Effects of different microwave power on the drying kinetics and physicochemical quality of brown shrimp (Metapenaeus dobsoni)
Received
23rd August 2023
, Accepted 2nd October 2023
First published on 17th October 2023
Abstract
Microwave processing is used in industry for drying food commodities as it improves the quality of products and reduces the drying period. In the present study, the effects of microwave power of 600 W (105 and 100 min), 800 W (95 and 90 min), and 1000 W (80 and 65 min) and temperature (60 and 70 °C) on the drying kinetics and quality of microwave-dried shrimp were investigated. The results revealed that increasing the microwave power and temperature increase the drying rate. During the drying process, protein, fat, and ash content increased, whereas the moisture content decreased. The energy value of the dried shrimp increased from 54.41 to 287 kcal g−1. The yield of dried shrimp was in the range of 24–25%. The pH, trimethylamine nitrogen (TMA-N), total volatile base nitrogen (TVB-N), free fatty acids (FFA), thiobarbituric acid-reactive substances (TBARS), and non-protein nitrogen (NPN) values increased slightly in the dried shrimp. Moreover, the hardness of the fried shrimp decreased, while its springiness, gumminess, cohesiveness, and chewiness increased slightly. The lightness (L*), redness (a*), yellowness (b*), chroma, browning index, whiteness index, and ΔE increased in the dried shrimp. The results indicated that the quality of the dried shrimp and drying time decreased when the microwave power was increased.
Sustainability spotlight
Microwave processing technology is an advanced processing method which is attractive for many applications, including the drying of food. Fish drying is an age-old preservation technique and is practiced conventionally using sun, solar and electrical energy. In most cases, fish is dried un-hygienically in coastal regions, which may affect the health of consumers. Studies on the drying of shrimp using this novel technique assume that there is great importance in terms of sustainability as it reduces the drying time compared to other drying techniques, which also improves product quality considerably. The work is in line with UNSDGs 3, 9, 12 and 14.
|
1 Introduction
Shrimps are highly sought after food products due to their unique taste and culinary properties. Shrimp-based snack products take less than 3 minutes to prepare. Due to their extreme perishability nature, fishery products, including shrimps, are preserved at different stages to extend their shelf life, with drying being one of the oldest preservation methods adopted. Of the total fishery products exported from India, dried fish products constitute 7.45% in terms of quantity and represented a market value of 156.94 million US $ in 2020–21 (https://mpeda.gov.in/?page_id=438). This signifies the importance of dried fishery products. Traditionally shrimps are dried in the sun or using solar or electrical drying techniques. The drying of food products, including fishery products, is considered to be an important preservation method. Currently the planet finds itself fixed between two extreme positions. First, the global population is expected to increase from its current 7.2 billion to 12.3 billion by 2100.35,46,47 Due to increasing population, hunger and malnourishment is increasing and nearly 3.1 billion people do not have access to a healthy diet (SOFI, 2022). Second, nearly 1.3 billion tons (1/3rd of the total food produced in the world) is lost every year due to post-harvest loss, according to Gustavsson et al.51 According to the ICAR-Central Institute of Post-Harvest Engineering and Technology 2015 report, 5.23 and 10.52% inland and marine fish loss is reported from India.52 Adopting appropriate harvest and post-harvest measures across the entire supply chain will help in reducing this huge loss of valuable food commodity.
Among the various processing and preservation methods, drying has been practiced for many centuries, with continuous improvements being made. Drying is considered to be one of the earliest methods used to preserve food. Drying is crucial for enhancing global food security at a relatively cheap price. Drying can reduce the cost of packaging, transportation, storage, and processing, reduce postharvest losses, extend shelf life, and increase added value by removing moisture to a safe level. It can also prevent the growth and reproduction of microorganisms, and mitigate moisture-mediated deteriorative biochemical reactions.36 Dried fish is one of the most important exported marine products in many countries such as Turkey, Iran, India, Thailand, Russia, China, Malaysia and United States (Darvishi et al. 2013). Traditional drying methods that are energy-intensive have been replaced with newer energy-saving, fast and reliable advanced drying techniques (Xiao et al., 2015). Microwave drying is one such novel drying technique in which microwaves generated are used to remove moisture rapidly.1 This is mainly due to the deep penetration of microwaves into food products that results in volumetric heating and the subsequent rapid removal of moisture.
There are very few reports on the drying of shrimps,38 hot air-assisted continuous microwaving,39 microwave-dried white-leg shrimp43 and microwave vacuum-dried shrimp.42 Darvishi et al.2 studied the influence of microwave heating on drying rate, effective moisture diffusivity (EMD), and energy for sardine fish drying at four different microwave powers of 200 W, 300 W, 400 W, and 500 W. A high rehydration rate, reddish color of the dried shrimp, low shrinkage, and approximately 20% moisture content are acceptable quality attributes of dried shrimp.3 The effects of different hot air temperatures and microwave powers on the quality characteristics of tilapia fillets were examined and it was revealed that increasing temperature and microwave power decreased moisture content while increasing rehydration rate and shrinkage in the fish.4 Vacuum microwave drying techniques produce high-quality dried fish, consuming less energy and requiring less drying time.5 Many research studies have reported in detail on the various aspects of traditional and advanced drying processes48–50 and the quality changes in the product during the process of drying.37,44
Microwave technology has gained in popularity due to its benefits, which include reduced drying time, energy conservation, improved product quality and uniform temperature distribution.6 Based on a literature survey, limited work has been reported on shrimp drying using a microwave. Hence, the present study was undertaken to investigate the effect of microwave drying on the drying kinetics and physiochemical quality of dried shrimp at different microwave powers and temperatures.
2 Material and methods
Shrimp (Metapenaeus dobsoni) of grade (200/300 no. per kg, length 40–60 mm) were peeled and transported from Cochin, Kerala, in an insulated box with ice to. The sample was immediately washed with tap water after arriving at the ICAR-Central Institute of Fisheries Technology pilot plant facility in Cochin and dried in a microwave dryer (Model MDR-1601-V5, Enerzi Microwave Systems Pvt. Ltd., India). Microwave drying experiments were performed at 60 °C and 70 °C for peeled shrimp at microwave powers of 600 W, 800 W, and 1000 W to assess the quality of the products dried under different conditions.
2.1. Determination of the drying kinetics
2.1.1. Moisture content.
The moisture content of the shrimp was calculated as a percentage under both wet and dry conditions using a typical hot air oven (105 ± 2 °C).7 The wet moisture content was determined using the following formula:
where Wi represents the initial sample weight and Wf represents the final sample weight.
Similarly, moisture content on a dry basis was calculated using the formula as follows:
where
Wi represents the initial sample weight,
Wf represents the final sample weight, and
Wd represents the dry matter in the sample.
2.1.2. Drying rate and moisture ratio.
The following formula was used to calculate the drying rate (DR):
where Mt is the initial moisture content, Mt+dt is the final moisture content, and dt is the time interval.
The moisture ratio (MR) was determined using the following formula:
where
Mo is the initial moisture content and
M is the moisture content of the dried sample.
2.1.3. Specific energy consumption.
The specific energy consumption (SEC) was calculated using the following equation and expressed as kW h kg−1 of water evaporated:46
where Et is the total energy in kW h and Mw is the mass of water evaporated in kg.
2.1.4. Water activity.
An Aqua lab instrument (AquaLab Series 3L, Decagon Devices, Inc. Pullman, Washington DC, USA) was used to determine the water activity of the sample.
2.2. Determination of the yield of dried shrimp
The differences in the weight of shrimp before and after drying was used to calculate its yield:
2.3. Rehydration ratio
The dried shrimp rehydration ratio was determined using the method of Doymaz and Ismail:8
2.4. Determination of proximate composition
To evaluate moisture content, a known quantity of homogenized shrimp meat was placed in a hot air oven at 105 ± 2 °C for 16 h. The moisture content was then calculated and expressed as a percentage.7 The crude protein content of the shrimp sample was evaluated by digesting and distilling the samples in micro-Kjeldahl distillation apparatus. The crude protein content was determined and expressed as a percentage by multiplying the total nitrogen content by a conversion factor of 6.25.7 Petroleum ether (60–80 °C boiling point) was used to extract the crude fat content using Soxhlet apparatus.7 The crude fat content was calculated and expressed as a percentage. The ash content was determined using a muffle furnace maintained at 550 °C for about 4 h,7 and calculated and expressed as a percentage. The energy value of the shrimp meat was calculated using factors for protein and fat, i.e., 4.27 and 9.02 kcal g−1, respectively.9
Energy value (kcal per 100 g) = protein × protein factor (4.27 kcal) + fat × fat factor (9.02 kcal) |
2.5. Determination of physicochemical quality
The pH of the homogenized shrimp meat was measured using a digital pH meter (Cyberscan 510, Eutech Instruments, Singapore). Trimethylamine nitrogen (TMA-N) and total volatile base nitrogen (TVB-N) contents were measured using a micro-diffusion technique.10 The TMA-N and TVB-N of the shrimp meat were calculated and expressed in mg N2 per 100 g. AOCS11 methods were used to analyze the peroxide value (PV) and the free fatty acids (FFA). PV and FFA were expressed as milliequivalents of O2 per kg fat and as % oleic acid, respectively. The thiobarbituric acid-reactive substances (TBARS) value was analyzed according to Tarladgis et al.12 The TBARS value was calculated and expressed as mg malonaldehyde per kg of shrimp meat. The AOAC13 technique was used to evaluate the non-protein nitrogen (NPN) compounds in the shrimp meat.
2.6. Determination of texture
A Universal Testing Machine (Lloyd Instruments LRX Plus, Lloyd Instruments Ltd., Hampshire, UK) was used to analyze the texture of the shrimp meat. Using a 50 mm diameter cylindrical probe and a 50 N load cell, samples were compressed twice at a speed of 12 mm s−1 for analysis. Different textural parameters, such as hardness (N) (hardness 1 and 2, maximum force required to result in deformation during first and second cycle, respectively), springiness (mm), cohesiveness, gumminess (N), chewiness (N), adhesiveness (N mm), and stiffness (N mm−1), were measured during double compression of 40% with a trigger force of 0.5 kg. The Nexygen software of the instrument was used to generate a curve of the sample's deformation due to load.14
2.7. Determination of instrumental color
The color of the shrimp meat was determined using a Hunter Lab MiniScan® XP Plus Spectro Colorimeter, Model No. D/8-S (Hunter Associates Laboratory Inc., Reston, VA, USA).
A 2.5 inch-diameter glass cell with a circular shape was filled with half of the sample and inserted into the grove for color examination. Using a D65 illuminant and a 10° standard observer, parameters such as L* (lightness), which has a range of 0 to 100, a* [redness (+)/greenness (−)] and b* [yellowness (+)/blueness (−)] were evaluated.15 To calculate the chroma, hue angle, whiteness index, and browning index, eqn (1)–(4) were used. Delta E (ΔE) was calculated as the total of the color differences between L*, a*, and b* using eqn (5).
| Chroma (C) = √a*2 + b*2 | (1) |
| Hue angle (h) = tan−1(b*/a*) | (2) |
| Whiteness index (WI) = 100 − √(100 − L*)2 + a*2 + b*2 | (3) |
| Browning index (BI) = [100(X − 0.31)]/0.17 | (4) |
where,
X = (
a* + 1.75
L*)/(5.645
L* +
a* − 3.012
b*)
| ΔE = [(L0 − L*)2 + (a0 − a*)2 + (b0 − b*)2]½ | (5) |
where,
L0,
a0,
b0 is the initial value,
L*,
a*,
b* is the final value.
2.8. Statistical analysis
The experimental data of microwave-dried shrimps were analyzed using IBM SPSS version 28.0.0.0, Statistical Package for Social Science. For data analysis, the mean, standard deviation, and one-way analysis of variance (ANOVA) were used. The Duncan's multiple range test was used to calculate the mean separations, and 95% of differences were considered significant (P < 0.05).
3 Results and discussion
3.1. Drying kinetics, rehydration ratio, drying time, water activity and yield of dried shrimp
Drying shrimp in a microwave dryer resulted in the rapid removal of moisture within the drying period. The surface moisture of the shrimp decreased initially, resulting in rapid drying which removed the moisture very quickly. Microwave drying at an increased power (1000 W) and heating temperature (70 °C) resulted in a faster drying rate and subsequently reduced the drying time (Fig. 1). During the drying process, increasing the microwave power (600, 800, and 1000 W) and temperature (60 and 70 °C) resulted in a shorter drying time. At 60 °C, drying times were 105, 100 and 95 min, respectively, compared to 90, 80, and 65 min at 70 °C. The shortest drying time was observed for 1000 W microwave power at both the temperatures studied (Fig. 1). This could be due to the higher power, which caused more heat to be generated inside the product, resulting in rapid moisture loss.
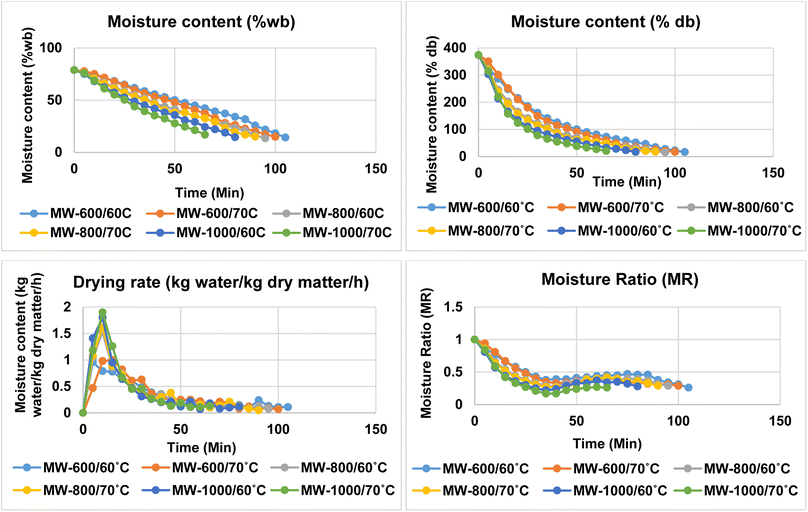 |
| Fig. 1 Drying kinetics of microwave-dried shrimp. | |
Drying at higher temperature (70 °C) and higher microwave power (1000 W) resulted in 46.15 and 38.46% less drying time compared to shrimp dried at 600 and 800 W, respectively, at 70 °C. When drying was done at lower temperature (60 °C) at a higher microwave power of 1000 W, reductions in drying time of 31.25 and 18.75% were observed for 600 and 800 W microwave power, respectively, at 60 °C. This indicates that microwave drying at a higher temperature is advantageous to obtain better drying time reduction. Between 70 and 60 °C, drying time reductions of 18.75, 5.26 and 4.76% were observed for shrimps processed at 1000, 800 and 600 W, respectively, indicating a higher process time reduction for higher microwave power. Similar to this, Lin et al.16 also noted that a decrease in shrimp drying time was associated with increased microwave power (300, 500, 700, and 900 W). Dried shrimp should possess a reddish colour, low shrinkage, a high rate of rehydration, and very low moisture content.3 High microwave power and temperature cause faster moisture evaporation from the surface as well as from inside the muscle, resulting in a low moisture content.17 According to Duan et al.,4 hot air temperature and microwave power both accelerate the drying rate of fish fillets. Darvishi et al.2 reported that the drying period for sardine fish was shown to decrease as microwave power increased during the drying process. The results of this study indicated that as the microwave power and temperature increased, the shrimp drying time decreased considerably.
The specific energy consumption of microwave dried shrimp at 60 °C and 70 °C with 600, 800 and 1000 W microwave power was 41.67 kW h kg−1, 71.43 kW h kg−1, 83.33 kW h kg−1, 55.53 kW h kg−1, 213.68 kW h kg−1, and 198.41 kW h kg−1, respectively (Fig. 2). This clearly indicates that at a higher microwave power, energy consumption was lower at a high temperature compared to a low temperature. However, this was not the same for low microwave power. The water activity of microwave-dried shrimp at various microwave powers and temperatures ranged from 0.58 to 0.63 (Table 1). The water activity of the anchovy fish dried by hot air-assisted continuous infrared drier was reported to be in the range of 0.61–0.68.29,38,39 According to Wang and K. Sheng (2006)45 and Jayaraman and Das Gupta (2014),55 reduction in water activity inhibits enzymatic and oxidative reactions. The yield of dried products is controlled by the moisture content in the end products. The weight of the sample decreased due to moisture diffused during drying. Yields in the range of 24.32 to 25.09% were observed for the different microwave power used in the study (600, 800, and 1000 W at 60 and 70 °C, Table 1). The rehydration ratio is widely used to evaluate the structural quality of dried food products. The hydration ratio of dried shrimp at different microwave powers and temperatures ranged from 1.45 to 1.85 (Table 1). The rehydration decreased upon increasing the microwave power and temperature. For dried shrimp, Murali et al.18 reported a rehydration ratio of 2.4.
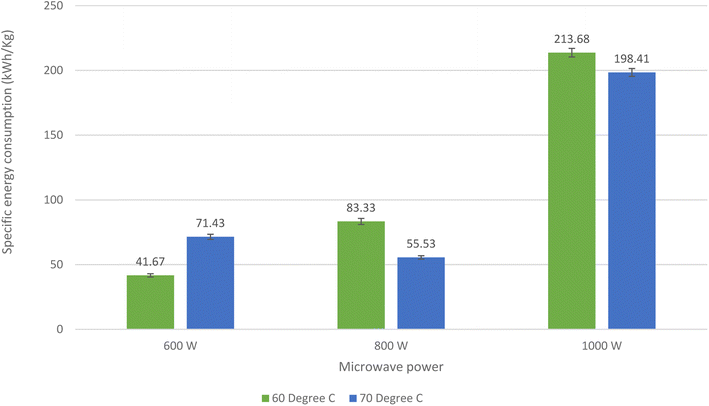 |
| Fig. 2 Specific energy consumption of microwave-dried shrimp. | |
Table 1 Drying time, yield, water activity, rehydration ratio and proximate composition of microwave (MW)-dried shrimpa
Treatment |
Drying time (min) |
Yield (%) |
Water activity |
Rehydration ratio |
Moisture (%) |
Protein (%) |
Fat (%) |
Ash (%) |
Energy value (kcal per 100 g) |
Mean ± SD (n = 3); a–g values in the same column for each attribute followed by a different letter are significantly different (p < 0.05).
|
PUD shrimp |
— |
NA |
— |
NA |
78.90 ± 0.25d |
16.25 ± 0.21a |
0.23 ± 0.01a |
0.43 ± 0.00a |
54.41 ± 0.84a |
MW-600/60 °C |
105 |
24.6 |
0.60 |
1.82 |
8.92 ± 1.02abc |
83.14 ± 1.35c |
4.24 ± 0.11b |
3.18 ± 0.08d |
272.41 ± 3.90cd |
MW-600/70 °C |
100 |
24.7 |
0.61 |
1.67 |
9.70 ± 0.45bc |
82.46 ± 1.34c |
5.37 ± 0.07d |
3.11 ± 0.12d |
266.01 ± 4.00c |
MW-800/60 °C |
95 |
24.32 |
0.60 |
1.70 |
8.59 ± 0.96ab |
84.35 ± 2.71c |
4.21 ± 0.05b |
3.06 ± 0.07d |
279.22 ± 8.31de |
MW-800/70 °C |
90 |
25.09 |
0.60 |
1.85 |
8.85 ± 1.18abc |
77.65 ± 1.15b |
5.10 ± 0.09c |
2.80 ± 0.05c |
264.87 ± 3.81c |
MW-1000/60 °C |
80 |
24.26 |
0.63 |
1.51 |
10.29 ± 0.84c |
77.13 ± 2.54b |
5.82 ± 0.05e |
2.44 ± 0.03b |
241.50 ± 7.14b |
MW-1000/70 °C |
65 |
25.04 |
0.58 |
1.45 |
7.87 ± 0.99a |
78.28 ± 0.75b |
6.41 ± 0.04f |
2.52 ± 0.05b |
287.20 ± 2.89e |
3.2. Changes in the proximate composition of microwave-dried shrimp
The amount of protein (77.13 to 84.35%), fat (4.21 to 6.41%), and ash (2.44 to 3.18%) increased with a subsequent decrease in the moisture content (Table 1). The moisture content of the microwave-dried products was in the range of 7.87–10.29%. The lowest moisture content was observed for shrimps dried at 1000 W microwave power at 70 °C. With a decrease in the moisture content, the protein and fat content showed a significant (P < 0.05) increase. The highest and lowest protein content were observed for microwave-processed shrimp at 800 W and 1000 W, respectively, at a temperature of 60 °C. The fat content of the microwave-dried shrimp was higher in comparison to that of fresh shrimp (Table 1). The highest fat content was observed for shrimp microwave-processed at 1000 W at 70 °C and the lowest fat content was determined in shrimp processed at 800 W at 60 °C, which could be due to the relative loss of moisture content resulting in an increase in fat content. According to Wu and Mao,19 microwave drying increased the protein (69.20%), fat (5.56%), and ash (4.28%) content of carp fillets. According to Murali et al.18 the protein content of shrimp, mackerel, and sardines increased as a result of water loss after drying. Similar findings were reported by Murali et al.18 and Wu and Mao.19 Moreover, microwave-dried shrimp had a higher ash level after drying, which could be due to the concentration of chemical ingredients during the drying process. Murali et al.18 also observed that ash levels in dried shrimp increased during drying.
3.3. Biochemical quality of dried shrimp
3.3.3. Changes in the pH in microwave-dried shrimp.
The pH of shrimp indicates the quality of its freshness. The fresh shrimp had an initial pH value of 7.02. During microwave drying at various microwave powers (600, 800, and 1000 W) and temperatures (60 and 70 °C), the pH slightly increased (7.12 to 7.58). A higher increase in pH was determined in samples dried in a microwave dryer at a higher temperature (Table 2). Similar results were obtained by Chaijan et al.,20 who reported that the pH of microwave semi-dried tilapia increased with increasing microwave power. According to Chaijan et al.,20 the pH of microwave-dried tilapia was found to be greater than that of sun-dried tilapia, probably due to the formation of volatile bases during drying. According to Benjakul et al.,21 the degradation of nitrogenous substances increases the pH value in fish flesh after drying.
Table 2 Biochemical quality analysis of microwave (MW)-dried shrimpab
Treatment |
pH |
TMA-N (mg N per 100 g) |
TVB-N (mg N per 100 g) |
TBARS (mg MDA per kg) |
FFA (mg% oleic acid) |
NPN (mg g−1) |
Mean ± SD (n = 3); a–g values in the same column for each attribute followed by a different letter are significantly different (p < 0.05).
MW – microwave (600, 800 and 1000 W power), W – watts, TMA – N-trimethylamine nitrogen, TVBN – total volatile base nitrogen, TBARS – thiobarbituric acid-reactive substances, FFA – free fatty acids, NPN – non protein nitrogen.
|
PUD shrimp |
7.02 ± 0.02a |
0.20 ± 0.01a |
7.60 ± 0.00a |
0.31 ± 0.04a |
8.31 ± 0.13a |
203.00 ± 9.90a |
MW-600/60 °C |
7.23 ± 0.03d |
3.50 ± 0.99b |
35.65 ± 0.99g |
0.40 ± 0.01ab |
22.18 ± 0.51f |
643.10 ± 19.77c |
MW-600/70 °C |
7.15 ± 0.02b |
2.10 ± 0.99b |
32.16 ± 1.98f |
0.52 ± 0.01c |
22.31 ± 1.02f |
678.13 ± 9.89d |
MW-800/60 °C |
7.12 ± 0.02b |
3.49 ± 0.99b |
15.37 ± 1.98b |
0.44 ± 0.01bc |
20.29 ± 0.49e |
440.03 ± 9.88b |
MW-800/70 °C |
7.58 ± 0.04e |
3.40 ± 0.96b |
27.87 ± 0.96e |
0.44 ± 0.08bc |
14.90 ± 0.49d |
455.46 ± 9.61b |
MW-1000/60 °C |
7.19 ± 0.04c |
2.09 ± 0.99b |
24.42 ± 0.99d |
0.36 ± 0.07ab |
12.07 ± 0.71c |
746.52 ± 29.60e |
MW-1000/70 °C |
7.23 ± 0.03d |
2.03 ± 0.96b |
18.30 ± 0.96c |
0.53 ± 0.09c |
10.18 ± 1.20b |
433.87 ± 19.17b |
3.3.4. Changes in TMA-N and TVB-N.
In this study, the fresh shrimp had a trimethylamine nitrogen (TMA-N) value of 0.20 mg N2 per 100 g (Table 2). The TMA-N value slightly increased during microwave drying (2.03 to 3.50 mg N2 per 100 g) at different microwave powers (600, 800, and 1000 W) and temperatures (60 and 70 °C). The breakdown of NPN (non-protein nitrogen) components such as TMAO by bacterial activity and some intrinsic enzymes is the primary cause of the rise in TMA-N content.22,33,54 According to Lakshmanan et al.,23 TMA-N values are greater in salted dried sardines compared to fresh sardines. Total volatile base nitrogen (TVB-N) is a quality and freshness indicator for fishery products. The initial TVB-N value was 7.60 mg N2 per 100 g for fresh shrimp. During microwave drying at different microwave powers (600, 800, and 1000 W) and temperatures (60 and 70 °C), the TVB-N value (15.37 to 35.65 mg N2 per 100 g) slightly increased (Table 2). The TVB-N content observed in this study was found to be well below the limits of 200 mg N2 per 100 g suggested for dried and salted fishery products.40,41 Murali et al.18 observed an increased TVB-N value of 22 mg N2 per 100 g in shrimp after solar drying. At higher temperatures, the TVB-N value of dried shrimp was lower. Using different microwave powers for drying, the lowest TVB-N value was observed for microwave-dried shrimp at 800 and 1000 W at 60 and 70 °C. Studies indicated that drying temperature and drying time influence the formation of volatile bases directly (Table 2). Drying at a higher microwave power resulted in lower TVB-N values.
3.3.5. Changes in the TBARS value of microwave-dried shrimp.
The TBARS value is commonly used to assess the degree of lipid oxidation. In fresh shrimp, the initial TBARS value was 0.31 mg MDA equivalent per kg (Table 2). The TBARS value increased during microwave drying at different microwave powers (600, 800, and 1000 W) and temperatures (60 and 70 °C) (0.36 to 0.53 mg MDA equivalent per kg). According to Murali et al.,18 shrimp dried using a solar LPG hybrid dryer had a TBARS value of 0.52 mg MDA equivalent per kg. The lipid oxidation that occurred during microwave drying, according to Qiu et al.,24 was mainly due to the increased product temperature and drying time. Murali et al.18 reported that TBARS value increased after solar drying in dried shrimp and suggested that lipid oxidation occurred in the drying process. Shrimp dried with 1000 W of microwave power at 60 °C had the lowest TBARS value. The present study indicated that as the drying temperature of the microwave processing increases, the TBARS value also increased. Chaijan et al.20 observed that the TBARS value increased after microwave drying. The present study indicated that during the drying process, the formation of oxidized compounds leading to increased TBARS values was inevitable. However, microwave drying at a higher microwave power (1000 W) and lower temperature resulted in a lower TBARS value compared to at a higher temperature. According to Connell,34 the upper limit of TBARS is 1–2 mg MDA equivalent per kg of meat and the values observed in the present study are well within this limit for shrimps dried using different conditions.
3.3.6. Changes in the FFA value in microwave-dried shrimp.
The FFA value was used to evaluate lipid hydrolysis. Fresh shrimp had an initial FFA value of 8.31 (as % oleic acid) (Table 2). FFA values in the range of 10.18–22.18 as % oleic acid were observed in the present study for samples dried at different microwave powers at two different temperatures. Microwave drying at different powers and temperatures resulted in increased FFA values. However, microwave drying at a higher temperature resulted in lower FFA values compared to samples processed at lower temperature at the same microwave power. During the drying process, the free fatty acid value indicated decreasing trends as the microwave power and temperature increased (Table 2). Additionally, Chaijan et al.20 noted that sun-dried tilapia had higher FFA values than microwave-dried tilapia. Lipases and phospholipases could be inactivated by microwave drying. It has been reported that microwave-dried carp fillets showed decreased FFA values, which could be due to lipase inactivation or the loss of volatile FFAs.25 According to Wan et al.,26 the hot air drying process accelerates the breakdown of peroxides into their carbonyl compounds while the microwave vacuum drying technique significantly reduces fat oxidation.
3.3.7. Changes in the non-protein nitrogen content.
Non-protein nitrogen is a constituent of flavor-bearing compounds, which is high in crustaceans as compared to fin fishes.32 The fresh shrimp had 203 mg g−1 of non-protein nitrogen compounds (Table 2). During microwave drying, NPN compounds increased at different microwave powers (600, 800, and 1000 W) and temperatures (60 and 70 °C). The value of the NPN compounds decreased as the microwave power increased. It was also noted that temperature had some influence on NPN compounds; higher temperatures during the microwave drying of shrimp resulted in slightly higher amounts of NPN compounds than in samples dried at lower temperatures. TVB-N content increases as a result of the breakdown of protein and NPN components by bacterial and enzymatic processes.31
3.4. Changes in textural quality in microwave-dried shrimp
The texture of dried food products is a crucial aspect of their quality. The texture profile analysis (TPA) parameters for dried shrimp, such as hardness, springiness, gumminess, cohesiveness, chewiness adhesiveness and stiffness, are shown in Table 3. The hardness 1 and 2 values of fresh shrimp are 22.32 and 17.87 N. The dried shrimp showed decreased hardness after microwave exposure at different powers (600, 800 and 1000 W) and temperatures (60 and 70 °C). Wang et al.27 also observed that microwave drying decreased the hardness of fish slices from silver carp at different temperatures and powers. This decrease in the hardness could be due to cellular changes in microstructure. Microwave drying causes cavitation or void formation in cellular structure, which in turn makes it spongy and soft as compared to other conventional drying techniques. The cohesiveness of dried shrimp increased with increasing microwave power and temperature. Drying shrimp at different microwave powers and temperatures increased the springiness, gumminess, and chewiness of the shrimp slightly (Table 3). The gumminess and chewiness increased as the microwave power and temperature increased during the microwave drying of the shrimp. Higher chewiness, gumminess, and stiffness values were observed for dried shrimp exposed to 1000 W microwave power as compared to shrimp exposed to a lower microwave power (Table 3).
Table 3 Texture profiling of microwave (MW)-dried shrimpa
Treatment |
Hardness 1 (N) |
Hardness 2 (N) |
Cohesiveness |
Springiness (mm) |
Gumminess (N) |
Chewiness (N mm) |
Adhesiveness (N mm) |
Stiffness (N mm−1) |
Mean ± SD (n = 3); a–d values in the same column for each attributes followed by a different letter are significantly different (p < 0.05).
|
PUD shrimp |
22.32 ± 2.89d |
17.87 ± 1.85d |
0.20 ± 0.01a |
1.07 ± 0.10a |
4.36 ± 0.51ab |
4.73 ± 0.99a |
−0.03 ± 0.02d |
38.01 ± 11.95ab |
MW-600/60 °C |
11.06 ± 1.62ab |
9.58 ± 1.10a–c |
0.44 ± 0.02bc |
1.21 ± 0.06a |
4.85 ± 0.83ab |
5.89 ± 1.24ab |
−0.16 ± 0.04bc |
52.12 ± 19.14a–c |
MW-600/70 °C |
10.63 ± 3.05ab |
9.10 ± 2.39ab |
0.42 ± 0.02b |
1.24 ± 0.24a |
4.47 ± 1.38ab |
5.53 ± 1.85ab |
−0.19 ± 0.06b |
23.50 ± 3.45ab |
MW-800/60 °C |
7.45 ± 2.87a |
6.75 ± 2.57a |
0.44 ± 0.04bc |
1.53 ± 0.19bc |
3.37 ± 1.51a |
5.27 ± 2.87ab |
−0.31 ± 0.05a |
19.78 ± 13.20a |
MW-800/70 °C |
17.95 ± 5.92cd |
16.81 ± 5.30d |
0.54 ± 0.05d |
1.67 ± 0.13c |
9.56 ± 2.81c |
15.70 ± 3.28c |
−0.19 ± 0.10b |
32.80 ± 15.13ab |
MW-1000/60 °C |
15.33 ± 0.81bc |
13.66 ± 0.27b–d |
0.47 ± 0.03bc |
1.28 ± 0.08ab |
7.14 ± 0.31bc |
9.18 ± 0.99ab |
−0.12 ± 0.03bc |
66.78 ± 32.54bc |
MW-1000/70 °C |
16.86 ± 4.34b–d |
14.71 ± 3.28cd |
0.49 ± 0.02c |
1.17 ± 0.14a |
8.23 ± 2.45c |
9.83 ± 3.95b |
−0.09 ± 0.01cd |
89.84 ± 41.74c |
3.5. Changes in the instrumental color of microwave-dried shrimp
Color is an essential attribute to determine consumer acceptability and desirability. Fresh shrimp had an L* (lightness) of 50.19 (Table 4). It was noted that after microwave drying at different powers and temperatures (600, 800, and 1000 W at 60 and 70 °C), the lightness (L*) increased from its initial value of 50.19 to final values in the range of 60.37–61.29. The temperature of microwave processing had little influence on the L* values of the dried shrimp. The a* value of fresh shrimp was +4.23, indicating slight redness, which increased significantly to the final values in the range of 15.51–18.6 upon microwave drying under different conditions. Ersan and Tugrul53 reported redness values, a*, for an oven-drying method of between 1.51 and 5.04 and for a vacuum-oven-drying method of between 0.44 and 4.12, which are much lower than the observed values for microwave-processed shrimp. Cheng et al.37 reported that the a* value increased during the drying process due to the release of astaxanthin, caused by the breakdown of carotenoproteins during protein denaturation. The results indicated that the redness observed for shrimps dried using a microwave is better than that of conventionally-dried shrimps, indicating their superior quality, as color is one of the prime quality criteria for purchasing dried shrimps. A slightly higher level of redness was observed when processing at a higher temperature. The highest level of redness was observed for shrimp dried at 1000 W microwave power, followed by 800 and 600 W. Fresh shrimp had a b* (yellowness) value of 12.56. Upon microwave drying at different microwave powers and temperatures, the b* (yellowness) value increased. Wang et al.27 noted that the hue angle (h°) of restructured silver carp fish slices increased during air drying, microwave-vacuum drying, freeze drying, and vacuum drying. Wang et al.5 also found that during hot air, vacuum microwave, osmosis microwave, and osmosis vacuum microwave drying, the L* (lightness), a* (redness), and b* (yellowness) values of tilapia fillets increased. The color of the dried product changed due to several factors, including pigment deterioration and both enzymatic and non-enzymatic browning.28 The chroma values showed an increasing trend with increasing microwave power (Table 4). Moreover, the chroma values increased as the drying temperature increased. It was observed that during microwave drying at various powers and temperatures, the hue angle tended to decrease compared to that of fresh shrimp. Fresh shrimp had a browning index of 48.46 (Table 4). The browning index increased as the microwave power increased. In comparison to fresh shrimp, the whiteness value increased for all the different microwave powers used for drying at both temperatures studied (Table 4). The highest whiteness value was observed for microwave-dried shrimp at 600 W at both 60 and 70 °C whereas the lowest whiteness value was observed for shrimp dried at 1000 W microwave power at 70 °C. The maximum total color change (ΔE) value of 24.87 was recorded for shrimp microwave dried at 1000 W at 70 °C (Table 4). With an increase in both microwave power and temperature, an increasing trend was observed for ΔE. Alfiya et al.29 also reported an increase in the color change (ΔE) value measured for microwave-dried shrimp. Guo et al.,30 in their study of microwave processing techniques, reported that the brief exposure time to microwave heating leads to less denaturation of myoglobin and other proteins. The studies indicated an increase in browning index, chroma values, yellowness and resultant increase in the whiteness index in the shrimps studied. This could be correlated with the lipid oxidation as observed in the formation of secondary oxidation compounds (TBARS values, Table 2). It should be noted that formation of TBARS could be due to the reaction of thiobarbituric acid with substances such as amines, amino acids, sugars, salt, acids, esters, amines, imides and oxidized proteins, resulting in the color change of the product. Also, the interaction between amines and aldehydes leading to the Maillard reaction could be another reason for the color change.
Table 4 Color profiling of microwave (MW)-dried shrimpab
Treatment |
L* |
a* |
b* |
Chroma |
Hue angle |
Browning index |
Whiteness index |
ΔE |
Mean ± SD (n = 3); a–g values in the same column for each attributes followed by a different letter are significantly different (p < 0.05).
L* – lightness, a* – red (+) and green (−), b* – yellow (+) and blue (−), ΔE – total color difference.
|
PUD shrimp |
50.19 ± 0.08a |
4.23 ± 0.06a |
12.56 ± 0.15a |
13.26 ± 0.16a |
73.07 ± 2.92b |
48.46 ± 0.11a |
34.50 ± 0.52a |
— |
MW-600/60 °C |
61.29 ± 0.08d |
16.24 ± 0.10c |
26.50 ± 0.14c |
31.08 ± 0.17c |
58.49 ± 0.02a |
74.87 ± 0.62b |
50.36 ± 0.17e |
21.49 ± 0.10b |
MW-600/70 °C |
60.37 ± 0.03b |
15.51 ± 0.03b |
26.13 ± 0.08b |
30.39 ± 0.08b |
59.31 ± 0.05a |
74.39 ± 0.23b |
50.06 ± 0.04e |
20.38 ± 0.07a |
MW-800/60 °C |
60.95 ± 0.04c |
16.66 ± 0.03d |
27.41 ± 0.01d |
32.08 ± 0.02d |
58.71 ± 0.04a |
78.45 ± 0.04c |
49.46 ± 0.02d |
22.16 ± 0.04c |
MW-800/70 °C |
60.51 ± 0.04b |
17.59 ± 0.07e |
28.12 ± 0.02e |
33.17 ± 0.03e |
57.97 ± 0.11a |
82.29 ± 0.15d |
48.43 ± 0.05c |
22.38 ± 0.02d |
MW-1000/60 °C |
60.94 ± 0.07c |
18.38 ± 0.08f |
28.87 ± 0.02f |
34.22 ± 0.05f |
57.51 ± 0.11a |
84.65 ± 0.23e |
48.07 ± 0.08c |
23.54 ± 0.03e |
MW-1000/70 °C |
60.79 ± 0.20c |
18.60 ± 0.15g |
30.67 ± 0.14g |
35.86 ± 0.19g |
58.77 ± 0.16a |
90.56 ± 0.86f |
46.86 ± 0.25b |
24.87 ± 0.13f |
4 Conclusion
This study has shown that microwave drying of shrimp at 1000 W is very rapid (65 and 80 min only at 70 and 60 °C, respectively) compared to at 800 W (90 and 95 min) and 600 W (100 and 105 min). At 70 °C, a drying time reduction of 18.75% was observed for 1000 W microwave power in comparison to only 5.26 and 4.76% at 800 and 600 W microwave power. The specific energy consumption was lower at higher microwave power and temperature of processing. Microwave drying at different microwave powers resulted in a yield of 24–25% with a rehydration ratio of 1.45–1.85. Reduction in the moisture content of the shrimp resulted in an increase in protein and fat content, which subsequently influenced the energy value. pH, TMA-N, TVB-N and NPN content increased upon microwave drying. TBARS values showed an increasing trend; however, the values were lower at higher microwave power and lower drying temperature. The voids created during microwave drying resulted in decreased hardness, whereas the springiness, chewiness and gumminess of the shrimp increased. Redness increased significantly, with better results than the reported values for other drying techniques, indicating the superior quality of the microwave-dried shrimps. The advantages of microwave drying may result in its widespread adoption in industry, which will further aid in ensuring the supply of better quality dried products to the consumer.
Author contributions
Author contributes to the acquisition of data, analysis, interpretation of data, as well as drafting, revising the article, and final approval of the version to be published. Sumit Kumar Verma: conceptualization (equal); investigation (lead); methodology (equal); writing – original draft (equal). P. Ganesan: supervision (equal); writing, review, editing (equal). Pankaj Kishore: supervision (equal); writing, review, editing (equal). Remya Sasikala: supervision (equal); writing, review, editing (equal). C. O. Mohan: conceptualization (lead); methodology (equal); funding acquisition (equal); supervision (equal); writing, review, editing (equal). Pandurengan Padmavathy: supervision (equal); writing, review, editing (equal). Nagarajan Muralidhran: supervision (equal); writing, review, editing (equal). Bindu Jagannath: review, editing, supervision; Soottawat Benjakul: final editing, and correction.
Conflicts of interest
The authors have no potential conflicts of interest to declare regarding the research, authorship, and/or publication of this article.
Acknowledgements
This work was supported by ICAR-Central Institute of Fisheries Technology, Cochin and Tamil Nadu Dr J. Jayalalithaa Fisheries University, Nagapattinam, Tamil Nadu, India under Institute and University research grants. Authors wish to thank the Director, ICAR-Central Institute of Fisheries Technology, Cochin and Tamil Nadu Dr J. Jayalalithaa Fisheries University, Nagapattinam, Tamil Nadu, India for providing essential resource support for this work. Help and support given by the staff of the Fish Processing and Quality Assurance & Management Division is highly acknowledged.
References
- S. Chandrasekaran, S. Ramanathan and T. Basak, Microwave food processing- a review, Food Res. Int., 2013, 52(1), 243–261, DOI:10.1016/j.foodres.2013.02.033.
- H. Darvishi, M. Azadbakht, A. Rezaeiasl and A. Farhang, Drying characteristics of sardine fish dried with microwave heating, J. Saudi Soc. Agric. Sci., 2013, 12(2), 121–127, DOI:10.1016/j.jssas.2012.09.002.
- C. Niamnuy, S. Devahastin and S. Soponronnarit, Effects of process parameters on quality changes of shrimp during drying in a jet-spouted bed dryer, J. Food Sci., 2007, 72(9), 553–563 CrossRef.
- Z. H. Duan, L. N. Jiang, J. L. Wang, X. Y. Yu and T. Wang, Drying and quality characteristics of tilapia fish fillets dried with hot air-microwave heating, Food Bioprod. Process., 2011, 89(4), 472–476, DOI:10.1016/j.fbp.2010.11.005.
- Q. Wang, B. Liu, J. Cao, C. Li and Z. Duan, The impacts of vacuum microwave drying on osmosis dehydration of tilapia fillets, J. Food Process Eng., 2018, 42(1), e12956, DOI:10.1111/jfpe.12956.
- I. Alibas, Energy consumption and colour characteristics of nettle leaves during microwave, vacuum and convective drying, Biosyst. Eng., 2007, 96(4), 495–502, DOI:10.1016/j.biosystemseng.2006.12.011.
-
AOAC, Official Methods of Analysis, Association of Official Analytical Chemists, Washington, DC, 21st edn, 2019 Search PubMed.
- I. Doymaz and O. Ismail, Drying characteristics of sweet cherry, Food Bioprod. Process., 2011, 89(1), 31–38, DOI:10.1016/j.fbp.2010.03.006.
-
FAO, Yield and nutritional value of the commercially more important fish species, FAO Fisheries Technical Paper 309 Part I, FAO, Rome, 1989, pp. 1–187 Search PubMed.
-
E. J. Conway, Microdiffusion Analysis of Volumetric Error, Lockwood and Son Ltd, London, Crosby, 5th edn, 1962, p. 467 Search PubMed.
-
AOCS, Official Methods and Recommended Practices of American Oil Chemists Society, AOCS, Champaign, 5th edn, 1989 Search PubMed.
- B. G. Tarladgis, B. M. Watts, M. T. Younathan and L. Dugan, A distillation method for the quantitative determination of malonaldehyde in rancid foods, J. Am. Oil Chem. Soc., 1960, 37(1), 44–48 CrossRef CAS.
-
AOAC, Official Methods of Analysis, Association of Official Analytical Chemists, Washington, DC, 2005 Search PubMed.
- M. C. Bourne, Texture profile analysis, Food Technol., 1978, 33, 62–66 Search PubMed.
- S. E. Papdakis, M. S. Abdul, R. E. Kamdem and K. L. Yam, A versatile and inexpensive technique for measuring color of foods, Food Technol., 2000, 54, 48–51 Search PubMed.
- Y. Lin, Y. Gao, A. Li, L. Wang, Z. Ai, H. Xiao and X. Li, Improvement of Pacific White Shrimp (Litopenaeus vannamei) Drying Characteristics and Quality Attributes by a Combination of Salting Pretreatment and Microwave, Foods, 2022, 11(14), 2066, DOI:10.3390/foods11142066.
- Z. Duan, M. Zhang, Q. Hu and J. Sun, Characteristics of microwave drying of bighead carp, Drying Technol., 2005, 23(3), 637–643, DOI:10.1081/DRT-200054156.
- S. Murali, D. A. Delfiya, K. S. Kumar, L. R. Kumar, S. E. Nilavan, P. R. Amulya and M. P. Samuel, Mathematical modeling of drying kinetics and quality characteristics of shrimps dried under a solar–LPG hybrid dryer, J. Aquat. Food Prod. Technol., 2021, 30(5), 561–578, DOI:10.1080/10498850.2021.1901814.
- T. Wu and L. Mao, Influences of hot air drying and microwave drying on nutritional and odorous properties of grass carp (Ctenopharyngodon idellus) fillets, Food Chem., 2008, 110(3), 647–653, DOI:10.1016/j.foodchem.2008.02.058.
- M. Chaijan, W. Panpipat and M. Nisoa, Chemical deterioration and discoloration of semi-dried tilapia processed by sun drying and microwave drying, Drying Technol., 2017, 35(5), 642–649, DOI:10.1080/07373937.2016.1199565.
- S. Benjakul, W. Visessanguan, S. Riebroy, S. Ishizaki and M. Tanaka, Gel-forming properties of surimi produced from bigeye snapper, (Priacanthus tayenus and P macracanthus), stored in ice, J. Sci. Food Agric., 2002, 82(13), 1442–1451, DOI:10.1002/jsfa.1207.
- Y. Li, S. Jia, H. Hong, L. Zhang, S. Zhuang, X. Sun and Y. Luo, Assessment of bacterial contributions to the biochemical changes of chill-stored blunt snout bream (Megalobrama amblycephala) fillets: protein degradation and volatile organic compounds accumulation, Food Microbiol., 2020, 91, 103495, DOI:10.1016/j.fm.2020.103495.
-
P. T. Lakshmanan, Fish spoilage and quality assessment, in Quality Assurance in Seafood Processing, ed. M. K. Kandoran, M. Thomas, P. T. Mathew and T. S. G. Iyer, Society of Fisheries Technologists, Cochin, 2000, pp. 26–40 Search PubMed.
- X. Qiu, S. Chen and H. Lin, Oxidative stability of dried seafood products during processing and storage: a review, J. Aquat. Food Prod. Technol., 2019, 28(3), 329–340, DOI:10.1080/10498850.2019.1581317.
- J. Zhang, D. Wu, D. Liu, Z. Fang, J. Chen, Y. Hu and X. &Ye, Effect of cooking styles on the lipid oxidation and fatty acid composition of grass carp (Ctenopharynyodon idellus) fillet, J. Food Biochem., 2013, 37(2), 212–219, DOI:10.1111/j.1745-4514.2011.00626.x.
- J. Wan, M. Zhang, Y. Wang, A. S. Mujumdar and W. Yong-Jun, Drying kinetics and quality characteristics of slightly salted grass carp fillets by hot air drying and vacuum microwave drying, J. Aquat. Food Prod. Technol., 2013, 22(6), 595–604, DOI:10.1080/10498850.2012.677969.
- Y. Wang, M. Zhang, A. S. Mujumdar and K. J. Mothibe, Quality changes of dehydrated restructured fish product from silver carp (Hypophthalmichthys molitrix) as affected by drying methods, Food Bioprocess Technol., 2013, 6, 1664–1680 CrossRef CAS.
- B. Ling, J. Tang, F. Kong, E. J. Mitcham and S. Wang, Kinetics of food quality changes during thermal processing: a review, Food Bioprocess Technol., 2015, 8, 343–358 CrossRef CAS.
- P. V. Alfiya, G. K. Rajesh, S. Murali, D. A. Delfiya, M. P. Samuel and M. V. Prince, Development and evaluation of hot air-assisted microwave dryer for shrimp (Metapenaeus dobsoni), J. Food Process. Preserv., 2022, 46(11), 17112, DOI:10.1111/jfpp.17112.
- Q. Guo, D. W. Sun, J. H. Cheng and Z. Han, Microwave processing techniques and their recent applications in the food industry, Trends Food Sci. Technol., 2017, 67, 236–247, DOI:10.1016/j.tifs.2017.07.007.
- P. K. Prabhakar, P. P. Srivastav and S. S. Pathak, Kinetics of Total Volatile Basic Nitrogen and Trimethylamine Formation in Stored Rohu (Labeo rohita) Fish, J. Aquat. Food Prod. Technol., 2019, 28(5), 452–464, DOI:10.1080/10498850.2019.1604598.
-
C. E. Hebard, G. J. J. Flick and R. E. Martin, Occurrence and significance of trimethylamine oxide and its derivates in fish and shellfish, in Chemistry and Biochemistry of Marine Food Products, ed. R. E. Martin, G. J. J. Flick, and C. E.Hebard, AVI Publishing, Westport, Connecticut, 1982, pp. 149–304 Search PubMed.
- L. Gram and P. Dalgaard, Fish spoilage bacteria–problems and solutions, Curr. Opin. Biotechnol., 2002, 13(3), 262–266 CrossRef CAS PubMed.
-
J. J. Connell, Control of Fish Quality, Surrey: Fishing News (Books), Farnham, 4th edn, 1995 Search PubMed.
- P. Gerland, A. E. Raftery, H. Ev Ikova, N. Li, D. Gu, T. Spoorenberg, L. Alkema, B. K. Fosdick, J. Chunn and N. Lalic,
et al., World Population Stabilization Unlikely This Century, Science, 2014, 346, 234–237, DOI:10.1126/science.1257469.
-
R. Sehrawat, H. W. Xiao, S. V. Jangam and A. S. Mujumdar, Food Security: Impact of Climate Change and Technology, 2019, https://www.arunmujumdar.com/e-books.htm Search PubMed.
- S. Cheng, Y. Tang, T. Zhang, Y. Song, X. Wang, H. Wang and M. Tan, Approach for monitoring the dynamic states of water in shrimp during drying process with LF-NMR and MRI, Drying Technol., 2018, 36(7), 841–848 CrossRef CAS.
- P. V. Alfiya, G. K. Rajesh, S. Murali, D. A. Delfiya, M. P. Samuel and M. V. Prince, Quality evaluation of solar and microwave dried shrimps-a comparative study on renewable and dielectric heating methods, Sol. Energy, 2022, 246, 234–244, DOI:10.1016/j.solener.2022.09.048.
- P. V. Alfiya, G. K. Rajesh, S. Murali, D. S. Aniesrani Delfiya, M. P. Samuel and M. V. Prince, Development and evaluation of hot air-assisted microwave dryer for shrimp (Metapenaeus dobsoni), J. Food Process. Preserv., 2022, 46(11), e17112, DOI:10.1111/jfpp.17112.
-
M. Rahman, Drying of fish and seafood, in Handbook of Industrial Drying, ed. A. S. Mujumdar, Taylor and Francis Group, CRC Press, Boca Raton, Florida, 2007, p. 552 Search PubMed.
-
J. J. Connell, Methods of assessing and selecting for quality, Control of Fish Quality, Fishing News Books, Oxford, 3rd edn, 1990, p. 240 Search PubMed.
- W. Sun, H. Ji, D. Zhang, Z. Zhang, S. Liu and W. Song, Evaluation of Aroma Characteristics of Dried Shrimp (Litopenaeus vannamei) Prepared by Five Different Procedures, Foods, 2022, 11(21), 3532, DOI:10.3390/foods11213532.
- H. Duppeti, S. N. Manjabhatta, A. Martin and B. B. Kempaiah, Effects of different processing methods on the biochemical composition, color and non-volatile taste active compounds of whiteleg shrimp (Litopenaeus vannamei), Food Chemistry Advances, 2022, 1, 100118, DOI:10.1016/j.focha.2022.100118.
- S. Hosseinpour, S. Rafiee, M. Aghbashlo and S. S. Mohtasebi, Computer Vision System (CVS) for In-Line Monitoring of Visual Texture Kinetics During Shrimp (Penaeus spp.) Drying, Drying Technol., 2014, 33, 238–254, DOI:10.1080/07373937.2014.947513.
- J. Wang and K. Sheng, Far infrared and microwave drying of peach, LWT–Food Sci. Technol., 2006, 39(3), 247–255, DOI:10.1016/j.lwt.2005.02.001.
- H. W. Xiao and A. S. Mujumdar, Importance of drying in support human welfare, Drying Technol., 2020, 38(12), 1542–1543 CrossRef.
- P. Gerland, A. E. Raftery, H. Ev Ikova, N. Li, D. Gu, T. Spoorenberg, L. Alkema, B. K. Fosdick, J. Chunn, N. Lalic and et al,
., World Population Stabilization Unlikely This Century, Science, 2014, 346, 234–237, DOI:10.1126/science.1257469.
- M. Zielinska, E. Ropelewska, H. W. Xiao, A. S. Mujumdar and C. L. Law, Review of recent applications and research progress in hydrid and combined microwave-assisted drying of food products: quality properties, Crit. Rev. Food Sci. Nutr., 2020, 60(13), 2212–2264 CrossRef.
- S. S. Cheng, W. T. Su, L. Yuan and M. Q. Tan, Recent developments of drying techniques for aquatic products: with emphasis on drying process monitoring with innovative methods, Drying Technol., 2021, 39(11), 1577–1594 CrossRef.
- S. Mounir, E. Amami, T. Allaf, A. Mujumdar and K. Allaf, Instant controlled pressure drop (DIC) coupled to intermittent microwave/airflow drying to produce shrimp snacks: pProcess performance and quality attributes, Drying Technol., 2020, 38(5–6), 695–711 CrossRef.
-
J. Gustavsson, C. Cederberg, U. Sonesson, R. van Otterdijk and A. Meybeck, Global Food Losses and Food Waste, Food and Agriculture Organization of the United Nations, Rome, Italy, 2011 Search PubMed.
-
NABCONS, Study to Determine Post-harvest Losses of Agri. Produces in India, Ministry of Food Processing Industries, Govt. of India, 2022, p. 338 Search PubMed.
- A. C. Ersan and N. Tugrul, The drying kinetics and characteristics of shrimp dried by conventional methods, Chem. Ind. Chem. Eng. Q., 2020, 27, 50, DOI:10.2298/CICEQ201114050E.
-
FAO, Assessment of Fish Quality, Chapter 8: Biochemical and Chemical Methods, in Quality and Quality Changes in Fresh Fish, FAO Fisheries Technical Paper 348, ed. H. H. Huss, FAO, Rome, 1995 Search PubMed.
-
K. S. Jayaraman and D. K. Das Gupta, Drying of fruits and vegetables, in Handbook of industrial drying, ed. A. Mujumdar, CRC Press, Boca Raton, Florida, 4th edn, 2014, pp. 611–635, ISBN-13: 978-14665-9666-5, https://mpeda.gov.in/?page_id=438, accessed on 12 April 2023 Search PubMed.
|
This journal is © The Royal Society of Chemistry 2024 |
Click here to see how this site uses Cookies. View our privacy policy here.