DOI:
10.1039/D4AY00597J
(Paper)
Anal. Methods, 2024,
16, 4539-4550
Determination of ethylene-bis-dithiocarbamates and their degradation product ethylenethiourea in dry herbs by UHPLC-MS/MS†
Received
1st April 2024
, Accepted 3rd June 2024
First published on 7th June 2024
Abstract
Dithiocarbamates are a class of fungicides widely used in many countries. In this study, methods for determining the ethylene-bis-dithiocarbamate (EBDC) subclass, and their degradation product ethylenethiourea (ETU) were validated by UHPLC-MS/MS in different types of dry herbs, which can be used as food and/or medicinal purposes. Mancozeb was used in the validation of the EBDC method, where it was initially complexed with EDTA, derivatized, extracted with dimethyl sulfate in acetonitrile, magnesium sulfate (MgSO4), and sodium chloride (NaCl), and then purified using primary secondary amine (PSA). In the ETU method, L-cysteine hydrochloride monohydrate was added to the samples before extraction with acetonitrile, MgSO4, and NaCl, followed by purification with PSA. A pesticide-free blend of seven herbs (boldo, artichoke, “espinheira-santa”, cat's claw, senna, chamomile, and cascara buckthorn) comprising distinct parts of the plants (leaves, bark, flowers and/or stems) was used as a control for method validation. Recoveries ranged from 79 to 113% for EBDC and 81 to 109% for ETU. Repeatability and intermediate precision were <20% for both methods. The limit of quantification was 0.03 mg kg−1 for EBDC (0.02 mg kg−1 of CS2) and ETU. The limit of detection (LOD) was set at 1/3 of the LOQ (0.01 mg kg−1 for both analytes). In total, 103 samples of 33 different dry herbs were analyzed, of which 19.4% were positive for EBDC (≥LOD), but no ETU residues were found in any of the analyzed samples. Given the absence of registered dithiocarbamates for use in the investigated herbs in Brazil, the positive results suggest potential illegal pesticide use or cross-contamination, especially considering the low concentrations detected in most samples. Although exposure to EBDC through the consumption of medicinal herbs from positive samples did not indicate a health risk to consumers, these plants must be monitored to prevent illicit pesticide usage, particularly when the herbs are intended for therapeutic purposes.
Introduction
The use of dry herbs for tea preparation and medicinal purposes has an ancient origin, and over the centuries, their useful and harmful properties have been discovered.1,2 Additionally, these herbs may contain potentially toxic substances, such as heavy metals, radioactive particles, mycotoxins, and pesticides,3 whose levels should be monitored when intended for medicinal use.4,5
Pesticides are products applied in agriculture to control pests, such as insects and fungi, which can compromise food production and quality. However, they can also have an impact on human health and the environment if not adequately used. According to Brazilian legislation, the use of pesticides is not recommended in the cultivation of herbs intended for therapeutic use,4 but some compounds are registered in plants that are also used as food, such as chamomile, blackberry, guarana, and passion fruit, which in their dry forms are used for tea preparation, including for therapeutic purposes.6
Five fungicides from the dithiocarbamate class, including the ethylene-bis-dithiocarbamates (EBDCs) mancozeb and metiram, are registered for use on various crops in Brazil.6 In 2022, mancozeb was the most used fungicide (approximately 45
560 tons) and the third best-selling pesticide in the country.7 Data from Brazilian monitoring programs showed that dithiocarbamates are among the most detected pesticides in food samples,8,9 but dry herbs are not included in the programs, and the presence of pesticides in these products in Brazil is unknown.
The chronic toxicity of the EBDC subclass is mainly attributed to the metabolite ethylenethiourea (ETU), formed in mammals and plants, which has been shown to be carcinogenic in laboratory animal studies.10–12 ETU can also be formed during the processing of products of plant origin, including drying processes.13,14
Currently, the determination of dithiocarbamates in food in monitoring programs is based on the carbon disulfide (CS2) formed after the acid hydrolysis of any dithiocarbamate present in the sample,15 and the maximum residue level (MRL) for dithiocarbamates established in Brazil and other countries are based on CS2.6,16–18 However, CS2 determination does not allow the distinction between dithiocarbamate compounds present in the sample, a differentiation that is necessary to identify the application of good agricultural practices in the field and for a refined risk assessment of exposure to these fungicides in the diet, since the toxicity is not the same between the compounds.19
Analytical methods for the direct determination of dithiocarbamates have been reported in the literature, but most studies have been carried out on fresh fruits and vegetables.20–23 Sayed et al.24 developed an LC-MS/MS method for determining EBDC in chamomile and Zhang et al.25 validated a method for determining mancozeb in Schisandra chinensis (Turcz.) Bail by UPLC with a UV detector (272 nm) after methylation. ETU analytical methods by liquid chromatography have also been reported in fresh fruits and vegetables.26–28
This work aimed to validate analytical methods for determining EBDC and ETU in different types of dry herbs by ultra-high-performance liquid chromatography coupled to tandem mass spectrometry (UHPLC-MS/MS), analyze samples collected in the local market and conduct a risk assessment of consumers due to exposure to these compounds through the consumption of tea.
Experimental
Chemicals and reagents
Certified reference material of EBDC-dimethyl (purity 98.9%) was obtained from Hayashi Pure Chemical® (Japan), mancozeb (purity 97.5%) and metiram (purity 90.0%) from AccuStandard®, and ethylenethiourea (purity 99.68%) from Dr. Ehrenstorfer®. HPLC and LC-MS/MS grade methanol and acetonitrile were obtained from Merck®, while ammonium formate (purity ≥ 99.0%) and formic acid (purity 98%) were purchased from Fluka®. Anhydrous magnesium sulfate (MgSO4, purity ≥ 99.5%), L-cysteine hydrochloride monohydrate (Cys-HCl; purity ≥ 99.0%), EDTA (purity 99.0–101%), and dimethyl sulfate (purity ≥ 99.9%) were obtained from Sigma-Aldrich®. Sodium chloride (NaCl, purity 100.1%) was obtained from the J.T. Baker® brand, and primary secondary amine (PSA) from Supelco®.
Standard solutions and suspensions
Stock solutions or suspensions of mancozeb, metiram, EBDC-dimethyl and ETU were prepared in acetonitrile at a concentration of 1000 mg L−1. Intermediate solutions at 10 mg L−1 of EBDC-dimethyl and ETU were prepared in acetonitrile and of mancozeb and metiram in aqueous L-cysteine–EDTA solution. The intermediate solutions/suspensions were diluted with the same solvent to obtain the working solutions at 1000 ng mL−1. L-Cysteine–EDTA solution was prepared by adding 25 g of L-cysteine hydrochloride monohydrate and 37.2 g of EDTA into 500 mL of Milli-Q® water, with pH adjusted to 9.6–10.0 with a 10% NaOH aqueous solution, and dimethyl sulfate solution (0.05 mol L−1) was prepared in acetonitrile.
Mancozeb working solutions were used to fortify the control sample, and EBDC-dimethyl solutions were used to prepare the analytical curve. The ETU working solutions used to prepare the analytical curve and to fortify the control sample were prepared by diluting the intermediate solution in acetonitrile (1000, 100, and 10 ng mL−1). All solutions were stored in amber bottles at <−15 °C. The analytical curve concentrations were 3, 5, 15, 25, 40, 60, 80, and 100 ng mL−1 for mancozeb, and 1.5, 2.5, 5, 10, and 20 ng mL−1 for ETU. The fortification levels were 0.03, 0.05, 0.15 and 0.4 mg kg−1 for mancozeb and 0.03, 0.05, 0.1 and 0.2 mg kg−1 for ETU.
Sample extraction and clean-up
The extraction method for EBDC (mancozeb and/or metiram) determination was based on the study on chamomile (dry matrix) developed by Sayed et al.24 Ten milliliters of L-cysteine–EDTA aqueous solution were transferred to a 50 mL Falcon tube containing 1 g of the sample, followed by manual shaking. Dimethyl sulfate solution in ACN (10 mL) was added to the sample, followed by shaking on a Certomat® BS-T shaker table. MgSO4 (4 g) and NaCl (1 g) were added to the tube, which was manually shaken for 1 min. The mixture was centrifuged (5 min, 4500 rpm), and 3 mL of the organic phase (upper) was transferred to a Falcon tube containing MgSO4 (450 mg) and PSA (150 mg). The tube was vortexed (30 s) and centrifuged (5 min, 4500 rpm), and the resulting extract was filtered through a PTFE hydrophilic syringe filter (0.22 μm) directly into the vial for analysis by UHPLC-MS/MS. Fig. 1 shows details of the extraction procedure of the EBDC method.
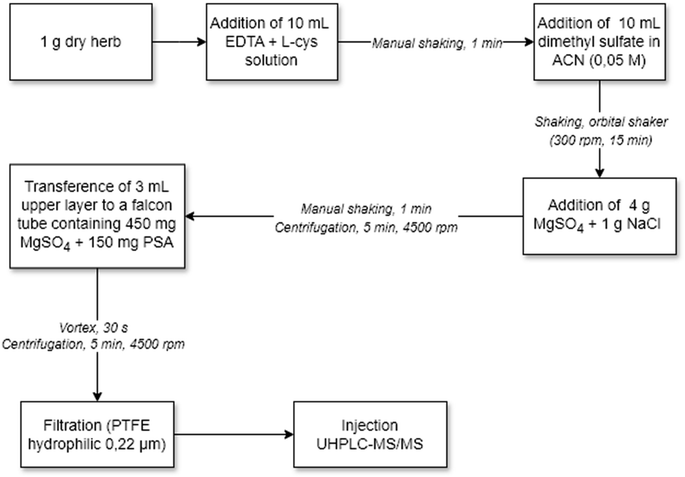 |
| Fig. 1 Flowchart of sample preparation for the determination of ethylene-bis-dithiocarbamates (mancozeb and/or metiram) in dry herbs. | |
The sample preparation procedure for ETU determination consisted of adding 0.5 g L-cysteine and 10 mL Milli-Q® water to a 50 mL Falcon tube containing 1 g of sample. The sample was kept at rest for 15 minutes to absorb the water, with subsequent addition of 10 mL of ACN and manual stirring. MgSO4 (4 g) and NaCl (1 g) were transferred to the tube, followed by manual shaking for 1 min and centrifugation (5 min, 3800 rpm); 3 mL of the organic phase was transferred to a Falcon tube containing MgSO4 (450 mg) and PSA (150 mg), the tube was vortexed (30 s) and centrifuged (5 min, 3800 rpm); 500 μL of the purified extract was transferred to a vial, evaporated to dryness in Centrivap®, resuspended with 1000 μL of MeOH
:
H2O (50
:
50) and filtered with a PTFE hydrophilic syringe filter (0.22 μm) for subsequent analysis by UHPLC-MS/MS. Fig. 2 illustrates the extraction procedure of the ETU method.
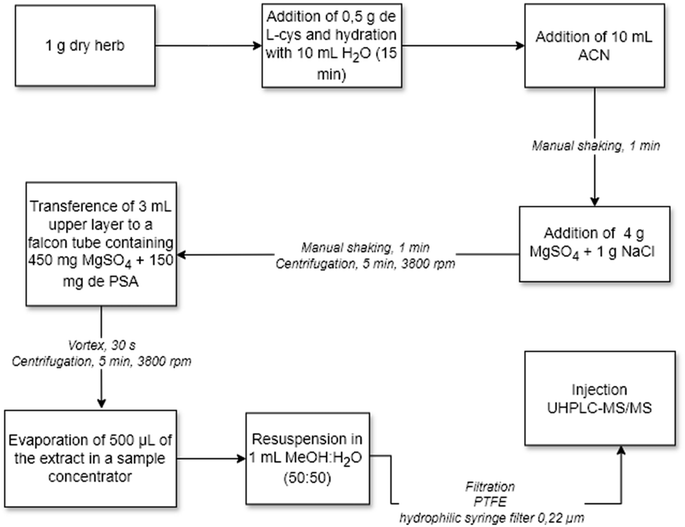 |
| Fig. 2 Flowchart of sample preparation for the determination of ethylenethiourea in dry herbs. | |
Applied Biosystems®/MDS Sciex® 6500+ QTrap UHPLC-MS/MS
The system consists of an Exion LC Sciex AD Series UHPLC (Ultra-High Performance Liquid Chromatography) (MA, USA), with a binary pump, degasser, automatic sampler, column oven (AC) and controller, coupled to a triple quadrupole mass spectrometer with an IonDrive™ Turbo V source and electrospray ionization in positive mode. Data acquisition was performed using Analyst® v. 1.7.2 and processed in Sciex OS v. 1.6.2. MS/MS optimization was performed by direct infusion of the ETU and EBDC-dimethyl in MeOH
:
H2O (50
:
50) with 0.1% acid formic (EBDC-dimethyl) or 5 mM ammonium formate (ETU) at a flow rate of 10 μL min−1.
Table 1 shows the optimized mass spectrometer parameters for the source, and the compounds EBDC-dimethyl and ETU in positive mode with a dwell time of 250 ms. The source was maintained at 450 °C and the entrance potential at 10 eV.
Table 1 Optimized 6500+ QTrap UHPLC-MS/MS parameters for ethylenethiourea (ETU) and dimethyl ethylene-bis-dithiocarbamate (EBDC-dimethyl)
Compound |
CUR,a psi |
CADa |
IS,a V |
G1/G2,a psi |
m/z |
Product ions (m/z) |
DPb (V) |
CEb (V) |
CXPb (V) |
Source optimized parameters – CUR: curtain gas; CAD: collision gas; IS: ion spray voltage; G1/G2: ion source gas.
MS/MS optimized parameters – DP: declustering potential; CE: collision energy; CXP: collision cell exit potential. Q: quantifier; q: qualifier.
|
ETU |
30 |
Medium |
5000 |
45/45 |
103 |
44.2 (Q) |
86 |
21 |
20 |
60 (q) |
86 |
45 |
28 |
EBDC-dimethyl |
50 |
Low |
4500 |
50/40 |
241 |
193 (Q) |
41 |
10 |
16 |
184 |
117 (q) |
41 |
15 |
16 |
For both methods, a UHPLC column LUNA Omega Polar C18 1.6 μm 100 A, 100 × 2.1 mm (Phenomenex), with a SecurityGuard ULTRA Cartridges UHPLC Fully Porous Polar C18 2.1 mm pre-column was used for chromatographic separation. The chromatography methods differ only in the gradient program employed. The mobile phase consisted of water with 5 mM ammonium formate and 0.1% formic acid (A) and methanol with 5 mM ammonium formate and 0.1% formic acid (B), the injection volume was set at 1 μL, the column oven temperature was maintained at 40 °C, and the flow rate was set at 0.2 mL min−1 for both methods. For the EBDC method, the gradient ranged from 10% B at 0–6 min, to 95% B at 6–8 min, and back to 10% B at 8.1–10 min, resulting in a 10-minute run. For the ETU method, the gradient varied from 10% B at 0–3 min, up to 100% B at 3–9 min, 100% B at 9–11 min, and back to 10% B at 11–11.50 min, with a total run time of 14 minutes.
Dry herb samples
One hundred and three samples of 33 types of dry herbs were acquired between 2018 and 2023 from different establishments and compounding pharmacies in the Federal District (Table S1, ESI†): artichoke (Cynara scolymus, n = 8); blackberry (Morus nigra, n = 2); angelica (Angelica officinalis L., n = 3); “arnica-do-mato” (Arnica montana, n = 1); mountain arnica (Solidago microglossa n = 2); “assa-peixe” (Vernonia polyanthes, n = 1); “barbatimão” (Stryphnodendron barbatiman, n = 1); boldo (Peumus boldus, n = 7); chamomile (Matricaria chamomilla/Matricaria recutita, n = 9); “canela-de-velho” (Miconia albicans, n = 2); carqueja (Baccharis trimera, n = 1); cascara buckthorn (Rhamnus purshiana, n = 2); horse chestnut (Aesculus hippocastanum, n = 1); horsetail (Equisetum arvense/Equisetum hyemale, n = 6); gotu kola (Hydrocotyle asiatica, n = 2); green tea (Camellia sinensis, n = 7, including a sample of green tea + bitter orange); leather hat (Echinodorus macrophyllus, n = 3); chlorella (Chlorella pyrenoidosa, n = 2); comfrey (Symphytum officinale, n = 1); “espinheira-santa” (Maytenus ilicifolia, n = 3); bladderwrack (Fucus vesiculosus, n = 2); ginkgo (Ginkgo biloba, n = 4); guarana (Paullinia cupana, n = 3); hibiscus (Hibiscus rosa-sinensis/Hibiscus sabdariffa, n = 2); Peruvian maca (Lepidium meyenii, n = 1); muira puama (Ptychopetalum olacoides, n = 2); organic moringa (Moringa oleifera, n = 1); mulungu (Erythrina velutina/Erythrina mulungu, n = 2); passion fruit (Passiflora incarnata/Passiflora alata, n = 2); myrcia (Myrcia multiflora, n = 1); senna (Senna alexandrina/Cassia angustifolia Vahl./Cassia acutifolia, n = 10); spirulina (Arthrospira (Spirulina) platensis, n = 2); puncture vine (Tribulus terrestris, n = 1); cat's claw (Uncaria tomentosa, n = 6).
Upon arrival at the laboratory, the samples were stored at room temperature, processed, and homogenized in a blender (leaves, stems, and flowers) or mill (stem and bark), except for powdered products, which were only homogenized before weighing and analysis. The selection of medicinal herbs investigated was based on the Memento Fitoterápico,29 National List of Essential Medicines, RENAME,30 Braga and Silva,31 and an informal survey conducted in compounding pharmacies and establishments on the best-selling herbs in the Federal District.
Method validation
A mixture of different types and parts of dry herbs was used as a control sample to validate the methods. The selection of the plants to include in the control sample is described in Mello et al.32 and included leaves/barks of boldo, artichoke, “espinheira-santa”, cat's claw and senna, flowers and stems of chamomile and bark of cascara buckthorn and cat's claw. All selected herbs were previously tested using the optimized methods and gave negative results for EBDC and ETU.
The parameters selectivity, linearity, matrix effect, recovery, repeatability, and intermediate precision were evaluated.33,34 As mancozeb is the most used fungicide in Brazil, and with the largest number of crops for human consumption registered in the country (70 crops),6 it was used as a representative compound of EBDC (mancozeb and metiram) for method validation and quality control (QC) for method performance check during routine analysis. EBDC-dimethyl (analytical standard) was used to prepare the analytical curves.
The selectivity of the methods was evaluated by checking the presence of interferents in the control sample and the solvents at the same retention time as the monitored ions.
The matrix effect was evaluated by comparing the analytical curve prepared in control sample extract with the analytical curve prepared in acetonitrile or MeOH
:
H2O (50
:
50) for EBDC and ETU methods, respectively (8 levels and 3 replicates in the EBDC method and 5 levels and 3 replicates in the ETU method). The percentage of the matrix effect was calculated from the equation [% EM = ((analyte peak area in the matrix/analyte peak area in the solvent) − 1) × 100].34 Values below and above 0% indicate signal suppression and enhancement, respectively, and values below ±20% were considered acceptable.
Linearity was evaluated by analyzing analytical curves prepared in a control sample extract. In the EBDC method, curves were prepared at eight levels and three replicates for each level (3; 5; 15; 25; 40; 60; 80; 100 ng mL−1). In the ETU method, each of the five calibration levels was analyzed in three replicates (1.5; 2.5; 5; 10; 20 ng mL−1). The least squares method was used to estimate linear regression, the Grubbs test to verify the presence of outliers, the Cochran test for homogeneity of variances, and ANOVA to determine the correlation coefficients (r) and the significance of the regressions.33 Curves with heteroscedastic behavior were adjusted by testing different weighted linear regressions and selecting the most appropriate weighting factor (wi).35 The weighting factors 1/x, 1/x2, 1/x0.5, 1/y, 1/y2, and 1/y0.5 were tested, and the selection of the most appropriate factor was carried out by calculating the percentage of relative error (% RE) for each wi, according to the equation: % RE = [(Cexperimental − Cnominal)/Cnominal] × 100. The % RE compares the experimental concentration (Cexperimental), calculated from the equation of the straight line obtained for the factors of weighting tested, with the theoretical concentration (Cnominal). The best wi is the one that generates the lowest % RE.36
Recovery (%) and repeatability (relative standard deviation, % RSD) were evaluated by fortifying the control sample at four concentration levels (n = 5 for each level), on the same day, by the same analyst. Levels 0.03; 0.05; 0.15; and 0.40 mg kg−1 were evaluated in the EBDC method and 0.03; 0.05; 0.10; and 0.20 mg kg−1 in the ETU method. Intermediate precision (% RSD) was evaluated by repeating the recovery experiment for each method by the same analyst on a different day.
The limits of quantification (LOQ) of the methods were defined as the lowest level where acceptable recoveries (70–120%), repeatability and intermediate precision (RSD ≤ 20%) were achieved.33,34 The limit of detection (LOD) was set at 1/3 of the LOQ. Compounds detected at levels ≥LOD but <LOQ were reported as traces. Knowing that 1 mole of mancozeb (271.2 g) yields 2 moles of CS2 (152.3 g), a conversion factor of 0.56 was applied to convert mancozeb values to CS2.
To verify the efficiency of EBDC decomposition/complexation and methylation, a test was conducted, in which the chromatograms of EBDC-dimethyl standard solution were compared with chromatograms of EBDC-dimethyl solutions resulting from the methylation of mancozeb, metiram, and the mixture of mancozeb and metiram, all at a concentration of 15 ng mL−1 in acetonitrile. The experiment was carried out on two different days.
Results and discussion
EBDC method
Dithiocarbamates from the EBDC subclass are insoluble in organic solvents, therefore, complexing agents such as EDTA can be used during sample preparation to transform the EBDC compounds into their respective water-soluble sodium salt. EDTA acts as a chelator of the metals present in the EBDC structure, allowing its anions to become accessible for analysis.20,37,38 Sodium salts formed after treatment with EDTA can react with co-extractives present in the samples, which decreases recovery. To solve this problem, Gustafsson and Fahlgren39 introduced L-cysteine to the process, which acts as an antioxidant stabilizer of sodium salts (Fig. 3). Furthermore, Gustafsson and Thompson40 reported that the efficiency of transforming EBDC into sodium salts decreases if the pH of the medium is lower than 9.5, a phenomenon also observed by Hayama and Takada20 during the analysis of acidic samples, such as tangerine and strawberry. Therefore, a L-cysteine–EDTA solution with the pH in the range of 9.6–10.0,24 was used in the EBDC decomposition/complexation and the hydration of dry herb samples.
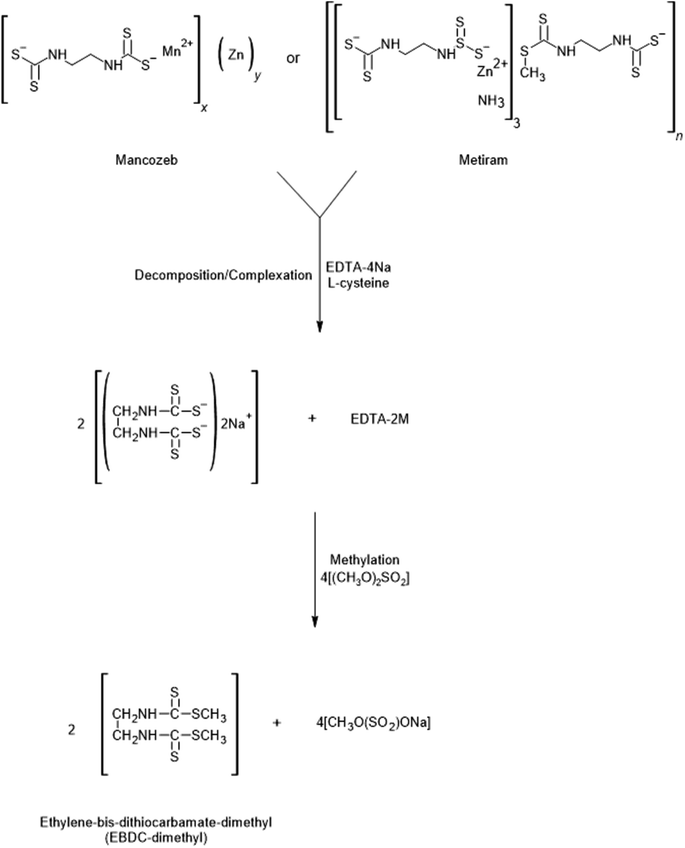 |
| Fig. 3 Chemical reaction showing the decomposition/complexation and methylation of ethylene-bis-dithiocarbamates. M = Mn and Zn for mancozeb and Zn for metiram, resulting in EBDC-dimethyl. | |
After the decomposition/complexation step, a dimethyl sulfate solution in acetonitrile was added to methylate the compounds (mancozeb and/or metiram), transforming them into EBDC-dimethyl (Fig. 3). The use of iodomethane as a methylation reagent dissolved in non-polar solvents has also been reported,39,40 but it gives lower derivatization yields compared to dimethyl sulfate.20 Furthermore, the acetonitrile extract obtained after sample preparation can be directly injected into the UHPLC-MS/MS, reducing the number of steps in the procedure. Hence, like other studies available in the literature,24,41 dimethyl sulfate in ACN (0.05 mol L−1) was selected as the methylation reagent.
The EBDC decomposition (complexation)/methylation test showed that the area of the mixture of two post-methylation EBDC compounds (mancozeb and metiram) is twice the area of the chromatograms of each methylated EBDC injected separately, confirming the efficiency of the reactions for both EBDCs (Fig. S1†).
ETU method
Different elution types and solvents were evaluated to obtain the best conditions for ethylenethiourea determination. Initially, isocratic elution was tested with a mobile phase composed of 5% water + 5 mM ammonium formate and 95% ACN, flow rate of 0.2 mL min−1, injection volume of 5 μL, and column oven at 30 °C.26 Under these conditions, instability in the baseline intensity and poor repeatability at the quantification transition (m/z 103 > 44) were observed, which also affected the chromatographic peak intensity.
ACN was then replaced by methanol as the organic phase under the same chromatographic conditions evaluated previously. Although the baseline remained stable over the days, the chromatographic efficiency decreased compared to when ACN was used as the mobile phase, affecting the signal response. Gradient elution was then used (A: water + 5 mM ammonium formate + 0.1% formic acid and B: MeOH + 5 mM ammonium formate + 0.1% formic acid: 0–3 min 10% B, 3–9 min up to 100% B, 9–11 min 100% B, 11–14 min, 10% B; run time: 14 min; flow rate: 0.2 mL min−1; injection volume: 1 μL), and the oven temperature was adjusted to 40 °C to reduce system pressure. Fig. S2† shows the extracted ion chromatograms of a 20 ng mL−1 ETU solution (in the control sample) using isocratic and gradient elution. The analysis of the chromatograms showed an improvement in the peak shape when the gradient method was used, which was selected for this study.
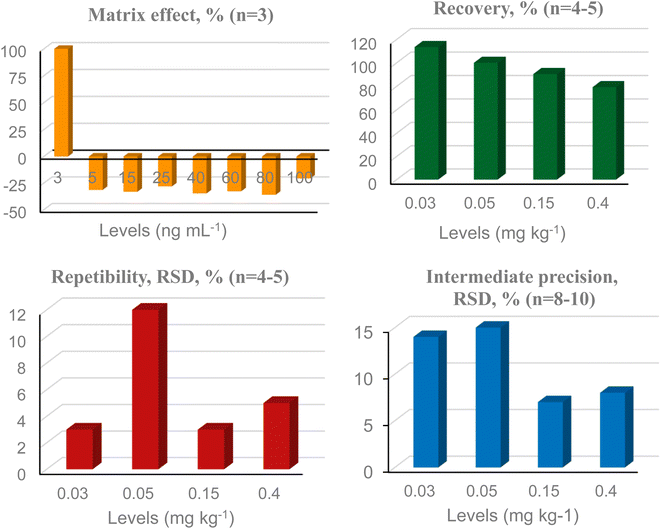 |
| Fig. 4 Summary of validation data for EBDC (mancozeb) in dry herbs by UHPLC-MS/MS. RSD = relative standard deviation. | |
Low recovery of ETU was initially observed (<40%), which was overcome after adding 0.5 g of Cys-HCl to the sample extraction, which prevents ETU from degrading into ethyleneurea or other compounds.42
Method validation
No interferents were observed in the control sample at the same retention time of the monitored ions, both for EBDC-dimethyl and ETU, indicating satisfactory selectivity. The matrix effect was greater than ±20% for all 8 concentration levels evaluated in the EBDC method, with signal suppression at all levels, except for the lowest, where a clear signal increase was observed (Fig. 4). Therefore, a matrix-matched analytical curve was used for quantification of EBDC-dimethyl. In the ETU method, the matrix effect reached −19% at the highest level (Fig. 5), and despite being less than 20%, it was decided to also use a matrix-matched analytical curve. Linearity evaluation showed heteroscedastic behavior for both methods (Ccalculated > Ctabulated;5;3) and weighting factors 1/x2 and 1/x were selected to quantify EBDC and ETU, respectively. The correlation coefficient (r) was satisfactory for both methods, with values ≥0.99.33Fig. 4 and 5 summarize the results of recovery, repeatability, and intermediate precision for EBDC and ETU methods, respectively. Recovery ranged from 70 to 120% for all evaluated levels (EBDC: 79–113%; ETU: 81–109%), and repeatability and intermediate precision results (% RSD) were less than 20%, with the LOQ defined at 0.03 mg kg−1 for both compounds. The LOQ of EBDC, expressed in CS2, was 0.02 mg kg−1 CS2. Sayed et al.24 obtained a LOQ of 0.05 mg kg−1 for EBDC and 0.03 mg kg−1 CS2 for chamomile (dry herb) by UHPLC-MS/MS and as far as we know, there are no other studies in the literature on the specific determination of dithiocarbamates in herbs using this technique. A method for determining dithiocarbamates in yerba mate (Ilex paraguariensis) by GC-MS (as CS2) was validated by da Silva et al.,43 with a LOQ of 0.1 mg kg−1 (extraction with isooctane, tin chloride II, and hydrochloric acid).
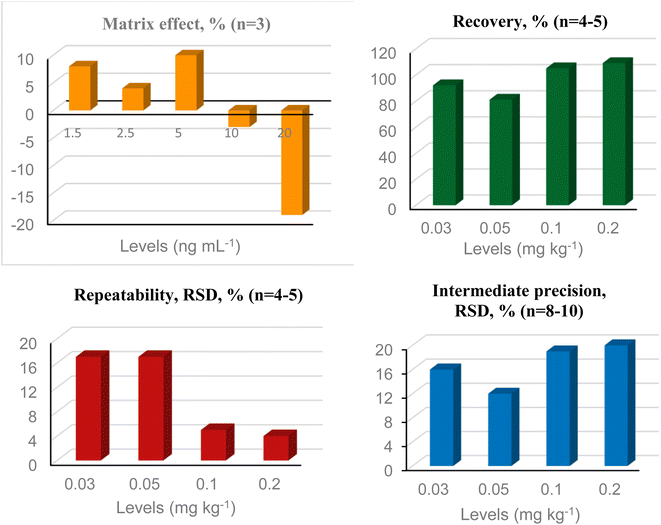 |
| Fig. 5 Summary of validation data for ETU, a degradation product of EBDCs, in dry herbs by UHPLC-MS/MS. RSD = relative standard deviation. | |
The analysis of pesticides in dry herbs presents challenges due to their low water content (<25%) and the presence of numerous co-extractives in the matrix, including lipids, chlorophyll, sugars, and natural pigments.44–46 Methods for determining dithiocarbamate compounds in fruits and vegetables have been reported in the literature with much lower LOQs. Hayama and Takada20 validated a method for determining EBDC by LC-MS/MS in persimmon, pear, strawberry, cabbage, lettuce, and spinach, with a LOQ of 0.0008 mg kg−1 for maneb (decomposition/complexation and methylation with EDTA, L-cysteine, and dimethyl sulfate solution in acetonitrile, extraction with MgSO4 and NaCl, and clean-up with PSA). Al-Alam et al.21 developed a method for determining dazomet, metam-sodium, dimethyldithiocarbamates, EBDC, and propineb in vegetables, with the LOQ ranging from 0.00013 to 0.00066 mg kg−1. The method combined techniques of ion-pair methylation, detection by HPLC at 272 nm, and atomic absorption spectrometry (decomposition/complexation, methylation, and extraction with EDTA, L-cysteine, and iodomethane in chloroform–hexane). Kakitani et al.22 validated a method for determining 10 dithiocarbamates in beer, fruit juice, and malt samples by LC-MS/MS, with the LOQ ranging between 0.00014 and 0.00697 mg kg−1 (conversion of dithiocarbamates into sodium salts in the presence of sodium bicarbonate and methylation with dimethyl sulfate; extraction by QuEChERS).
Sample analysis
The two validated methods were applied in the analysis of 103 samples from 33 types of dry herbal plants. The performance of the methods during sample analysis was evaluated through the inclusion of mancozeb fortified control samples (quality control, QC) in each analysis batch at 2 different levels and two replicates for each level (0.03 and 0.15 mg kg−1 for EBDC; 0.03 and 0.1 mg kg−1 for ETU). Recovery of QC samples was 80–110% for EBDC and ranged from 72 to 80% for the ETU method. Of the 103 samples analyzed for EBDC, approximately 19.4% (20 samples) were positive, with concentrations varying between traces and 1.05 mg kg−1 of EBDC (0.59 mg kg−1 CS2). The herbs with the highest number of positive samples were ginkgo (4 samples) and green tea (4 samples). The results of the positive samples for EBDC are shown in Table 2. No residues of ETU were detected in any of the analyzed samples (Table S1†).
Table 2 Results of dry herb positive samples for EBDC, analyzed by UHPLC-MS/MSa
Sample |
EBDC (mg kg−1) |
CS2 (mg kg−1) |
Traces: ≥LOD (0.01 mg kg−1) and <LOQ (0.03 mg kg−1); concentrations of EBDC, as mancozeb, converted to CS2.
|
Artichoke |
0.22 |
0.13 |
Artichoke |
Traces |
Traces |
Arnica |
Traces |
Traces |
Arnica |
Traces |
Traces |
Arnica |
Traces |
Traces |
Chamomile |
0.06 |
0.03 |
Chamomile |
0.14 |
0.08 |
Horsetail |
Traces |
Traces |
Horsetail |
0.09 |
0.05 |
Gotu koka |
0.35 |
0.20 |
Green tea |
Traces |
Traces |
Green tea |
0.03 |
0.02 |
Green tea |
Traces |
Traces |
Green tea + bitter orange |
Traces |
Traces |
Ginkgo biloba |
0.07 |
0.04 |
Ginkgo biloba |
0.04 |
0.02 |
Ginkgo biloba |
Traces |
Traces |
Ginkgo biloba |
1.05 |
0.59 |
Mulungu |
Traces |
Traces |
Senna |
0.03 |
0.02 |
Among the studied crops, mancozeb and metiram are registered for use only in guarana, seed (mancozeb) and passion fruit, fruit (mancozeb and metiram), with MRL of 3 mg kg−1 of CS2 (dithiocarbamates).6 Mozzaquatro et al.47 detected the presence of dithiocarbamate residues (as CS2 levels) in 40% of the analyzed fresh passion fruit leaf samples and the drying process of the leaves, one of the stages of passiflora production, increased the levels of CS2 by up to 60%. However, no residues of EBDC were found in the two passion fruit dried leaf samples analyzed in this study.
The Brazilian Pharmacopoeia includes a list containing pesticide limits for 71 pesticides in medicinal herbs,48 which is the same as the European Pharmacopoeia, including for dithiocarbamates, at 2 mg kg−1 of CS2, a value 10 times greater than the EBDC LOQ (0.02 mg kg−1 of CS2) in the present study. However, dithiocarbamates or any pesticide should not be applied if the goal is to use the crop as a medicinal plant or in the preparation of herbal medicine.4
The Codex Alimentarius established MRL for 5 pesticides in herbs, with levels varying between 0.01 (for abamectin) to 70 mg kg−1 (for azoxystrobin), but does not include dithiocarbamates.51 Brazilian Pharmacopeia and ANVISA do not establish maximum residue limits of ethylenethiourea in food or any matrix, and no studies that analyzed ETU analysis in dry herbs were found in the literature.
In Brazil, dithiocarbamates are not registered for use in any of the herbs whose result was positive for EBDC,6 therefore, the results indicate illegal use of pesticides or cross-contamination. EBDC was found in most samples at trace levels (≥LOD and <LOQ), which may indicate drifting from nearby crop applications. The low concentrations found for EBDC can also justify the non-detection of its degradation product ETU, in addition to the fact that ethylenethiourea can degrade into ethyleneurea or other compounds also during storage.42 The highest concentration of EBDC detected was 0.59 mg kg−1 of CS2, a value that did not exceed the limit of dithiocarbamates for medicinal herbs of 2 mg kg−1 of CS2 established in the Brazilian Pharmacopoeia.48
Mancozeb is the third best-selling pesticide in Brazil,7 and is registered in a larger number of human consumption crops (70), followed by thiram (27, seed application) and metiram (17). Therefore, although it is not possible to identify by the developed method which EBDC was applied in the crop, it is likely that crops have been treated with mancozeb.
Chronic exposure assessment
The daily intake of EBDC fungicides through the consumption of herbs was estimated for herbs with at least one positive samples. For samples with trace levels (≥LOD and <LOQ), the concentration was considered as ½LOQ for the calculation of the averages of the concentrations for each herb. Chronic intake calculation is made through the equation: (pesticide concentration × intake)/body weight.49,50 Not all dry herb samples had consumption instructions on the product label, therefore, in a conservative approach, a daily consumption of 2 tablespoons (30 g) indicated on some labels was assumed for all evaluated herbs. The intake estimate considered the consumption of the dry herb by a person weighing 60 kg. The values found were compared with the acceptable daily intake (ADI) for the EBDC (mancozeb and metiram, 0.03 mg kg−1 bw).6
The results of the risk assessment show that the intake of the EBDC found in the samples does not indicate a potential risk to consumers, representing less than 1% of the ADI (Table 3). Jardim et al.52 carried out a risk assessment of dithiocarbamates in the Brazilian population, assuming that 93% of the CS2 detected in the evaluated food samples came from the use of mancozeb or metiram, and 7% from the use of propineb. In this approach, the total intake represented less than 7% of EBDC ADI, not representing a risk to consumers. The results of the present study confirm this conclusion, even considering the inclusion of the consumption of herbs in the Brazilian diet. Although EBDC residues were detected in three samples of “arnica”, the Pharmacopoeia of Herbal Medicines recommends only the external use of the products of this plant.53 Therefore, the samples were not included in Table 3.
Table 3 Risk assessment of exposure to EBDC through the consumption of dry herbsa
Sample |
Mean concentration,b mg kg−1 (n) |
Intake, μg kg−1 bw (% ADI) |
ADI: acceptable daily intake; bw: body weight.
Calculated for the positive samples, with trace levels considered as ½LOQ.
|
Artichoke |
0.05 (3) |
0.03 (0.1) |
Chamomile |
0.04 (3) |
0.02 (0.1) |
Horsetail |
0.03 (2) |
0.02 (0.09) |
Gotu koka |
0.2 (1) |
0.1 (0.6) |
Green tea |
0.01 (4) |
0.006 (0.04) |
Green tea + bitter orange |
0.01 (1) |
0.005 (0.03) |
Ginkgo biloba |
0.2 (4) |
0.08 (0.5) |
Mulungu |
0.01 (1) |
0.005 (0.03) |
Senna |
0.02 (1) |
0.01 (0.06) |
Residues of ETU were not found in the evaluated samples, but it is important to note that for risk assessment, the JMPR (Joint FAO/WHO Meeting on Pesticide Residues) includes the metabolite ETU in the residue definition for dietary intake assessment, as it is 7.5 times more toxic than mancozeb. Thus, if ETU had been detected in the samples, its residues should be expressed as “mancozeb toxicity equivalents”, applying a factor of 7.5 (ratio between the ADIs of mancozeb and ETU) to the obtained ETU concentrations.54
Conclusion
Methods for the determination of EBDC and ethylenethiourea in dry herbs by UHPLC-MS/MS were satisfactorily validated using a control sample containing 7 different plants. The methods were applied for the analysis of 103 samples of dry herbs, with 19.4% positive for EBDC and no sample containing ETU. The exposure to EBDC through the consumption of dry herbs did not indicate a risk to health. To the best of our knowledge, this is the first work that evaluated the presence of EBDC and ETU in dry herbs. The analysis of these compounds is important, given that mancozeb is the third most marketed pesticide in Brazil and ethylenethiourea, one of its degradation products, is responsible for its chronic toxicity. Dry herbs are not included in the pesticide monitoring programs in Brazil and the results of this work showed possible illegal use of dithiocarbamates in these plants, which reinforces the importance of investigating these herbs for pesticide residues.
Data availability
Requests to access the data should be directed to the corresponding author.
Conflicts of interest
There are no conflicts to declare.
Acknowledgements
This project was financially supported by the Brazilian Ministry of Justice and Public Security (MJ; TED FDD No. 58/2019). D. C. Mello received a PhD scholarship from the Coordination for the Improvement of Higher Education Personnel (CAPES) and the Foundation for Research Support of the Federal District (FAP-DF).
References
-
M. Z. Almeida, Plantas medicinais: abordagem histórico-contemporânea, in Plantas Medicinais, EDUFBA, Salvador, 3rd edn, 2011, pp. 34–66, ISBN 978-85-232-1216-2, 221pp, available from https://books.scielo.org/id/xf7vy/pdf/almeida-9788523212162-03.pdf, accessed June 19 2024 Search PubMed.
- M. I. Tomazzoni and R. R. B. Negrelle, Fitoterapia popular: a busca instrumental enquanto prática terapêuta, Texto & Contexto – Enfermagem, 2006, 15(1), 115–121 Search PubMed.
- N. S. Shaban, K. A. Abdou and N. E.-H. Y. Hassan, Impact of toxic heavy metals and pesticide residues in herbal products, Beni-Suef University Journal of Basic and Applied Sciences, 2016, 5(1), 102–106 CrossRef.
-
ANVISA (Agência Nacional de Vigilância Sanitária), Análise de resíduos de pesticidas em fitoterápicos - perguntas e respostas, 3rd edn, 2019, available from: https://www.gov.br/anvisa/pt-br/setorregulado/regularizacao/medicamentos/fitoterapicos-dinamizados-e-especificos/informes/fitoterapicos/faq_agrotoxicos_fito_3ed_publicar_limpo.pdf, accessed on June 16, 2024 Search PubMed.
- BRASIL, Ministério da Saúde, Agência Nacional de Vigilância Sanitária, Resolução RDC N° 26, de 13 de maio 2014, Dispõe sobre o registro de medicamentos fitoterápicos e o registro e a notificação de produtos tradicionais fitoterápicos, Diário Oficial da União, 13/05/2014.
-
ANVISA (Agência Nacional de Vigilância Sanitária), Monografia de Agrotóxicos, 2024, available from: https://www.gov.br/anvisa/pt-br/setorregulado/regularizacao/agrotoxicos/monografias, accessed June 19, 2024 Search PubMed.
-
IBAMA (Instituto Brasileiro do Meio Ambiente e dos Recursos Naturais Renováveis), Relatório de Comercialização de pesticidas, 2024, available from: https://www.gov.br/ibama/pt-br/assuntos/quimicos-e-biologicos/agrotoxicos/relatorios-de-comercializacao-de-agrotoxicos, accessed June 19, 2024 Search PubMed.
- A. N. O. Jardim and E. D. Caldas, Brazilian monitoring programs for pesticide residues in food - results from 2001 to 2010, Food Control, 2012, 25, 607–616 CrossRef CAS.
-
ANVISA (Agência Nacional de Vigilância Sanitária), Programa de Análise de Resíduos de Pesticidas em Alimentos (PARA), 2023, available from: https://www.gov.br/anvisa/pt-br/assuntos/agrotoxicos/programa-de-analise-de-residuos-em-alimentos/relatorios-do-programa, accessed June 19 2024 Search PubMed.
- D. R. Doerge and R. S. Takazawa, Mechanism of thyroid peroxidase inhibition by ethylenethiourea, Chem. Res. Toxicol., 1990, 3, 98–101 Search PubMed.
-
F. M. Rubino, E. J. Mrema and C. Colosio, Pesticide Residues: Dithiocarbamates, in Encyclopedia of Food Safety, 2013, vol. 3, pp. 5–10 Search PubMed.
- E. A. Kazos, C. D. Stalikas, C. G. Nanos and C. N. Konidari, Determination of dithiocarbamate fungicide propineb and its main metabolite propylenethiourea in airborne samples, Chemosphere, 2007, 68, 2104–2110 CrossRef CAS PubMed.
-
IPCS/INCHEM, Ethylenethiourea (ETU), 1993, available from https://www.inchem.org/documents/jmpr/jmpmono/v93pr08.htm, acessed June 19 2024 Search PubMed.
-
IARC (International Agency for Research on Cancer), IARC Monographs on the Evaluation of Carcinogenic Risks to Humans - Ethylenethiourea, IARC Press, Lyon, 2001, vol. 79, pp. 659–701 Search PubMed.
- C. Campanale, M. Triozzi, A. Ragonese, D. Losacco and C. Massarelli, Dithiocarbamates: Properties, Methodological Approaches and Challenges to Their Control, Toxics, 2023, 11(10), 851 CrossRef CAS PubMed.
-
European Commission, EU - Pesticides Database, available from: https://ec.europa.eu/food/plant/pesticides/eu-pesticides-database/public/?event=homepage&language=EN, accessed June 19 2024 Search PubMed.
-
FSANZ (Food Standards Australia New Zealand), Schedule 20 Maximum Residue Limits, 2023, available from: https://www.legislation.gov.au/Series/F2015L00468, accessed June 19 2024 Search PubMed.
-
FAO Food and Agricultural Organization/World Health Organization (FAO/WHO), Pesticide residues in food. Joint FAO/WHO meeting on pesticide residues, in Report of the Joint Meeting of the FAO Panel of Experts on Pesticides in Food and the Environment and the WHO Cores Assessment Group on Pesticide Residues, 1993, available from: https://www.fao.org/pest-and-pesticide-management/guidelines-standards/faowho-joint-meeting-on-pesticide-residues-jmpr/reports/en/, accessed June 19 2024 Search PubMed.
-
IPCS (International Program on Chemical Safety), Pesticide residues in food-1993 evaluations. Part II, 1993, available from: http://www.inchem.org/documents/jmpr/jmpmono/v93pr01.htm, accessed June 19 2024.
- T. Hayama and M. Takada, Simple and rapid method for the determination of ethylenebisdithiocarbamate fungicides in fruits and vegetables using liquid chromatography with tandem mass spectrometry, Anal. Bioanal. Chem., 2008, 392, 969–976 CrossRef CAS PubMed.
- J. Al-Alam, L. Bom, A. Chbani, Z. Fajloun and M. Millet, Analysis of Dithiocarbamate Fungicides in Vegetable Matrices Using HPLC-UV Followed by Atomic Absorption Spectrometry, J. Chromatogr. Sci., 2017, 55(4), 429–435 CAS.
- A. Kakitani, T. Yoshioka, Y. Nagatomi and K. Harayama, A rapid and sensitive analysis of dithiocarbamate fungicides using modified QuEChERS method and liquid chromatography–tandem mass spectrometry, J. Pestic. Sci., 2017, 42(4), 145–150 CrossRef CAS PubMed.
- H. Yang, P. Gong, J. Wang, D. Wang, X. Xue and W. Yu, Determination of four ethylene bis-dithiocarbamates in vegetables and fruits by UPLC-MS/MS, Chinese Journal of Food Hygiene., 2023, 35(1), 79–85 Search PubMed.
- R. Sayed and O. E. Hussein, Method optimization and validation for the determination of mancozeb in chamomile by modified QuEChERS and liquid chromatography–tandem mass spectrometry, J. Food Compos. Anal., 2022, 111, 104646 CrossRef CAS.
- Y. Zhang, Z. H. Zhang, A. M. Li, Y. F. Wang, X. D. Cheng and W. W. Zhao, Determination of mancozeb in Schisandra chinensis (Turcz.) bail. by methylation derived UPLC, Hubei Agricultural Sciences, 2015, 54(12), 3000–3002 Search PubMed.
- L. Zhou, X. Liu, S. Kang, F. Zhang and C. Pan, A rapid determination method for ethylenethiourea in potato and cucumber by modified QuEChERS – high performance liquid chromatography–tandem mass spectrometry, Food Chem., 2013, 138(2–3), 1355–1359 CrossRef CAS PubMed.
- O. López-Fernández, R. Rial-Otero, A. Cid and J. Simal-Gándara, Combined determination and confirmation of ethylenethiourea and propylenethiourea residues in fruits at low levels of detection, Food Chem., 2014, 145, 1002–1010 CrossRef PubMed.
- R. Garcinuño, P. Fernández-Hernando and C. Cámara, Simultaneous determination of maneb and its main metabolites in tomatoes by liquid chromatography using diode array ultraviolet absorbance detection, J. Chromatogr. A, 2004, 1043(2), 225–229 CrossRef PubMed.
-
ANVISA (Agência Nacional de Vigilância Sanitária), Memento Fitoterápico da Farmacopeia Brasileira, 1st edn, 2016, available from: https://www.gov.br/saude/pt-br/composicao/sectics/daf/pnpmf/publicacoes/memento-fitoterapico-da-farmacopeia-brasileira/view, accessed June 19 2024 Search PubMed.
- BRASIL, Ministério da Saúde, Secretaria de Ciência, Tecnologia, Inovação e Insumos Estratégicos em Saúde, Departamento de Assistência Farmacêutica e Insumos Estratégicos, Relação Nacional de Medicamentos Essenciais: RENAME, 2020, p. 217.
- J. C. B. Braga and L. R. Silva, Consumption of medicinal plants and herbal medicines in Brazil: consumer profile and its relationship with the COVID-19 pandemic, Brazilian Journal of Health Review, 2021, 4(1), 3831–3839 CrossRef.
- D. C. Mello, N. L. Pires, C. S. Evangelista and E. D. Caldas, Pesticide residues in dry herbs used for tea preparation by UHPLC-MS/MS: method validation and analysis, J. Food Compos. Anal., 2024, 125, 105817 CrossRef CAS.
-
INMETRO (Instituto Nacional de Metrologia, Normalização e Qualidade Industrial), Orientações sobre Validação de Métodos de Ensaios Químicos, DOQ-CGCRE-008, Revisão: 09, 2020 Search PubMed.
-
SANTE, Guidance Document on Analytical Quality Control and Method Validation Procedures for Pesticide Residues Analysis in Food and Feed (SANTE 11212/2021), Directorate General for Health and Food Safety, European Commission, Health & Consumer Protection Directorate-General, 2021, implemented by 01.01.2022 Search PubMed.
-
J. N. Miller and A. Ambrus, Statistics in calibration analysis, in Manual on Basic Statistics, FAO/IAEA Training and Reference Centre for Food and Pesticide Control, Vienna, Australia, 2000, ch. 9, pp. 1–18 Search PubMed.
- A. M. Almeida, M. M. Castel-Branco and A. C. Falcão, Linear regression for calibration lines revisited: weighting schemes for bioanalytical methods, J. Chromatogr. B: Anal. Technol. Biomed. Life Sci., 2002, 774, 215–222 CrossRef CAS PubMed.
- G. Crnogorac and W. Schwack, Residue analysis of dithiocarbamate fungicides, Trends Anal. Chem., 2009, 28(1), 40–50 CrossRef CAS.
- S. W. C. Chung and W. W. K. Wong, Chromatographic analysis of dithiocarbamate residues and their metabolites in foods employed in dietary exposure studies—a review, Food Addit. Contam.: Part A, 2022, 39(10), 1731–1743 CrossRef CAS PubMed.
- K. H. Gustafsson and C. H. Fahlgren, Determination
of dithiocarbamate fungicides in vegetable food stuffs by high-performance liquid chromatography, J. Agric. Food Chem., 1983, 31(2), 461–463 CrossRef CAS PubMed.
- K. H. Gustafsson and R. A. Thompson, High-pressure liquid chromatographic determination of fungicidal dithiocarbamates, J. Agric. Food Chem., 1981, 29(4), 729–732 CrossRef CAS PubMed.
- O. López-Fernández, R. Rial-Otero, C. González-Barreiro and J. Simal-Gándara, Surveillance of fungicidal dithiocarbamate residues in fruits and vegetables, Food Chem., 2012, 134(1), 366–374 CrossRef.
- H. Kobayashi, M. Nishida and O. Matano, Effect of cysteine on the stability of ethylenebisthiourea and ethylenebis(dithiocarbamate) in crops during storage and/or analysis, J. Agric. Food Chem., 1992, 40(1), 76–80 CrossRef CAS.
- R. C. da Silva, I. D. dos Santos, J. P. Neu, R. D. Wouters, M. E. Z. Fontana, P. D. R. Balbinot, R. Wagner and I. R. Pizzutti, Commercial yerba mate (Ilex paraguariensis) produced in South America: determination of dithiocarbamate residues by gas chromatography-mass spectrometry, Food Chem., 2022, 394, 133513 CrossRef CAS PubMed.
- M. S. Abbas, A. Sh Soliman, H. A. El-Gammal, M. E. Amer and E. R. Attallah, Development and validation of a multiresidue method for the determination of 323 pesticide residues in dry herbs using QuEChERS method and LC-ESI-MS/MS, Int. J. Environ. Anal. Chem., 2017, 97(11), 1003–1023 CrossRef CAS.
- S. B. A. Ghani, Determination of multiclass pesticides in dry herbs using GC-ECD, Journal of Applied Science and Agriculture, 2014, 9(3), 955–959 Search PubMed.
- E. Rutkowska, B. Łozowicka and P. Kaczyński, Modification of multiresidue QuEChERS protocol to minimize matrix effect and improve recoveries for determination of pesticide residues in dried herbs followed by GC-MS/MS, Food Anal. Methods, 2018, 11, 709–724 CrossRef.
- J. O. Mozzaquatro, D. C. Mello, R. C. S. Oliveira, R. C. C. Rosa, A. M. Costa and E. D. Caldas, Dithiocarbamate Residues in Fruits and Leaves of Passion Fruit (Passiflora edulis) from Different Brazilian Regions, J. Braz. Chem. Soc., 2019, 30(9), 1834–1840 CAS.
-
ANVISA (Agência Nacional de Vigilância Sanitária), Farmacopeia Brasileira, 6th edn, 2019, available from: https://www.gov.br/anvisa/pt-br/assuntos/farmacopeia/farmacopeia-brasileira, accessed June 19 2024 Search PubMed.
-
WHO World Health Organization, Principles and methods for the risk assessment of chemicals in food, Environmental Health Criteria 240, 2009 Search PubMed.
-
E. D. Caldas and T. V. D. Velde-Koerts, Dietary exposure and risk characterization for pesticide residues in food, in Food Safety Assessment of Pesticide Residues, ed. A. Ambrus and D. Hamilton, World Scientific Publishing Europe Ltd, 2017, ch. 6, pp. 243–267 Search PubMed.
-
Codex Alimentarius International Food Standards, available from: https://www.fao.org/fao-who-codexalimentarius/codex-texts/dbs/pestres/commodities/en/, acessed June 19 2024.
- A. N. O. Jardim, D. C. Mello, P. B. Brito, H. van der Voet, P. E. Boon and E. D. Caldas, Probabilistic dietary risk assessment of triazole and dithiocarbamate fungicides for the Brazilian population, Food Chem. Toxicol., 2018, 118, 317–327 CrossRef CAS PubMed.
-
ANVISA (Agência Nacional de Vigilância Sanitária), Formulário de Fitoterápicos da Farmacopeia Brasileira, 2nd edn, 2021, available from: https://www.gov.br/anvisa/pt-br/assuntos/farmacopeia/formulario-fitoterapico/arquivos/2021-fffb2-final-c-capa2.pdf, accessed June 19 2024 Search PubMed.
-
FAO Food and Agricultural Organization/World Health Organization (FAO/WHO), Pesticide residues in food. Joint FAO/WHO meeting on pesticide residues, in Report of the Joint Meeting of the FAO Panel of Experts on Pesticide Residues in Food and the Environment and the WHO Core Assessment Group on Pesticide Residues, 2014, available from: https://www.fao.org/3/i4245e/I4245E.pdf, June 19 2024 Search PubMed.
|
This journal is © The Royal Society of Chemistry 2024 |