DOI:
10.1039/D4AY00243A
(Paper)
Anal. Methods, 2024,
16, 4093-4103
Application of the QuEChERS method combined with UHPLC-QqQ-MS/MS for the determination of isoprocarb and carbaryl pesticides in Indonesian coffee†
Received
9th February 2024
, Accepted 20th May 2024
First published on 21st May 2024
Abstract
The performance of the QuEChERS method in this study, as indicated by a high percentage (>90%) of recovery observations falling within the range of 60–140% and a sample replicate deviation (% RSD) of <20%, for the routine analysis of isoprocarb and carbaryl pesticides, has been evaluated over a 14-month period for the export of Indonesian coffee. Following a seven-day observation of the stability of these pesticides in coffee extract, it was found that the added standard calibration solution remained stable and useable for seven days when stored at 4 °C and −20 °C. This validated method, with high sensitivity (a LOQ of 0.001 mg kg−1 for isoprocarb and carbaryl), has been employed to monitor residues in Indonesian coffee exports to comply with maximum residue limits (MRLs). The samples with higher contamination levels were predominantly from robusta coffee (57.76%), followed by arabica coffee (6.17%). The detection rates for residues decreased by more than 90% in the last two months of the method's application. In the observation of coffee processing, it was found that isoprocarb residues in contaminated samples could be transferred to the processed coffee (roasted and its infusion) to a limited extent, while residues from the carcinogenic carbaryl were not detected due to evaporation. Additionally, chronic dietary risk assessment showed that contaminated samples of robusta and arabica coffees should not be considered a significant public health concern (hazard index HI < 1). However, continuous monitoring of pesticide residues in Indonesian coffee is still recommended, not only to conform to the MRLs of importing countries but also to ensure food trade.
Introduction
QuEChERS and modified QuEChERS with sample hydration and acetonitrile as the extraction solvent are frequently used methods for determining pesticide residues in coffee beans.1–6 The analysis of 117 pesticides via LC-MS/MS was possible using QuEChERS extraction without further dispersive-SPE clean-up6 as opposed to previous methods that involved lengthy procedures to eliminate interference from coffee co-extractives.7 For example, interference was minimized by precipitating lipids and waxes through freeze-out treatment, removing caffeine with dispersive liquid–liquid micro-extraction (DLLME)1 and incorporating the ChloroFiltr sorbent in dispersive-SPE.8 Despite the availability of QuEChERS methods for detecting pesticides in raw coffee, these methods have so far only been reported in the context of initial method development and/or applications with laboratory-fortified samples.2,3,5–7,9 These conditions can differ significantly from those found in actual samples. Currently, the QuEChERS method is proving highly efficient in monitoring and controlling pesticides in coffee, as previously reported with flutriafol and pyraclostrobin, which were technical barriers to the export of Brazilian green coffee.10 Due to these issues, the QuEChERS method will be applied to other pesticides such as carbaryl and isoprocarb, which are critical to monitor in Indonesian coffee following a rejected export status.
An export rejection of Indonesian coffee was reported due to carbaryl (2009) and isoprocarb (2021) residues exceeding the Japanese standard, 0.01 mg kg−1.11,12 The occurrence of carbaryl is probably from spraying pesticides used by the farmer (Fig. S1† captures a farmer applying insecticides to coffee plants immediately before harvest) for controlling plant pests and diseases. Also, isoprocarb was used to deter ants attracted to ripe coffee cherries due to their high sugar content. Unfortunately, this treatment was deemed illegal because isoprocarb was exclusively registered for controlling mealybug pests (Planococcus citri) on coffee plants.13
Carbaryl and isoprocarb are carbamate insecticides, having different properties. Carbaryl can only act as the parent compound and does not metabolize into an active intermediate.14 This pesticide is classified as class 3, possibly carcinogenic to humans. According to the USEPA, carbaryl is considered a moderate oral toxicant (category II) with an LD50 (oral, rat) of 108–840 mg kg−1 and is listed as a group C carcinogen, implying that it is “likely to be carcinogenic in humans”.15 Isoprocarb residue may pose a risk to humans as it disrupts an enzyme that regulates acetylcholine, a neurotransmitter, because of its low toxicity to humans.16 As a carbamate insecticide, isoprocarb is thermally unstable and can decompose with increasing temperature to produce mono-aromatics and polycyclic aromatic hydrocarbons.17
As mentioned previously, due to the frequent presence of the carbamate insecticides isoprocarb and carbaryl in Indonesian coffee, it is crucial to confirm that the QuEChERS method is effective for detecting these residues in contaminated samples within the complex or “unique” matrices of coffee beans. Therefore, this paper aims to validate the QuEChERS method and evaluate its efficacy in identifying isoprocarb and carbaryl in the exports of Indonesian coffee beans. These toxic carbamate pesticides are considered hazardous to human health following coffee consumption and are a cause of export difficulties. Consequently, additional findings related to the effects of coffee processing and dietary risk assessment of these residues will be presented in this study.
Materials and methods
Chemicals
Multi-component pesticide analytical standards (LC pesticide kit and GC pesticide kit) with a concentration of 100 mg L−1 in methanol were purchased from Restek (Bellefonte, USA). These multi-component standards were used in the initial monitoring of 320 pesticide residues in coffee beans produced in several Indonesian provinces. Meanwhile the individual standards of isoprocarb (purity > 98%) and carbaryl (purity > 98%) for application in the routine analysis were obtained from Sigma-Aldrich (St. Louis, Missouri, USA). The analytical standards were stored at −20 °C. Acetonitrile and formic acid were provided by Merck (Darmstadt, Germany). Anhydrous magnesium sulfate (MgSO4) and sodium chloride (NaCl) were purchased from Agilent (Santa Clara, California, USA). Bulk sorbents of primary–secondary amine (PSA), graphitized carbon black (GCB), C18, and sodium citrate dibasic sesqui-hydrate were also purchased from Agilent. The HPLC grade water used for analysis and also for hydration of the samples was obtained using an ARIOSO water purification system (Korea).
Samples
Surveyed samples.
Green coffee bean samples used for the initial monitoring of residues were sampled from 3 coffee producing regions, namely Lampung (35 samples), Aceh (14 samples), and East Java province (31 samples) between October and November 2021.
Routine sample analysis of coffee exports.
Furthermore, pesticide residue analyses of isoprocarb and carbaryl were continuously conducted for the coffee export (1385 samples) from November 2021 to December 2022, receiving rejection notifications.
Preparation of the standard and spiked samples
The LC and GC pesticide kit stock solutions were dissolved in acetonitrile to generate a multi-component mix solution containing 320 pesticide residues at a concentration of 5 mg L−1. Then, employing appropriate fortification on each of 5 g of homogenized milling samples, standard additions (matrix based standards) were made at concentrations of 10, 20, 50, 100, and 200 μg kg−1 and prepared following the sample preparation procedure. This standard series was used to quantify the residues of the initial monitoring sample test.
Individual stock solutions of isoprocarb and carbaryl at 1000 mg L−1 were made by weighing approximately 5 mg into a 10 mL flask and diluting with acetonitrile. The preparation, storage conditions, and storage time of the stock standard followed procedures from EU guidance18 to ensure the stability and purity of the standard. The stock solutions were kept in a freezer at −20 °C. A working solution mixture of isoprocarb and carbaryl with a concentration of 5 mg L−1 was prepared by appropriate dilution in acetonitrile. Then, spiked samples for the recovery study and standard additions at the concentration levels of 1, 5, 10, 50, and 100 μg kg−1 were made by spiking working solution in 5 g of the sample in 5 g of finely ground green coffee sample. After vortexing, the solvent of working solution has definitely evaporated, cold water was added and the spiked sample was then stored at – 20 °C for 1 h to make a contaminated sample comprising the incurred real sample prior to the sample preparation procedure as we did in a previous study in another part.19 This addition standard series was used for the quantitation of routine sample analysis.
The use of standard addition could eliminate the matrix effect and improve the test result accuracy, but it will waste time and chemicals in its preparation. Since pesticides could remain stable in sample extracts used as calibrants for several days,20,21 and the stability study of isoprocarb and carbaryl in sample extracts of coffee beans was performed. The storage stability of the spike concentrations of 10, 50, and 100 μg kg−1, which were stored at 25, 4, and −20 °C in clear and amber vials, respectively, was observed for 7 days.
Sample preparation
Green coffee beans and roasted coffee.
Immediately after the green coffee samples were received at the laboratory, 500 g of the legal sample obtained by sampling was homogenized in a plastic bag and divided into two parts. The first part was stored overnight at −60 °C and ground using a Retsch mill (Germany) and the second part was stored at −20 °C for control purposes. It was ensured that the temperature didn't increase while the grinding was being conducted. The QuEChERS method, which had previously been used to analyze flutriafol and pyraclostrobin in coffee beans,10 was used to extract the samples. In the beginning, the effect of GCB on the recovery of carbaryl, which has a planar structure as highlighted by Wang et al.,22 was investigated using a standard solution of 100 mg L−1.
In a 50 mL centrifuge tube, 5 g of milled samples were weighed, and 10 mL of cold water was added. To make the sample hydrated, the mixture was left out for roughly 15 min. For extraction, 15 mL of acetonitrile was added and vortexed for 30 s, and extraction was continued for 30 min using an automated agitator. Salt (4 g MgSO4, 1 g NaCl, and 0.5 g sodium citrate dibasic sesquihydrate) was added, immediately shaken by hand, and continuously shaken in an automated agitator for 15 min. The tube was centrifuged at 7000 rpm for 5 min to obtain a partition between the water and organic layers. The organic phase (6 mL) was transferred to a 15 mL tube containing 4 g MgSO4, 0.4 g C18, 0.4 g PSA, and 0.2 g GCB. The tube was vigorously shaken for 1 min and centrifuged again. For initial monitoring of residues, 1 mL of clean acetonitrile extract was passed through a 0.22 μm PTFE syringe filter and placed in a vial for further measurement by LC-MS/MS and GC-MS/MS. Meanwhile, 3 mL of the extract was evaporated using a nitrogen evaporator until dry and then redissolved in 0.5 mL of acetonitrile for continuous specific measurements of isoprocarb and carbaryl residues. This evaporation or concentration step was used to improve the detection capability of LC-MS/MS. The same procedure was employed for roasted coffee.
Brewed coffee.
15 g of finely roasted coffee powder was brewed with 250 mL boiling water (distilled) in an Erlenmeyer flask for 10–12 min. After brewing, the flask was immersed in cold water for 10 min for cooling, followed by separating the infusion from the coffee ground by passing it through filter paper (Whatman No. 1). For pesticide determination, 10 mL of brewed coffee solution was extracted using ethyl acetate as described by R. E. Kartasasmita.23 The final residue was then redissolved using acetonitrile. For quantitation, standard addition with the concentration levels of 1.0, 2.5, 10, 20, 30, and 50 μg L−1 was used.
Instrumentation
LC-MS/MS instrument.
A Triple Quadrupole 3500 LC-MS/MS system (AB Sciex, USA) coupled with a 1290 Infinity Liquid Chromatography system (Agilent) was used to analyze pesticides amenable to LC. The multiple reaction monitoring (MRM) operation of the target list of the analytes including isoprocarb and carbaryl is summarized (Table S1†). Water and acetonitrile containing 0.1% formic acid were used as mobile phases A and B respectively. For the separation of 210 pesticides in initial monitoring, a Merck Purospher STAR RP-18 Endcapped Hibar HPLC Column (L × ID, 150 × 4.6 mm) was used with a flow rate of 0.5 mL min−1 under the following gradient conditions: 5% B (0–2 min); 5–75% B (2–5 min); 100% B (5–9 min); 100–0% B (9–12.5 min); 5% B (12–12.5 min); 5% B (12.5–16 min). Meanwhile for continuous analysis of isoprocarb and carbaryl, a Chromolith® RP-18e End-capped HPLC Column (L × ID, 100 × 3 mm) was used with a flow rate of 0.4 mL min−1 and a shorter gradient elution: 10% B (0–1 min); 10–90% B (1–4 min); 90% B (4–6 min); 90–10% B (6–7 min); 10% B (7–7.5 min).
GC-MS/MS instrument.
A Shimadzu GC-MS-TQ8050 (Shimadzu Scientific Instruments, Japan) was used to analyze 200 pesticides amenable to GC. The chromatographic separation was done using a capillary HP-5MS column. The GC operation conditions and MRM parameters for target pesticides are shown respectively in Tables S2 and S3.†
Method validation
The validation of the isoprocarb and carbaryl analysis methods in coffee beans was carried out following the procedures and performance criteria of the European Commission, SANTE 11312/2021.18 Method performance was evaluated through validation parameters: linearity, precision, recovery, and limit of quantification (LOQ). The matrix effect was investigated using standards prepared in solvents and sample extracts and then calculated using the following formula. |  | (1) |
Coffee processing
The contaminated green coffee samples (4 samples of robusta) with isoprocarb and carbaryl were roasted using a Probat Probatino coffee roaster at 3 different roasting levels (light, medium, and dark) based on the Agtron System/Specialty Coffee Association of America (SCAA) classification. The light-roast bean was exposed to 200 °C for 8 min or right at the first crack; the medium-roast bean was exposed to 220 °C for 12 min or to the end of the first crack or the beginning of the second crack; the dark-roast bean was exposed to 230 °C for 14 min or at the end of the second crack. The residue at each roasting level and its infusion were analyzed in triplicate. The processing factor (PF) that indicates the effect of coffee processing on the level of pesticide residue was evaluated using the formula: |  | (2) |
The transfer rate of pesticide residue from roasted coffee to its infusion was calculated using the following equation.24
| R = Cin × Vin/C × M × 100 | (3) |
where
R is the transfer rate (%),
Cin is the concentration of pesticide in infusion coffee (μg L
−1),
Vin is the volume of coffee infusion (mL),
C is the concentration of pesticide in roasted coffee (μg kg
−1) and
M is the weight of roasted coffee (g).
Deterministic risk assessment
Chronic exposure to isoprocarb and carbaryl residues from coffee was calculated by comparing consumption data by age group from Indonesia25 and the United States,26 the country with the highest consumption. Risk as the chronic hazard quotient (HQ) was obtained from the quotient between exposure or estimated daily intake (EDI, mg per kg BW) and acceptable daily intake (ADI, mg per kg BW). The ADI of isoprocarb (0.002 mg per kg BW) and carbaryl (0.008 mg per kg BW) was obtained from the report of the Joint FAO/WHO Meeting on Pesticide Residues (JMPR) and other references.27,28 The calculation formula are eqn (4) and (5). |  | (4) |
|  | (5) |
The cumulative risk due to isoprocarb and carbaryl in coffee was assessed as a hazard index (HI) using eqn (6).
| HI = HQisoprocarb + HQcarbaryl | (6) |
Pesticide exposure poses no risk to the consumer if the HI and/or HQ value is less than or equal to 1. In contrast, if the HI and/or HQ value is greater than 1, the consumer's health risk is unacceptable.
Results and discussion
Method validation
The evaluation of the validation results (Table 1) can be declared to meet the requirements in the European guideline;18 therefore, the method used in this study is valid for carbaryl and isoprocarb determination. Good repeatability and reproducibility precision were obtained from fortified coffee samples at concentration levels of 0.001 mg kg−1, 0.01 mg kg−1, and 0.05 mg kg−1, i.e. in the range of 2.0–10.6% and 5.2–15.2%, respectively. All fortified levels showed excellent recoveries (ranging from 85.6 to 102.6%). In addition, after being purified with GCB, the fortified sample resulted in isoprocarb and carbaryl recoveries of 102.6 ± 1.9% and 83.2 ± 1.9%, respectively. These results confirm that planar pesticides can be strongly absorbed by GCB as previously reported by Li et al.29 The recovery reduction of carbaryl was not particularly considerable (<20%) and remains within the performance criteria of the method validation guidelines in SANTE 11312/2021.18 Since the extract was concentrated six times through evaporation, high sensitivity was attained (a LOQ of 0.001 mg kg−1 in green and roasted coffee). This LOQ value indicated that the test method's detection sensitivity was up to 10 times below the standard (Japanese regulation). In brewed coffee, achieving a lower LOQ (0.0001 mg L−1) was made possible by pre-concentrating the sample 10 times before injection. This high factor was feasible due to the very low matrix effects, a consequence of the dilution that occurs during brewing.30 Further information on the method validation result of roasted coffee and brewed coffee is shown in Table S4.† The QuEChERS method had a low matrix effect for isoprocarb (ME = −15%) and a moderate matrix effect for carbaryl (ME = 28%). Therefore, a matrix matched standard was applied to reduce the error and increase the accuracy.
Table 1 Validation result and uncertainty estimation of isoprocarb and carbaryl in green coffee beans
Analytical parameter |
Value |
Isoprocarb |
Carbaryl |
Recovery (n = 6), (%)
|
Level 0.001 (mg kg−1) |
92.4 |
88.1 |
Level 0.01 (mg kg−1) |
87.3 |
102.6 |
Level 0.05 (mg kg−1) |
85.6 |
100.8 |
![[thin space (1/6-em)]](https://www.rsc.org/images/entities/char_2009.gif) |
Repeatability (n = 6), RSD
r
(%)
|
Level 0.001 (mg kg−1) |
10.6 |
5.5 |
Level 0.01 (mg kg−1) |
3.1 |
2.0 |
Level 0.05 (mg kg−1) |
5.2 |
5.3 |
![[thin space (1/6-em)]](https://www.rsc.org/images/entities/char_2009.gif) |
Within-laboratory reproducibility, RSD
R
(%)
|
Level 0.001 (mg kg−1) |
11.3 |
15.2 |
Level 0.01 (mg kg−1) |
6.9 |
5.3 |
Level 0.05 (mg kg−1) |
9.4 |
5.2 |
Linear range (mg kg
−1
)
|
0.001–0.100 |
0.001–0.100 |
Correlation coefficients (
r
)
|
1.0000 |
0.9994 |
Limit of quantification (LOQ), (mg kg
−1
)
|
0.001 |
0.001 |
Expanded measurement uncertainty,
U
′ (%)
|
33 |
35 |
From the stability observation, isoprocarb and carbaryl in coffee bean extracts can be stable for 7 days when stored at 4 °C and −20 °C, respectively (Table 2). Meanwhile, the effect of solvent evaporation may cause a trend of increasing residue for the standard stored at room temperature, 25 °C, causing a higher uncertainty result. Therefore, it can be inferred that daily preparation of the addition standard is unnecessary and it can be stored for 7 days at 4 °C and −20 °C before being measured for pesticide determination. This finding was triggered by the fact that other pesticide standards in plant extracts of ethyl acetate were stable for more than 40 days when stored at low temperatures.20
Table 2 Isoprocarb and carbaryl stability in coffee bean extract stored at 25 °C, 4 °C, and −20 °C in clear and amber vials
Storage period (day) |
Recovery (%) |
Isoprocarb |
Carbaryl |
Storage conditions |
Storage conditions |
25 °C |
4 °C |
−20 °C |
25 °C |
4 °C |
−20 °C |
Clear vial |
Amber vial |
Clear vial |
Amber vial |
Clear vial |
Amber vial |
Clear vial |
Amber vial |
Clear vial |
Amber vial |
Clear vial |
Amber vial |
Spike level: 0.01 mg kg−
1
|
1 |
93 ± 1 |
98 ± 1 |
93 ± 5 |
105 ± 1 |
92 ± 2 |
102 ± 4 |
92 ± 12 |
86 ± 2 |
90 ± 6 |
88 ± 9 |
82 ± 6 |
94 ± 3 |
2 |
116 ± 7 |
122 ± 5 |
114 ± 2 |
116 ± 6 |
116 ± 4 |
118 ± 2 |
97 ± 15 |
112 ± 8 |
108 ± 2 |
92 ± 1 |
97 ± 2 |
94 ± 5 |
3 |
172 ± 4 |
142 ± 2 |
117 ± 1 |
117 ± 1 |
117 ± 7 |
114 ± 4 |
145 ± 29 |
184 ± 6 |
127 ± 4 |
118 ± 1 |
105 ± 2 |
95 ± 4 |
4 |
154 ± 3 |
146 ± 1 |
101 ± 3 |
118 ± 1 |
108 ± 4 |
113 ± 3 |
160 ± 28 |
130 ± 1 |
99 ± 3 |
97 ± 8 |
87 ± 7 |
88 ± 2 |
7 |
193 ± 4 |
187 ± 1 |
88 ± 3 |
96 ± 0 |
88 ± 1 |
96 ± 16 |
139 ± 18 |
161 ± 1 |
77 ± 5 |
74 ± 0 |
78 ± 4 |
81 ± 6 |
![[thin space (1/6-em)]](https://www.rsc.org/images/entities/char_2009.gif) |
Spike level: 0.05 mg kg−
1
|
1 |
110 ± 4 |
114 ± 2 |
108 ± 3 |
107 ± 2 |
103 ± 2 |
107 ± 4 |
99 ± 2 |
100 ± 3 |
95 ± 1 |
94 ± 3 |
93 ± 3 |
92 ± 5 |
2 |
119 ± 2 |
113 ± 2 |
113 ± 4 |
114 ± 7 |
113 ± 6 |
109 ± 2 |
115 ± 4 |
115 ± 1 |
108 ± 6 |
107 ± 4 |
120 ± 7 |
106 ± 2 |
3 |
137 ± 1 |
108 ± 2 |
119 ± 4 |
120 ± 4 |
106 ± 0 |
110 ± 2 |
106 ± 1 |
86 ± 2 |
95 ± 4 |
96 ± 2 |
104 ± 1 |
89 ± 1 |
4 |
160 ± 1 |
151 ± 4 |
114 ± 1 |
127 ± 4 |
116 ± 1 |
116 ± 0 |
136 ± 2 |
129 ± 4 |
96 ± 1 |
110 ± 1 |
98 ± 1 |
93 ± 0 |
7 |
151 ± 6 |
162 ± 2 |
91 ± 1 |
115 ± 5 |
84 ± 2 |
109 ± 5 |
134 ± 3 |
152 ± 6 |
77 ± 4 |
102 ± 5 |
73 ± 0 |
101 ± 2 |
![[thin space (1/6-em)]](https://www.rsc.org/images/entities/char_2009.gif) |
Spike level: 0.100 mg kg−
1
|
1 |
101 ± 4 |
105 ± 5 |
110 ± 2 |
115 ± 1 |
105 ± 2 |
100 ± 1 |
81 ± 2 |
85 ± 7 |
84 ± 2 |
89 ± 0 |
84 ± 3 |
113 ± 5 |
2 |
117 ± 1 |
111 ± 4 |
104 ± 4 |
110 ± 3 |
110 ± 3 |
108 ± 4 |
104 ± 6 |
101 ± 3 |
92 ± 4 |
100 ± 3 |
99 ± 4 |
100 ± 2 |
3 |
141 ± 0 |
134 ± 2 |
110 ± 1 |
122 ± 6 |
114 ± 1 |
112 ± 4 |
101 ± 1 |
94 ± 2 |
100 ± 2 |
97 ± 3 |
88 ± 2 |
86 ± 3 |
4 |
123 ± 7 |
130 ± 8 |
125 ± 6 |
124 ± 4 |
109 ± 4 |
111 ± 9 |
98 ± 9 |
131 ± 4 |
99 ± 2 |
100 ± 5 |
86 ± 3 |
88 ± 7 |
7 |
183 ± 1 |
144 ± 6 |
100 ± 3 |
91 ± 4 |
92 ± 1 |
84 ± 1 |
142 ± 6 |
184 ± 5 |
82 ± 4 |
72 ± 4 |
121 ± 4 |
81 ± 7 |
On-going method validation
The on-going method validation was necessary to be observed for long-term purposes through the recovery of fortified samples and the deviation of sample replicas that were analyzed by internal quality control (IQC) as shown Fig. 1. A blank coffee sample previously tested to be free of pesticides was fortified at concentration levels of 0.01 mg kg−1 and 0.05 mg kg−1 alternately during routine daily analysis. Outstanding performance was indicated by the high percentage (>90%) of internal quality assurance of recovery observations, which are in the range of 60–140% and % RSD < 20% for 14 months. As a result, all recoveries still meet the acceptance criteria for routine recovery tests (60–140%).18 Testing results with % RSD > 20 have been investigated and re-analyzed. Thus, this observation of the acceptability of IQC is very important to ensure the accuracy of the method applied in this study for long routine analysis periods.
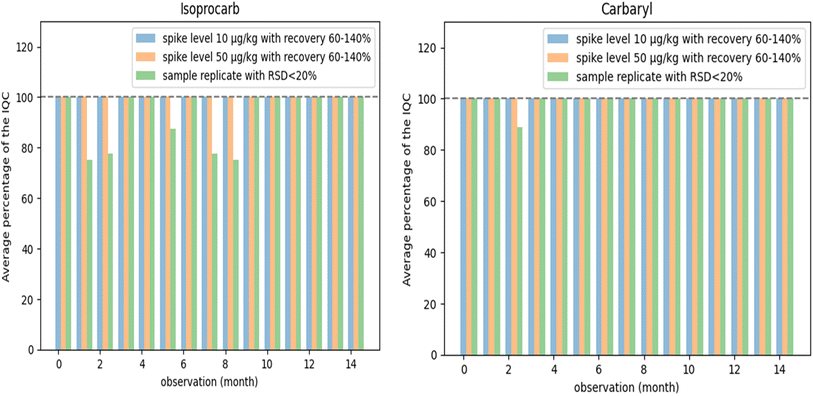 |
| Fig. 1 The percentage average of internal quality control (IQC) in routine analysis (7 to 15 analyses every month) with recovery within the range 60–140% and % RSD < 20. | |
The chromatogram peaks of a positive sample of isoprocarb (248 μg kg−1 with 5× dilution) and carbaryl (57 μg kg−1) in green beans are presented in Fig. 2. For early eluting chemicals, on the other hand, interference from the simultaneous extraction of the coffee matrix can have a considerable impact on the analyte's signal response. Contrarily, interference caused by the co-extractive compounds of the coffee matrix (e.g. caffeine, theobromine, etc.) has not considerably influenced the signal response with our target compounds as previously reported.2 Isoprocarb was still detected down to 1.1 μg L−1 in brewed coffee. Meanwhile, carbaryl was undetectable after the roasting process.
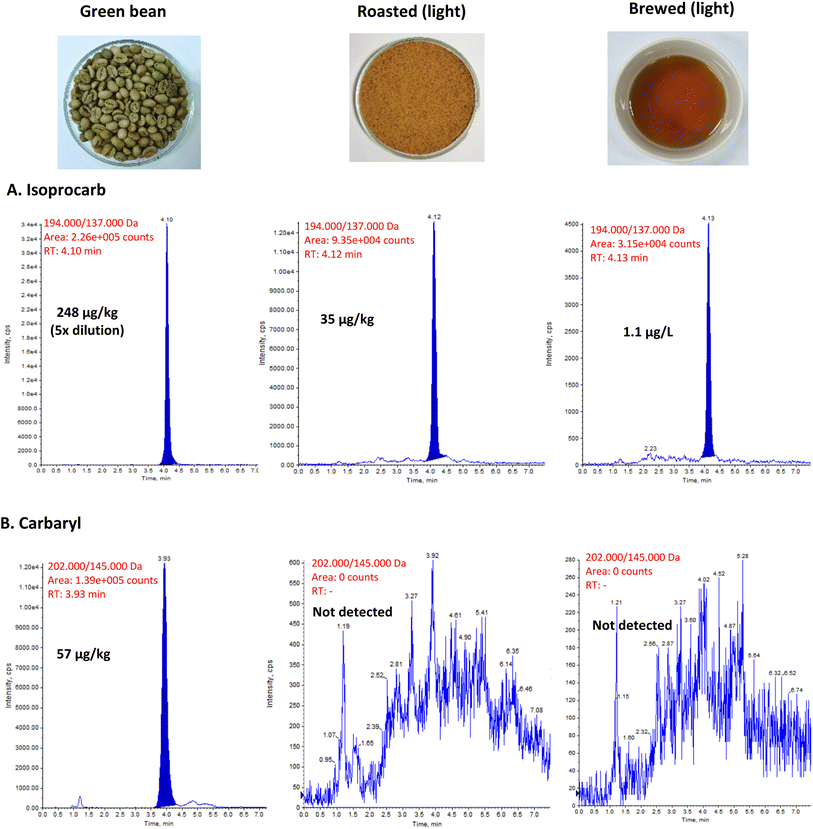 |
| Fig. 2 LC-MS/MS chromatogram of (a) isoprocarb and (b) carbaryl in contaminated robusta coffee during coffee processing. | |
Investigation of isoprocarb and carbaryl in Indonesian coffee
Occurrence of pesticides in surveyed coffee samples.
Carbamate insecticides of isoprocarb and carbaryl were detected in around 34% of all samples (80 samples) and all detected samples were originally from Lampung province (robusta coffee). High concentrations of carbaryl and isoprocarb were detectable in one and three samples, respectively. Following these findings, continuous monitoring is required to control residue levels and evaluate compliance with the safety standards of importing countries. Insecticides isoprocarb and carbaryl were used extravagantly to repel ants before harvest, generating residual problems on green coffee beans. In addition, a few other pesticides (320 pesticides, Table S5†) such as chlorpyrifos were detected but below the LOQ. Therefore, addressing the presence of carbaryl and isoprocarb was key to eliminating rejection notifications during exportation.
Isoprocarb and carbaryl in routine sample analysis of coffee exports.
Robusta (57.76%) coffee has more contaminated samples than arabica coffee (6.17%), as indicated by the distribution of pesticides shown in Fig. 3. This method was appraised as applicable for the evaluation of isoprocarb and carbaryl in positive samples of robusta coffee in the range of 0.001 mg kg−1 to 0.239 mg kg−1 and 0.001 mg kg−1 to 0.249 mg kg−1, respectively, and positive samples of arabica coffee, each in the range of 0.001 mg kg−1 to 0.022 mg kg−1 and 0.001 mg kg−1 to 0.020 mg kg−1. Purified extract from a blank coffee sample was used to dilute the sample extract, which had a higher pesticide content than the addition calibration standard's highest level (0.100 mg kg−1), during sample analysis. From these results, the method in this study can be applied to determine isoprocarb and carbaryl for long-term routine analysis from November 2021 to December 2022 (1385 samples). Surprisingly, the detected samples in this study were reduced by more than 90% in the last two months (November to December 2022). These results can be considered as a benefit derived from the Ministry of Agriculture's official dissemination of good agricultural practices (GAP) to farmers.13 Mechanical trapping was found to be an effective technique for controlling black ants (Dolichoderus thoracicus) on Indonesian coffee plants.31
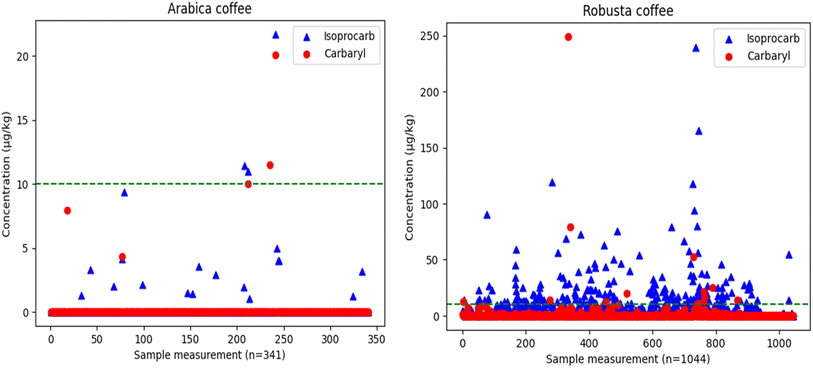 |
| Fig. 3 Isoprocarb and carbaryl distribution in arabica coffee (n = 341) and robusta coffee (n = 1044) detected by the method. | |
Isoprocarb and carbaryl have been detected worldwide, not only in coffee and agricultural products32–37 but also in other products, including animal products,38,39 environmental materials,40–47 and biological samples,48 as summarized in Table 3. Beyond being beneficial in controlling insecticide-target organisms, the use of carbamate pesticides has a negative impact on non-target organisms and the environment. Therefore, the evaluation of its occurrence and its effect on humans and the environment is essential as a consequence of applying this carbamate pesticide.
Table 3 Comparison of isoprocarb and carbaryl concentrations in coffee and other products worldwide
Country |
Product |
Pesticides |
No. (%) of positive samples |
Concentration |
Ref. |
Indonesia |
Green coffee |
Isoprocarb |
678 (49) |
0.001–0.239 mg kg−1 |
This study |
Carbaryl |
200 (14) |
0.001–0.249 mg kg−1 |
United Arab Emirates |
Milk |
Carbaryl |
9 (53) |
0.606–7.771 μg kg−1 |
38
|
China |
Greenhouse cucumbers |
Isoprocarb |
16 (9) |
0.03–1.13 mg kg−1 |
32
|
Ethiopia |
Fish |
Carbaryl |
— |
0.2–56.5 μg kg−1 |
39
|
Brazil |
Sweet pepper |
Carbaryl |
— |
5 mg kg−1 |
33
|
Malaysia |
Cocoa bean |
Isoprocarb |
2 (1) |
0.010–0.017 mg kg−1 |
34
|
South Africa |
Air |
Carbaryl |
54 (100) |
Median = 0.02 ng m−3 |
40
|
Max = 1.3 ng m−3 |
USA |
Bat hair |
Carbaryl |
— |
41.4–216.7 pg mg−1 |
48
|
China |
Surface water from a lake |
Isoprocarb |
208 (100) |
Average = 17 ng L−1 |
41
|
Max = 406 ng L−1 |
USA |
Dust |
Carbaryl |
163 (64.7) |
Median = 22.4 ng g−1 |
42
|
Pakistan |
Date palm fruit |
Carbaryl |
1 (5) |
2.8 μg kg−1 |
35
|
Poland |
Soil |
Carbaryl |
45 (20) |
<0.01–28.07 μg kg−1 |
43
|
Africa |
Air |
Carbaryl |
68 (41) |
— |
44
|
Malawi |
Surface water |
Carbaryl |
— |
0.083–0.254 mg L−1 |
45
|
Groundwater |
Carbaryl |
— |
0.07–0.492 mg L−1 |
Soil |
Carbaryl |
— |
1.154–1.305 mg L−1 |
China |
Surface watersheds |
Isoprocarb |
25 (100) |
0.47–39.06 ng L−1 |
46
|
Spain |
Citrus fruit |
Carbaryl |
94 (1) |
0.03 mg kg−1 |
36
|
Bangladesh |
Water from paddy and vegetable fields |
Carbaryl |
2 (7) |
14.1–18.1 μg L−1 |
47
|
Saudi Arabia |
Tomato |
Carbaryl |
1 (0.6) |
0.390 mg kg−1 |
37
|
Squash |
3 (1.9) |
0.209–1.148 mg kg−1 |
Cucumber |
3 (1.9) |
0.384–1.457 mg kg−1 |
Egg-plant |
2 (1.3) |
1.686–1.917 mg kg−1 |
Green pepper |
2 (1.3) |
0.653–2.228 mg kg−1 |
Lettuce |
3 (1.9) |
0.538–1.641 mg kg−1 |
Carrot |
1 (0.6) |
0.891 mg kg−1 |
Cabbage |
3 (1.9) |
0.069–1.765 mg kg−1 |
Effect of coffee processing
The roasting of coffee can reduce or even remove pesticide residues. Previous studies investigated some organochlorine, pyrethroid, and organophosphate pesticides using spiked/fortified samples rather than those using incurred pesticide or naturally contaminated samples. As a result, residues were still detected in roasted coffee for stable organochlorine pesticides (reduction > 80%) and deltamethrin (reduction > 70%). Meanwhile for other organophosphates and pyrethroids, the reduction was 100%.3,49 In comparison, the field trial's contaminated sample of the organonitrogen compound dinotefuran was reduced by 62.2% to 100% as a result of the roasting process, and residues could still be detected in the brew.50 As revealed in Table 4, the coffee roasting process can reduce the residues by up to 100% for isoprocarb at medium and dark roasting degrees and for carbaryl at all roasting degrees in contaminated robusta coffee. Isoprocarb reduction ranges from 83.60 to 93.55% at a light degree.
Table 4 Effect of coffee processing on pesticide residues in robusta coffee reported with standard deviation from replicated samples (n = 3)a,b
Sample code |
Pesticide |
Concentration (μg kg−1) |
Processing factor (PF) |
Transfer rate (%) |
Green bean |
Roasted coffee |
Brewed coffee |
ND = not detected (below the LOQ).
N/A = not applicable to be analyzed and/or calculated because the residue was not detected after the roasting process.
|
Robusta A |
Isoprocarb |
35.20 ± 4.33 |
Light = 2.27 ± 0.16 |
ND |
0.064 |
N/A |
Medium = ND |
N/A |
N/A |
N/A |
Dark = ND |
N/A |
N/A |
N/A |
Carbaryl |
57.20 ± 10.55 |
Light = ND |
N/A |
N/A |
N/A |
Medium = ND |
N/A |
N/A |
N/A |
Dark = ND |
N/A |
N/A |
N/A |
Robusta B |
Isoprocarb |
248.33 ± 21.57 |
Light = 35.43 ± 5.53 |
1.117 ± 0.061 |
0.143 |
L = 56.75 |
Medium = ND |
N/A |
N/A |
N/A |
Dark = ND |
N/A |
N/A |
N/A |
Carbaryl |
9.05 ± 0.74 |
Light = ND |
N/A |
N/A |
N/A |
Medium = ND |
N/A |
N/A |
N/A |
Dark = ND |
N/A |
N/A |
N/A |
Robusta C |
Isoprocarb |
21.90 ± 1.97 |
Light = 3.57 ± 0.23 |
0.138 ± 0.007 |
L = 0.163 |
L = 69.58 |
Medium = ND |
N/A |
N/A |
N/A |
Dark = ND |
N/A |
N/A |
N/A |
Carbaryl |
12.57 ± 0.97 |
Light = ND |
N/A |
N/A |
N/A |
Medium = ND |
N/A |
N/A |
N/A |
Dark = ND |
N/A |
N/A |
N/A |
Robusta D |
Isoprocarb |
10.55 ± 0.78 |
Light = 1.73 ± 0.24 |
N/A |
L = 0.164 |
N/A |
Medium = ND |
N/A |
N/A |
N/A |
Dark = MD |
N/A |
N/A |
N/A |
Carbaryl |
13.07 ± 1.51 |
Light = ND |
N/A |
N/A |
N/A |
Medium = ND |
N/A |
N/A |
N/A |
Dark = ND |
N/A |
N/A |
N/A |
Previous studies on coffee roasting mentioned that physicochemical properties, such as solubility and vapor pressure, might be responsible factors for the pesticide loss caused by evaporation and thermal degradation.3,49,50 Pesticides characterized as volatile with low water solubility and high vapor pressure could considerably contribute to their residue reduction.51 Pesticides with low vapor pressure cannot be easily removed by heating. From their physicochemical properties, isoprocarb (solubility = 504.1 mg L−1 at 25 °C and vapor pressure = 1.01 × 10−4 mmHg at 25 °C) and carbaryl (solubility = 416.2 mg L−1 at 25 °C and vapor pressure = 2.09 × 10−5 mmHg at 25 °C) as an insecticide carbamate derived from a carbamic acid have moderate solubility and low volatility. However, both are more volatile than organochlorine, organophosphate, and pyrethroid pesticides, the residues of which can be lost during coffee bean roasting, as previously observed.3,49 Additionally, other physical properties, such as molecular weight, are related to the phenomenon of residue loss as a result of thermal processes.52 The small molecular size of isoprocarb (193.242 g mol−1) can cause a higher penetration rate or diffusion into the coffee bean matrix, which may indicate why the residue still remains in light-roasted coffee.
The transfer rate of isoprocarb from the light degree of roasted coffee to the brew is shown in Table 4, ranging from 0 to 69.58%. Isoprocarb can be transformed into their metabolites through thermal processing.17 It implied that exposure to isoprocarb and other possible metabolites is feasible because the residue can enter the human body through drinking coffee if it is roasted to brightness. Conversely, carbaryl will remain as a precursor when it evaporates during thermal processing.14 Consequently, it can be concluded that the presence of the probable carcinogenic carbaryl in coffee beans does not correlate with health risks due to coffee consumption.
Risk assessment
Using mean residue and consumption data, a simple calculation of chronic dietary exposure was completed using a deterministic approach. Based on age group comparisons between populations in Indonesia and the United States, the risk was calculated and differentiated for robusta and arabica coffee, as shown in Table 5. These results were calculated using the worst-case upper-bound (UB) scenario, where test results below the LOQ were replaced with the LOQ value. The estimated body weights of the Indonesian population used in the calculation are 11.67 kg (0–59 months), 27.5 kg (5–12 years), 46.33 kg (13–18 years), 57.87 kg (19–55 years), 52.3 kg (>55 years), and 50.78 (all ages).53 Meanwhile for the US population, an average body weight of 60 kg was used for all groups of adult age.54
Table 5 Comparison of chronic risk assessment between Indonesia and the country with the highest consumption, the United States
Commodity |
Country/population |
Age group |
EDI (mg per kg BW per day) |
Risk index |
Hazard quotient, HQ |
Hazard index, HI |
Isoprocarb |
Carbaryl |
Isoprocarb |
Carbaryl |
Arabica coffee |
Indonesia |
0–59 months |
1.05 × 10−8 |
9.80 × 10−9 |
5.24 × 10−6 |
1.22 × 10−6 |
6.46 × 10−6 |
5–12 years |
1.78 × 10−8 |
1.66 × 10−8 |
8.89 × 10−6 |
2.08 × 10−6 |
1.10 × 10−5 |
13–18 years |
5.01 × 10−8 |
4.69 × 10−8 |
2.51 × 10−5 |
5.86 × 10−6 |
3.09 × 10−5 |
19–55 years |
1.65 × 10−7 |
1.54 × 10−7 |
8.24 × 10−5 |
1.93 × 10−5 |
1.02 × 10−4 |
>55 years |
1.68 × 10−7 |
1.57 × 10−7 |
8.42 × 10−5 |
1.97 × 10−5 |
1.04 × 10−4 |
All ages |
1.44 × 10−7 |
1.35 × 10−7 |
7.22 × 10−5 |
1.69 × 10−5 |
8.91 × 10−5 |
United States |
20–30 |
2.86 × 10−6 |
2.68 × 10−6 |
1.43 × 10−3 |
3.35 × 10−4 |
1.77 × 10−3 |
31–50 |
5.38 × 10−6 |
5.03 × 10−6 |
2.69 × 10−3 |
6.29 × 10−4 |
3.32 × 10−3 |
51–70 |
7.51 × 10−6 |
7.02 × 10−6 |
3.76 × 10−3 |
8.78 × 10−4 |
4.63 × 10−3 |
≥71 |
6.31 × 10−6 |
5.90 × 10−6 |
3.15 × 10−3 |
7.37 × 10−4 |
3.89 × 10−3 |
All ages |
5.66 × 10−6 |
5.29 × 10−6 |
2.83 × 10−3 |
6.62 × 10−4 |
3.49 × 10−3 |
Robusta coffee |
Indonesia |
0–59 months |
6.09 × 10−8 |
1.48 × 10−8 |
3.04 × 10−5 |
1.85 × 10−6 |
3.23 × 10−5 |
5–12 years |
1.03 × 10−7 |
2.52 × 10−8 |
5.17 × 10−5 |
3.14 × 10−6 |
5.48 × 10−5 |
13–18 years |
2.91 × 10−7 |
7.09 × 10−8 |
1.46 × 10−4 |
8.87 × 10−6 |
1.55 × 10−4 |
19–55 years |
9.58 × 10−7 |
2.33 × 10−7 |
4.79 × 10−4 |
2.91 × 10−5 |
5.08 × 10−4 |
>55 years |
9.78 × 10−7 |
2.38 × 10−7 |
4.89 × 10−4 |
2.98 × 10−5 |
5.19 × 10−4 |
All ages |
8.39 × 10−7 |
2.04 × 10−7 |
4.20 × 10−4 |
2.55 × 10−5 |
4.45 × 10−4 |
United States |
20–30 |
1.66 × 10−5 |
4.05 × 10−6 |
8.32 × 10−3 |
5.07 × 10−4 |
8.83 × 10−3 |
31–50 |
3.13 × 10−5 |
7.61 × 10−6 |
1.56 × 10−2 |
9.51 × 10−4 |
1.66 × 10−2 |
51–70 |
4.36 × 10−5 |
1.06 × 10−5 |
2.18 × 10−2 |
1.33 × 10−3 |
2.31 × 10−2 |
≥71 |
3.66 × 10−5 |
8.92 × 10−6 |
1.83 × 10−2 |
1.12 × 10−3 |
1.94 × 10−2 |
All ages |
3.29 × 10−5 |
8.01 × 10−6 |
1.64 × 10−2 |
1.00 × 10−3 |
1.74 × 10−2 |
The hazard quotient (HQ) for isoprocarb (5.24 × 10−6 to 2.18 × 10−2) was higher than that of carbaryl (1.22 × 10−6 to 1.33 × 10−3). Robusta coffee has a much higher HQ value than arabica coffee because it was more contaminated with isoprocarb and carbaryl. When comparing the populations of Indonesia and the US, the risk is lower for Indonesian consumers in comparison to US consumers. Children (0–59 months) in Indonesia have the lowest HQ values, which can be associated with comparatively low consumption. Remarkably, comparable findings were observed when an evaluation was executed to identify the highest risk by age group, revealing that the highest risk was found for Indonesian customers aged over 55 years and US consumers aged 51–70 years. Overall, the potential risk of pesticide exposure through Indonesian coffee consumption did not pose a serious concern to human health, as confirmed by HQ and/or HI values of less than 1 for all age groups for both robusta and arabica coffee. Similarly, a low risk was also found for exposure to other pesticides in coffee.55
Conclusion
The validated QuEChERS method for detecting isoprocarb and carbaryl insecticides in coffee beans has good performance, with a high percentage (>90%) of recovery in the range of 60–140% and % RSD of sample replication < 20%. Following the application of the method, isoprocarb and carbaryl in positive samples could be evaluated in robusta coffee in the ranges of 0.001 mg kg−1 to 0.239 mg kg−1 and 0.001 mg kg−1 to 0.249 mg kg−1, respectively, and in arabica coffee in the ranges of 0.001 mg kg−1 to 0.022 mg kg−1 and 0.001 mg kg−1 to 0.020 mg kg−1, respectively. Based on the additional data related to the effect of coffee processing and chronic dietary exposure conducted using a deterministic approach, it can be concluded that the consumption of coffee contaminated with isoprocarb and carbaryl does not pose a risk to human health.
Author contributions
Harmoko Harmoko: conceptualization, methodology, formal analysis, visualization, investigation, writing – original draft, and writing – review & editing. Hasim Munawar: writing – original draft and writing – review & editing. Syaiful Bahri: validation. Nuri Andarwulan: supervision. Daryono Hadi Tjahjono: supervision. Rahmana E. Kartasasmita: supervision and writing – review & editing. Amadeo R. Fernández-Alba: conceptualization, data presentation, supervision and writing – review & editing.
Conflicts of interest
There are no conflicts to declare.
Acknowledgements
This study was supported by the Directorate of Standardization and Quality Control, Ministry of Trade of the Republic of Indonesia.
References
- B. Bresin, M. Piol, D. Fabbro, M. A. Mancini, B. Casetta and C. Del Bianco, J. Chromatogr. A, 2015, 1376, 167–171 Search PubMed.
- C. M. Dias, F. A. Oliveira, F. D. Madureira, G. Silva, W. R. Souza and Z. L. Cardeal, Food Addit. Contam.: Part A, 2013, 30, 1308–1315 Search PubMed.
- S. Mekonen, A. Ambelu and P. Spanoghe, J. Agric. Food Chem., 2015, 63, 8568–8573 Search PubMed.
- J. Menzio, S. Tagliapietra, E. Calegari, B. Serito, A. Binello and G. Cravotto, J. Agric. Food Chem., 2021, 69, 4858–4864 Search PubMed.
- I. R. Pizzutti, A. de Kok, C. D. Cardoso, B. Reichert, M. de Kroon, W. Wind, L. W. Righi and R. Caiel da Silva, J. Chromatogr. A, 2012, 1251, 16–26 Search PubMed.
- B. Reichert, A. de Kok, I. R. Pizzutti, J. Scholten, C. D. Cardoso and M. Spanjer, Anal. Chim. Acta, 2018, 1004, 40–50 Search PubMed.
- X. Yang, J. Wang, D. C. Xu, J. W. Qiu, Y. Ma and J. Cui, Food Anal. Methods, 2011, 4, 186–195 Search PubMed.
- S. Walorczyk, D. Drozdzyński and R. Kierzek, Talanta, 2015, 132, 197–204 Search PubMed.
- A. Gamal, M. Soliman, M. S. Al-Anany and F. Eissa, Food Chem., 2024, 449, 139223 Search PubMed.
- L. A. B. De Oliveira, H. P. Pacheco and R. Scherer, Food Chem., 2016, 190, 60–63 Search PubMed.
-
Pharmaceutical Safety and Environmental Health Bureau, Ministry of Health, Labour and Welfare, Results of monitoring and guidance based on the imported foods monitoring and guidance plan for FY 2021, 2022, https://www.mhlw.go.jp/content/Results_FY2021.pdf Search PubMed.
-
USDA Foreign Agricultural Service, Coffee Annual Indonesia GAIN Report Number: ID1320, 2013, https://apps.fas.usda.gov/newgainapi/api/report/downloadreportbyfilename?filename=CoffeeAnnual_Jakarta_Indonesia_5-15-2013.pdf, accessed, 02 February, 2023 Search PubMed.
-
Ministry of Agriculture of Indonesia, Sistem Pertanian Organik Sebagai Jawaban Penanganan Isu Residu Isoprocarb Pada Biji Kopi Indonesia, 2022, https://ditjenbun.pertanian.go.id/sistem-pertanian-organik-sebagai-jawaban-penanganan-isu-residu-isoprocarb-pada-biji-kopi-indonesia/, accessed, 10 October, 2022 Search PubMed.
-
P. Iyer and S. Makris, in Hayes' Handbook of Pesticide Toxicology, Elsevier, 3rd edn, 2010, vol. 1, pp. 381–440 Search PubMed.
-
S. E. Koshlukova and N. R. Reed, in Encyclopedia of Toxicology, Elsevier, 3rd edn, 2014, pp. 668–672 Search PubMed.
- T. Yang, C. Ma, H. Chen, Y. Zhang, X. Dang and J. Huang, J. Sep. Sci., 2014, 37, 587–594 Search PubMed.
- X. Shao, G. Wang, Y. Sun, R. Zhang, K. Xiu and H. Liu, J. Chromatogr. Sci., 2006, 44, 141–147 Search PubMed.
-
European Commission, Method Validation Procedures for Pesticide Residues Analysis in Food and Feed, SANTE 11312/2021, 2021 Search PubMed.
- H. Harmoko, R. E. Kartasasmita, H. Munawar, A. Rakhmawati and B. Budiawan, J. Food Compos. Anal., 2022, 105, 104256 Search PubMed.
- V. Kocourek, J. Hajslova, K. Holadova and J. Poustka, J. Chromatogr. A, 1998, 800, 297–304 Search PubMed.
- G. Guo, N. Jiang, F. Liu and Y. Bian, R. Soc. Open Sci., 2018, 5, 180757 Search PubMed.
- S. Wang, M. Li, X. Li, X. Li, X. Li, S. Li, Q. Zhang and H. Li, J. Chromatogr. A, 2020, 1631, 461526 Search PubMed.
- R. E. Kartasasmita, F. Kurniawan, T. Amelia, C. M. Dewi, H. Harmoko and Y. Pratama, ACS Omega, 2020, 5, 20162–20169 Search PubMed.
- Z. Chen, S. Song, L. Mao, J. Wei, Y. Li, H. Tan and X. Li, J. Sci. Food Agric., 2019, 99, 1267–1274 Search PubMed.
-
Ministry of Health of Republic of Indonesia, Buku Survei Konsumsi Makanan Individu Dalam Studi Diet Total SKMI, 2014, https://perpustakaan.setneg.go.id/repository/SDT2015comp-min.pdf, accessed, 05 February, 2023 Search PubMed.
- C. D. Rehm, J. C. Ratliff, C. S. Riedt and A. Drewnowski, Nutrients, 2020, 12, 1–13 Search PubMed.
-
G. 2763-2016 USDA Foreign Agricultural Service, China Releases New Maximum Residue Limits for Pesticides in Food, 2016, https://www.fas.usda.gov/data/china-national-food-safety-standard-maximum-residue-limits-112-pesticides-foods-released Search PubMed.
- L. Song, Z. Zhong, Y. Han, Q. Zheng, Y. Qin, Q. Wu, X. He and C. Pan, Ecotoxicol. Environ. Saf., 2020, 188, 109842 Search PubMed.
- L. Li, W. Li, D. Qin, S. Jiang and F. Liu, J. AOAC Int., 2009, 92(2), 538–547 Search PubMed.
- C. Ferrer, A. Lozano, A. Agüera, A. J. Girón and A. R. Fernández-Alba, J. Chromatogr. A, 2011, 1218, 7634–7639 Search PubMed.
- A. A. Muliasari and M. F. Bawazir, Jurnal Ilmiah Inovasi, 2018, 18(3), 104–111 Search PubMed.
- C. Dong, Y. Huang and J. Hu, Microchem. J., 2023, 189, 108500 Search PubMed.
- J. C. de Andrade, D. Galvan, L. S. Kato and C. A. Conte-Junior, Chemosphere, 2023, 322, 138244 Search PubMed.
- B. H. Zainudin, S. Salleh, A. S. Yaakob and R. Mohamed, Food Chem., 2022, 368, 130778 Search PubMed.
- S. Qayyum, T. Taj, M. Tauseef, M. Ishtiaq, N. Rafique, K. Ahad, M. A. Mirza, M. A. Choudhary and F. Mehboob, Environ. Monit. Assess., 2021, 193, 613 Search PubMed.
- L. Quijano, V. Yusà, G. Font and O. Pardo, Food Chem. Toxicol., 2016, 89, 39–46 Search PubMed.
- K. A. Osman, A. M. Al-Humaid, S. M. Al-Rehiayani and K. N. Al-Redhaiman, Ecotoxicol. Environ. Saf., 2010, 73, 1433–1439 Search PubMed.
- R. Morsi, K. Ghoudi, M. M. Ayyash, X. Jiang and M. A. Meetani, J. Dairy Sci., 2024, 107, 1916–1927 Search PubMed.
- W. Zelalem, W. Anteneh, M. Mingist, M. Kibret, E. Adgo, F. Erarto, J. De Rop, A. De Cock, P. Spanoghe, P. L. M. Goethals, J. Nyssen, E. Verleyen and A. De Vocht, Environ. Monit. Assess., 2023, 95, 988 Search PubMed.
- A. F. Veludo, D. M. Figueiredo, C. Degrendele, L. Masinyana, L. Curchod, J. Kohoutek, P. Kukučka, J. Martiník, P. Přibylová, J. Klánová, M. A. Dalvie, M. Röösli and S. Fuhrimann, Chemosphere, 2022, 289, 133162 Search PubMed.
- T. Wang, M. Zhong, M. Lu, D. Xu, Y. Xue, J. Huang, L. Blaney and G. Yu, Sci. Total Environ., 2021, 782, 146826 Search PubMed.
- J. M. Madrigal, R. R. Jones, R. B. Gunier, T. P. Whitehead, P. Reynolds, C. Metayer and M. H. Ward, Environ. Res., 2021, 201, 111501 Search PubMed.
- A. Ukalska-Jaruga, B. Smreczak and G. Siebielec, Molecules, 2020, 25, 587 Search PubMed.
- S. Fuhrimann, J. Klánová, P. Přibylová, J. Kohoutek, M. A. Dalvie, M. Röösli and C. Degrendele, Chemosphere, 2020, 258, 127333 Search PubMed.
- C. Kanyika-Mbewe, B. Thole, R. Makwinja and C. C. Kaonga, Environ. Monit. Assess., 2020, 192, 595 Search PubMed.
- M. Xu, H. Huang, N. Li, F. Li, D. Wang and Q. Luo, Ecotoxicol. Environ. Saf., 2019, 175, 289–298 Search PubMed.
- M. A. Z. Chowdhury, S. Banik, B. Uddin, M. Moniruzzaman, N. Karim and S. H. Gan, Int. J. Environ. Res. Public Health, 2012, 9, 3318–3329 Search PubMed.
- S. E. Hooper, S. K. Amelon and C. H. Lin, Toxics, 2022, 10, 73 Search PubMed.
- K. Sakamoto, H. Nishizawa and N. Manabe, Food Hyg. Saf. Sci., 2012, 53(5), 233–236 Search PubMed.
- Z. Chen, S. Song, L. Mao, J. Wei, Y. Li, H. Tan and X. Li, J. Sci. Food Agric., 2019, 99, 1267–1274 Search PubMed.
- I. Hrynko, P. Kaczyński, M. Pietruszyńska and B. Łozowicka, Food Control, 2023, 143, 109267 Search PubMed.
- C. J. Wang and Z. Q. Liu, Pestic. Biochem. Physiol., 2007, 87, 1–8 Search PubMed.
- Y. Oktaviany, N. Andarwulan and P. E. Giriwono, Asian J. Chem., 2018, 30, 2241–2248 Search PubMed.
-
World Health Organization (WHO), Generic risk assessment model for insecticide-treated nets, 2nd edn, 2018, https://apps.who.int/iris/bitstream/10665/260305/1/9789241513586-eng.pdf Search PubMed.
- Y. J. H. Galani, M. Houbraken, A. Wumbei, J. F. Djeugap, D. Fotio, Y. Y. Gong and P. Spanoghe, Food Control, 2020, 118, 107416 Search PubMed.
|
This journal is © The Royal Society of Chemistry 2024 |