Microbial bioconversion of food waste to bio-fertilizers†
Received
8th March 2023
, Accepted 19th January 2024
First published on 2nd April 2024
Abstract
Food waste is a matter of concern in our society. A large amount of waste has been reported in municipalities (households, markets, food courts, and ceremonies), the agriculture sector, food-based industries and airports. Food wastage involves economic losses and entails the use of resources and has an environmental impact that could be avoided by educating society in environmental values and looking for new revalorization strategies. Food waste contains organic matter in a rich amount which depends on the type of waste such as oils, fruits, agro-industrial waste, bakery, dairy, meat etc. A number of strategies are applied for the management of food waste such as recycling, bioconversion, animal feed etc. Among them microbial bioconversion is now becoming popular. It employs a biochemical process in which organic waste is converted into manure that improves soil quality while taking part in the sustainable development of society and contributing to our environment. Some bacteria, fungi, and actinomycete fermentations have been described to perform this upcycling since they present many advantages such as ease, safety, cost-effectiveness, eco-friendliness, and a rapid process. A microbial bio-converted fertilizer also meets quality and safety which enhances the growth of crops. Therefore, the present work aims to present the sources and types of food waste, recent food waste scenarios around the world, the diversity of microbes that convert food waste to fertilizers, the mechanism of bioconversion, and the use of the converted fertilizer.
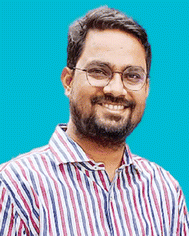 Pramod Kumar Mahish | Pramod Kumar Mahish is presently serving as Assistant Professor of Biotechnology at Govt. Digvijay Autonomous Postgraduate College, Rajnandgaon (Chhattisgarh), India, with the postal code 491441. He had 10 years of teaching and 13 years of research experience. Dr Mahish had published 21 research papers, 10 books and 21 book chapters (including Wiley, Springer, De-Gruyter, Elsevier and IGI). He had completed 3 research projects and is presently guiding 2 PhD scholars. He has received a UGC National Fellowship (New Delhi) for doing his PhD. He is also a fellow member of the World Researchers Association. Dr Mahish is engaged in the editing of 2 books on the American Chemical Society and Tylor and Francis platforms. |
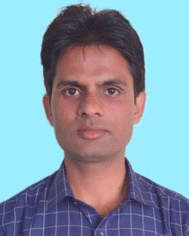 Dakeshwar Kumar Verma | Department of Chemistry, Govt. Digvijay Autonomous Postgraduate College, Rajnandgaon, Chhattisgarh, India 491441 E-mail: dakeshwarverma@gmail.com; . Dr Dakeshwar Kumar Verma, holding a PhD, serves as an Assistant Professor of Chemistry at Govt. Digvijay Autonomous Postgraduate College in Rajnandgaon, Chhattisgarh, India, with the postal code 491441. Driven by a profound passion for scientific exploration, his research focuses primarily on the preparation and design of organic compounds for diverse applications. With an impressive track record, Dr Verma has authored over 100 research papers, review articles, and book chapters that have found their place in esteemed peer reviewed international journals, including those published by ACS, RSC, Wiley, Elsevier, Springer, and Taylor & Francis, among others. This extensive body of work showcases his dedication to contributing to the collective knowledge of the scientific community. Beyond his role as an author, Dr Verma is an active and valued participant in the peer-review process. He serves as a reviewer for high-impact journals such as the Journal of Molecular Liquids (Elsevier), Materials Chemistry and Physics (Elsevier), and Scientific Reports (Nature), underscoring his commitment to maintaining the rigor and quality of scientific research. As a testament to his academic influence, Dr Verma has taken on editorial responsibilities (editor and author) for published as well as upcoming books slated to be published by renowned publishers such as ACS, RSC, Wiley, Taylor & Francis, Elsevier, Springer and De Gruyter. This role highlights his dedication to shaping and disseminating knowledge in various scientific domains. With a cumulative citation count of over 1800, an h-index of 24, and an i-10 index of 34, Dr Verma's impact is evident through the recognition and relevance of his work in the scientific community. Furthermore, his commitment to fostering the growth of future scholars is evident in his supervision of two full-time PhD research scholars. Dr Verma's dedication to research excellence has been acknowledged through prestigious awards such as the Council of Scientific and Industrial Research Junior Research Fellowship award in 2013. During his PhD journey in 2013, he also availed of the MHRD National Fellowship, further solidifying his commitment to academic growth and advancement. In essence, Dr Dakeshwar Kumar Verma's biography exemplifies his unwavering dedication to advancing the realm of chemistry through prolific research contributions, editorial engagements, and mentorship of emerging scholars. His multifaceted role as a researcher, reviewer, editor, and mentor underscores his substantial impact on the academic landscape. Google Scholar link: https://scholar.google.com/citations?user=J663VtkAAAAJ%26hl=en, SCOPUS link: https://www.scopus.com/authid/detail.uri?authorId=56835167300, WOS/PUBLONS ID: https://publons.com/researcher/4446851/dr-dakeshwar-kumar-verma. |
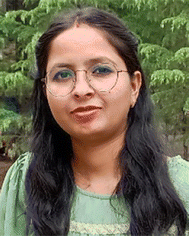 Anjali Ghritlahare | Anjali Ghritlahare is presently working as Subject Matter Specialist of Soil Science at Krishi Vigyan Kendra (Agriculture Science Centre) in Rajnandgaon affiliated with Indira Gandhi Agriculture University, Raipur (Chhattisgarh), India. She has obtained her Bachelors (Agriculture) from the BTC College of Agriculture & Research Station, Sarkanda, Bilaspur, India and Masters (Soil Science) from Junagarh Agriculture University, Gujrat, India. She has been engaged in the research, extension, field trials and training of farmers since 2012. She was awarded a gold medal as a merit student in her MS program. Her former institute was awarded the Best National Institute Award in 2017. |
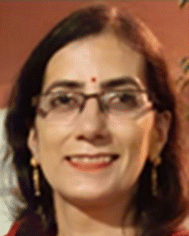 Charu Arora | Dr Charu Arora, working as Professor of Chemistry at Guru Ghasidas University (a central university), Bilaspur, C. G., India, has 21 years of teaching and research experience. Her areas of interest and research are Environmental Chemistry, Kinetics and Natural Product Chemistry. She has completed her PhD and postgraduation in Chemistry at GK University, Haridwar and the Indian Institute of Technology, Roorkee in 2003 and 1995 respectively. She received the SARC Gold Medal Award 2011 conferred by the Scientific and Applied Research Centre, CCS University, Meerut for her outstanding contribution in the field of Chemistry Research. She has published more than 60 research papers, one book and 17 book chapters with reputed publishers viz. Elsevier, Bentham Science, Wiley, Academic Excellence, Springer etc. Dr Arora is an editor, co-editor and author of several books on the Wiley science, Elsevier and De-Gruyter platforms. |
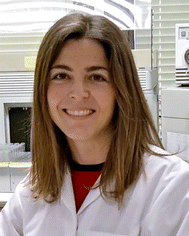 Paz Otero | Dr Paz Otero received her bachelor's degree in Food Science from the University of Santiago de Compostela (USC), Spain, in 2006. She obtained a PhD from the Pharmacology Department of the Veterinary Faculty at the same university. Dr Otero has held postdoctoral research positions at the Limerick Institute of Technology, Ireland (2014–2017), and the Institute of Food Science Research, Autonomous University of Madrid (2017–2018). She has published extensively in the fields of food chemistry, toxicology, analytical chemistry, and nutrition with more than fifty-three research articles, seventy contributions to international congresses, and ten book chapters to her credit. Dr Otero also serves as an invited reviewer for several research journals and a guest editor for special issues. |
Sustainability spotlight
Food waste is a matter of concern in our society. In this context, the use of microbial capacity to decompose organic matter is a very vibrant technique to valorize food waste. Several sources and types of food waste such as waste cooking oils, agro-industrial wastes such as vegetables, fruit, juice, wheat, rice, potato, sugarcane, bakery waste, dairy waste and different types of meat, fish and poultry wastes have been proven to be profitable sources for their use for bioconversion. And some bacteria, fungi, and actinomycete fermentations have been described to perform this upcycling since they present many advantages such as ease, safety, cost-effectiveness, eco-friendliness, and a rapid process. The present work aims to present the sources and types of food waste, recent food waste scenarios around the world, the diversity of microbes that convert food waste to fertilizers, the mechanism of bioconversion, and the use of the converted fertilizer.
|
1. Introduction
According to the Food and Agriculture Organization (FAO), the difference between food loss and food waste is that the former refers to loss of food due to quantitative or qualitative reasons, whereas if the food is expired or soiled due to carelessness of the consumer, then it is defined as food waste.1 In addition, the FAO points out that food waste is a waste of inputs, land, and labor (FAO 2011).2 In parallel, the EPA explains that it is a little different than that in a residence or commercial establishment where uneaten food or food preparation residues have been known as food waste.3 Therefore, food waste is foodstuff of all types excluded from the food supply chain fit for the consumption of humans. While 98% of the hunger population lives in developing countries among which 15% suffer from malnutrition4 and nutritional deficiency and hunger challenges, that billions of people are suffering nowadays,5 the situation in developed societies is entirely different. From one side, wealthier civilization with home delivery, better food chain systems, and supermarkets act as simple accessibility of food; however, this modern food delivery scenario brings challenges in terms of urban food waste increase and thus, has an impact on the environment and sustainable development and food safety and security.6 In addition, the anaerobic digestion of food waste makes a contribution to the greenhouse gases in the environment.7 Leachate is another environmental challenge linked to food waste. Apart from environmental challenges, several other difficulties arise from food waste, which are listed in Fig. 1. In this context, food safety and security are a globally important challenge nowadays because they are sandwiched between the commodity demand increase from the increase in population and the shortage of agricultural land due to urbanization and industrialization.8 Increasing consumption rates and shrinking crop areas are major concerns related to sustainable food demand.9 Related to safety and security, wasting food is another burning issue, of which about one-third is produced for human feasting.2 This is related to post-harvesting, pre-consumption, and post-consumption levels. An increase in greenhouse gases is directly proportional to food waste.10 Food waste creates a burden on production and demand which reflects the use of crop fields, the use of irrigated water, manpower & inputs, and fertilizers.11 The loss of biodiversity is also related to habitat destruction by agricultural areas.12
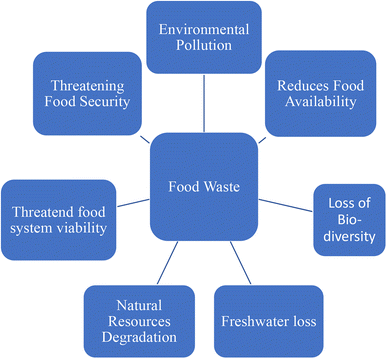 |
| Fig. 1 Effect of food waste on the environment and sustainable development. | |
In industrialized countries, governments and authorities demand limitations on waste generation in the whole food supply chain, commencing with primary production, processing, and whole food distribution, in individual households, restaurants and food services. Households account for up to 45% food waste and its amount is increasing over time.13–16 In a recent study of Russia, overproduction and consumer leftovers have been found to be the reasons for food waste and about 14 tons of food waste was averaged per restaurant per year.17 The United States Department of Agriculture-The Economic Research Service (USDA-ERS) (USA), Waste & Resources Action Programme (WRAP) (UK), FAO (UN) and UNEP (UN) are some national agencies and organizations of government that are actively engaged in the prevention of food waste and FUSION and REFRESH programs have been funded by the European Commission for sustainable utilization of food resources.18 The quantification of food waste in each sector and food chain process plays an important role in the global management of food waste. Bakery products such as bread, fruits and vegetables, and meat have been recorded as a major proportion of urban food waste.19 Apart from these, cereals, root crops, oilseeds, dairy, and fish waste form 20–50% of the waste worldwide.2
Food waste could be mainly measured by direct and indirect methods. Direct methods are based on measuring, counting and waste composition analysis, i.e., based on the primary data of infield observation. For example, weighing is one of the traditional direct methods by which food waste can be weighed in hospitals, households, schools, restaurants, etc.20 In the household collection of garbage, waste is collected from homes and food waste is separated from others to measure the actual quantity.21 When physical access to food waste is not possible, then the application of indirect methods with the use of coefficients and mass balance is employed. Indirect methods use statistical tools, modern computational software, and modeling based on mathematical formulae to estimate the quantity of food waste, so that data from indirect measurement are derived from the primary record of the direct method. In the EU, the most recommended method for all processes in the agri-food supply chains is the direct measurement and analysis of the waste composition.22
1.1 Methodology
The most popular bibliographic databases have been used to search for literature in the study viz. Scopus, Web of Science and Google Scholar. The key words used are – “Food waste”; “Food loss”; “Biofertilizer from food”; “Bioconversion of food waste”; “Valorization of food waste” and so on. The literature obtained from the database has been filtered. Books and review and research articles have been selected and other literature has been eliminated. Literature published in English has been selected and literature in other languages has been excluded. Valorization of food waste other than microbial methods was also excluded. Literature studies were also selected according to the publication period. The selected literature studies have been read and analyzed to satisfy the objective of the study.
2. Sources and management of food waste
Households and surroundings that are directly or indirectly related to individuals are the major sources of food waste after production and processing.23 Our neighbors, friends, educational institutes, markets, and working places such as physical settings influence the wastage of food.24 In a household, dependence on each other, togetherness, and structure of the family affect the use or misuse of food.25,26Fig. 2 indicates a step-by-step loss of food from land to the consumer and Fig. 3 is related to the reasons for food losses in the different steps. The quantification of overall waste in the food chain is a little challenging because it depends on harvested items, situation, process utilized etc. A study in the US suggests about 20% food waste at the production stage, 20% in processing and distribution and the rest (60%) in the consumption stage.27 Food losses are also observed to be much higher in low-income countries due to infrastructure, deprived technology, a lack of awareness about environmental parameters and market coordination.28 About 26% of food waste is recorded at the production stage in South Africa and nearly half (13%) is observed in Canada in the overall food chain. These data may vary according to foods such as cereals, fruits and vegetables and meats. Post-harvest food waste also depends on the food type. Cereal waste was observed to be maximum at post-harvest and storage steps; fruit and vegetables at the production step; meat, fish, milk and eggs at the retailing step due to a lack of coolant facility. Household or consumer waste is more in high income countries. About 40–50% household food waste has been recorded in the European Union and Canada.29
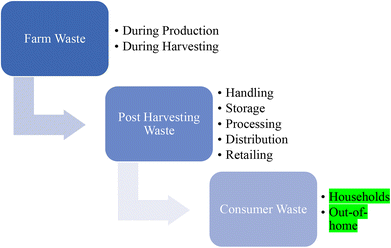 |
| Fig. 2 Loss of food waste in the various steps of food production and transport to the consumer. | |
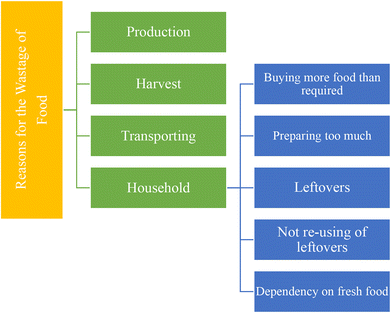 |
| Fig. 3 Reasons for the wastage of food from production to use in households. | |
There are several possibilities for managing food waste.43 Averting food waste, landfills, donations, animal feeding, recycling, production of chemicals, composting and biogas are among the most applied waste management methods on large and moderate scales (Fig. 4). All of them show advantages and disadvantages (Table 1). For example, in a fast urban lifestyle averting food waste is not possible except for some exceptions. Landfilling is a simple disposal practice; however, it presents some environmental concerns since in the long term, this method can produce contamination and pollution of the surrounding environment with a negative impact on air and groundwater.30 In addition, it contributes to leachate production, unpleasant smell around the site and sources of pathogenic microorganisms. Animal feeding is a common method found in countries with a high demand for animal food such as South Korea and the United States since it does not lead to negative environmental impacts.44 Nevertheless, this is not a profitable method for low livestock production countries. Moreover, it has to follow strict guidelines as in the case of low salt content or caffeine free products, as they can cause harmful effects on animals.34 Donation is another waste management practice currently successfully implemented in the UAE in which supermarkets, hotels and restaurants donate excess food to families in need. However, the collected food for donation represents a very small amount of the total waste. Other management methods, such as recycling food waste, seem very poor because of their rapid degradation. On the other hand, chemical conversion methods are relatively new and only implemented recently at a moderate scale; therefore they still need intensive implementation and development. Finally, composting is a suitable method for the management of food waste that involves the conversion of organic matter to ammonia-nitrogen, carbon dioxide or humic substances under an aerobic atmosphere.45 The collection of food waste in wheeled bins followed by the separation of waste on the basis of texture (dryness or wetness), source (organic or synthetic), nature (metallic or nonmetallic) and approach (recyclable or non-recyclable) is increasing in developed countries. Fig. 5 shows the organic fertilizer and biogas energy production process from household food waste. In this sense, household food waste can be recycled into biogas energy and organic fertilizers. However, the fertilizer can be used to improve crop growth, which could be beneficial for society and biogas generation could not fulfill the high demand of society.
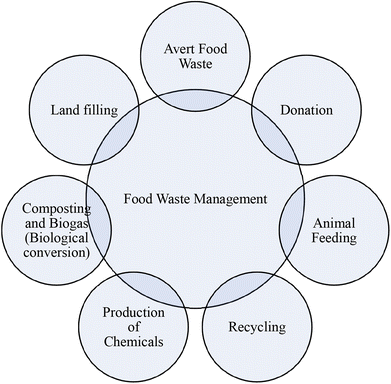 |
| Fig. 4 Main management strategies for food waste. | |
Table 1 Advantages and disadvantages of some possible management methods for food waste
S. no. |
Method of management |
Advantages |
Disadvantages |
References |
1 |
Landfill |
Easy method; no tools/equipment required; no need for scientific knowledge |
Contamination and pollution; negative impact on groundwater; emission of greenhouse gases; unpleasant odor; leachate formation; growth of mosquitoes and other insects |
6 and 30 |
2 |
Donation |
Fulfils the needs of underprivileged communities |
Improper disposal of food by the receiver |
31
|
3 |
Animal feed |
No environmental impact; more nutrients for animals |
Risk of contamination for animals |
32
|
4 |
Pyrolysis |
No emission of harmful gases; conversion to biofuels and biochar |
Needs equipment setup with high energy requirements |
33
|
5 |
Incineration |
No need for land; minimum requirement of human resources; generation of energy from waste |
Release of toxic gas and ash; need for setup |
34 and 35 |
6 |
Gasification |
Less environmental concern; production of future fuels |
High temperature and high energy requirement; release of hydrocarbons and ash |
36
|
7 |
Transesterification |
Minimizes soil and water pollution by oils; production of biodiesel from waste oil |
Complex methodology; requirement of high energy; release of water |
37 and 38 |
8 |
Composting |
Bioconversion to green manure; no environmental concern; bioremediation of harmful chemicals |
Very slow process; need for setup with skilled knowledge; generates unpleasant smell |
39
|
9 |
Anaerobic digestion |
Bioconversion of food waste to green manure and biogas; no environmental concern |
Need for a digester; low capacity; needs scientific knowledge to maintain pH and temperature |
40
|
10 |
Anaerobic fermentation |
Bioconversion of food waste to alcohols and acids; production of future fuels and green manure; less emission of CO2 and greenhouse gases; high efficiency |
Need for a fermenter or reactor; costly method; need for scientific knowledge; difficulty in product recovery |
41
|
11 |
Hydrogen production |
Bioconversion of food waste to green energy; use of byproducts as manure |
Need for production setup with skilled manpower; low capacity |
42
|
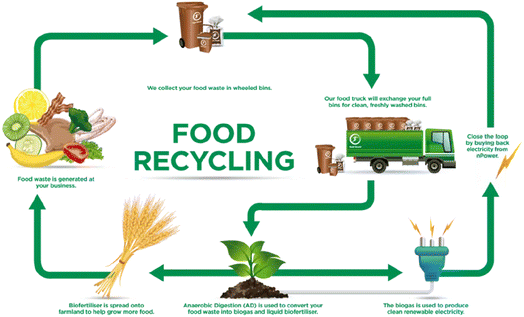 |
| Fig. 5 Scheme of organic fertilizer and biogas energy production from household food waste [Copyright 2022 @ BioEnergy Consult from Zafar S.46 source: https://www.bioenergyconsult.com/renewable-energy-food-residuals/]. | |
3. Organic composition and valorization of some food waste
In general, food waste has a good nutritional value because it is rich in high quality organic nutrients since it was intended for human consumption (see the figure in the ESI†). Produce from farms, restaurants, and manufacturing facilities, such as fruits, vegetables, meats, and cereals, make up the majority of food waste that affects every aspect of daily life. Plant-based food losses from the farming land to the customer are over 40% and cumulative. This contains vegetables which are not gathered due to inappropriate maturity, do not satisfy strict excellence stipulations by the superstores, and are disallowed harvests or parts or fiber/pomace after extracting juice by processing industries.47 However, food waste typically has high moisture content in the range of 50–85%, which reduces shelf-life and makes food waste collection and use for animal feeding difficult because of the possible spoilage.32 Therefore, a good option is its use for bioconversion. The nutritional composition of some food waste is presented in Fig. 6.
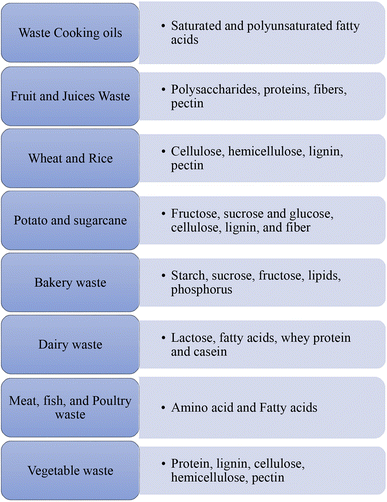 |
| Fig. 6 Organic matter of some common food waste for possible valorisation to biofertilizers. | |
3.1 Waste cooking oils
Cooking oil waste is rich mainly in saturated fatty acids such as palmitic acid, monounsaturated fatty acids such as oleic acid and polyunsaturated fatty acids such as linolenic and linoleic acids.48,49 Due to this composition, cooking oils have been biologically converted into fuel, acids, metabolites, enzymes, polymers, etc, using microorganisms. Biodiesel is one of the important products obtained from waste cooking oils.48,50 Waste cooking oils have been observed as the source of lactic acid and citric acids bio-converted by Rhizopus microspores and Lichtheimia corymbifera.51,52 Metabolites such as polyhydroxyalkanoates and carotenoids were produced from the waste of cooking oil by Paracoccus sp. strain LL1.53 Bioplastics are another important product produced from Escherichia coli in the presence of waste cooking oils as substrates.54Lichtheimia corymbifera is also used for the production of single-cell oils and lipase from waste cooking oils.52
3.2 Fruit and juice waste
Fruit waste has been reported worldwide because of its high content of protein, fiber, and carbohydrates which could be recovered and re-used. Waste oranges contain D-limonene, a type of monoterpene.55 Cellulose, hemicellulose, pectin, lignin, and sugar have been reported from pineapple waste.56 Similarly, banana peel is rich in various organic materials such as polysaccharides, proteins, fibers, and pectin.57 Fiber, proteins, and lipids are the major components of waste pomegranates and tangerines.58,59 The bioconversion of waste fruit and juices is a virtuous idea because many fruits contain only 30–50 percent edible parts while the other part is related to the peel that generally wastes after fruit consumption. Tangerine biomass has 40–50% peel, pomegranates 50%, and bananas have 30–40% peel.60 Therefore, half of the fruit contains waste having organic materials which possibly valorizes useful products. Methane as a source of fuel has been obtained from the bioconversion of passion fruit peel, cashew bagasse, and D-limonene of orange peel by the presence of microbes in anaerobic digestion.55,61 Biologically derived adsorbents have been obtained from pomegranate peel, orange juice, and tangerines.60,62 Biosorbents produced from the pomegranate peel and orange juice were able to remove phenol from the wastewater. Orange peel has been biologically converted to bioelectricity by Pseudomonas63 while bio-pigments are produced by Monascus purpureus ATCC 16365 and Penicillium purpurogenum CBS 113139.64 Orange peel waste is also used to produce biofertilizers.65 Pineapple waste has been utilized for the production of ethanol, phenolic anti-oxidants, citric acid, lactic acid, biogas, and fiber, those are achieved by Saccharomyces cerevisiae and Zymomonas mobilis (ethanol), Rhizopus oligosporus (phenol), Aspergillus niger (citric acid) and Lactobacillus lactic (lactic acid).56 Biochar and environmentally friendly substances have been produced from the banana peel.66 Banana peel is also used for the synthesis of single cell proteins biologically converted by Rhodotorula glutinis NRRL Y-1091 and Ganoderma wiiroense.67 Biofertilizers and biogas have been produced from peels of fruits such as pomegranates, sweet lime, bananas, and tangerines.60,65
3.3 Agro-industrial waste – wheat and rice
Agro-industrial waste is a rich source of cellulose, chitin, hyaluronic acid, inulin, and pectin, i.e., organic nutrients which are important substrates for biochemical conversion.68 For example, paddy straw and wheat straw particularly contain cellulose, hemicellulose, and lignin.69 In this sense, agro-industrial waste has been converted to cellulase, xylanase, and other industrially important enzymes with the aid of microorganisms such as Aspergillus flavus under solid-state fermentation.70,71 Economically significant acids such as acetic acid have been produced from agro waste by fermentative bacteria Bacillus sp. PM06 and butyric acid is obtained from Clostridium tyrobutyricum.72,73 Similarly, vanillic acid is synthesized from corn bran biologically converted by Pseudomonas putida KT2440.74 Future fuel bioethanol is synthesized from agro waste by Bacillus sp. PM06, Saccharomyces cerevisiae MTCC 4780 and mushrooms.72,75,76 Other future energies such as biohydrogen, bioelectricity, and biobutanol were also produced from agro-industrial waste.76
3.4 Agro-industrial waste – potato and sugarcane
Potatoes are composed of carbohydrates, especially starch. Potatoes contain three main types of sugars: fructose, sucrose and glucose and other compounds such as cellulose, hemicellulose, lignin, and fiber.77 In the study, ash, proteins, lipids, total sugar, fiber, starch, and phosphorus were recovered from potato processing waste.78 Bioethanol is obtained from the waste of sugarcane biochemically converted by Saccharomyces cerevisiae.79 Sugarcane bagasse is used for the production of bio-hydrogen by the thermophilic Clostridiales consortium.42 In this study, hydrogen production (1.71 mL H2 per mL culture) and yield (586.19 mL H2 per g carbohydrate) produced by Clostridiales were augmented by 113.75% and 32.48% equated with that of fermentation from solely pretreated sugarcane bagasse.
Mannooligosaccharides are obtained from potato peel and sugarcane bagasse by Penicillium oxalicum KUB-SN2-1.80Monascus rubber has been used for the production of natural pigments from sugarcane.81 Lactic acid has been obtained from the nutrients available in the waste of potatoes from the conversion of Lactobacillus pentosus.82 Sugarcane molasses is used for the production of polyhydroxybutyrate, a bioplastic produced by the action of Bacillus cereus 2156.83 Another biodegradable composite has been obtained from the waste of potato and sugarcane bagasse.84Cellulomonas sp., Klebsiella sp., Proteus sp., Enterobacter sp., and Salmonella sp. have been used to obtain organic compost. This compost was found to be rich with carbon content (26.75%) and C
:
N ratio (12.44%). In parallel, it increased the nitrogen (2.34%), phosphorus (1.15%), and potassium (1.37%) content along with the population of microorganisms i.e., bacteria, fungi, and actinomycetes.85
3.5 Bakery waste
Bakery waste contains high protein materials, although this waste is also rich in starch, sucrose, fructose, lipids, phosphorus, etc.86 Due to the availability of such nutrients, bakery waste has been used as a media nutrient for the growth of bakery yeast.87 Succinic acid is one of the important products synthesized from bakery waste biologically converted from Actinobacillus succinogenes.86,88Thermomyces sp. and Monascus purpureus have been studied to produce some important enzymes such as glucoamylase89,90 and protease89 from bakery waste. Future fuels bioethanol and biohydrogen have also been produced from bakery waste by the action of Saccharomyces cerevisiae91 and Rhodo pseudomonas palustris 42OL.92 Bakery waste is utilized for the production of bio-pigments by Monascus purpureus89 and lactate by Lactobacillus amylovorus DSM 20532.92 Environmentally friendly bioplastic (PBH) has also been obtained from the waste of bakeries.88
3.6 Dairy waste
Dairy waste is mainly composed of lactose and fatty acids. The other components are whey protein and casein.93,94 Dairy waste is converted to bioethanol by Lactococcus lactis.95 Lactose bioconversion has also been used for the production of bioethanol by microbes.96 Another future fuel biodiesel is produced from dairy wastewater by Chlamydomonas polypyrenoideum.97 By the action of microbes, bioplastics and biosurfactants have also been produced from the waste of dairy.98 Single-cell proteins are another valuable product obtained by microbial action from dairy waste96 and panner whey.99Kluyveromyces marxianus was used for the production of single-cell proteins from paneer whey. Kasmi96 also used lactose bioconversion for the production of lactic acid, citric acid, and biopolymers from dairy waste. Green manure has been obtained from the conversion of Hermetia illucens from dairy waste.100 Similarly, a liquid biofertilizer was obtained from dairy waste by the action of some geosphere microbes.101
3.7 Meat, fish, and poultry waste
Animal meat and its waste contain a high volume of fatty acids and amino acids. Fatty acids – palmitic acid, eicosapentaenoic acid (EPA), and docosahexaenoic acid (DHA) have been recorded in some fish such as pink perch (Nemipterus japonicus), Indian mackerel (Rastrelliger kanagurta) and Indian oil sardine (Sardinella longiceps).102Lactobacillus plantarum has been used for fish waste fermentation and the product was utilized as broiler chicken feed.103 Similarly, microbial bioconversion has been utilized to produce biodiesel/biogas, dietetic products (chitosan), natural pigments, and cosmetics (collagen) from the waste of fish.104 Bioactive peptides have been obtained from the waste of meat and have relevant physiological effects such as antihypertensive, antioxidant, antidiabetic, and antimicrobial.105 Waste bone meal and meat were utilized as absorbents for the removal of lead from the aqueous solution.106 Protein hydrolysates, enzymes, and polyunsaturated fatty acids have been recovered from chicken waste.107 High protein feed and gelatin are utilized in the food industry and cosmetics have been obtained from the waste of meat.108 Biofuel has also been produced from the same waste. Biofertilizers are also obtained from the conversion of waste from meat and poultry108,109Aspergillus niger was utilized for the conversion of poultry waste to biofertilizers. Similarly, Bacillus megaterium has been used for the production of liquid phosphate fertilizers from the waste of animal bones.110
3.8 Vegetable waste
Proteins, dry matter, organic matter, lignin, cellulose, hemicellulose, pectin, and digestible nutrients have been obtained from waste fruit and vegetables.111,112 Lignin (19%), hemicellulose (14%), cellulose (50%), and pectin have been specially obtained from tomato waste.113 Vegetable waste has been biologically converted to so many types of valuable products with the aid of microbial conversion. Lactic acid was obtained from the waste of vegetables by the conversion of lactic acid bacteria and Penicillium sp. The same waste is used to get protein extracts by Bacillus subtilis, Rhizopus oligosporus, and Fusarium flocciferum. Aspergillus, Yarrowia, and Trichoderma species have been used to obtain carbohydrates and glycosidases from the above waste.114 Leafy vegetable waste contains pigments of photosynthetic importance. Pigments such as anthocyanins, betalains, carotenoids, and chlorophylls have been obtained by Usmani et al.98 and have been used in the food, pharma, and cosmetic industries. Several bioactive compounds such as antioxidants, oils, fiber, fatty acids, isoprenoids, lipids, proteins, saponins, and phytoestrogens have been isolated from vegetable waste by Jiménez-Moreno et al.115 Nano-emulsions of some bioactive compounds such as carotenoids and polyphenols have also been made from vegetable waste by Saini et al.116 Bayram et al.117 synthesized food packing materials such as biopolymers and bio-composites, edible films, and coatings from the waste of vegetables. Valuable products such as fermented beverages, single-cell proteins, single-cell oils, bio-colors, flavors, fragrances, polysaccharides, biopesticides, and plant growth regulators have been obtained from the waste of vegetables through the conversion of microbes.118 Sources of energy such as bioethanol, biogas, methane, and biohydrogen have been produced from the waste of vegetables by microbial action.113,118,119 Vegetable waste has been biologically converted into biofertilizers by Trichoderma reesei.119 A similar conversion was also noted by Fritsch et al.113 from the waste of tomatoes.
4. Mechanism of bioconversion of food waste by microbes
A number of microbial groups have demonstrated their ability to convert food waste to valuable products. These microbial groups mainly belong to bacteria, actinomycetes, yeast and molds. The microbes belong to various functional groups such as aerobic nitrogen fixers, ammonifiers, denitrifiers, and nitrifiers capable of converting nutrients from food waste to fertilizers and biogas.120 To achieve this bioconversion microorganisms produce a number of enzymes viz. amylase, cellulase, xylanase and pectinase.121
The methods and mechanisms applied to the valorization of food waste into useful products play a very important role because the product quality is dependent on the process used. Food waste can be converted to produce different organic fertilisers and soil amendments by a wide range of biological, physical and chemical methods following different procedures such as composting, anaerobic digestion, dehydration, biochar production, and chemical hydrolysis. Among these, anaerobic fermentation and composting are the main large-scale conversion methods currently used.122 In addition, Fig. 7 represents some methods of microbial bioconversion of food waste to various products including biofertilizers. The incubation period, temperature, and moisture content parameters play a crucial role in the bioconversion of waste. However, emerging conversion methods for food waste such as biochar production, dehydration, and chemical hydrolysis have shown promising properties, which also makes them useful approaches for food waste bioconversion.123 Sometimes both physical and biological approaches were used for the valorization of food waste. For example, Mahmood et al.124 first utilized a household garbage dryer & processor for the valorization of vegetable and fruit peels and leftover food to dry the biomass, and then, favourable moisture waste was treated with Aspergillus niger UY2015_11.
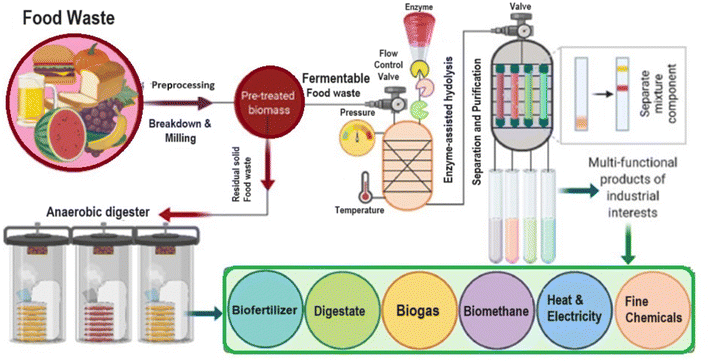 |
| Fig. 7 Microbial bioconversion methods of food waste resulting in various valuable products including biofertilizers. This conversion is achieved by methods such as anaerobic digestion, composting, fermentation by microbes of microbial enzymes, etc. [Copyright @ 2022 Elsevier from Sufficiency et al.131]. | |
The bibliography shows a wide range of food waste bioconversion processes by anaerobic fermentation using different sources and types of microorganisms. For instance, an anaerobic digestion method was applied by Dahunsi et al.125 for the bioconversion of Carica papaya (pawpaw) fruit peels into green fertilizers using bovine rumen as a source of microbes. For this, preliminary peel was converted to a slurry which was then treated with the rumen in a reactor to carry out the fermentation under anaerobic conditions for 28–34 days. Li et al.126 utilized Pseudomonas aeruginosa for the digestion of kitchen waste to obtain biofertilizers. However, in this study, the authors used a glass flask to produce anaerobic conditions at the laboratory experimental scale. Fermentation strategies play an important role in direct production or enhancement of production of biomolecules. Various fermentation methods have been applied to produce astaxanthin from food waste by Phaffia rhodozyma.127,128
In another study, a 40-L-biogas reactor was utilized for biogas production from the digestion of boiled rice, boiled cassava products, bread, boiled yam, and boiled maize by using different genera of bacteria such as Escherichia, Citrobacter, Bacillus, Pseudomonas, Proteus, Klebsiella, Clostridium, Bacteroides, Enterobacter, Staphylococcus, Salmonella, Streptococcus, Aspergillus, Mucor, Rhizopus and Penicillium.129 The biogas reactor design contained various manometers for temperature and pH control and a plastic tube for the collection of gas produced during digestion. Wang et al.130 used a mixture of bread, cabbage, pork, and rice with sawdust for the composting and production of manure for seed germination. The mixture was placed in a 20-L reactor with a controlled temperature and moisture. After day 35 of incubation nutritional profiling and seed germination of compost have been carried out.
The composting method was also applied by Tsai et al.132 in which a mechanical composter has been used to valorise food waste collected from restaurants. Initially, the food waste was chopped and mixed with sawdust to maintain moisture for favourable fermentation inside the composter. Strains of Streptomyces thermonitrificans NTU-88, isolate CH18, Brevibacillus borstelensis SH168 and Bacillus stearothermophilus ATCC 10149 were utilized for composting over 28 days. A typical composter was used by Sangamithirai et al.133 for the bioconversion of vegetable and fruit waste. Mesophilic bacteria were responsible for the composting over 3 months with mixing at regular intervals. Composting of huge volumes of food waste was performed by Lin et al.134 Sawdust and mature compost were mixed with about 800 kg of pre-sheared food waste and allowed for composting in a large reactor. Moisture content and temperature were maintained to allow for fermentation.
Stabnikova et al.135 obtained organic fertilizers from the waste of some food materials. The mechanism was adopted as an aerobic bioconversion of food waste by the bacterial strain Bacillus thermoamylovorans SW25. It took 10 days to convert food waste into organic fertilizers in a pilot scale reactor. Jiang et al.136 performed thermophilic anaerobic digestion reactions over 230 days with the aid of Methano sarcina and Methano thermobacter to digest food waste. Coffee pulp and husk were valorized by the method of composting by Dadi et al.137 A mixture of coffee waste, cow dung, and rock phosphate was treated with Bacillus megaterium in a cement cistern. The compost was turned into a consistent interlude for up to 120 days. The final products were dried in shade and fortified with some minerals before application.
5. Advanced bioprocessing methods used for the conversion of food waste to fertilizers
The bioconversion of solid waste rich in organic matter is usually performed by conventional biowaste valorisation technologies such as anaerobic digestion or composting which have been widely adopted for food waste management. Some systematic techniques are presented in Fig. 8 describing the valorisation of food waste by microbes to meet the end product as a biofertilizer.
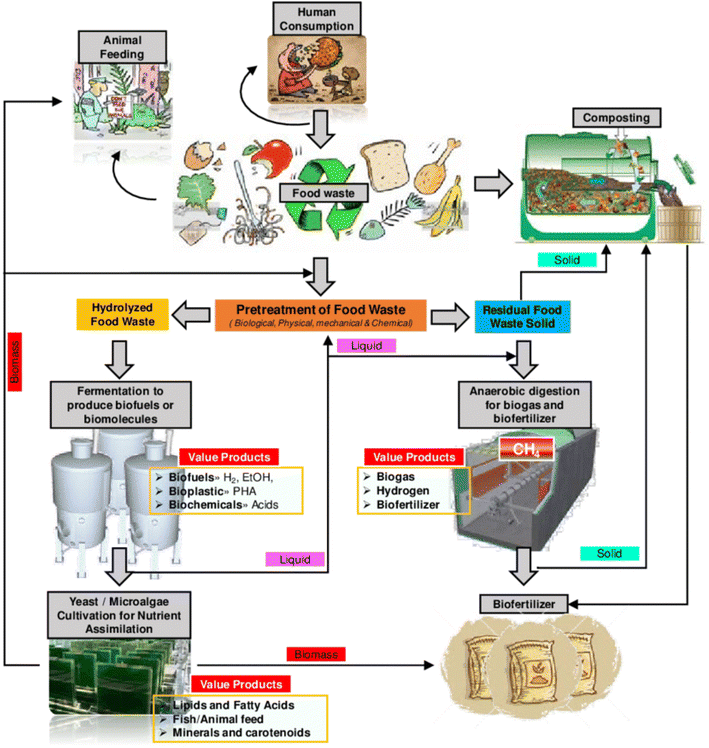 |
| Fig. 8 Techniques and methods employed for the production of biofertilizers from food waste. The steps also generate many other valuable products [Copyright 2023; Karthikeyan et al.141]. | |
However, they can sometimes be limited due to their high operation costs, low recovery efficiency and system instability.138 Advanced tools and techniques have emerged in the last few years to cover new uses and needs as they are easy, accurate, and cost-effective methods. Food waste was composted by Lin134 using a pilot-scale active aeration reactor. This is a negative-pressure fermentation technology which is a good system for maintaining proper moisture, promoting the growth of prolific active thermophilic microorganisms and reducing NH3 formation during the composting process while reducing the composting time. Evans et al.139 proposed a large bioreactor plant for the bioconversion of food waste, able to convert 30
000 tonnes of food waste per year resulting in more than 13
000 tonnes of biofertilizer as well as a huge volume of electricity and natural gas. An advanced pilot-scale reactor for the bioconversion of food waste to fertilizers was used by Stabnikova et al.135 The reactor was designed with an inlet and outlet of air, an agitator, a motor, a control panel, a cooling tower, and other necessary devices for an anaerobic reaction. Bacillus thermoamylovorans SW25 has been used for the valorization of food waste into fertilizers.
Chung et al.138 utilized microbial electrochemical technology for the biological conversion of food waste to compost and biogas. This technology has been highly promising for efficiently harvesting high value-added products from food waste. Microbial electrochemical technology is used to convert the chemical energy from organic substrates to electrical energy by using special types of biocatalysts, electrodes and bacteria resulting in the production of compost and energy gas. This technology has various advantages over conventional composting methods such as low operation cost, high recovery efficiency, system stability, and high process kinetics.
Microbial fuel cells are another advanced method utilized nowadays for the production of energy from waste and recovery of resources also gives rise to solid matter readily converted to fertilizers. This method was utilized by Xin et al.140 for the anaerobic digestion of food waste for energy production followed by solid matter for fertilizers. Fungal cells were used to prepare microbial fuel cells. The microbial fuel cell allows ultra-fast hydrolysis of waste and is therefore ideal for the production of fertilizers.112
6. Bio-fertilizers produced from food waste by microbes
Biofertilizers produced from food waste need to meet some requirements to increase soil fertility and crop production such as being rich in organic sources and macro- and microelements and having an appropriate C
:
N ratio, among others. In this sense, biofertilizers have been identified as an alternative to chemical fertilizers for the improvement of crop production in sustainable farming142,143 Some quality parameters of biofertilizers produced from food waste by microorganisms and listed in Fig. 9 are a balanced C
:
N ratio, low coliform bacteria, an increase of microbiota, organic matter and essential micro- and macroelements, a decrease of lignin cellulose and better phosphate solubilisation. Some studies confirming these properties are shown below.
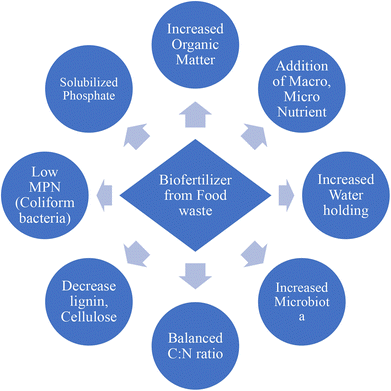 |
| Fig. 9 Quality properties of a biofertilizer produced from food waste by microorganisms. | |
Tsai et al.132 collected food waste from restaurants on the university campus to produce an organic fertilizer. The experiment was performed in bioreactor A, in which thermophilic lipolytic microbes were inoculated with food waste and bioreactor B was taken as a control without microbes. The fertilizer made from microbes has been proven to be a quality fertilizer by an increase in total nitrogen from 2.01% to 2.10%, ash increased from 24.94% to 29.21%, crude fat decreased from 4.88% to 1.34% and the C/N ratio decreased from 18.02 to 17.65. To produce this fertilizer Streptomyces thermonitrificans NTU-88, isolate CH18, Brevibacillus borstelensis SH168, and Bacillus stearothermophilus ATCC 10149 were used. Sangamithirai et al.133 produced a biofertilizer from the combination of fruit and vegetable waste that has advantages such as high water holding capacity that is useful for tolerance to droughts. The quality is field tested with the germination of cucumber seeds. A significant increase in the germination index was observed and shoot and root length increased compared to the control. Mesophilic bacteria were used to produce biofertilizers from the waste. Food waste was used to obtain compost through thermophilic bacteria.134 The resulting compost was advanced when pH drop from 5.2 to 4.3 on the first day, followed by a gradual pH gain to 7.4 on the 60th day. After 60 days, the C/N ratio dropped from 32 to below 20. The final compost contains 1.6% nitrogen, 0.6% phosphate, and 1.4% potassium with total coliforms below 3 MPN/100 mL. Lower coliform is a sign of the absence of various pathogenic organisms and hence the compost is suitable for field applications.
Aspergillus niger UY2015_11 was used for the production of green fertilizers from the waste of vegetable and fruit peels and leftover food that comes from diverse meat and vegetable menus by Mahmood et al.124 During the bioconversion of waste, phosphate has been solubilized by the fungus through the production of citric acid. The solubilization of phosphate is easily available for the utilization of plants during the growth stage. Similarly, iron- and calcium-based inorganic phosphates were also recorded for solubilization by the fungus. Zhu et al.144 used a combination of seaweed, plant ash, animal proteins, and edible mushroom residues for the bioconversion of these waste to biofertilizers with the aid of Bacillus criculans, Bacillus sphaericus, Bacillus firmus, Bacillus schlegelii, Bacillus stearothermophilus, Bacillus subtilis, Candida tropicali, and Lactobacillus delbrueckii. The application of the fertilizer to the field has increased organic matter by 1.31 and 1.55-fold compared with soil without fertilization as well as increased the quality of humus and aggregation of large particles.
The fruit peel waste of Carica papaya (pawpaw) was used for the production of organic fertilizers by Dahunsi et al.125 This valorization was achieved by the anaerobic digestion of waste in the presence of microbes. The obtained fertilizer was found to be advantageous over the chemical fertilizer with an increase of nitrogen, phosphorus, and potassium by 28, 40, and 22% respectively. However, improvements in many other macro- and microelements were also recorded. Trichoderma reesei was used for the anaerobic digestion followed by aerobic digestion of fruit and vegetable waste for biofertilizers and biogas production by Chakravarty et al.119 The obtained biofertilizer achieved a C
:
N ratio of 10–11 and a decrease in lignin by 64% in the case of food waste and 70% in the case of vegetable waste. Dadi et al.137 used coffee pulp waste for the production of an organic fertilizer. The C
:
N ratio of compost materials has decreased with the advancement of the period of decomposition from 17.6 to 7.25. Greater reduction in lignin content (37.54% to 28.46%), cellulose content (25.95 to 16.75%), and total phenol content (76.75 mg/100 g to 38.8 mg/100 g) with an increase in humic substances (40.65%) was recorded during the composting period.
Biofertilizers and their nutritional composition help promote the growth of crops and play a crucial role because a food waste fertilizer serves as a source of nutrients.122 Therefore, it is necessary to understand the promotion of plant growth by biofertilizers obtained from the valorisation of food waste (Table 2).
Table 2 Promotion of growth in plants by application of bio-fertilizers obtained from food waste
S. no. |
Food waste |
Valorisation method |
Crop to which fertilizers were applied |
Outcome of the application of bio-fertilizers |
Reference |
1 |
Carica papaya (pawpaw) fruit peels |
Anaerobic digestion |
Maize |
Better performance in terms of number of leaves, leaf area, plant height, stem girth, total shoot and root biomass, and length of the root as compared to chemical fertilizers |
125
|
2 |
Rice bran, rapeseed mill cake and fish processing waste |
Use of lactic acid bacteria, yeast and phototrophic bacteria |
Tomato (L. esculentum L. cv. Momotaro T 96) |
More active young leaves and developed more young fruit as compared to chemical fertilizers. Fruit yield is higher in the later growth stage and vitamin C is higher in the fruit as compared to chemical fertilizers |
145
|
3 |
Vegetable and fruit peels and leftover food |
Use of Aspergillus niger UY2015_11 |
Lettuce and Brassica rapa |
Similar growth was recorded from the green fertilizer obtained from fruit and vegetable waste as compared to chemical and rapeseed oil cake organic fertilizers. Therefore, no significant difference was observed |
124
|
4 |
Kitchen waste oil |
Use of Pseudomonas aeruginosa PA-3 |
Cabbage |
Plant height reached 7.83 cm in 12 days, while that of the control group was 5.17 cm. Leaf area on the 12th day and 15th day was 1.08 cm2 and 1.35 cm2, respectively while in the control it was recorded to be 0.56 cm2 and 0.71 cm2. Similarly the stem diameter of cabbage plants was also increased with the addition of a fertilizer obtained from kitchen waste oil as compared to the control |
126
|
5 |
Vegetable and fruit waste |
Use of mesophilic bacteria |
Cucumber |
A significant increase in cucumber shoots and root length. A germination index of more than 80% was obtained showing phytotoxic-free and mature compost |
133
|
6 |
Food waste |
Use of Streptomyces thermonitrificans NTU-88, Brevibacillus borstelensis SH168 and Bacillus stearothermophilus ATCC 10149 |
Alfalfa |
The germination rate of alfalfa seed increased from 10.50 (day 7) to 97.50% (day 28) |
132
|
7 |
Food waste |
Bacillus thermoamylovorans SW25 |
Ipomoea aquatica (kang kong plant) |
The addition of organic fertilizers to the subsoil increased the yield and growth of the plant by 1.5 to 2 times |
135
|
7. Safety concerns of biofertilizers obtained from the valorisation of food waste
Increasing the biological origin fertilizer technology has brought forward the safety of plants from the co-existence of pathogenic microbes' growth during production steps. Exceptionally, Salmonella, Klebsiella and total coliforms have been obtained from the fertilizer produced from food waste.129 However, this kind of fertilizer may be used for the crop field once the pathogen has been treated. But pathogen-free fertilizers do not require such chemical treatment to kill the pathogen. The biofertilizer obtained from the valorization of household waste, slaughter residues, and some organic food waste does not support the growth of some pathogens such as S. enterica and L. monocytogenes even after supplementation with rich substrates in the waste sources containing carbon sources and other trace elements.146 Therefore, this provides a promising alternate biofertilizer with crop safety.
A pilot-scale active aeration reactor was studied for composting food in which a biological filter bed was used to remove NH3 from the emission by Lin.134 Using this composting system less than 1 ppm NH3 was recorded which is below the detectable limit and prevents its emission into the ambient atmosphere. This fermentation reaction system is also advantageous to promote the growth of active thermophilic microorganisms. The acidification and the growth of thermophilic microbes disinfect the compost to eliminate pathogenic microorganisms. With decreasing pH and increasing temperature during the initial stage of the composting process, the number of total coliforms decreases. On the first day, the compost pH was about 4.3, the temperature was 65 °C, and total coliforms dropped to 240 MPN/100 mL. After the process enters the thermophilic fermentation period (higher than 65 °C in this case) on the 14th day, the total coliforms decrease to below 3 MPN/100 mL which is the LDL (lowest detection limit) for MPN.
The biofertilizer obtained from vegetable and food waste was found to have good hygienic quality, since, pathogenic bacteria such as Escherichia coli and Salmonella dysenteriae were not detected in the mature compost.133 During the experimental stage low CO2 emission was also recorded in environmentally friendly production of compost from food waste. Food waste (including vegetable and fruit peels and leftover food which comes from diverse meat and vegetable menus) collected from the university restaurant was used to produce a green fertilizer using Aspergillus niger UY2015_11. Results showed that one strain of Aspergillus niger UY2015_11 significantly inhibited the growth of Fusarium. Mahmood et al.124 worked with 112 strains, of which 16 inhibited the growth of Fusarium spp.
8. Economic aspects of bioconversion of food waste
Food waste management with traditional ways such as landfilling could not be economically sustainable because an expenditure is needed to collect, transport and landfill waste, while microbial bioconversion gives rise to products such as fertilizers, bioelectricity, and biofuels which can have value. In an estimate by Yong et al.147 biofertilizers and biogas produced from waste provide a sustainable economy as well as protection of the environment in Malaysia. Revenue from the sale of bio-fertilizers and biogas electricity to the grid is estimated to be much higher and profitable. The initial expenditure to make a setup for production of fertilizers from waste was recovered from the year 4 and the business estimate was profitable from the same year. A similar observation is noted in the study by Ma et al.148 The estimated co-location of food waste and municipal waste water treatment gives rise to a profitable corporation. The energy expenditure for waste water treatment in a year (16.86 billion kW h) and recoverable electric energy from food waste (26.44 billion kW h) provides about 9.58 billion kW h of surplus electric energy in China for year 2012. Therefore, bioconversion of food waste to fertilizers and their co-products definitely contributes to a green environment as well as a sustainable economy.
9. Conclusion and future prospects
Increasing global population, demographic shifting, urbanization, economic growth, and evolution of life standards are some very important reasons for the generation of enormous amounts of food waste. It is necessary to minimize food loss to increase food safety; however, this is multifaceted as losses are found in the crop production and stock cycling process. Food waste from household ingestion contributes mainly to food loss as demonstrated in various studies. Urban waste is among the top priorities and an important challenge for authorities. Various alternate management systems such as landfilling, burning, and dumping are traditional and non-environmentally friendly methods that contribute to the toxic emissions in the atmosphere. Therefore, the use of microbial capacity to decompose organic matter is a very vibrant technique to valorize food waste. Several sources and types of food waste such as waste cooking oils, agro-industrial wastes such as vegetables, fruit, juice, wheat, rice, potato, sugarcane, bakery waste, dairy waste and different types of meat, fish and poultry wastes have been proven to be profitable sources for their use for bioconversion. Nowadays composting, anaerobic and aerobic digestion, and fermentation are the most likely utilized methods for bioconversion of food waste to biofertilizers, organic compost, biochar, and biogas.
However, scientific communities are now utilizing genetically engineered microbes for the valorization of urban waste as a valuable product. These engineering approaches include gene deletion, overexpression, modification in a targeted manner, or addition of metabolic routes.149 Similar approaches are now utilized to valorize organic waste as a valuable product. Liu et al.150 recently expressed an A. niger pectinase in Pichia pastoris to enable oligogalacturonide (OG) production directly from ground mandarin and orange peel waste. Recombinant yeast Yarrowia lipolytica was used to produce long chain fatty acid from waste.151 Grohmann et al.152 used a recombinant strain of Escherichia coli KO11 for the fermentation of sugar present in orange peel. The most recent genetically engineered strains of microorganisms have been utilized for better composting and digestion capacity for the valorization of food waste.153
Author contributions
Pramod Kumar Mahish: preparation of TOC, literature review, manuscript writing, drawing of figures. Dakeshwar Kumar Verma: initial editing, plagiarism check, supervision. Anjali Ghritlahare: manuscript writing, drawing of figures, reference arrangement, copyright. Charu Arora: conceptualization, final editing. Paz Otero: final modification, supervision.
Conflicts of interest
The authors declare no conflict of interest.
References
-
Food and Agriculture Organization, Mitigation of Food Wastage: Societal Costs and Benefits, 2014, https://www.fao.org/documents/card/en?details=a530f2ad-499c-400f-b9c0-6c8988f2ee9d/.
-
Food and Agriculture Organization, Global Food Losses and Food Waste: Extent, Causes and Prevention, 2011, https://www.fao.org/3/mb060e/mb060e00.htm.
-
United States Environmental Protection Agency, Terms of Environment: Glossary, Abbreviations and Acronyms, 2008, http://www.epa.gov/OCEPAterms/fterms.html.
-
World Food Programme, The Year in Review, 2012, https://www.wfp.org/publications/year-review-2012-0.
-
Food and Agriculture Organization, Food Wastage Footprint: Impacts on Natural Resources, 2018, https://www.fao.org/documents/card/en?details=000d4a32-7304-5785-a2f1-.
- M. Melikoglu, C. C. Lin and C. Webb, Analysing global food waste problem: Pinpointing the facts and estimating the energy content, Cent. Eur. J. Eng., 2013, 3(2), 157–164, DOI:10.2478/s13531-012-0058-5.
- J. O'Connor, B. S. Mickan, J. Rinklebe, H. Song, K. H. Siddique, H. Wang, M. B. Kirkham and N. S. Bolan, Environmental implications, potential value, and future of food-waste anaerobic digestate management: A review, J. Environ. Manage., 2022, 318, 115519, DOI:10.1016/j.jenvman.2022.115519.
- P. Alexander, C. Brown, A. Arneth, J. Finnigan, D. Moran and M. D. Rounsevell, Losses, inefficiencies and waste in the global food system, Agric. Syst., 2017, 53, 190–200, DOI:10.1016/j.agsy.2017.01.014.
- D. Tilman and M. Clark, Global diets link environmental sustainability and human health, Nature, 2014, 515, 518–522, DOI:10.1038/nature13959.
- S. D. Porter, D. S. Reay, P. Higgins and E. Bomberg, A half-century of production-phase greenhouse gas emissions from food loss & waste in the global food supply chain, Sci. Total Environ., 2016, 571, 721–729, DOI:10.1016/j.scitotenv.2016.07.041.
- C. Chen, A. Chaudhary and A. Mathys, Nutritional and environmental losses embedded in global food waste, Resour., Conserv. Recycl., 2020, 160, 104912, DOI:10.1016/j.resconrec.2020.104912.
- A. Chaudhary and T. M. Brooks, National consumption and global trade impacts on biodiversity, World Dev., 2019, 121, 178–187, DOI:10.1016/j.worlddev.2017.10.012.
- C. Beretta, F. Stoessel, U. Baier and S. Hellweg, Quantifying food losses and the potential for reduction in Switzerland, Waste Manage., 2013, 33(3), 764–773, DOI:10.1016/j.wasman.2012.11.007.
- M. Gjerris and S. Gaiani, Household food waste in Nordic countries: Estimations and ethical implications, Nord. J. Appl. Ethics, 2013, 14, 6–23, DOI:10.5324/eip.v7i1.1786.
-
Waste & Resources Action Programme, Household Food and Drink Waste: A Product Focus, 2016, https://wrap.org.uk/resources/report/household-food-drink-waste-product-focus.
-
FUSIONS, Food Waste Data Set for EU-28, 2015, https://www.wur.nl/en/Publication-details.htm?publicationId=publication-way-353033373834.
- V. Filimonau and V. A. Ermolaev, A sleeping giant? Food waste in the foodservice sector of Russia, J. Cleaner Prod., 2021, 297, 126705, DOI:10.1016/j.jclepro.2021.126705.
-
L. Xue and G. Liu, Introduction to global food losses and food waste, in Saving Food, Academic Press, 2019, pp. 1–31, DOI:10.1016/B978-0-12-815357-4.00001-8.
- S. Kirkpatrick and V. Tarasuk, The relationship between low income and household food expenditure patterns in Canada, Public Health Nutr., 2003, 6, 589–597, DOI:10.1079/PHN2003517.
- C. Dias-Ferreira, T. Santos and V. Oliveira, Hospital food waste and environmental and economic indicators–A Portuguese case study, Waste Manage., 2015, 46, 146–154, DOI:10.1016/j.wasman.2015.09.025.
- B. E. Gutiérrez-Barba and A. Ortega-Rubio, Household food-waste production and a proposal for its minimization in Mexico, Life Sci. J., 2013, 10(3), 1772–1783, DOI:10.7537/marslsj100313.266.
- S. Łaba, M. Niedek, K. Szczepański, R. Łaba and A. Kamińska-Dwórznicka, Regulation of the food waste measuring in the EU in the light of the need of counteracting the food wastage, Environ. Nat. Resour., 2019, 4(82), 1–6, DOI:10.2478/oszn-2019-0015.
- M. Boulet, A. C. Hoek and R. Raven, Towards a multi-level framework of household food waste and consumer behavior: Untangling spaghetti soup, Appetite, 2021, 156, 104856, DOI:10.1016/j.appet.2020.104856.
- M. Story, K. M. Kaphingst, R. Robinson-O'Brien and K. Glanz, Creating healthy food and eating environments: policy and environmental approaches, Annu. Rev. Public Health, 2008, 29(1), 253–272, DOI:10.1146/annurev.publhealth.29.020907.090926.
- A. Scott, C. Oates and W. Young, Conceptual framework of the adoption and practice of environmental actions in households, Sustain, 2015, 7(5), 5793–5818, DOI:10.3390/su7055793.
-
D. R. Forsyth, Group Dynamics, Wadsworth, 2012 Search PubMed.
- M. Griffin, J. Sobal and T. A. Lyson, An analysis of a community food waste stream, Agric. Human Values, 2009, 26, 67–81, DOI:10.1007/s10460-008-9178-1.
- L. Manzocco, M. Alongi, S. Sillani and M. C. Nicoli, Technological and consumer strategies to tackle food wasting, Food Eng. Rev., 2016, 8, 457–467, DOI:10.1007/s12393-016-9149-z.
- L. Xue, G. Liu, J. Parfitt, X. Liu, E. Van Herpen, Å. Stenmarck, C. O'Connor, K. Östergren and S. Cheng, Missing food, missing data? A critical review of global food losses and food waste data, Environ. Sci. Technol., 2017, 51(12), 6618–6633, DOI:10.1021/acs.est.7b00401.
- T. N. B. Dung, B. Sen, C. C. Chen, G. Kumar and C. Y. Lin, Food waste to bioenergy via anaerobic processes, Energy Procedia, 2014, 61, 307–312, DOI:10.1016/j.egypro.2014.11.1113.
- J. A. Aitken, B. Alaybek, R. Hartman, G. Mika, E. M. Broad Leib, R. Plekenpol, K. Branting, D. Rao, L. Leets and A. Sprenger, Initial assessment of the efficacy of food recovery policies in US States for increasing food donations and reducing waste, Waste Manage., 2024, 176, 149–158, DOI:10.1016/j.wasman.2023.12.051.
- A. Georganas, E. Giamouri, A. C. Pappas, G. Papadomichelakis, F. Galliou, T. Manios, E. Tsiplakou, K. Fegeros and G. Zervas, Bioactive Compounds in Food Waste: A Review on the Transformation of Food Waste to Animal Feed, Foods, 2020, 9, 291, DOI:10.3390/foods9030291.
- S. Elkhalifa, T. Al-Ansari, H. R. Mackey and G. McKay, Food waste to biochars through pyrolysis: A review, Resour., Conserv. Recycl., 2019, 144, 310–320, DOI:10.1016/j.resconrec.2019.01.024.
- F. H. Pour and Y. T. Makkawi, A review of post-consumption food waste management and its potentials for biofuel production, Energy Rep., 2021, 7, 7759–7784, DOI:10.1016/j.egyr.2021.10.119.
- H. A. Rahman, Incinerator in Malaysia: really needs?, Int. J. Chem. Environ. Biol. Sci., 2013, 1, 678–681 Search PubMed.
- R. Muangrat, A review: utilization of food wastes for hydrogen production under hydrothermal gasification, Environ. Technol. Rev., 2013, 2(1), 85–100, DOI:10.1080/21622515.2013.840682.
- B. Kayode and A. Hart, An overview of transesterification methods for producing biodiesel from waste vegetable oils, Biofuels, 2019, 10(3), 419–437, DOI:10.1080/17597269.2017.1306683.
- F. Moazeni, Y. C. Chen and G. Zhang, Enzymatic transesterification for biodiesel production from used cooking oil, a review, J. Cleaner Prod., 2019, 216, 117–128, DOI:10.1016/j.jclepro.2019.01.181.
- S. Fan, A. Li, A. ter Heijne, C. J. Buisman and W. S. Chen, Heat potential, generation, recovery and utilization from composting: A review, Resour., Conserv. Recycl., 2021, 175, 105850, DOI:10.1016/j.resconrec.2021.105850.
- C. Jin, S. Sun, D. Yang, W. Sheng, Y. Ma, W. He and G. Li, Anaerobic digestion: An alternative resource treatment option for food waste in China, Sci. Total Environ., 2021, 779, 146397, DOI:10.1016/j.scitotenv.2021.146397.
- M. Y. Areeshi, Recent advances on organic biofertilizer production from anaerobic fermentation of food waste: Overview, Int. J. Food Microbiol., 2022, 374, 109719, DOI:10.1016/j.ijfoodmicro.2022.109719.
- S. J. Chen, X. Chen, B. B. Hu, M. Y. Wei and M. J. Zhu, Efficient hydrogen production from sugarcane bagasse and food waste by thermophilic clostridiales consortium and Fe–Mn impregnated biochars, Renewable Energy, 2023, 211, 166–178, DOI:10.1016/j.renene.2023.04.114.
- X. Xiong, I. K. M. Yu, D. C. W. Tsang, N. S. Bolan, Y. Sik Ok, A. D. Igalavithana, M. B. Kirkham, K.-H. Kim and K. Vikrant, Value-added chemicals from food supply chain wastes: State-of-the-art review and future prospects, Chem. Eng. J., 2019, 375, 121983, DOI:10.1016/j.cej.2019.121983.
- N. B. D. Thi, G. Kumar and C.-Y. Lin, An overview of food waste management in developing countries: Current status and future perspective, J. Environ. Manage., 2015, 157, 220–229, DOI:10.1016/j.jenvman.2015.04.022.
- K. M. Kibler, D. Reinhart, C. Hawkins, A. M. Motlagh and J. Wright, Food waste and the food-energy-water nexus: A review of food waste management alternatives, Waste Manage., 2018, 74, 52–62, DOI:10.1016/j.wasman.2018.01.014.
-
S. Zafar, Food Waste to Energy Plant | BioEnergy Consult, https://www.bioenergyconsult.com/tag/food-waste-to-energy-plant, (accessed September 3, 2023).
-
T. A. A. Nasrin and M. A. Matin, Valorization of Vegetable Wastes, in Food Processing By-Products and Their Utilization, John Wiley & Sons, Ltd, Chichester, UK, 2017, pp. 53–88, DOI:10.1002/9781118432921.ch4.
- M. Sharma, Z. Usmani, V. K. Gupta and R. Bhat, Valorization of fruits and vegetable wastes and by-products to produce natural pigments, Crit. Rev. Biotechnol., 2021, 41, 535–563, DOI:10.1080/07388551.2021.1873240.
- A. Mannu, G. Vlahopoulou, P. Urgeghe, M. Ferro, A. Del Caro, A. Taras, S. Garroni, J. P. Rourke, R. Cabizza and G. L. Petretto, Variation of the chemical composition of waste cooking oils upon bentonite filtration, Resources, 2019, 8, 108, DOI:10.3390/resources8020108.
- Sahar, S. Sadaf, J. Iqbal, I. Ullah, H. N. Bhatti, S. Nouren, Habib-ur-Rehman, J. Nisar and M. Iqbal, Biodiesel production from waste cooking oil: an efficient technique to convert waste into biodiesel, Sustain. Cities Soc., 2018, 41, 220–226, DOI:10.1016/j.scs.2018.05.037.
- T. Yuwa-amornpitak and K. Chookietwatana, Bioconversion of waste cooking oil glycerol from cabbage extract to lactic acid by Rhizopus microspores, Braz. J. Microbiol., 2018, 49, 178–184, DOI:10.1016/j.bjm.2018.06.007.
- A. H. Hashem, A. M. Khattab and M. Abdelraof, A facile one-pot bioconversion of frying oil waste to single cell oils and related products using fungi via response surface methodology, Biomass Convers. Biorefin., 2022, 13, 16711–16721, DOI:10.1007/s13399-021-02165-7.
- P. Kumar and B. S. Kim,
Paracoccus sp. strain LL1 as a single cell factory for the conversion of waste cooking oil to polyhydroxyalkanoates and carotenoids, Appl. Food Biotechnol., 2019, 6(1), 53–60, DOI:10.22037/afb.v6i1.21628.
- Y. Li, Z. Cheng, C. Zhao, C. Gao, W. Song, L. Liu and X. Chen, Reprogramming Escherichia coli metabolism for bioplastics synthesis from waste cooking oil, ACS Synth. Biol., 2021, 10, 1966–1979, DOI:10.1021/acssynbio.1c00155.
- M. A. Martín, J. A. Siles, A. F. Chica and A. Martín, Biomethanization of orange peel waste, Bioresour. Technol., 2010, 101, 8993–8999, DOI:10.1016/j.biortech.2010.06.133.
- A. Upadhyay, J. P. Lama and S. Tawata, Utilization of pineapple waste: a review, J. Food Sci. Technol., 2013, 6, 10–18, DOI:10.3126/jfstn.v6i0.8255.
- J. K. Bediako, A. K. Sarkar, S. Lin, Y. Zhao, M.-H. Song, J.-W. Choi, C.-W. Cho and Y.-S. Yun, Characterization of the residual biochemical components of sequentially extracted banana peel biomasses and their environmental remediation applications, Waste Manage., 2019, 89, 141–153, DOI:10.1016/j.wasman.2019.04.009.
- M. Xu, G. Tian, C. Zhao, A. Ahmad, H. Zhang, J. Bi, H. Xiao and J. Zheng, Infrared drying as a quick preparation method for dried tangerine peel, Int. J. Anal. Chem., 2017, 1–11, DOI:10.1155/2017/6254793.
- P. Kandylis and E. Kokkinomagoulos, Food applications and potential health benefits of pomegranate and its derivatives, Foods, 2020, 9, 122, DOI:10.3390/foods9020122.
- A. El Barnossi, F. Moussaid and A. Iraqi Housseini, Tangerine, banana and pomegranate peels valorisation for sustainable environment: A review, Biotechnol. Rep., 2021, 29, e00574, DOI:10.1016/j.btre.2020.e00574.
- L. A. d. Santos, R. B. Valença, L. C. S. d. Silva, S. H. d. B. Holanda, A. F. V. d. Silva, J. F. T. Jucá and A. F. M. S. Santos, Methane generation potential through anaerobic digestion of fruit waste, J. Cleaner Prod., 2020, 256, 120389, DOI:10.1016/j.jclepro.2020.120389.
- M. Ververi and A. M. Goula, Pomegranate peel and orange juice by-product as new biosorbents of phenolic compounds from olive mill wastewaters, Chem. Eng. Process., 2019, 138, 86–96, DOI:10.1016/j.cep.2019.03.010.
- W. Miran, M. Nawaz, J. Jang and D. S. Lee, Conversion of orange peel waste biomass to bioelectricity using a mediator-less microbial fuel cell, Sci. Total Environ., 2016, 547, 197–205, DOI:10.1016/j.scitotenv.2016.01.004.
- A. Kantifedaki, V. Kachrimanidou, A. Mallouchos, S. Papanikolaou and A. A. Koutinas, Orange processing waste valorisation for the production of bio-based pigments using the fungal strains Monascus purpureus and Penicillium purpurogenum, J. Cleaner Prod., 2018, 185, 882–890, DOI:10.1016/j.jclepro.2018.03.032.
- M. Nossier, Impact of organic fertilizers derived from banana and orange peels on tomato plant quality, Arab Univ. J. Agric. Sci., 2021, 29(1), 459–469, DOI:10.21608/ajs.2021.46495.1278.
- T. A. Sial, M. N. Khan, Z. Lan, F. Kumbhar, Z. Ying, J. Zhang, D. Sun and X. Li, Contrasting effects of banana peels waste and its biochar on greenhouse gas emissions and soil biochemical properties, Process Saf. Environ. Prot., 2019, 122, 366–377, DOI:10.1016/j.psep.2018.10.030.
- S. Chaturvedi, A. Kumari, A. Bhatacharya, A. Sharma, L. Nain and S. K. Khare, Banana peel waste management for single-cell oil production, Energy, Ecol. Environ., 2018, 3, 296–303, DOI:10.1007/s40974-018-0101-3.
- M. A. d. Souza, I. T. Vilas-Boas, J. M. Leite-da-Silva, P. d. N. Abrahão, B. E. Teixeira-Costa and V. F. Veiga-Junior, Polysaccharides in Agro-Industrial Biomass Residues, Polysaccharides, 2022, 3, 95–120, DOI:10.3390/polysaccharides3010005.
-
C. M. Hussain and R. K. Kadeppagari, Biotechnology for Zero Waste: Emerging Waste Management Techniques, Wiley & Sons, Limited, John, 2021 Search PubMed.
- R. Ravindran, S. Hassan, G. Williams and A. Jaiswal, A review on bioconversion of agro-industrial wastes to industrially important enzymes, Bioengineering, 2018, 5(4), 93, DOI:10.3390/bioengineering5040093.
- A. Singh, S. Bajar, A. Devi and N. R. Bishnoi, Adding value to agro-industrial waste for cellulase and xylanase production via solid-state bioconversion, Biomass Convers. Biorefin., 2021, 1–10, DOI:10.1007/s13399-021-01503-z.
- R. Rajesh and S. N. Gummadi, Production of multienzymes, bioethanol, and acetic acid by novel Bacillus sp. PM06 from various lignocellulosic biomass, Biomass Convers. Biorefin., 2022 DOI:10.1007/s13399-022-02418-z.
- T. Akhtar, A. S. Hashmi, M. Tayyab, A. A. Anjum, S. Saeed and S. Ali, Bioconversion of agricultural waste to butyric acid through solid state fermentation by Clostridium tyrobutyricum, Waste Biomass Valorization, 2018, 11, 2067–2073, DOI:10.1007/s12649-018-0475-7.
- P. Upadhyay, N. K. Singh, R. Tupe, A. Odenath and A. Lali, Biotransformation of corn bran derived ferulic acid to vanillic acid using engineered Pseudomonas putida KT2440, Prep. Biochem. Biotechnol., 2019, 50, 341–348, DOI:10.1080/10826068.2019.1697935.
- T. Agrawal, S. K. Jadhav and A. Quraishi, Bioethanol production from an agro-waste, deoiled rice bran by Saccharomyces cerevisiae MTCC 4780 via optimization of fermentation parameters, EnvironmentAsia, 2019, 12, 20–24, DOI:10.14456/ea.2019.3.
-
A. Kosre, D. Koreti, P. K. Mahish and N. K. Chandrawanshi, Current perspective of sustainable utilization of agro waste and biotransformation of energy in mushroom, in Energy: Crises, Challenges and Solutions, John Wiley & Sons, Inc., Hoboken, New Jersey, 2021, p. 352 Search PubMed.
- N. V. Hoang, A. Furtado, L. Donnan, E. C. Keeffe, F. C. Botha and R. J. Henry, High-throughput profiling of the fiber and sugar composition of sugarcane biomass, BioEnergy Res., 2016, 10, 400–416, DOI:10.1007/s12155-016-9801-8.
- M. Leonel, E. L. do Carmo, A. M. Fernandes, R. P. Soratto, J. A. M. Ebúrneo, É. L. Garcia and T. P. R. dos Santos, Chemical composition of potato tubers: the effect of cultivars and growth conditions, J. Food Sci. Technol., 2017, 54, 2372–2378, DOI:10.1007/s13197-017-2677-6.
- K. C. Khaire, V. S. Moholkar and A. Goyal, Bioconversion of sugarcane tops to bioethanol and other value-added products: an overview, Mater. Sci. Energy Technol., 2021, 4, 54–68, DOI:10.1016/j.mset.2020.12.004.
- S. Chantorn, C. Piyapittayanun and P. Dangpram, Bioconversion of agricultural wastes to mannooligosaccharides and their prebiotic potential, Chiang Mai J. Sci., 2018, 45(1), 60–67 CAS , http://epg.science.cmu.ac.th/ejournal/.
- R. Terán Hilares, R. A. de Souza, P. F. Marcelino, S. S. da Silva, G. Dragone, S. I. Mussatto and J. C. Santos, Sugarcane bagasse hydrolysate as a potential feedstock for red pigment production by Monascus ruber, Food Chem., 2018, 245, 786–791, DOI:10.1016/j.foodchem.2017.11.111.
- J. de Oliveira, L. Porto de Souza Vandenberghe, P. Zwiercheczewski de Oliveira, A. Fátima Murawski de Mello, C. Rodrigues, P. Singh Nigam, V. Faraco and C. R. Soccol, Bioconversion of potato-processing wastes into an industrially-important chemical lactic acid, Bioresour. Technol. Rep., 2021, 15, 100698, DOI:10.1016/j.biteb.2021.100698.
- S. S. Suryawanshi, S. S. Sarje, P. C. Loni, S. bhujbal and P. P. Kamble, Bioconversion of sugarcane molasses into bioplastic (polyhydroxybutyrate) using Bacillus cereus 2156 under statistically optimized culture conditions, Anal. Chem. Lett., 2020, 10, 80–92, DOI:10.1080/22297928.2020.1746197.
- R. Jumaidin, S. N. Mohd Zainel, N. W. Adam, M. S. F. Hussin, A. F. Ab Ghani, M. Mohammad Taha, M. R. Mansor, M. Y. Yaakob, M. N. Ahmad, N. A. Maidin, M. K. Wahid, N. A. Mohamad Yatim, M. H. Ab Rahman and M. H. Osman, Thermal Degradation and Mechanical Characteristics of Sugarcane Bagasse Reinforced Biodegradable Potato Starch
Composites, J. Adv. Res. Fluid Mech. Therm. Sci., 2020, 78, 157–166, DOI:10.37934/arfmts.78.1.157166.
- T. C. Sarker, M. A. Mannan, P. C. Mondol, A. H. Kabir, S. M. Parvez and M. F. Alam, Physico-chemical profile and microbial diversity during bioconversion of sugarcane press mud using bacterial suspension, Not. Sci. Biol., 2013, 5, 346–353, DOI:10.15835/nsb539080.
- A. Y.-z. Zhang, Z. Sun, C. C. J. Leung, W. Han, K. Y. Lau, M. Li and C. S. K. Lin, Valorisation of bakery waste for succinic acid production, Green Chem., 2013, 15, 690, 10.1039/c2gc36518a.
- O. Benabda, M. Kasmi, F. Kachouri and M. Hamdi, Valorization of the powdered bread waste hydrolysate as growth medium for baker yeast, Food Bioprod. Process., 2018, 109, 1–8, DOI:10.1016/j.fbp.2018.02.007.
- R. A. D. Arancon, C. S. K. Lin, K. M. Chan, T. H. Kwan and R. Luque, Advances on waste valorization: new horizons for a more sustainable society, Energy Sci. Eng., 2013, 1, 53–71, DOI:10.1002/ese3.9.
- M. A. Haque, V. Kachrimanidou, A. Koutinas and C. S. K. Lin, Valorization of bakery waste for biocolorant and enzyme production by Monascus purpureus, J. Biotechnol., 2016, 231, 55–64, DOI:10.1016/j.jbiotec.2016.05.003.
- A. Cerda, M. El-Bakry, T. Gea and A. Sánchez, Long term enhanced solid-state fermentation: inoculation strategies for amylase production from soy and bread wastes by Thermomyces sp. in a sequential batch operation, J. Environ. Chem. Eng., 2016, 4, 2394–2401, DOI:10.1016/j.jece.2016.04.022.
- F. Ebrahimi, M. Khanahmadi, S. Roodpeyma and M. Taherzadeh, Ethanol production from bread residues, Biomass Bioenergy, 2008, 32, 333–337, DOI:10.1016/j.biombioe.2007.10.007.
- A. Adessi, M. Venturi, F. Candeliere, V. Galli, L. Granchi and R. De Philippis, Bread wastes to energy: sequential lactic and photo-fermentation for hydrogen production, Int. J. Hydrogen Energy, 2018, 43, 9569–9576, DOI:10.1016/j.ijhydene.2018.04.053.
- M. Romero-Huelva, E. Ramos-Morales and E. Molina-Alcaide, Nutrient utilization, ruminal fermentation, microbial abundances, and milk yield and composition in dairy goats fed diets including tomato and cucumber waste fruits, J. Dairy Sci., 2012, 95, 6015–6026, DOI:10.3168/jds.2012-5573.
- S. Roufou, S. Griffin, L. Katsini, M. Polańska, J. F. M. Van Impe and V. P. Valdramidis, The (potential) impact of seasonality and climate change on the physicochemical and microbial properties of dairy waste and its management, Trends Food Sci. Technol., 2021, 116, 1–10, DOI:10.1016/j.tifs.2021.07.008.
- J. Liu, S. H. Dantoft, A. Würtz, P. R. Jensen and C. Solem, A novel cell factory for efficient production of ethanol from dairy waste, Biotechnol. Biofuels, 2016, 9(1), 1–11, DOI:10.1186/s13068-016-0448-7.
- M. Kasmi, Biological processes as promoting way for both treatment and valorization of dairy industry effluents, Waste Biomass Valorization, 2016, 9, 195–209, DOI:10.1007/s12649-016-9795-7.
- R. Kothari, R. Prasad, V. Kumar and D. P. Singh, Production of biodiesel from microalgae Chlamydomonas polypyrenoideum grown on dairy industry wastewater, Bioresour. Technol., 2013, 144, 499–503, DOI:10.1016/j.biortech.2013.06.116.
- Z. Usmani, M. Sharma, J. Gaffey, M. Sharma, R. J. Dewhurst, B. Moreau, J. Newbold, W. Clark, V. K. Thakur and V. K. Gupta, Valorization of dairy waste and by-products through microbial bioprocesses, Bioresour. Technol., 2022, 346, 126444, DOI:10.1016/j.biortech.2021.126444.
- M. Babu, S. P. Raj, C. B. Nirmala, M. Deccaraman and E. Sagadevan, Production of Single Cell Protein using Kluyveromyces marxianus isolated from paneer whey, Int. J. Biomed. Adv. Res., 2014, 5(05), 255–257 Search PubMed.
- K. u. Rehman, R. Ur Rehman, A. A. Somroo, M. Cai, L. Zheng, X. Xiao, A. Ur Rehman, A. Rehman, J. K. Tomberlin, Z. Yu and J. Zhang, Enhanced bioconversion of dairy and chicken manure by the interaction of exogenous bacteria and black soldier fly larvae, J. Environ. Manage., 2019, 237, 75–83, DOI:10.1016/j.jenvman.2019.02.048.
- M. Gogoi, S. Banerjee, S. Pati and S. Ray Chaudhuri, Microbial Bioconversion of Dairy Wastewater in Packed Bed Biofilm Reactor into Liquid Biofertilizer, Geomicrobiol. J., 2022, 39(3–5), 249–258, DOI:10.1080/01490451.2021.1980921.
- A. K. Rai, H. C. Swapna, N. Bhaskar and V. Baskaran, Potential of seafood industry by products as sources of recoverable lipids: Fatty acid composition of meat and nonmeat component of selected Indian marine fishes, J. Food Biochem., 2011, 36, 441–448, DOI:10.1111/j.1745-4514.2011.00549.x.
- A. Shabani, F. Boldaji, B. Dastar, T. Ghoorchi and S. Zerehdaran, Preparation of fish waste silage and its effect on the growth performance and meat quality of broiler chickens, J. Sci. Food Agric., 2018, 98, 4097–4103, DOI:10.1002/jsfa.8926.
- K. Jayathilakan, K. Sultana, K. Radhakrishna and A. S. Bawa, Utilization of by products and waste materials from meat, poultry and fish processing industries: a review, J. Food Sci. Technol., 2011, 49, 278–293, DOI:10.1007/s13197-011-0290-7.
- F. Toldrá, L. Mora and M. Reig, New insights into meat by-product utilization, Meat Sci., 2016, 120, 54–59, DOI:10.1016/j.meatsci.2016.04.021.
- D. Vamvuka, S. Dermitzakis, D. Pentari and S. Sfakiotakis, Valorization of meat and bone meal through pyrolysis for soil amendment or lead adsorption from wastewaters, Food Bioprod. Process., 2018, 109, 148–157, DOI:10.1016/j.fbp.2018.04.002.
- A. Lasekan, F. Abu Bakar and D. Hashim, Potential of chicken by-products as sources of useful biological resources, Waste Manage., 2013, 33(3), 552–565, DOI:10.1016/j.wasman.2012.08.001.
- U. u. Rahman, A. Sahar and M. A. Khan, Recovery and utilization of effluents from meat processing industries, Food Res. Int., 2014, 65, 322–328, DOI:10.1016/j.foodres.2014.09.026.
- F. Z. Ozi, N. Boutaleb, M. Hadidi, B. Bahlaouan, M. Bennani, A. Silkina and S. El Antri, Production of bio-fertilizer by biotransformation of poultry waste enriched with molasses and algae, Environ. Qual. Manag., 2022, 32(3), 123–134, DOI:10.1002/tqem.21868.
- A. Saeid, M. Labuda, K. Chojnacka and H. Górecki, Valorization of bones to liquid phosphorus fertilizer by microbial solubilization, Waste Biomass Valorization, 2013, 5, 265–272, DOI:10.1007/s12649-013-9238-7.
- J. S. Lamba, M. Wadhwa and M. P. S. Bakshi, Methane Production Potential of Fruit and Vegetable Wastes in Vitro, Anim. Nutr. Feed Technol., 2016, 16, 363–372, DOI:10.5958/0974-181x.2016.00032.9.
- N. G. Das, K. S. Huque, S. M. Amanullah, S. Dharmapuri and H. P. S. Makkar, Study of chemical composition and nutritional values of vegetable wastes in Bangladesh, Vet. Anim. Sci., 2018, 5, 31–37, DOI:10.1016/j.vas.2018.02.003.
- C. Fritsch, A. Staebler, A. Happel, M. Cubero Márquez, I. Aguiló-Aguayo, M. Abadias, M. Gallur, I. Cigognini, A. Montanari, M. López, F. Suárez-Estrella, N. Brunton, E. Luengo, L. Sisti, M. Ferri and G. Belotti, Processing, valorization and application of bio-waste derived compounds from potato, tomato, olive and cereals: A review, Sustainability, 2017, 9, 1492, DOI:10.3390/su9081492.
- C. Sabater, L. Ruiz, S. Delgado, P. Ruas-Madiedo and A. Margolles, Valorization of vegetable food waste and by-products through fermentation processes, Front. Microbiol., 2020, 11, 581997, DOI:10.3389/fmicb.2020.581997.
- N. Jiménez-Moreno, I. Esparza, F. Bimbela, L. M. Gandía and C. Ancín-Azpilicueta, Valorization of selected fruit and vegetable wastes as bioactive compounds: Opportunities and challenges, Crit. Rev. Environ. Sci. Technol., 2019, 50, 2061–2108, DOI:10.1080/10643389.2019.1694819.
- A. Saini, P. S. Panesar and M. B. Bera, Valorization of fruits and vegetables waste through green extraction of bioactive compounds and their nanoemulsions-based delivery system, Bioresour. Bioprocess., 2019, 6, 26, DOI:10.1186/s40643-019-0261-9.
- B. Bayram, G. Ozkan, T. Kostka, E. Capanoglu and T. Esatbeyoglu, Valorization and application of fruit and vegetable wastes and by-products for food packaging materials, Molecules, 2021, 26(13), 4031, DOI:10.3390/molecules26134031.
- S. K. Panda, R. C. Ray, S. S. Mishra and E. Kayitesi, Microbial processing of fruit and vegetable wastes into potential biocommodities: a review, Crit. Rev. Biotechnol., 2017, 38, 1–16, DOI:10.1080/07388551.2017.1311295.
- I. Chakravarty and S. A. Mandavgane, Valorization of fruit and vegetable waste for biofertilizer and biogas, J. Food Process Eng., 2020, 44(2), e13512, DOI:10.1111/jfpe.13512.
- A. El Barnossi, F. Moussaid and A. Iraqi Housseini, Quantitative Research of Systematic and Functional Microbial Groups Associated with Decaying Solid Green Household Waste in Water and Soil, Pol. J. Environ. Stud., 2020, 29, 2631–2639, DOI:10.15244/pjoes/112365.
- S. Dantroliya, C. Joshi, A. Mohapatra, D. Shah, P. Bhargava, S. Bhanushali, R. Pandit, C. Joshi and M. Joshi, Creating wealth from waste: An approach for converting organic waste in to value-added products using microbial consortia, Environ. Technol. Innovation, 2022, 25, 102092, DOI:10.1016/j.eti.2021.102092.
- J. O’Connor, S. A. Hoang, L. Bradney, S. Dutta, X. Xiong, D. C. W. Tsang, K. Ramadass, A. Vinu, M. B. Kirkham and N. S. Bolan, A review on the valorisation of food waste as a nutrient source and soil amendment, Environ. Pollut., 2021, 272, 115985, DOI:10.1016/j.envpol.2020.115985.
- J. O'Connor, S. A. Hoang, L. Bradney, J. Rinklebe, M. B. Kirkham and N. S. Bolan, Value of dehydrated food waste fertiliser products in increasing soil health and crop productivity, Environ. Res., 2022, 204, 111927, DOI:10.1016/j.envres.2021.111927.
- A. Mahmood, R. Iguchi and R. Kataoka, Multifunctional food waste fertilizer having the capability of Fusarium-growth inhibition and phosphate solubility: A new horizon of food waste recycle using microorganisms, Waste Manage., 2019, 94, 77–84, DOI:10.1016/j.wasman.2019.05.046.
- S. O. Dahunsi, S. Oranusi, V. E. Efeovbokhan, A. T. Adesulu-Dahunsi and J. O. Ogunwole, Crop performance and soil fertility improvement using organic fertilizer produced from valorization of Carica papaya fruit peel, Sci. Rep., 2021, 11, 4696, DOI:10.1038/s41598-021-84206-9.
- Y. Li, T. Cui, Y. Wang and X. Ge, Isolation and characterization of a novel bacterium Pseudomonas aeruginosa for biofertilizer production from kitchen waste oil, RSC Adv., 2018, 8(73), 41966–41975, 10.1039/c8ra09779h.
- J.-X. Lai, X. Chen, J. Bu, B.-B. Hu and M.-J. Zhu, Direct production of astaxanthin from food waste by Phaffia rhodozyma, Process Biochem., 2022, 113, 224–233, DOI:10.1016/j.procbio.2022.01.003.
- J. Lai, W. Liu, J. Bu, X. Chen, B. Hu and M. Zhu, Enhancement of astaxanthin production from food waste by Phaffia rhodozyma screened by flow cytometry and feed application potential, Biotechnol. Appl. Biochem., 2023, 70, 1817–1829, DOI:10.1002/bab.2484.
- H. I. Owamah, S. O. Dahunsi, U. S. Oranusi and M. I. Alfa, Fertilizer and sanitary quality of digestate biofertilizer from the co-digestion of food waste and human excreta, Waste Manage., 2014, 34, 747–752, DOI:10.1016/j.wasman.2014.01.017.
- X. Wang, A. Selvam and J. W. C. Wong, Influence of lime on struvite formation and nitrogen conservation during food waste composting, Bioresour. Technol., 2016, 217, 227–232, DOI:10.1016/j.biortech.2016.02.117.
- E. Sufficiency, S. A. Qamar, L. F. R. Ferreira, M. Franco, H. M. N. Iqbal and M. Bilal, Emerging biotechnological strategies for food waste management: A green leap towards achieving high-value products and environmental abatement, Energy Nexus, 2022, 6, 100077, DOI:10.1016/j.nexus.2022.100077.
- S.-H. Tsai, C.-P. Liu and S.-S. Yang, Microbial conversion of food wastes for biofertilizer production with thermophilic lipolytic microbes, Renewable Energy, 2007, 32, 904–915, DOI:10.1016/j.renene.2006.04.019.
- K. M. Sangamithirai, J. Jayapriya, J. Hema and R. Manoj, Evaluation of in-vessel co-composting of yard waste and development of kinetic models for co-composting, Int. J. Recycl. Org. Waste Agric., 2015, 4, 157–165, DOI:10.1007/s40093-015-0095-1.
- C. Lin, A negative-pressure aeration system for composting food wastes, Bioresour. Technol., 2008, 99(16), 7651–7656, DOI:10.1016/j.biortech.2008.01.078.
- O. Stabnikova, H.-B. Ding, J.-H. Tay and J.-Y. Wang, Biotechnology for aerobic conversion of food waste into organic fertilizer, Waste Manage. Res., 2005, 23(1), 39–47, DOI:10.1177/0734242x05049768.
- M. Jiang, W. Qiao, Y. Wang, T. Zou, M. Lin and R. Dong, Balancing acidogenesis and methanogenesis metabolism in thermophilic anaerobic digestion of food waste under a high loading rate, Sci. Total Environ., 2022, 824, 153867, DOI:10.1016/j.scitotenv.2022.153867.
- D. Dadi, G. Daba, A. Beyene, P. Luis and B. Van der Bruggen, Composting and co-composting of coffee husk and pulp with source-separated municipal solid waste: a breakthrough in valorization of coffee waste, Int. J. Recycl. Org. Waste Agric., 2019, 8, 263–277, DOI:10.1007/s40093-019-0256-8.
- T. Hyun Chung and B. Ranjan Dhar, A multi-perspective review on microbial electrochemical technologies for food waste valorization, Bioresour. Technol., 2021, 125950, DOI:10.1016/j.biortech.2021.125950.
-
T. D. Evans, M. Boor and D. MacBrayne, Presented in Part at the 12th CIWEM European Biosolids & Biowastes Conference, West Yorkshire, 2007 Search PubMed.
- X. Xin, Y. Ma and Y. Liu, Electric energy production from food waste: Microbial fuel cells versus anaerobic digestion, Bioresour. Technol., 2018, 255, 281–287, DOI:10.1016/j.biortech.2018.01.099.
-
O. P. Karthikeyan, S. Mehariya and J. W. Wong, Presented in Part at the 4th International Conference on Energy and Environment Research (ICEER), Porto, Portugal, 2017 Search PubMed.
- C. Du, J. J. Abdullah, D. Greetham, D. Fu, M. Yu, L. Ren, S. Li and D. Lu, Valorization of food waste into bio-fertilizer and its field application, J. Cleaner Prod., 2018, 187, 273–284, DOI:10.1016/j.jclepro.2018.03.211.
- O. Oyetunji, N. Bolan and G. Hancock, comprehensive review on enhancing nutrient use efficiency and productivity of broadacre (arable) crops with the combined utilization of compost and fertilizers, J. Environ. Manage., 2022, 317, 115395, DOI:10.1016/j.jenvman.2022.115395.
- L. Zhu, X. Jia, M. Li, Y. Wang, J. Zhang, J. Hou and X. Wang, Associative effectiveness of bio-organic fertilizer and soil conditioners derived from the fermentation of food waste applied to greenhouse saline soil in Shan Dong Province, China, Appl. Soil Ecol., 2021, 167, 104006, DOI:10.1016/j.apsoil.2021.104006.
- H.-L. Xu, R. Wang and M. A. U. Mridha, Effects of organic fertilizers and a microbial inoculant on leaf photosynthesis and fruit yield and quality of tomato plants, J. Crop Prod., 2001, 3(1), 173–182, DOI:10.1300/j144v03n01_15.
- J. Södergren, C. U. Larsson, L. Wadsö, K.-J. Bergstrand, H. Asp, M. Hultberg and J. Schelin, Food waste to new food: Risk assessment and microbial community analysis of anaerobic digestate as a nutrient source in hydroponic production of vegetables, J. Cleaner Prod., 2022, 333, 130239, DOI:10.1016/j.jclepro.2021.130239.
- Z. J. Yong, M. J. K. Bashir and M. S. Hassan, Biogas and biofertilizer production from organic fraction municipal solid waste for sustainable circular economy and environmental protection in Malaysia, Sci. Total Environ., 2021, 776, 145961, DOI:10.1016/j.scitotenv.2021.145961.
- Y. Ma and Y. Liu, Turning food waste to energy and resources towards a great environmental and economic sustainability: An innovative integrated biological approach, Biotechnol. Adv., 2019, 37, 107414, DOI:10.1016/j.biotechadv.2019.06.013.
- S. Cheon, H. M. Kim, M. Gustavsson and S. Y. Lee, Recent trends in metabolic engineering of microorganisms for the production of advanced biofuels, Curr. Opin. Chem. Biol., 2016, 35, 10–21, DOI:10.1016/J.CBPA.2016.08.003.
- M.-Q. Liu, X.-J. Dai, L.-F. Bai and X. Xu, Cloning, expression of Aspergillus niger JL-15 endo-polygalacturonase A gene in Pichia pastoris and oligo-galacturonates production, Protein Expression Purif., 2014, 94, 53–59, DOI:10.1016/j.pep.2013.10.025.
- P. Gajdoš, J. Hambalko, O. Slaný and M. Čertík, Conversion of waste materials into very long chain fatty acids by the recombinant yeast Yarrowia lipolytica, FEMS Microbiol. Lett., 2020, 367(6), fnaa042, DOI:10.1093/femsle/fnaa042.
-
K. Grohmann, R. G. Cameron and B. S. Buslig, Fermentation of Orange Peel Hydrolysates by Ethanologenic Escherichia coli, Humana Press, Totowa, NJ, 1996 Search PubMed.
- I. Ben Tahar and P. Fickers, Metabolic engineering of microorganisms for urban waste valorization, Case Stud. Chem. Environ. Eng., 2021, 4, 100148, DOI:10.1016/j.cscee.2021.100148.
|
This journal is © The Royal Society of Chemistry 2024 |
Click here to see how this site uses Cookies. View our privacy policy here.