the senescence of postharvest nectarine fruit in relation to changes of redox state and respiratory pathway metabolism, Food Chem., 2019, 289, 160–168 CrossRef CAS PubMed.
- L. Palou, M. Serrano, D. Martínez-Romero and D. Valero, New approaches for postharvest quality retention of table grapes, Fresh Prod., 2010, 4(1), 103–110 Search PubMed.
- S. Horvitz and M. J. Cantalejo, Application of ozone for the postharvest treatment of fruits and vegetables, Crit. Rev. Food Sci. Nutr., 2014, 54(3), 312–339 CrossRef CAS PubMed.
- O. J. Williams, G. S. Raghavan, K. D. Golden and Y. Gariepy, Postharvest storage of Giant Cavendish bananas using ethylene oxide and sulphur dioxide, J. Sci. Food Agric., 2003, 83(3), 180–186 CrossRef CAS.
- G. Sortino, A. Allegra, R. Passafiume, G. Gianguzzi, G. Gullo and A. Gallotta, Postharvest application of sulphur dioxide fumigation to improve quality and storage ability of “Red Globe” grape cultivar during long cold storage, Chem. Eng. Trans., 2017, 58, 403–408 Search PubMed.
- S. M. Rahman, I. Khan and D. H. Oh, Electrolyzed water as a novel sanitizer in the food industry: current trends and future perspectives, Compr. Rev. Food Sci. Food Saf., 2016, 15(3), 471–490 CrossRef.
- M. S. Aday, Application of electrolyzed water for improving postharvest quality of mushroom, LWT-Food Sci. Technol., 2016, 68, 44–51 CrossRef CAS.
- A. Hussien, Y. Ahmed, A. H. Al-Essawy and K. Youssef, Evaluation of different salt-amended electrolysed water to control postharvest moulds of citrus, Trop. Plant Pathol., 2018, 43, 10–20 CrossRef.
- F. Shi, X. Li, H. Meng, W. Wei and Y. Wang, Reduction in chilling injury symptoms by hot electrolyzed functional water treatment may function by regulating ROS metabolism in Satsuma orange fruit, LWT, 2020, 125, 109218 CrossRef CAS.
-
F. Forghani, Application of electrolyzed water in agriculture, Electrolyzed Water in Food: Fundamentals and Applications, 2019, pp. 223–230 Search PubMed.
- W. Zheng, Z. Li, S. B. Shah and B. Li, Removal of ammonia and airborne culturable bacteria by proof-of-concept windbreak wall with slightly acidic electrolyzed water spray for a layer breeding house, Appl Eng Agric., 2016, 32(3), 393–399 CrossRef.
- S. Y. Hsu, Effects of flow rate, temperature and salt concentration on chemical and physical properties of electrolyzed oxidizing water, J. Food Eng., 2005, 66(2), 171–176 CrossRef.
- S. Y. Hsu, Effects of water flow rate, salt concentration and water temperature on efficiency of an electrolyzed oxidizing water generator, J. Food Eng., 2003, 60(4), 469–473 CrossRef.
- S. M. Rahman, J. H. Park, J. Wang and D. H. Oh, Stability of low concentration electrolyzed water and its sanitization potential against foodborne pathogens, J. Food Eng., 2012, 113(4), 548–553 CrossRef.
- L. Zhao, S. Li and H. Yang, Recent advances on research of electrolyzed water and its applications, Curr. Opin. Food Sci., 2021, 41, 180–188 CrossRef CAS.
- C. Kim, Y. C. Hung and R. E. Brackett, Roles of oxidation–reduction potential in electrolyzed oxidizing and chemically modified water for the inactivation of food-related pathogens, J. Food Prot., 2000, 63(1), 19–24 CrossRef CAS PubMed.
- D. R. Athayde, D. R. Flores, J. S. da Silva, A. L. Genro, M. S. Silva, B. Klein, R. Mello, P. C. Campagnol, R. Wagner, C. R. de Menezes and J. S. Barin, Application of electrolyzed water for improving pork meat quality, Food Res. Int., 2017, 100, 757–763 CrossRef CAS PubMed.
- L. Ni, W. Zheng, Q. Zhang, W. Cao and B. Li, Application of slightly acidic electrolyzed water for decontamination of stainless steel surfaces in animal transport vehicles, Prev. Vet. Med., 2016, 133, 42–51 CrossRef PubMed.
- Y. R. Huang, Y. C. Hung, S. Y. Hsu, Y. W. Huang and D. F. Hwang, Application of electrolyzed water in the food industry, Food Control, 2008, 19(4), 329–345 CrossRef.
- N. I. Khalid, N. S. Sulaiman, N. Ab Aziz, F. S. Taip, S. Sobri and M. A. Nor-Khaizura, Sustainable sanitation: Screening methods of electrolysing parameters for a custom-built electrolysed water generator, Clean. Eng. Technol., 2022, 8, 100482 CrossRef.
- D. Hricova, R. Stephan and C. Zweifel, Electrolyzed water and its application in the food industry, J. Food Prot., 2008, 71(9), 1934–1947 CrossRef CAS PubMed.
- B. S. Mahmoud, Electrolyzed water: A new technology for food decontamination-A review, Dtsch. Lebensm.-Rundsch., 2007, 103(5), 212–221 CAS.
- B. S. Mahmoud, K. Yamazaki, K. Miyashita, S. Il-Shik, C. Dong-Suk and T. Suzuki, Decontamination effect of electrolysed NaCl solutions on carp, Lett. Appl. Microbiol., 2004, 39(2), 169–173 CrossRef CAS PubMed.
- S. Koseki, K. Yoshida, S. Isobe and K. Itoh, Decontamination of lettuce using acidic electrolyzed water, J. Food Prot., 2001, 64(5), 652–658 CrossRef CAS PubMed.
- N. Favre, A. Bárcena, J. V. Bahima, G. Martínez and L. Costa, Pulses of low intensity light as promising technology to delay postharvest senescence of broccoli, Postharvest Biol. Technol., 2018, 142, 107–114 CrossRef.
- A. Jalali, S. Seiiedlou, M. Linke and P. Mahajan, A comprehensive simulation program for modified atmosphere and humidity packaging (MAHP) of fresh fruits and vegetables, J. Food Eng., 2017, 206, 88–97 CrossRef CAS.
- A. Mditshwa, L. S. Magwaza, S. Z. Tesfay and U. L. Opara, Postharvest factors affecting vitamin C content of citrus fruits: A review, Sci. Hortic., 2017, 218, 95–104 CrossRef CAS.
- D. Sardella, R. Gatt and V. P. Valdramidis, Physiological effects and mode of action of ZnO nanoparticles against postharvest fungal contaminants, Food Res. Int., 2017, 101, 274–279 CrossRef CAS PubMed.
- G. Romanazzi, S. M. Sanzani, Y. Bi, S. Tian, P. G. Martínez and N. Alkan, Induced resistance to control postharvest decay of fruit and vegetables, Postharvest Biol. Technol., 2016, 122, 82–94 CrossRef CAS.
- S. Khayankarn, J. Uthaibutra, S. Setha and K. Whangchai, Using electrolyzed oxidizing water combined with an ultrasonic wave on the postharvest diseases control of pineapple fruit cv.‘Phu Lae’, Crop Prot., 2013, 54, 43–47 CrossRef CAS.
- J. L. Guentzel, K. L. Lam, M. A. Callan, S. A. Emmons and V. L. Dunham, Postharvest management of gray mold and brown rot on surfaces of peaches and grapes using electrolyzed oxidizing water, Int. J. Food Microbiol., 2010, 143(1–2), 54–60 CrossRef CAS PubMed.
- D. O. Okull and L. F. Laborde, Activity of electrolyzed oxidizing water against Penicilium expansum in suspension and on wounded apples, J. Food Sci., 2004, 69(1), FMS23 CrossRef CAS.
- M. I. Al-Haq, Y. Seo, S. Oshita and Y. Kawagoe, Disinfection effects of electrolyzed oxidizing water on suppressing fruit rot of pear caused by Botryosphaeria berengeriana, Food Res. Int., 2002, 35(7), 657–664 CrossRef.
- K. Whangchai, K. Saengnil, C. Singkamanee and J. Uthaibutra, Effect of electrolyzed oxidizing water and continuous ozone exposure on the control of Penicillium digitatum on tangerine cv.‘Sai Nam Pung’during storage, Crop Prot., 2010, 29(4), 386–389 CrossRef CAS.
- C. N. Tango, I. Khan, P. F. Kounkeu, R. Momna, M. S. Hussain and D. H. Oh, Slightly acidic electrolyzed water combined with chemical and physical treatments to decontaminate bacteria on fresh fruits, Food Microbiol., 2017, 67, 97–105 CrossRef CAS PubMed.
- T. Ding, Z. Ge, J. Shi, Y. T. Xu, C. L. Jones and D. H. Liu, Impact of slightly acidic electrolyzed water (SAEW) and ultrasound on microbial loads and quality of fresh fruits, LWT-Food Sci. Technol., 2015, 60(2), 1195–1199 CrossRef CAS.
- X. Chen, C. N. Tango, E. B. Daliri, S. Y. Oh and D. H. Oh, Disinfection efficacy of slightly acidic electrolyzed water combined with chemical treatments on fresh fruits at the industrial scale, Foods, 2019, 8(10), 497 CrossRef CAS PubMed.
- S. Koide, D. Shitanda, M. Note and W. Cao, Effects of mildly heated, slightly acidic electrolyzed water on the disinfection and physicochemical properties of sliced carrot, Food Control, 2011, 22(3–4), 452–456 CrossRef CAS.
- Q. Guo, X. Lv, F. Xu, Y. Zhang, J. Wang, H. Lin and B. Wu, Chlorine dioxide treatment decreases respiration and ethylene synthesis in fresh-cut ‘H ami’melon fruit, Int. J. Food Sci. Technol., 2013, 48(9), 1775–1782 CrossRef CAS.
- H. H. Zhi, Q. Q. Liu, Y. Dong, M. P. Liu and W. Zong, Effect of calcium dissolved in slightly acidic electrolyzed water on antioxidant system, calcium distribution, and cell wall metabolism of peach in relation to fruit browning, J. Hortic. Sci. Biotechnol., 2017, 92(6), 621–629 CrossRef CAS.
- D. Rico, A. B. Martín-Diana, C. Barry-Ryan, J. M. Frías, G. T. Henehan and J. M. Barat, Use of neutral electrolysed water (EW) for quality maintenance and shelf-life extension of minimally processed lettuce, Innovative Food Sci. Emerging Technol., 2008, 9(1), 37–48 CrossRef CAS.
- A. Tomás-Callejas, G. B. Martínez-Hernández, F. Artés and F. Artés-Hernández, Neutral and acidic electrolyzed water as emergent sanitizers for fresh-cut mizuna baby leaves, Postharvest Biol. Technol., 2011, 59(3), 298–306 CrossRef.
- V. M. Gómez-López, P. Ragaert, J. Ryckeboer, V. Jeyachchandran, J. Debevere and F. Devlieghere, Shelf-life of minimally
processed cabbage treated with neutral electrolysed oxidising water and stored under equilibrium modified atmosphere, Int. J. Food Microbiol., 2007, 117(1), 91–98 CrossRef PubMed.
- J. Navarro-Rico, F. Artés-Hernández, P. A. Gómez, M. Á. Núñez-Sánchez, F. Artés and G. B. Martínez-Hernández, Neutral and acidic electrolysed water kept microbial quality and health promoting compounds of fresh-cut broccoli throughout shelf life, Innovative Food Sci. Emerging Technol., 2014, 21, 74–81 CrossRef CAS.
-
L. Gillberg, A. D. Ørskov, M. Liu, L. B. Harsløf, P. A. Jones and K. Grønbæk, Vitamin C–A new player in regulation of the cancer epigenome, in Seminars in Cancer Biology, Academic Press, 2018, vol. 51, pp. 59–67 Search PubMed.
- I. Vandekinderen, J. Van Camp, B. De Meulenaer, K. Veramme, N. Bernaert, Q. Denon, P. Ragaert and F. Devlieghere, Moderate and high doses of sodium hypochlorite, neutral electrolyzed oxidizing water, peroxyacetic acid, and gaseous chlorine dioxide did not affect the nutritional and sensory qualities of fresh-cut iceberg lettuce (Lactuca sativa Var. capitata L.) after washing, J. Agric. Food Chem., 2009, 57(10), 4195–4203 CrossRef CAS PubMed.
- Q. Lin, R. Liang, P. A. Williams and F. Zhong, Factors affecting the bioaccessibility of β-carotene in lipid-based microcapsules: Digestive conditions, the composition, structure and physical state of microcapsules, Food Hydrocolloids, 2018, 77, 187–203 CrossRef CAS.
- P. Puligundla, J. W. Kim and C. Mok, Broccoli sprout washing with electrolyzed water: Effects on microbiological and physicochemical characteristics, LWT, 2018, 92, 600–606 CrossRef CAS.
- M. Jemni, M. Otón, J. G. Ramirez, F. Artés-Hernández, N. Chaira, A. Ferchichi and F. Artés, Conventional and emergent sanitizers decreased Ectomyelois ceratoniae infestation and maintained quality of date palm after shelf-life, Postharvest Biol. Technol., 2014, 87, 33–41 CrossRef CAS.
- H. Bessi, H. Debbabi, K. Grissa and S. Bellagha, Microbial reduction and quality of stored date fruits treated by electrolyzed water, J. Food Qual., 2014, 37(1), 42–49 CrossRef CAS.
- W. Zhu, B. Zhu, Y. Li, Y. Zhang, B. Zhang and J. Fan, Acidic electrolyzed water efficiently improves the flavour of persimmon (Diospyros kaki L. cv. Mopan) wine, Food Chem., 2016, 197, 141–149 CrossRef CAS PubMed.
- S. Solomon and P. Singh, Efficacy of electrolyzed water to minimize postharvest sucrose losses in sugarcane, Sugar Technol., 2009, 11, 228–230 CrossRef CAS.
- H. Qi, Q. Huang and Y. C. Hung, Effectiveness of electrolyzed oxidizing water treatment in removing pesticide residues and its effect on produce quality, Food Chem., 2018, 239, 561–568 CrossRef CAS.
- S. R. Azam, H. Ma, B. Xu, S. Devi, M. A. Siddique, S. L. Stanley, B. Bhandari and J. Zhu, Efficacy of ultrasound treatment in the removal of pesticide residues from fresh vegetables: A review, Trends Food Sci. Technol., 2020, 97, 417–432 CrossRef CAS.
-
J. Wang and R. Han, Removal of pesticide on food by electrolyzed water, Electrolyzed Water in Food: Fundamentals and Applications, 2019, pp. 39–65 Search PubMed.
- Y. Han, L. Song, Q. An and C. Pan, Removal of six pesticide residues in cowpea with alkaline electrolysed water, J. Sci. Food Agric., 2017, 97(8), 2333–2338 CrossRef CAS PubMed.
- H. Calvo, D. Redondo, S. Remón, M. E. Venturini and E. Arias, Efficacy of electrolyzed water, chlorine dioxide and photocatalysis for disinfection and removal of pesticide residues from stone fruit, Postharvest Biol. Technol., 2019, 148, 22–31 CrossRef CAS.
- S. Koide, J. I. Takeda, J. Shi, H. Shono and G. G. Atungulu, Disinfection efficacy of slightly acidic electrolyzed water on fresh cut cabbage, Food Control, 2009, 20(3), 294–297 CrossRef CAS.
- M. Al-Haq, J. Sugiyama and S. Isobe, Applications of electrolyzed water in agriculture & food industries, Food Sci. Technol. Res., 2005, 11(2), 135–150 CrossRef.
- A. Issa-Zacharia, Y. Kamitani, N. Miwa, H. Muhimbula and K. Iwasaki, Application of slightly acidic electrolyzed water as a potential non-thermal food sanitizer for decontamination of fresh ready-to-eat vegetables and sprouts, Food Control, 2011, 22(3–4), 601–607 CrossRef CAS.
- K. A. Fabrizio and C. N. Cutter, Stability of electrolyzed oxidizing water and its efficacy against cell suspensions of Salmonella Typhimurium and Listeria monocytogenes, J. Food Prot., 2003, 66(8), 1379–1384 CrossRef CAS.
- S. V. Len, Y. C. Hung, D. Chung, J. L. Anderson, M. C. Erickson and K. Morita, Effects of storage conditions and pH on chlorine loss in electrolyzed oxidizing (EO) water, J. Agric. Food Chem., 2002, 50(1), 209–212 CrossRef CAS PubMed.
- X. Cui, Y. Shang, Z. Shi, H. Xin and W. Cao, Physicochemical properties and bactericidal efficiency of neutral and acidic electrolyzed water under different storage conditions, J. Food Eng., 2009, 91(4), 582–586 CrossRef CAS.
-
S. R. Dubey and A. S. Jalal, Detection and classification of apple fruit diseases using complete local binary patterns, in 2012 Third International Conference on Computer and Communication Technology, IEEE, 2012, pp. 346–351 Search PubMed.
- C. L. Wilson and M. E. Wisniewski, Biological control of postharvest diseases of fruits and vegetables: an emerging technology, Annu. Rev. Phytopathol., 1989, 27(1), 425–441 CrossRef.
- A. O. Paulus, Fungal diseases of strawberry, HortScience, 1990, 25(8), 885–889 Search PubMed.
- P. L. Pusey and C. Wilson, Postharvest biological control of stone fruit brown rot by Bacillus subtilis, Plant Dis., 1984, 68(9), 753–756 CrossRef.
- M. H. Nam, M. S. Park, H. S. Kim, T. I. Kim and H. G. Kim, Cladosporium cladosporioides and C. tenuissimum cause blossom blight in strawberry in Korea, Mycobiology, 2015, 43(3), 354–359 CrossRef PubMed.
- Y. Aysan, F. Sahin, M. Mirik, M. F. Donmez and H. Tekman, First report of crown gall of apricot (Prunus armeniaca) caused by Agrobacterium tumefaciens in Turkey, Plant Pathol., 2003, 52(6), 793–801 CrossRef.
- S. Mohammadi and M. H. Aminifard, Effect of essential oils on postharvest decay and some quality factors of peach (Prunus persica var. Redhaven), J. Biol. Environ. Sci., 2012, 6(17), 147–153 Search PubMed.
- J. W. Eckert, Post-harvest diseases of citrus fruits, Outlook Agric., 1978, 9(5), 225–232 CrossRef.
- G. A. Secor and N. C. Gudmestad, Managing fungal diseases of potato, Can. J. Plant Pathol., 1999, 21(3), 213–221 CrossRef CAS.
- V. Kumar, S. S. Neeraj and N. A. Sagar, Post harvest management of fungal diseases in onion—a review, Int. J. Curr. Microbiol. Appl. Sci., 2015, 4(6), 737–752 CAS.
- F. J. Gea, M. J. Navarro, M. Santos, F. Diánez and J. Carrasco, Control of fungal diseases in mushroom crops while dealing with fungicide resistance: A review, Microorganisms, 2021, 9(3), 585 CrossRef CAS PubMed.
- J. Liu, S. Tian, X. Meng and Y. Xu, Effects of chitosan on control of postharvest diseases and physiological responses of tomato fruit, Postharvest Biol. Technol., 2007, 44(3), 300–306 CrossRef CAS.
- M. P. Srivastava and R. N. Tandon, Post-harvest diseases of tomato in India, Mycopathol. Mycol. Appl., 1966, 29, 254–264 CrossRef.
|
This journal is © The Royal Society of Chemistry 2024 |