DOI:
10.1039/D4AY00814F
(Paper)
Anal. Methods, 2024,
16, 3847-3858
Determination of 3-acetyl-11-keto-β-boswellic acid in analytical and biological samples using streamlined and robust RP-HPLC method†
Received
3rd May 2024
, Accepted 1st June 2024
First published on 6th June 2024
Abstract
AKBA (3-acetyl-11-keto-β-boswellic acid) is a phytoconstituent derived from Boswellia serrata extract and utilized in the management of rheumatoid arthritis. Drug delivery approaches showed interest in delivering AKBA with advanced nanotechnology. There is a need for a simple, sensitive, and robust HPLC method that can determine AKBA in complex nanoformulation and in vitro and ex vivo samples. In the proposed work, the RP-HPLC method was developed using a mobile phase comprising a mixture of acetonitrile
:
milli Q (90
:
10) at detection λmax 250 nm. The method exhibited a linearity of 250 to 20
000 ng mL−1 with a high correlation coefficient of 1. The limit of detection and limit of quantification for the analytes were found to be 41.32 ng mL−1 and 125.21 ng mL−1, respectively. In the accuracy study, the % recovery of AKBA was found to be 98% to 102%, and the precision study showed less than 2% relative standard deviation. The developed method was found to be robust under chromatographic conditions with changes in pH and mobile phase mixture ratio. The method was also explored for forced degradation study, and the results showed the successful separation of degradation products from the AKBA. Further, the RP-HPLC method was applied for the quantification of AKBA in topical nanoformulations and different matrices, such as skin matrices and adhesive tapes. The method was able to measure entrapment efficiency (93.13 ± 1.94%), drug loading (25.83 ± 0.54%), drug assay in a gel matrix (96.99 ± 3.89%), drug amount in stratum corneum (7.90 ± 0.62 μg cm−2), and drug amount in viable skin layers (33.94 ± 0.21 μg cm−2) with high-speed reproducibility. The developed method can be utilized for the routine analysis of AKBA in conventional and complex formulations in academia and industry.
1. Introduction
3-Acetyl-11-keto-β-boswellic acid (AKBA) is a naturally derived pentacyclic triterpene obtained from the gum resin of Boswellia serrata. Its molecular weight is around 512.7 g mol−1 with an empirical formula of C32H48O5.1 AKBA exists as a white crystalline powder with limited water solubility and high solubility in commonly used organic solvents such as ethanol, methanol, acetonitrile, chloroform, and acetone.2,3 Recently, it has become a drug of choice for the treatment of inflammatory conditions like rheumatoid arthritis (RA)4 because of its immunomodulatory, anti-inflammatory, and 5-lipoxygenase (5-LOX) inhibitory properties.5–7 AKBA is, therefore, able to reduce the tissue damage brought on by chronic inflammation by inhibiting the synthesis of inflammatory mediators through such mechanisms. Due to the possible health advantages of AKBA, it has generated proficient attention in the realm of pharmaceutical research.3
AKBA is explored for oral and topical delivery with multifaceted nanocarrier systems for its effective delivery in RA.8 Further, to increase the availability of AKBA at the disease site, the topical nanoformulations of AKBA can be a potential drug delivery approach. Developing and optimizing such AKBA-loaded nanocarrier-based delivery systems require a rapid and sensitive analytical method to evaluate in vitro, ex vivo, and in vivo samples. A sensitive method is required that can determine AKBA in the presence of various sample matrices, including solvents, buffers, and skin tissues.9,10
Analysis of AKBA using high-performance thin-layer chromatography, liquid chromatography-mass spectrometry, and high-performance liquid chromatography coupled with tandem mass spectrometry has been majorly reported for the quantification of different derivatives of boswellic acids such as AKBA, 11-keto-beta-boswellic (KBA), etc. or in combination with another analyte, in bulk drugs, dogs supplements and biological fluids such as rat plasma.11–14 Miscioscia et al. have developed a high-performance liquid chromatography (HPLC) method for the quantification of AKBA and KBA in Boswellia serrata dog supplements. In this method, the optimized parameters consist of a total of 29 min run time with gradient program and 50 μL injection volume. Such a long run time and high injection volume may lead to longer total analysis times, column overloading, and higher operating costs for labs when a large number of samples are to be analyzed. Additionally, the method showed limited sensitivity and linearity range that may be a challenge for the detection of AKBA in nanoformulations.14
In another study, Rehman et al. quantified AKBA in Boswellia sacra by using near-infrared spectroscopy coupled with partial least squares regression and cross-validated by HPLC. The method consists of a higher run time as well as a longer run time. The higher run time and retention time of the method may be substantial shortcomings for high-throughput laboratories. Such a longer time increases solvent utilization and complete working costs. The calibration curve range for the developed method was 5 to 100 μg mL−1, which offers a wide measurement window; however, accurately quantifying analytes at low concentrations will be difficult.15
Chemometric assisted stability indicating RP-HPLC method developed by Abdul et al. for Boswellia serrata (aflapin) and collagen type II in the combined dosage form using the mobile phase used was hexane and IPA in a ratio of 20
:
80 (v/v) with 0.1% acetic acid. The use of a mobile phase with a high proportion of IPA and hexane may lead to a higher cost of analysis and compatibility issues with the HPLC pump system and may disorient the stationary phase on long-term use.16 Similarly, Asteggiano et al. reported a retention time of AKBA at 16.1 min in the optimized parameters, indicating excessive utilization of solvent and overall costs. The calibration curve range for the developed method was 0.1 to 100 mg L−1; this might not be enough for applications that need ultra-trace level detection, needing further optimization to increase sensitivity.17
There are few reports available on the determination of AKBA and KBA in the market formulations containing Boswellia serrata extract using the HPLC method. However, these methods were not specific to only AKBA and did not establish the stability indication power of the method.18,19
Recently, we have developed a simple and cost-effective UV-visible spectroscopic method for the analysis of AKBA, which could be economically feasible, especially in academic sectors.2 However, the spectroscopic method has limitations for stability indication of nanoformulation and sensitivity to nanogram quantification.
Overall, reported HPLC methods manifested limitations such as longer run time, longer retention time, low sensitivity, variability in accuracy and precision, and not reflecting stability, indicating AKBA. Additionally, these methods do not explain in detail the optimization process of the developed methods. Importantly, no RP-HPLC method has been shown to detect AKBA at the nanogram level (below 100 ng mL−1), which clearly indicates the requirement for the more sensitive method.14,18 Furthermore, all these reported methods were utilized for the estimation of boswellic acids from the Boswellia serrata extract or Boswellia gum resin but not specific for the estimation of pure AKBA alone. Also, reported methods have not been explored for multiple applications for the estimation of AKBA in emerging drug delivery and a wide variety of sample matrices.
There is a need to develop robust HPLC analytical methods for the estimation of AKBA in its nanocarrier systems, which will be essential for the advanced drug delivery system. The sensitive and rapid method is required to maintain strict quality control for complex advanced drug delivery systems, which eventually advances nanomedicine and improves therapeutic effectiveness. By taking care of this analytical need, more dependable and efficient AKBA-based nanocarrier formulations would be possible, which will promote innovation and enhance patient outcomes.
Therefore, in the current work, a novel, simple, sensitive, stability-indicating, and robust RP-HPLC method with a UV-visible detector was developed for the accurate estimation of AKBA in complex nanocarrier systems, in vitro drug release samples and skin matrices. Afterward, the developed method was validated as per ICH Q2 (R1) guidelines, confirming parameters such as linearity & range, sensitivity, accuracy, precision, system suitability, and robustness. So that the established RP-HPLC method can be utilized efficiently in both academia and industry. Additionally, the focus of the study is on applying the method for routine analysis of AKBA in advanced formulations, indicating that the approach is simple to use and repeatable.
2. Materials and methods
2.1 Experimental solvents, chemicals, and reagents
AKBA was purchased from Gurjar Phytochem Pvt. Ltd, Madhya Pradesh, India. HPLC grade acetonitrile (ACN) and methanol were procured from Merck Life Science Pvt. Ltd Mumbai (India). Hydrogen peroxide, hydrochloric acid, and Tween 80 were purchased from S.D. Fine Chemicals (India). Orthophosphoric acid and sodium hydroxide were procured from Central Drug House (CDH) Pvt. Ltd (New Delhi, India). Labrafac CC and Dynasan 114 were received as gift samples from Gatteffose. 0.22 μm Millipore™ membrane filter was procured from Merck Darmstadt (Germany).
2.2 Instrumentation
The instrument Shimadzu HPLC system (Kyoto, Japan) was utilized for the development of the analytical method. The system has a binary pump (LC-20AD), degassing unit (DGU-20A5R), UV-visible detector (SPD-20A), autosampler (SIL-20AC HT), and column oven (CTO-10AS). The column utilized for analysis was Phenomenex InertClone™ 5 μm ODS (C18) column with the dimensions of 250 × 4.6 mm. The instrument was operated by the software LC solution version 5.97 for data acquisition, process monitoring, and system control.
2.3 RP-HPLC chromatographic conditions
The isocratic RP-HPLC method comprised mobile phase ACN
:
milli Q (pH 3.5, adjusted by 1% orthophosphoric acid) in a ratio of 90
:
10 (v/v %). The analyte was detected using a UV-visible detector at wavelength 250 nm, a flow rate of 1 mL min−1, with an injection volume of 20 μL and a column oven temperature of 30 °C.
2.4 Preparation of stock, calibration, and quality control standard solutions
A primary stock solution of 1 mg mL−1 concentration was prepared with 10 mg of AKBA dissolved in 10 mL ACN. The secondary stock solution (100 μg mL−1) was prepared in ACN from the primary stock solution. The calibration standard solutions (250–20
000 ng mL−1) were prepared through serial dilution using ACN from a secondary stock solution.20 Similarly, the three different quality control (QC) standard solutions, which are Low QC (LQC, 500 ng mL−1), medium QC (MQC, 2500 ng mL−1), and high QC (HQC, 15
000 ng mL−1), were prepared from the secondary stock solution.
2.5 Determination of validation parameters of the developed RP-HPLC method
The RP-HPLC method was developed and fully validated as per ICH Q2 (R1) guidelines for system suitability, linearity, range, LOD, LOQ, accuracy, intraday & interday precision, specificity, carryover effect, robustness, and solution stability.21
2.5.1 System suitability.
For chromatographic methods, a system suitability test was recommended to guarantee that the system is effective and produces repeatable results. The system's efficacy was assessed by injecting the same sample with a concentration of 5 μg mL−1 six times under optimal chromatographic conditions. The chromatogram was used to evaluate the peak area, retention time, theoretical plate, and tailing factor and considered acceptable when a theoretical plate number is more than 2000 and a tailing factor of <2.22
2.5.2 Linearity, range, limit of detection, and limit of quantification.
The linearity of the chromatographic method was determined by injecting six standard concentrations of AKBA in the range of 250–20
000 ng mL−1 using HPLC at 250 nm.23 After acquiring the results, these were incorporated into the linear regression equation. Subsequently, the graph of the calibration curve was constructed between the analyte peak area on the y-axis and analyte concentrations on the x-axis. The obtained linear regression equation was assessed for the slope, regression coefficient, and intercept. The signal-to-noise (S/N) ratio was used to determine the LOD and LOQ. Initially, the detector response was used to determine the S/N ratio in the system suitability test. For LOD and LOQ, the ideal S/N ratios were 3
:
1 and 10
:
1, respectively.
2.5.3 Accuracy and precision.
Accuracy is the determination of the closeness of an observed value to a specified value. Contrarily, precision is related to how closely measurement values are related to one another. Using the quality controls (low, medium, and high) and standard addition method, the accuracy was determined. Therefore, three different quality control level samples were determined using the calibration curve range, i.e., LQC (500 ng mL−1), MQC (2500 ng mL−1), and HQC (15
000 ng mL−1), and were prepared from the standard stock solution. And for the standard addition method, a known quantity of standard stock solution was added to 80%, 100%, and 120% of the preanalyzed solution (10
000 ng mL−1). Each quality control level samples were prepared in n = 3. The % recovery was used to indicate accuracy as per the ICH Q2 (R1) guideline.
The intraday precision was assessed at a different time point on the same day using the same prepared quality control level (LQC, MQC, and HQC) samples. Additionally, interday precision was assessed on different days (three consecutive days) using the same prepared quality control level (LQC, MQC, and HQC) samples. The % RSD was used to indicate precision as per the ICH Q2 (R1) guideline. The regulatory standards required that the precision and accuracy of QC samples be within <±2% RSD for approval.24
2.5.4 Carryover effect.
Analyzing subsequent samples (250 ng mL−1, 2000 ng mL−1, and 10
000 ng mL−1) of the linearity curve after a blank allowed for the assessment of carryover.25
2.5.5 Robustness.
The ICH standards define robustness as the analytical procedure's capacity to remain unaffected by minor modifications but intentional modifications in the experimental conditions. Various chromatographic parameters, including the mobile phase combination, column oven temperature, and pH, were chosen for the proposed experiments. Slight modifications to the operating parameters, such as the temperature of the column oven (±2 °C), mobile phase mixture (±2%), and pH of the milli Q (±0.5) were done.22,23
2.5.6 Specificity study.
To evaluate the specificity of the developed chromatographic method, AKBA was identified amidst additional formulation excipients that are commonly found in nanoformulations and probably obstruct the drug's retention time. To ensure method specificity, the blank LNCs, and AKBA-loaded LNCs were centrifuged at 14
000 rpm for 30 min, followed by dilution of the supernatant with ACN. The prepared samples underwent filtration through a 0.22 μm syringe filter and were analyzed through HPLC.2,26
2.5.7 Forced degradation studies of AKBA using the developed RP-HPLC chromatographic method.
As per ICH Q1A (R2) guideline, the stability-indicating nature of the developed chromatographic technique was analyzed under which the AKBA solution was subjected to different stress conditions such as acid hydrolysis, base hydrolysis, oxidation hydrolysis, and thermolytic hydrolysis. For acid and base hydrolysis, 1 M hydrochloric acid and 1 M sodium hydroxide were used to prepare a 200 μg mL−1 solution of AKBA, respectively. The solution was then held for reaction on a water bath at 60 °C for 6 h. In a similar manner, AKBA solution was made with 3% hydrogen peroxide and heated for 6 h at 60 °C in a reflux environment to facilitate oxidative breakdown. For thermal stress, the AKBA solution was heated for 6 h at 60 °C in a reflux environment. All the stress conditions samples were also kept separately at room temperature to check the sample stability at room temperature. After keeping the AKBA solution (200 μg mL−1) under the aforementioned stress conditions, a 10 μg mL−1 solution of AKBA was prepared using ACN. To safeguard the column, the acid and base samples were neutralized before being diluted with ACN. A 0.22 μm syringe filter was used to filter all the samples before they were injected into the HPLC for their analysis. The normal state and the chromatogram of various stress situations were compared. The different degradant peaks and drug peaks were identified, and the percentage of AKBA degradation was determined.25
2.6 Application of validated RP-HPLC method
The developed and validated chromatographic method of AKBA was effectively utilized in analyzing stability-related samples of AKBA and quantifying AKBA in prepared LNCs. Furthermore, the method facilitated the analysis of AKBA entrapment efficiency in LNCs, in vitro release, ex vivo permeation, and skin retention samples. The application of this RP-HPLC chromatographic method is shown in Fig. 1.
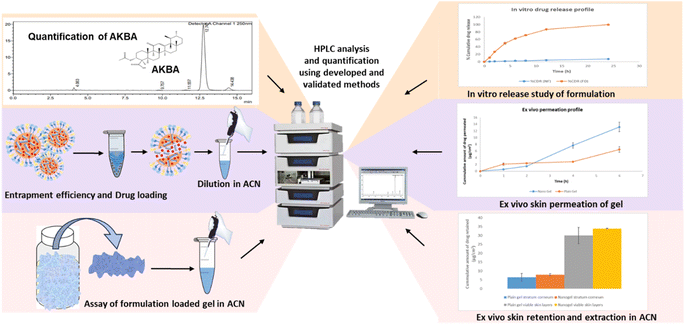 |
| Fig. 1 Diagrammatic representation of the application of the developed RP-HPLC chromatographic method. | |
2.6.1 Solution stability study.
The developed method was utilized to assess the stability of the sample solution at varied temperature conditions such as fridge temperature (2–8 °C) and room temperature (25 ± 2 °C). For the same, the QC samples that were maintained at fridge temperature (2–8 °C) and room temperature, their accuracy and precision were examined on different days.27
2.6.2 Preparation of AKBA-loaded lipid nanocarriers.
LNCs were formulated utilizing the hot emulsification process. The solid lipid (Dynasan 114) was melted first at 70 ± 5 °C, and then liquid lipid (Labrafac CC) was added along with AKBA to the melted lipid. The aqueous surfactant (Tween 80) phase was also heated at the same temperature. The lipid phase was progressively added dropwise to the heated aqueous phase while being stirred for 20 min. After hot stirring for 20 min, the prepared dispersion was sonicated for 6 min to reduce the size. Afterward, the dispersion was stirred for 30 min in an ice bath. The resulting formulation was allowed to cool at room temperature. Finally, the LNCs-loaded gel was obtained by loading AKBA-loaded LNCs dispersion into the gel matrix.28,29
2.6.3 Entrapment efficiency and drug loading analysis.
For determination of entrapment efficiency and drug loading, the developed formulation underwent centrifugation at 5000 rpm for 5 min. Further, 100 μL supernatant was diluted with ACN to 1 mL and again kept for centrifugation for 30 min at 14
000 rpm. This step facilitated the setting of lysed nanoparticle content and produced a clear supernatant. The supernatant was then diluted with ACN and filtered through a 0.22 μm syringe filter before injecting into the HPLC for their analysis.30,31 The % entrapment efficiency and drug loading were calculated using the formula mentioned below.
2.6.4 AKBA loaded LNCs gel assay.
The assay of the AKBA-loaded LNC gel was determined by dissolving the required quantity of gel in ACN. The resulting samples were filtered through a 0.22 μm syringe filter prior to injection into the HPLC for analysis.
2.6.5
In vitro drug release analysis.
For the in vitro drug release investigation, a dialysis membrane (Hi media, MW: 12 to 14 kDa) was utilized. As release media, 15 mL of pH 7.4 PBS media were selected, and 2 mL of formulation was added to the dialysis bag. At release assembly, the bag was maintained at 350 rpm and 37 ± 2 °C. To maintain sink conditions, 1 mL aliquots were taken out at intervals of 1, 2, 4, 6, 8, 12, and 24 h and replaced with fresh release medium. After the collection of samples at different time intervals, they were passed through a 0.22 μm filter and then analyzed through a developed chromatographic technique. Three sets of experiments were conducted. The acquired data were used to create a graph with time (x-axis) and percentage cumulative medication release (y-axis).26
2.7 Application in ex vivo skin studies
2.7.1 Extraction of AKBA from skin matrix.
The recovery percentage of AKBA from the animal skin was investigated before doing the ex vivo study. Goat ear skin obtained from an abattoir and cleaned further by removing the subcutaneous fat and hair. Following three freshwater washes, the skin was homogenized and spiked with a standard AKBA solution at a concentration of 100 μg mL−1. Afterward, the samples were centrifuged for 20 min at 15
000 rpm to eliminate any unnecessary skin tissue and debris, and then they were filtered using a 0.22 μm syringe filter and analyzed using a developed chromatographic technique. To calculate the percentage of recovery in triplicates, the AKBA quantity recovered upon analysis of these samples was compared with the amount that was initially added.26
2.7.2 AKBA skin permeation and retention study.
Goat ear skin was prepared as mentioned in Section 2.7.1 and placed on a Franz diffusion cell with a 1.33 cm2 surface area to examine the influence of the skin penetration of the developed formulation. Before placing the gel on the surface of the skin at the donor end, the device was equilibrated for 30 to 45 min at 32 ± 1 °C and 120 rpm using 20 mL of pH 7.4 PBS media that was employed as released media and poured in the receptor compartment. 1 mL of the sample was taken at specified intervals (1, 2, 4, and 6 h), and in order to preserve sink conditions, the same quantity of medium was substituted.32
After 6 h of skin permeation study, the skin was removed, and unabsorbed gel was collected separately. Tape stripping (Scotch™ 3 M, USA) was used to extract the drug from the stratum corneum and viable dermis. A total of 12 strips of tape were gathered in glass vials to remove the stratum corneum. Then viable dermal layer was collected in additional vials. ACN was added to each vial to extract the drug. The samples were sonicated for 1 h, and the aliquots were centrifuged for 20 min at 10
000 rpm in order to settle the debris. Following supernatant dilution and filtering, the developed chromatographic technique was used for analysis.32 Three sets of the experiment were conducted, and all samples were filtered through a 0.22 μm syringe filter before injecting into HPLC for their analysis. Plotting the percentage cumulative quantity of AKBA penetrated (y-axis) against time (x-axis) resulted in the permeation profile.
3. Results and discussion
3.1 Preliminary method development studies
Preliminary trials were taken to achieve optimum chromatographic conditions that can provide economic routine analysis. To enable drug elution in a shorter run time, the mobile phase condition was optimized. In the beginning, solvents such as methanol, ACN, aqueous buffers, and milli Q, along with different pH, were explored to get appropriate combinations of mobile phases.
In pre-formulation studies, it was found that AKBA has more solubility in ACN than methanol. Additionally, in the case of ACN, peak symmetry and peak area were found to be appropriate and more in comparison to methanol. Peak area, tailing factor, and retention duration of chromatograms were detected when ACN was mixed with various ratios of aqueous buffers and milli Q separately. The AKBA has not shown any difference in peak area and retention time by changing pH from 3 to 5. So, as per preliminary trials, 3.5 pH was finalized. With respect to mobile phase composition among ACN
:
phosphate buffer 10 mM (pH 3.5) and ACN
:
milli Q (pH 3.5), there was no significant difference observed in the retention duration, but peak symmetry was observed better in the case of ACN
:
milli Q (pH 3.5). The additional use of salts can be avoided to protect the column from deposition of salts inside it. Additionally, the pH adjusted for the milli Q was also found to be stable for 72 h. This clearly indicated that this aqueous phase can be used for 3 days for the analysis of samples. The mobile phase ratio is important here as the compound is highly hydrophobic, so the method requires more organic solvent. In initial trials where the milli Q ratio was higher and the organic solvent was lower, more retention time was observed. So, to get a shorter retention time, more organic solvent, i.e., ACN was kept. So, the mobile phase was used in the aforementioned exploratory investigations in a 90
:
10 v/v ratio. The flow rate is important to resolve the peaks properly and to get the separate peaks of AKBA. The flow rate explored was 0.8 mL min−1 to 1.2 mL min−1. It was observed that with a lower flow rate, the peak area was less and retention time was more, and with a higher flow rate, retention time was less, but peaks were not eluted properly. It showed a higher tailing factor. So, at a 1 mL min−1 flow rate, the resolution (more than 2) and tailing factor (less than 1.2) of the peak were observed as desirable. After such screening, injection volume of 10 μL and 20 μL was also screened; it was found that 20 μL had a good peak area and a lower tailing factor. The aforementioned experiments showed that the ACN and milli Q%, and flow rate had the greatest impact on the peak qualities. The optimized parameters were considered as mobile phase ACN
:
milli Q (pH 3.5) in a ratio of 90
:
10 (v/v%), wavelength 250 nm, injection volume of 20 μL, a flow rate of 1 mL min−1, and the column temperature of 30 °C. These conditions provided quick chromatographic separation, reduced tailings factor, improved resolution, and a high theoretical plate count. The chromatogram of blank (ACN) and AKBA is shown in Fig. 2.
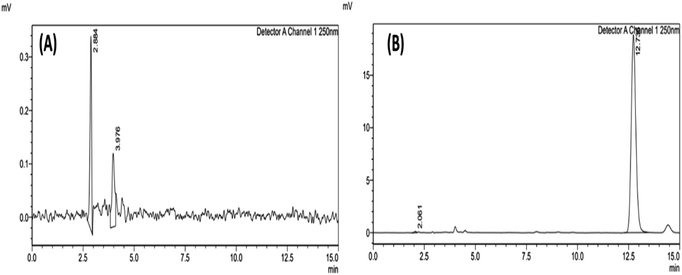 |
| Fig. 2 (A) Chromatogram of blank ACN and (B) chromatogram of 10 000 ng mL−1 AKBA sample. | |
3.2 Determination of validation parameters of the developed RP-HPLC chromatographic methods
3.2.1 System suitability.
Six duplicate injections of AKBA at a concentration of 5 μg mL−1 under optimal chromatographic conditions were used to determine the system suitability. The system's suitability was shown by the % RSD of peak area (0.5177), retention time (0.0744), height equivalent to a theoretical plate (HETP) (0.0888), and tailing factor (0.1451), which was less than 2%, showed that the system is working at its best as all of the assessed parameters met the predetermined acceptance standards. This is necessary for quantitative analysis to produce repeatable and trustworthy results.
3.2.2 Linearity, range, detection, and quantification limits.
The AKBA concentration and peak area were shown to be linear in the 250 ng mL−1 to 20
000 ng mL−1 range, with an excellent regression value of 1, indicating an excellent linear relationship between the response obtained to the concentration of the analyte. This suggests that the technique monitors analyte concentration fluctuations properly and does not introduce bias or distortion into the quantitative data. The linear regression equation was found to be y = 28.464x + 612.64, where “x” represents the AKBA concentration while “y” is the peak area at 250 nm. Fig. 3 shows the details of the AKBA calibration curve. The signal-to-noise ratio was used to calculate the LOD and LOQ of the developed analytical technique. The results showed that the values were 41.3195 and 125.2108 ng mL−1, respectively. The outcomes demonstrated that the developed approach could identify and measure AKBA at nanogram levels with sufficient sensitivity. Routine analysis of AKBA in nanoformulation, where the drug is entrapped, might benefit from this technique.
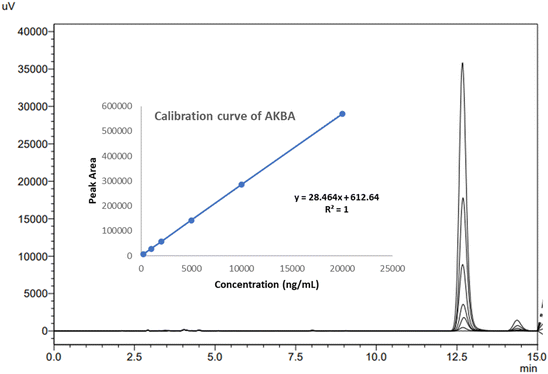 |
| Fig. 3 Calibration curve of AKBA along with an overlay of different calibration curve concentrations. | |
3.2.3 Accuracy and precision.
By using the quality controls (LQC (500 ng mL−1), MQC (2500 ng mL−1), and HQC (15
000 ng mL−1)) and the standard addition method, the accuracy was assessed. The method showed 98.5039 ± 0.8015 to 101.5737 ± 1.2018% recovery and % RSD less than 2%. The % recovery value (almost 100%) indicated accuracy in the measurement of the method without considerable variation. Further, the % RSD value emphasized the consistency of the procedure across several runs and samples. Fig. 4 shows the overlay of chromatograms for LQC, MQC, and HQC. Table 1 provides the accuracy results in terms of % recovery and % RSD.
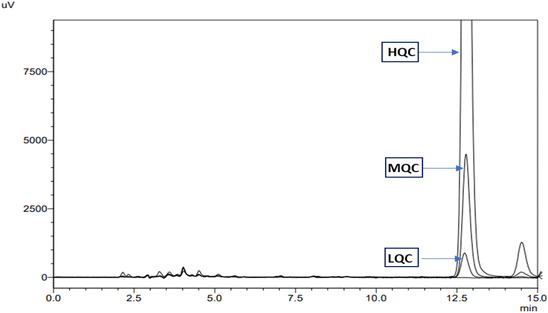 |
| Fig. 4 Overlay of chromatograms for LQC, MQC, and HQC samples of AKBA. | |
Table 1 Accuracy determination of developed HPLC method for AKBAa
Using quality controls |
Using standard addition method |
Concentration (ng mL−1) |
% Recovery (mean ± SD) n = 3 |
% RSD |
Amount added percentage (%) |
% Recovery (mean ± SD) n = 3 |
% RSD |
QC: quality control samples; LQC: lower quality control; MQC: medium quality control; HQC: high-quality control.
|
LQC (500) |
98.5039 ± 0.8015 |
1.3874 |
80 |
101.3451 ± 0.7337 |
0.0072 |
MQC (2500) |
99.3271 ± 0.6792 |
0.8052 |
100 |
100.3341 ± 0.7009 |
0.0069 |
HQC (15000) |
101.5737 ± 1.2018 |
0.8256 |
120 |
100.3483 ± 0.9462 |
0.0094 |
Further, by analyzing three quality control samples, i.e., LQC (500 ng mL−1), MQC (2500 ng mL−1), and HQC (15
000 ng mL−1), the precision was analyzed. All the QC samples showed % RSD less than 2%, indicating the preciseness of the method. Table 2 provides the intra- and inter-day precision data in terms of % RSD for AKBA.
Table 2 Precision results of determination of AKBA using the HPLC methoda
Sample QC |
Intra-day repeatability RSD (%) (n = 9) |
Inter-day repeatability RSD (%) (n = 9) |
Morning |
Afternoon |
Evening |
Day 1 |
Day 2 |
Day 3 |
QC: quality control samples; LQC: lower quality control; MQC: medium quality control; HQC: high-quality control.
|
LQC (500 ng mL−1) |
0.5280 |
1.7693 |
1.8651 |
0.5280 |
1.3278 |
1.7037 |
MQC (2500 ng mL−1) |
0.9437 |
1.0973 |
0.3747 |
0.9437 |
1.3943 |
1.2714 |
HQC (15 000 ng mL−1) |
0.9674 |
0.8296 |
0.6798 |
0.9674 |
1.8432 |
1.7542 |
3.2.4 Carryover effect.
The carryover impact was calculated by assessing subsequent samples with AKBA concentrations of 250, 2000, and 10
000 ng mL−1, followed by a blank sample. At 12.5 min into the retention period, there was no AKBA peak visible in the blank. The outcome showed that there was no carryover effect, meaning that this approach may be used continuously for a larger number of samples.
3.2.5 Robustness.
By altering the different chromatographic parameters, such as the pH of the milli Q (3 to 4), column oven temperature (28 to 32 °C), and mobile phase mixture ratio (88
:
12 to 92
:
08), robustness was evaluated. A significant difference was not observed in several essential factors (peak area, tailing factor, and theoretical plate) by changing the above-mentioned parameters, as depicted in Table 3. By adjusting the variable circumstances, the developed method's robustness has been verified, and the observed values fall within the acceptable range. The analytical guidelines state that the theoretical plate number exceeding 2000, the tailing factor <2, and the % RSD ≤ 2% are acceptable. These results highlight the resilience of the system, guaranteeing consistent and dependable outcomes under various operating scenarios. It demonstrated that even in the event of slight variations in chromatographic conditions, the procedure can reliably produce accurate and precise results.
Table 3 Robustness results of the developed RP-HPLC method
Robustness parameters |
Peak area mean ± SD (% RSD) |
Tailing factor mean ± SD (% RSD) |
HETP mean ± SD (% RSD) |
Standard samples |
146 132 ± 1542 |
1.16 ± 0.0015 |
9116 ± 77.5822 |
Column oven temp. (28 °C) |
148 048 ± 6071 (0.9212%) |
1.16 ± 0.0011 (1.7803%) |
8889 ± 87.3059 (0.0405%) |
Column oven temp. (32 °C) |
147 874 ± 1446 (0.8377%) |
1.16 ± 0.0045 (1.3293%) |
9289 ± 91.0329 (0.0405%) |
MP ratio (88 : 12) |
143 817 ± 1113 (1.1292%) |
1.15 ± 0.0030 (1.3152%) |
8948 ± 78.6786 (0.9381%) |
MP ratio (92 : 08) |
146 471 ± 1556 (0.1636%) |
1.19 ± 0.0045 (0.5178%) |
9183 ± 104.9333 (1.4835%) |
pH (3.0) |
146 498 ± 1351 (0.1768%) |
1.15 ± 0.0216 (0.0724%) |
9106 ± 50.3421 (0.8151%) |
pH (4) |
145 380 ± 803 (0.3648%) |
1.17 ± 0.0035 (0.8845%) |
9002 ± 70.7695 (0.6654%) |
3.2.6 Specificity study.
Lipids utilized in the preparation of LNCs were present during the specificity study. At the AKBA peak's retention time, no peak interference was detected, and the peak's 99.99% purity was determined. Fig. 5 illustrates the developed method's specificity, which clearly indicates the absence of a peak at the drug's RT. The method's ability to consistently and accurately measure AKBA without interference from the nanocarrier LNCs is confirmed by the unequivocal identification of the drug peak at its RT, without any comparable peak in the blank LNCs sample. These results strengthen the method's overall robustness and dependability and encourage its continued application in the development and quality control of nanocarrier systems loaded with AKBA.
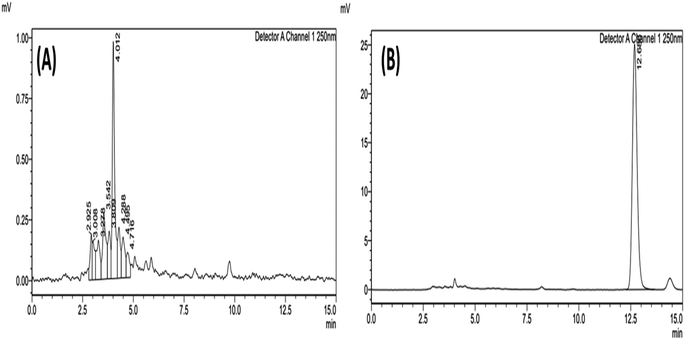 |
| Fig. 5 (A) Chromatogram of blank LNCs and (B) chromatogram of AKBA loaded LNCs. | |
3.2.7 Forced degradation study of AKBA using the developed method.
The forced degradation studies in different stress conditions, including acid hydrolysis, base hydrolysis, oxidative hydrolysis, and thermolytic hydrolysis, were conducted successfully. Table 4 depicts the AKBA stability results. The AKBA sample's acid hydrolysis chromatogram (Fig. 6A) displayed degradation peaks at different RT. Under acidic hydrolysis, 48.17% degradation of AKBA was seen. Similarly, the base hydrolysis chromatogram of the AKBA sample was shown in Fig. 6B, and 89.11% degradation of AKBA was observed. This suggests that AKBA can deteriorate in highly basic conditions if kept at a higher temperature for a longer duration. Additionally, the chromatogram of AKBA (Fig. 6C) was of oxidative stress conditions, and 5.15% degradation of AKBA was observed in oxidative stress conditions. The thermal hydrolysis chromatogram is shown in Fig. 6D, and 50.79% degradation of AKBA was observed in thermal hydrolysis conditions. The stress samples, which were kept at room temperature, were also analyzed, and it was observed that in all different conditions, the % recovery of AKBA was approximately more than 80 to 90% after 6 h. The outcomes demonstrated that the developed analytical approach was capable of differentiating between the degradation products and the AKBA. It was observed that the developed approach demonstrated the method's stability-indicating property and was selective for AKBA. This may be used for AKBA in-process and final product stability testing.
Table 4 Forced degradation results of AKBA in different stress conditions
Stress condition |
Solvent |
Temperature |
Sampling time |
% of AKBA degraded ± SD |
Acid hydrolysis |
1 N HCl |
60 °C |
6 h |
48.1777 ± 1.3060 |
Base hydrolysis |
1 N NaOH |
60 °C |
6 h |
89.1178 ± 1.4982 |
Oxidative hydrolysis |
30% H2O2 |
60 °C |
6 h |
5.1576 ± 7.1665 |
Thermal hydrolysis |
Water |
60 °C |
6 h |
50.7991 ± 14.3801 |
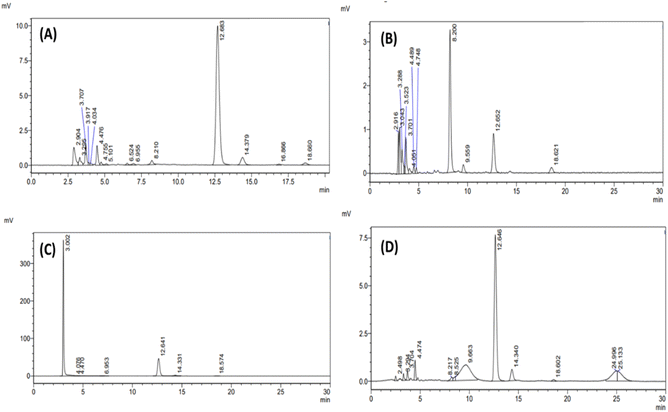 |
| Fig. 6 RP-HPLC chromatogram of (A) acid hydrolysis, (B) base hydrolysis, (C) oxidative hydrolysis, and (D) thermolytic hydrolysis. | |
3.3 Application of developed and validated RP-HPLC chromatographic method
3.2.5 Solution stability study.
The solution stability study was performed at two temperatures, room temperature (25 ± 2 °C) and fridge temperature (2 to 8 °C), for 7 days. The samples, which were kept at room temperature and fridge temperature, were found to be stable till 3 days and 7 days, respectively. After 3 days of room temperature samples, the % RSD for peak area, RT, HETP, and TF was observed at 1.6810, 0.3576, 1.2498, and 0.4538, respectively. Similarly, after 7 days of fridge temperature samples, the % RSD for peak area, RT, HETP, and TF was observed at 1.7618, 0.9530, 1.2627, and 0.3973, respectively. The method's efficiency in monitoring AKBA stability is demonstrated by the quantitative values produced, which exhibit low variability and excellent accuracy. This application showed hope for the utilization of this method for several preformulation studies required for formulation development.
3.3.2 Entrapment efficiency and drug loading analysis.
The entrapment efficiency (%) and drug loading (%) of AKBA-loaded LNCs were found to be 93.1380 ± 1.9394% and 25.8280 ± 0.5378%, respectively. The results of entrapment efficiency and drug loading showed that the developed method quantified the AKBA in AKBA-loaded LNCs with great precision and accuracy. This confirmed the proficiency of the developed RP-HPLC chromatographic method in measuring the AKBA in complex nanoformulations of AKBA.
3.3.3 AKBA loaded LNCs gel assay analysis.
The assay of AKBA-loaded LNC gel was performed, and it was observed that 96.9923 ± 3.8924% of AKBA was present in the AKBA-loaded LNC gel. The percentage recovery amount showed that the method was specific and sensitive in determining the active constituent in the presence of formulation excipients.
3.3.4
In vitro drug release analysis.
The in vitro drug release study showed 99.6718 ± 0.9998% release from free AKBA solution and 7.2816 ± 0.3497% release from AKBA-loaded LNCs formulation. Furthermore, no interference was identified at drug RT because of dissolution media. Also, the method was able to detect the lower amount of drug released (0.39 μg mL−1) from the AKBA-loaded LNCs at the initial time point. Fig. 7 shows the in vitro release profile of AKBA from free AKBA solution and AKBA-loaded LNCs.
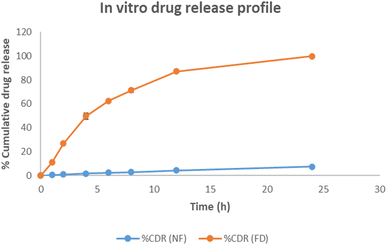 |
| Fig. 7
In vitro release profile of AKBA from its complex nanoformulation. | |
3.4 Application in ex vivo skin studies
3.4.1 Extraction of AKBA from skin matrix.
The percentage of AKBA recovered from skin tissue was 86.7407 ± 3.1736%. Persistent recovery and utility on AKBA measurement in skin permeation and retention investigations were demonstrated by the developed RP-HPLC chromatographic method.
3.4.2 AKBA skin permeation and retention study.
The skin permeation and retention study through goat ear skin was performed, and it was observed that 6.4634 ± 0.8333 μg cm−2 of AKBA was permeated from the plain gel and 13.1823 ± 1.4111 μg cm−2 of AKBA was permeated from AKBA loaded LNCs gel in 6 h. The ex vivo skin penetration profile of AKBA-loaded LNCs is displayed in Fig. 8A. The drug quantity in the stratum corneum was determined to be 6.4204 ± 2.1762 μg cm−2 and 7.9037 ± 0.6174 μg cm−2 and 30.0031 ± 4.6639 μg cm−2 and 33.9446 ± 0.2006 μg cm−2 in viable skin layers from plain gel and AKBA loaded LNCs gel respectively. AKBA retention in skin layers is seen in Fig. 8B. It was observed that the developed method was found to be efficient in determining the drug content in ex vivo studies. The method showed utility for further exploration in dermatopharmacokinetic studies of AKBA.
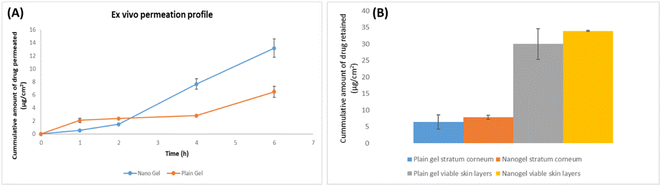 |
| Fig. 8 (A) AKBA ex vivo skin permeation profile from AKBA loaded LNCs (data represented as mean ± SD, n = 3), (B) bar graph representing the amount of AKBA in stratum corneum and viable skin layers (data represented as mean ± SD, n = 3). | |
4. Conclusion
The development of the HPLC method is crucial for pharmaceutical products, drug development, and drug discovery. In the present work, a novel, simple, sensitive, robust, reproducible, economic, and stability-indicating RP-HPLC method was developed. By monitoring the conditions that produced the optimum peak parameters, the composition of the mobile phase and the chromatographic conditions were optimized. Afterward, the method underwent thorough validation as per ICH Q2 (R1) guidelines, demonstrating to be linear in the concentration range, sensitive, accurate, precise, stable, and robust. Compared to the pre-existing method reported in the literature, the current method possesses several benefits, such as a detailed explanation of the optimization process of the developed methods, shorter retention time and run time, high sensitivity, eliminating variability in accuracy and precision, demonstrating significant robustness across a variety of conditions, and reflecting stability-indicating method for AKBA. Additionally, the current method is specific to the estimation of pure AKBA alone. The efficacy of the established HPLC method in analyzing the stability of AKBA under various stress conditions, including acidic hydrolysis, basic hydrolysis, oxidative hydrolysis, and thermal hydrolysis, has been proven by a forced degradation study. The developed HPLC method yielded a thorough stability indicating nature and demonstrated robustness and reliability in the detection of AKBA in the presence of degradants.
The developed method is novel for the estimation of AKBA in AKBA-based nanocarrier systems, indicating a requirement to quantify AKBA in emerging drug delivery systems, which eventually advances nanomedicine and improves therapeutic effectiveness. The developed method is able to estimate the AKBA in the presence of nanocarrier excipients in an in vitro drug release medium, ex vivo tape-stripped, and skin-permeated samples of AKBA at a biological pH medium. The usefulness of the validated analytical approach was demonstrated in the assessment of AKBA in pharmaceutical nanoformulations and for dermal product evaluation. As a result, the established approach may be applied in academia and industry to characterize and assess the stability of AKBA-loaded nanoformulations in a cost-effective way.
Data availability
The data supporting this article have been included as part of the ESI.†
Author contributions
Sakshi Priya: conceptualization; methodology; investigation; validation; data curation; formal analysis; writing – original draft; writing – review & editing. Gautam Singhvi: conceptualization; project administration; resources; supervision; validation; visualization; writing – review & editing.
Conflicts of interest
Authors declare no conflict of interest.
Acknowledgements
Sakshi Priya gratefully acknowledged the PhD fellowship from DST-INSPIRE, Govt. of India [#IF210090].
References
- M. Z. Siddiqui,
Boswellia serrata, a potential antiinflammatory agent: An overview, Indian J. Pharm. Sci., 2011, 73, 255–261 CAS
.
- S. Priya, V. M. Desai and G. Singhvi, Spectroscopic method to estimate 3-Acetyl-11-keto-β-boswellic acid in complex lipid nanocarriers and skin matrices, Results Chem., 2024, 7, 101261 CrossRef CAS
.
- S. Priya and G. Singhvi, Insights into the anti-inflammatory and anti-arthritic potential of 3-Acetyl-11-keto-β-Boswellic Acid as a therapeutic approach in Rheumatoid Arthritis, Expert Opin. Invest. Drugs, 2023, 1–5 Search PubMed
.
- S. Priya, J. Daryani, V. M. Desai and G. Singhvi, Bridging the gap in rheumatoid arthritis treatment with hyaluronic acid-based drug delivery approaches, Int. J. Biol. Macromol., 2024, 271, 132586 CrossRef CAS PubMed
.
- A. Siddiqui, Z. Shah, R. N. Jahan, I. Othman and Y. Kumari, Mechanistic role of boswellic acids in Alzheimer's disease: Emphasis on anti-inflammatory properties, Biomed. Pharmacother., 2021, 144, 112250 CrossRef CAS PubMed
.
- M. Rahman, S. Beg and A. Verma,
et al., Phytoconstituents as pharmacotherapeutics in rheumatoid arthritis: challenges and scope of nano/submicromedicine in its effective delivery, J. Pharm. Pharmacol., 2017, 69, 1–14 CrossRef CAS PubMed
.
- K. R. Gheorgehe, M. Korotkova and A. I. Catrina,
et al., Expression of 5-lipoxygenase and 15-lipoxygenase in rheumatoid arthritis synovium and effects of intraarticular glucocorticoids, Arthritis Res. Ther., 2009, 11, 1–11 Search PubMed
.
- K. Bairwa and S. M. Jachak, Development and optimisation of 3-Acetyl-11-keto-β-boswellic acid loaded poly-lactic-co-glycolic acid-nanoparticles with enhanced oral bioavailability and in-vivo anti-inflammatory activity in rats, J. Pharm. Pharmacol., 2015, 67, 1188–1197 CrossRef CAS PubMed
.
- U. Varia, D. Joshi, M. Jadeja, H. Katariya, K. Detholia and V. Soni, Development and evaluation of ultradeformable vesicles loaded transdermal film of boswellic acid, Futur. J. Pharm. Sci., 2022, 8, 39 CrossRef
.
- S. Y. Chuang, C. H. Lin, T. H. Huang and J. Y. Fang, Lipid-Based nanoparticles as a potential delivery approach in the treatment of rheumatoid arthritis, Nanomaterials, 2018, 8, 42 CrossRef PubMed
.
- M. Mehta, S. Satija and M. Garg, Comparison between HPLC and HPTLC densitometry for the determination of 11-keto-β-boswellic acid and 3- acetyl-11-keto-β-boswellic acid from Boswellia serrata extract, Indian J. Pharm. Educ. Res., 2016, 50, 418–423 CrossRef CAS
.
- S. A. Shah, I. S. Rathod, B. N. Suhagia, S. S. Pandya and V. K. Parmar, A simple high-performance liquid chromatographic method for the estimation of boswellic acids from the market formulations containing Boswellia serrata extract, J. Chromatogr. Sci., 2008, 46, 735–738 CAS
.
- A. Frank and M. Unger, Analysis of frankincense from various Boswellia species with inhibitory activity on human drug metabolising cytochrome P450 enzymes using liquid chromatography mass spectrometry after automated on-line extraction, J. Chromatogr. A, 2006, 1112, 255–262 CrossRef CAS PubMed
.
- E. Miscioscia, J. Shmalberg and K. C. Scott, Measurement of 3-acetyl-11-keto-beta-boswellic acid and 11-keto-beta-boswellic acid in Boswellia serrata Supplements Administered to Dogs, BMC Vet. Res., 2019, 15, 1–7 CrossRef CAS PubMed
.
- N. U. Rehman, L. Ali and A. Al-Harrasi,
et al., Quantification of AKBA in Boswellia sacra Using NIRS Coupled with PLSR as an Alternative Method and Cross-Validation by HPLC, Phytochem. Anal., 2018, 29, 137–143 CrossRef CAS PubMed
.
- S. A. Rahaman, L. P. Vistajasingamsetty and S. Adiki,
et al., Chemometric assisted new stability indicating rp-hplc method development and validation of boswellia serrata (aflapin) and collagen type ii in combined dosage form, J. Cardiovasc. Dis., 2021, 12, 996–1003 CAS
.
- A. Asteggiano, L. Curatolo, V. Schiavo, A. Occhipinti and C. Medana, Development, Validation, and Application of a Simple and Rugged HPLC Method for Boswellic Acids for a Comparative Study of Their Abundance in Different Species of Boswellia Gum Resins, Appl. Sci., 2023, 13, 1254 CrossRef CAS
.
-
R. Jain and S. Rajput, Boswellic Acid and Myristicin in Commercial Herbal Formulation, 2013 Search PubMed
.
- N. Sharma, V. Bhardwaj and S. Singh,
et al., Simultaneous quantification of triterpenoic acids by high performance liquid chromatography
method in the extracts of gum resin of Boswellia serrata obtained by different extraction techniques, Chem. Cent. J., 2016, 10, 1–10 CrossRef PubMed
.
- T. Waghule, R. Narayan Saha and G. Singhvi, UV spectroscopic method for estimation of temozolomide: Application in stability studies in simulated plasma pH, degradation rate kinetics, formulation design, and selection of dissolution media, Spectrochim. Acta, Part A, 2021, 258, 119848 CrossRef CAS PubMed
.
-
ICH Topic Q2 (R1) Validation of Analytical Procedures: Text and Methodology Step 5 Note for Guidance on Validation of Analytical Procedures: Text and Methodology (Cpmp/ich/381/95) Approval by Cpmp November 1994 Date for Coming into Operation, 1995 Search PubMed.
- M. Choudhari, M. R. Donthi, S. Damle, G. Singhvi, R. N. Saha and S. K. Dubey, Implementation of Quality by Design Approach for Optimization of RP-HPLC Method for Quantification of Abiraterone Acetate in Solid Dispersion in Forced Degradation Studies, Curr. Chromatogr., 2022, 9, 1–17 Search PubMed
.
- S. Chandran and R. S. P. Singh, Comparison of various international guidelines for analytical method validation, Pharmazie, 2007, 62, 4–14 CAS
.
- V. K. Rapalli, V. Kaul and S. Gorantla,
et al., UV Spectrophotometric method for characterization of curcumin loaded nanostructured lipid nanocarriers in simulated conditions: Method development, in-vitro and ex-vivo applications in topical delivery, Spectrochim. Acta, Part A, 2020, 224, 117392 CrossRef CAS PubMed
.
- S. Gorantla, R. N. Saha and G. Singhvi, Design of experiment-driven stability-indicating RP-HPLC method for the determination of tofacitinib in nanoparticles and skin matrix, Futur. J. Pharm. Sci., 2021, 7, 180 CrossRef
.
- S. Gorantla, R. N. Saha and G. Singhvi, Spectrophotometric method to quantify tofacitinib in lyotropic liquid crystalline nanoparticles and skin layers: Application in ex vivo dermal distribution studies, Spectrochim. Acta, Part A, 2021, 255, 119719 CrossRef CAS PubMed
.
-
World Health Organization, Q1F Stability testing of active pharmaceutical ingredients and finished pharmaceutical products, Annex, 2018, vol. 10, 1010, pp. 309–352. https://database.ich.org/sites/default/files/Q1F_Stability_Guideline_WHO_2018.pdf.
- V. K. Rapalli, V. Kaul and T. Waghule,
et al., Curcumin loaded nanostructured lipid carriers for enhanced skin retained topical delivery: optimization, scale-up, in-vitro characterization and assessment of ex-vivo skin deposition, Eur. J. Pharm. Sci., 2020, 152, 105438 CrossRef CAS PubMed
.
- S. Gorantla, E. R. Puppala, V. G. M. Naidu, R. N. Saha and G. Singhvi, Hyaluronic acid-coated proglycosomes for topical delivery of tofacitinib in rheumatoid arthritis condition: Formulation design, in vitro, ex vivo characterization, and in vivo efficacy studies, Int. J. Biol. Macromol., 2023, 224, 207–222 CrossRef CAS PubMed
.
- T. Waghule, V. K. Rapalli and G. Singhvi,
et al., Voriconazole loaded nanostructured lipid carriers based topical delivery system: QbD based designing, characterization, in-vitro and ex-vivo evaluation, J. Drug Delivery Sci. Technol., 2019, 52, 303–315 CrossRef CAS
.
- T. Waghule, V. K. Rapalli and G. Singhvi,
et al., Design of temozolomide-loaded proliposomes and lipid crystal nanoparticles with industrial feasible approaches: comparative assessment of drug loading, entrapment efficiency, and stability at plasma pH, J. Liposome Res., 2021, 31, 158–168 CrossRef CAS PubMed
.
- V. K. Rapalli, S. Sharma, A. Roy and G. Singhvi, Design and dermatokinetic evaluation of Apremilast loaded nanostructured lipid carriers embedded gel for topical delivery: A potential approach for improved permeation and prolong skin deposition, Colloids Surf., B, 2021, 206, 111945 CrossRef CAS PubMed
.
|
This journal is © The Royal Society of Chemistry 2024 |