DOI:
10.1039/D3VA00043E
(Critical Review)
Environ. Sci.: Adv., 2023,
2, 1060-1081
Preservation, storage, and sample preparation methods for freshwater microplastics – a comprehensive review†
Received
21st February 2023
, Accepted 18th June 2023
First published on 6th July 2023
Abstract
Due to their long-lasting negative effects on the environment and detrimental impact on the health of living organisms, microplastics (MPs), found in both water and sediment matrices, have attracted researchers' attention recently. Although various research and reviews have been conducted about MP occurrence and abundance in aqueous environments, less attention has been paid to freshwater matrices through which MPs enter oceans and seas. Freshwater is a vital source of drinking water supply, irrigation systems, and animal feeding systems, and it directly or indirectly affects human health. Thereby, it becomes important to study the occurrence of MPs in freshwater reserves, such as lakes and set up standardized methods for sample collection, storage, and preparation for analysis. Several recent studies have established best-practice in MP characterization and analysis. However, only a few studies have depicted the importance of the pre-analysis phase, including sampling methods, storage, preservation, and preparation strategies. Therefore, this review delineates different sampling methods from freshwater compartments – the surface and column of water and sediments, followed by storage and preservation of obtained samples. Finally, preparation (pre-treatment and extraction) methods have been elaborated, which are necessary to purify MP samples before further investigations. This review aims to provide a clear understanding of the analytical steps and tools, leading to accurate investigations regarding MP's occurrence in future studies.
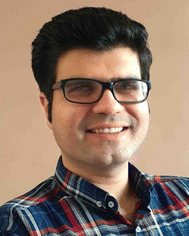 Behnam Nayebi | Behnam Nayebi, a PhD student at York University's Civil Engineering department, specializes in detecting and analyzing microplastics in Lake Ontario. His research during his PhD has focused on developing and optimizing methods for detecting microplastics in water and sediment samples. |
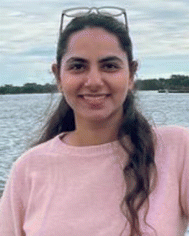 Pratishtha Khurana | Pratishtha is a graduate student at Lassonde School of Engineering, York University, Toronto, Canada, and a research fellow with FONCER-TEDGIEER, INRS, Quebec, Canada. Her research resolves around fate and treatment of emerging contaminants in environmental matrices. She is also a member of CentrEau, the Canadian Society for Chemistry (CSC) at the Chemical Institute of Canada (CIC), and Intersectoral Center for Endocrine Disruptor Analysis (CIAPE-ICEDA). |
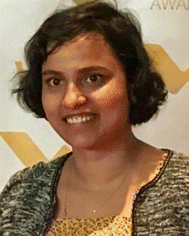 Rama Pulicharla | Rama Pulicharla holds a bachelor's degree in pharmacy and a master's degree in pharmaceutical sciences. Her PhD, obtained from Institut National de la Recherche Scientifique (Eau, Terre et Environnement, INRS-ETE, Université du Québec, Canada), concentrated on water science. During her doctoral studies, her main areas of focus involved the development of analytical methods and the investigation of the degradation of emerging contaminants in water sources. Rama Pulicharla has authored numerous peer-reviewed articles related to her research. Currently, she serves as a postdoctoral researcher at York University in Canada, where she continues her exploration of emerging contaminants. Her research primarily centers around environmental chemistry, with an emphasis on monitoring and implementing environmentally friendly treatment technologies for emerging contaminants. |
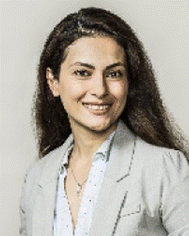 Shooka Karimpour | Dr Shooka Karimpour is an Assistant Professor of Civil Engineering at York University since 2019 where she leads the Environmental HydroDynamics (EHD) lab. She is also recipient of several international and national awards including Kefeer medal by Canadian Society of Civil Engineering. Turbulent mixing and entrainment play a significant role in freshwater and marine ecosystems, for instance, on sediment and nutrient transport and availability. Shooka's research is to investigate how these processes are induced and how they affect mass and contaminant transport. Currently, her team is working on entrainment of multi-phase flow, focusing on aerated flow and microplastic contaminants. |
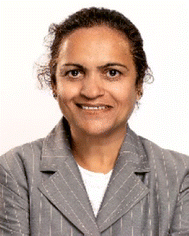 Satinder Kaur Brar | Dr Satinder K. Brar is Professor at Civil Engineering Department and the first James and Joanne Love Chair in Environmental Engineering at York University since January 2019. Her research interests lie in the development of finished products (formulations) of value-added bioproducts based on wastewater and wastewater sludge, such as enzymes, organic acids, platform chemicals, biocontrol agents, biopesticides, butanol and biohydrogen. She is also interested in the fate of endocrine disruptive compounds, pharmaceuticals, nanoparticles, and other toxic organic compounds during value-addition of wastewater and wastewater sludge in order to find suitable biological detoxification technologies. |
Environmental significance
Due to their long-lasting negative effects on the environment and detrimental impact on the health of living organisms, microplastics (MPs), found in both water and sediment matrices, have attracted researchers' attention recently. Although various research and reviews have been conducted about MP's occurrence and abundance in aqueous environments, less attention has been paid to freshwater matrices through which MPs enter oceans and seas. Freshwater is a vital source of drinking water supply, irrigation systems, and animal feeding systems, and it directly or indirectly affects human health. Thereby, it becomes important to study the occurrence of microplastics in freshwater reserves, such as lakes and set up standardized methods for sample collection, storage, and preparation for analysis. To perform research on microplastic detection and analysis, it is crucial to have high-quality sample preservation, storage, and pre-treatment. To the best of the authors' knowledge, this manuscript is the first review that shows the significance of storage and preservation processes has been overlooked. The review aims at summarizing different pre-analysis and pre-detection methods for microplastics in freshwater matrices, followed by highlighting the significance of storage and preservation, besides sampling and preparation methods.
|
1. Introduction
The production of plastics has been continuously increasing and is estimated to be doubled in the upcoming 20 years1,2 leading to a continual flow of plastic trash into the environment. Some of these plastics, which are smaller than 5 mm and larger than 1 μm, are called microplastics (MPs). MPs can enter the environment through different external sources, including wastewater treatment effluent,3 agricultural fields,4 urbanization,5 and even fishing nets.6 Based on their manufacturing and release into the environment, MPs are classified into two major categories: primary and secondary. Primary MPs have been intentionally produced in very fine sizes to be utilized in different applications, including, and not limited to, sandblasting7 and cosmetic products.8 Recent research shows that about 35% of primary MPs in water matrices stem from cloth washing.9 On the other hand, secondary MPs are generated through the fragmentation of plastic debris in the environment because of natural phenomena including weathering, solar UV radiation, tidal waves, etc.10
Different anthropogenic activities could also affect MP abundance in the environment. In a study, a comparison between particle presence in fish guts in urbanized and less-urbanized locations revealed a significant difference, showing the importance of urbanization in MP pollution.11 Sediment records also revealed the high impact of urbanization on MP presence in the sediment. It has been reported that some indicators like an abundance of PET and smaller particle size at the surface level show the significance of urbanization. Agricultural activities, on the other hand, proved to be an important factor in less-urbanized areas.12
Wastewater treatment plants' outflow is another factor causing a meaningful difference in MP abundance. It is shown that despite the relatively high rate of MP removal in sewage and wastewater treatment (up to 99%), a sheer number of MPs enter the environment.13 It should be noted that the entrapped particle in the wastewater treatment plant sludge is mainly used as fertilizer in agricultural lands, ending up in the environment through draining and stormwaters.14 Wear and tear of tires has also been reported as an anthropogenic source. One study revealed that the average flow of tire particles in the environment is 0.81 kg per year.15
Additionally, fishing nets and cages are another anthropogenic sources of MP presence in areas that rely on fish as their food resource, including the Chi River, Thailand.6 In the study of MP abundance in three Gogian dams, the abundance of fibers was assumed to be related to fishing ropes and nets.16
Domestic garbage landfills were revealed to have the most effect on MP abundance in lake environments.17 A study revealed that there are MPs in the landfill leachate and the treatment of leachate can decrease the MP presence by up to 50%.18
These findings reveal the significance of anthropogenic activities in the MP abundance in freshwater compartments, which can be harmful to different biotas. Different anthropogenic parameters affecting the abundance of MPs in the freshwater environment are shown in Fig. 1.
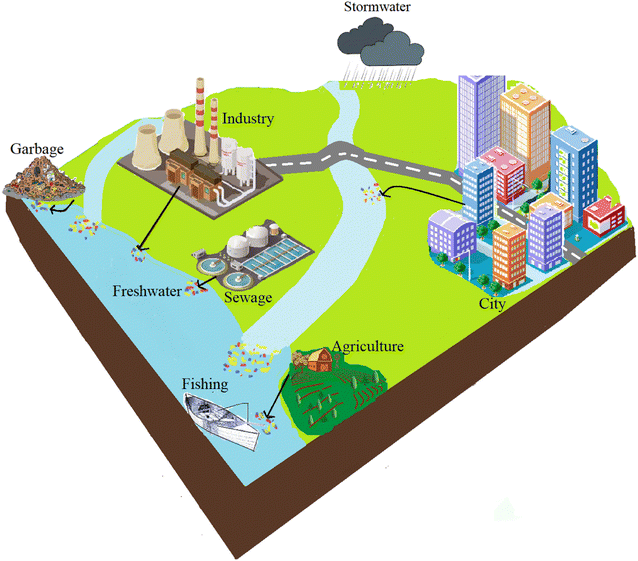 |
| Fig. 1 Anthropogenic parameters affecting microplastics (MP) abundance in freshwater environment. | |
Most of the MPs detected in freshwater compartments are made of polyethylene (PE), polypropylene (PP), polystyrene (PS), and polyethylene terephthalate (PET). These materials are highly recalcitrant and tend to accumulate in different environments,19 resulting in prolonged exposure and thereby harming living organisms, including humans.20 Further, their ability to adsorb on different types of toxic materials, for instance, heavy metals, perfluoro carboxylic acids, and other emerging contaminants21–23 allow them to act as vectors for contaminants, leading to severe damages to organisms. Other than the parent polymers, plastic additives also affect hormonal modulation and apoptosis, causing damage to organisms' cells and carcinogenesis.24,25
Although MPs' presence has been reported in many marine environments, only a few studies26 have investigated the presence of these emerging pollutants in freshwater compartments such as lakes and rivers. Freshwater compartments are vital matrices since they host a wide range of plants and animals, such as fish, one of the primary human food resources. Besides, these matrices are used as a source of drinking water and for recreational purposes.27 Moreover, it has been mentioned that MPs are roughly 80% more abundant in terrestrial compartments, including lakes and rivers than in marine environments.26 Also, it is reported that approximately 2 million tons of plastics enter the oceans through tributaries and rivers annually.13,28 Accordingly, without analyzing MP presence in freshwater environments, understanding their abundance in marine environments seems not sufficient.
Several review papers have investigated microplastic sampling, preparation, and analysis methods for obtaining microplastic samples from both marine and freshwater environments. However, there is a significant knowledge gap in finding protocols for the preservation of obtained samples till further investigations. MPs are materials made up of polymers and have been proven to be affected by different temperatures and chemicals. It should be noted that marine and freshwater environments have some similarities and differences, which should be considered in MP research, some of which have been identified in Fig. 2. Methods for sample storage, preservation, digestion/extraction, and characterization are similar for both compartments. However, the ambient properties and their vicinities to the pollution sources are different. Marine and freshwater are different in terms of density and salinity. Tursi et al., have shown that these two factors can significantly affect the MPs distribution. Many plastic polymers have densities marginally smaller or larger than freshwater. Due to the increase in marine water density, compared to freshwater, it has been discussed that in marine environments floating MPs may outnumber those in freshwater environments.29 However, it should be considered that buoyancy is affected by other factors apart from the density, for instance, flow currents, particle size, and biofilm formation.30 Another example is areas with higher expected fibers, as explained below: only sampling methods should be adopted that aren't selective and mesh-based methods should be avoided. In addition, it was shown in another study that an increase in salinity leads to a decrease in the settling velocity of particles.31 Settling velocity is an important factor since it can affect the abundance of MPs in water and sediment of both freshwater and marine environments. From a hydrodynamic perspective, rivers are highly dynamic systems, with smaller depths and higher speeds, compared to oceans which cause the resuspension and settling behaviors of particles as well as biofilm formation.32,33 Environmental factors like UV, wind, and rain can be different in freshwater and oceans. UV exposure combined with water movement speed up the fragmentation process, and particles are more exposed to the sun and tidal waves in oceans compared to lakes. Therefore, the fragmentation of particles may increase the number of fragments in the marine environment compared to the higher abundance of fibers in rivers and lakes.34 Another important factor affecting the shape distribution is the vicinity to the MP sources like wastewater treatments. In general, freshwater environments are closer to cities and wastewater treatments, which affects the abundance of microfibers in these environments in comparison with marine compartments.35 In a comparative study in China, it was observed that microfibers are the most dominant in rivers and estuaries. However, fragments were predominant in the marine environment which shows the impact of the source vicinity as well as environmental parameters.36 Biofilm formation is also different in freshwater compared to marine environments. It has been proven that PP fragments have a better biofilm condition for freshwater algae, impacting the distribution of specific MPs in freshwater and marine environments.37 Biofilm formation is also affected by the shape of particles. Microfibers show a faster sinking velocity when they expose to biofilm formation. This is important since the distribution of MPs is different in marine and freshwater environments, thus the abundance in water and sediment of freshwater is affected by their settling velocities.30 Biotas can mistake MPs for their food resources, being contaminated by these toxic materials. Issac et al., reported that species inhabiting freshwater environments may experience increased levels of exposure, especially in close proximity to industrial and densely populated regions, where concentrations of hydrophobic toxins and microplastics are potentially elevated.38
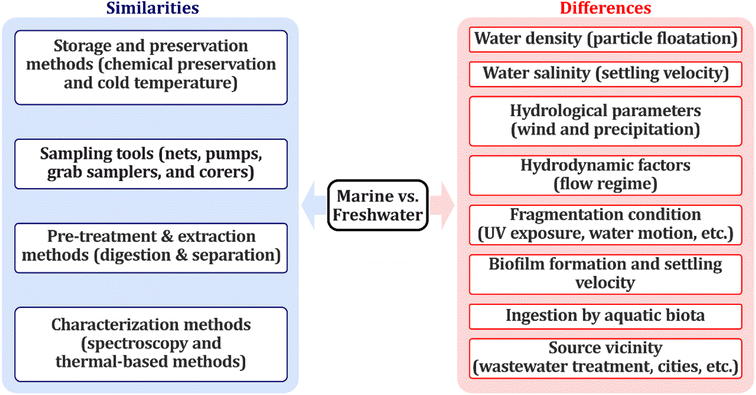 |
| Fig. 2 Similarities and differences between MP research in marine and freshwater environments. | |
In order for microplastic research to be precise, it is crucial to consider the potential effect of various parameters, including temperature, chemicals, etc., on microplastics. Since procedures for extracting, quantifying, and analyzing MPs in labs are time-consuming, finding adequate preservative and storage materials is crucial to prevent sample deterioration over time and ensure higher sample quality for analysis. Therefore, this review paper aims to provide a detailed methodology for pre-analysis methods, including sampling, sample storage and preservation, and sample preparation (extraction and pre-treatment) to have high-quality data reflecting MPs abundance in the freshwater water and sediment. First, since these freshwater sources are immense, it is vital to carefully select the sampling sites, giving an accurate image of the whole environment. Second, storage and preservation of collected samples are also necessary since, without proper preservation and storage methods, the quality of samples and MPs can change due to biological activities. Finally, extraction and pre-treatment of samples should be conducted since obtained samples contain impurities (organic tissues, silts, sands, etc.) which can attach to MPs and cause deviations in results for both qualitative and quantitative estimations about MPs. As shown in Fig. 3, this review briefly explains different applied sampling methods for freshwater water and sediment sampling, including using nets, pumps, and bulk sampling for water and utilizing corer and grabbers for sediment sampling. Then, the most dominant part of this research relates to the importance of sample preservation and storage using different physical, chemical, and biological methods. Afterward, obtained samples would be purified before moving to the analysis step with various digestion and separation methods. Considering the importance of pre-analysis measures and to the best of our knowledge, no comprehensive studies have focused on this area, and this critical step of MP studies is often overlooked in all review papers. In this sense, the primary objectives of this paper are:
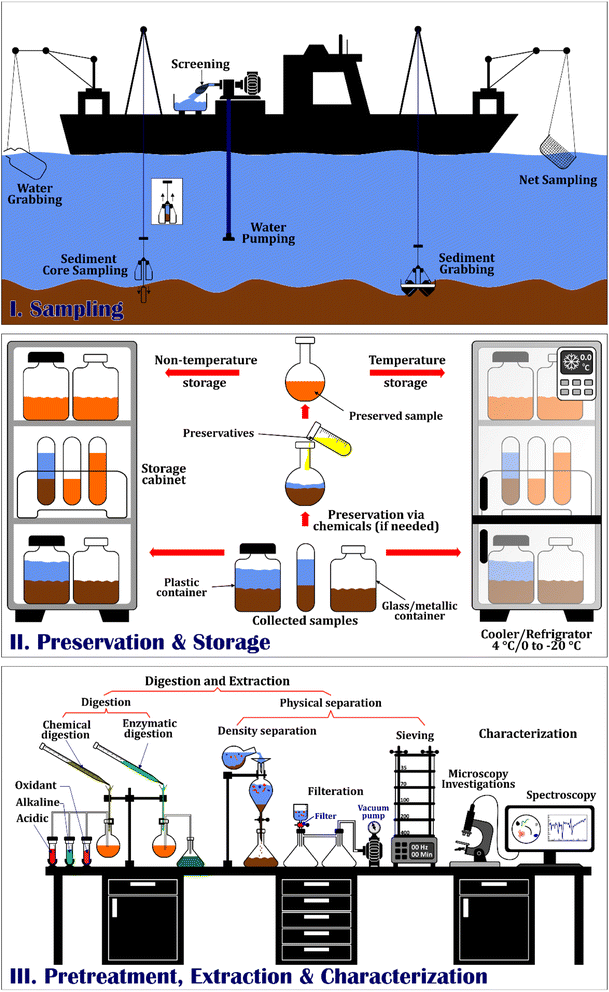 |
| Fig. 3 Different pre-analysis phases in MPs studies (starting from steps 1 to 3). | |
(1) Review the current state of sampling and preparation (extraction and pre-treatment) methods for freshwater water and sediment samples and other intermediate steps before the analysis stage.
(2) Provide data relating to different chemicals, storage materials, and preservation temperatures that should be used to improve the representativeness of obtained samples.
(3) Make recommendations for using different preservatives and storage materials based on the studied variables.
2. Sampling
Sampling is the first and one of the most crucial steps in MP detection studies and involves careful selection of the site for sampling and the sampling procedure to be employed. There are three different sampling procedures in any given matrix: selective, bulk, and volume-reduced methods.39 Selective sampling detects plastic debris using the naked eye and is common when desired MPs are larger – about 1 to 5 mm, for example, large MPs in sediment samples, preferably on the shorelines.40 Bulk sampling describes techniques that take a sample from the environment in its entirety without diluting it. This method is used when the entire sample volume needs to be explored and a smaller sample size could result in inaccurate results.41 The other type of sampling is volume-reduced methods and refers to procedures by those larger areas of investigation can be mapped and analyzed. This technique could be used both for water and sediment sampling but is preferable for water sampling, and the main applied instruments are nets and sieves.40,42,43 In several cases, these methods were combined and performed together to increase sampling accuracy. For instance, pumping large areas followed by sieving is the combination of bulk and volume-reduced methods used in MP studies.44 Different sampling methods have been used considering the goals of studies in freshwater compartments, elaborated on in the following sections.
2.1 Water sampling
Based on the study goals and site accessibility, various sampling methods are proposed, among which volume-reduced methods have been widely used in water sampling. Bulk sampling is another method which is less efficient for water sampling since it requires a high amount of water to be representative, decreasing its feasibility. This method is mostly used when the main objective of the study is to evaluate fibers that can pass through volume-reduced sampling instruments.45,46 Each sampling method has merits and demerits that should be considered before choosing the method. A comparison between different sampling methods for freshwater water sampling is summarized in Table 1.
Table 1 Advantages and disadvantages of sampling using nets, grab samplers, and pumps
Method |
Advantage |
Disadvantage |
Nets |
Able to analyze larger study areas and catch higher concentrations of MPs |
Underestimating small particles, particularly fiber, which could easily escape |
High risk of secondary MP contamination through exposure to air and net materials (nylon) |
Preferable when the MP detection is done through the naked eye |
Less accurate reported sampling volumes due to inaccuracy of volume calculating through flow meters or mathematical operations |
More number of preparation steps, increasing airborne contamination of samples |
Low price and easy accessibility of nets with large mesh size (>330 μm) |
Low repeatability to assure volume accuracy |
High cost of nets with a small mesh size (<330 μm) |
Grabs |
Could investigate the broader size of MPs by selecting smaller filters and sieves |
Small volumes of grabs cause high variability between samples |
Able to be used in an environment where net sampling is tough |
Able to be applied for sampling from deeper columns of water with Niskin bottles |
Decrease the risk of secondary pollution due to shorter contact time with the sampling compartment and using non-plastic containers |
Lower particle concentration compared to nets, culminating in more probable false zero reports |
Can be conducted by citizen science method, increasing the accuracy of sampling |
Appropriate reports of MP abundance in the precise volumes |
Non-plastic grab samplers could be heated up to 500 °C before sampling to eliminate any potential residues |
Difficulty in the transportation of large volumes of bulk samples to the laboratory |
Require only one filtration, decreasing the risk of airborne contamination |
High repeatability to assure volume accuracy |
Pumps |
Could investigate the broader size of MPs by selecting smaller filters and sieves |
Risk of secondary plastic contamination through the materials of pumps, ropes, and filters |
Able to be used in an environment where net sampling is not applicable |
Lower particle concentration compared to nets, culminating in more probable false zero reports |
High repeatability to assure volume accuracy |
High risk of the clogging of limited filters area when a large amount of water is investigated |
Fragmentation of MPs to nanoplastics due to shear stresses caused by pumps blades |
When a more extensive sampling area is preferred, nets hauled by boats or individuals, or stationary places, such as bridges, are more favorable. However, to minimize early clogging and avoid missing smaller particles, this approach necessitates the careful selection of the nets. Thereby, for smaller MPs (≤330 μm), bulk sampling and collecting samples through pumps, followed by filters and grab samplers can be used.
2.2 Sediment sampling
Apart from the water matrix, MPs have been abundantly found in freshwater sediments. Most plastic compounds in aquatic environments have different states of buoyancy based on their density, as shown in Table S1.†47 It is estimated that roughly 70% to 90% of MPs are present in the sediment.48 However, the percentages might change based on ambient characteristics such as hydrodynamic regimes and biofouling. Different factors can affect the presence of MPs in water and sediment as shown in Fig. 4. In general, low density particles are afloat and high density particles are sunk in the sediment. However, this behavior may be different in real conditions. For instance, in a turbulent flow, even positively buoyant particles can be transferred through the vortical structures to deeper water and, via other processes, such as density currents, be trapped in the sediment. Furthermore, MPs are known to possess hard and easy-to-colonize surfaces, facilitating the microorganisms' colonization. Also, the surface area of plastic particles on the micro and nanoscale grows, increasing open pores for microorganisms' colonization, causing even light MPs to settle in sediments.47,49–52 The combined effect of biofouling and turbulent-induced mixing can lead to the presence of positively buoyant MPs in sediments.30
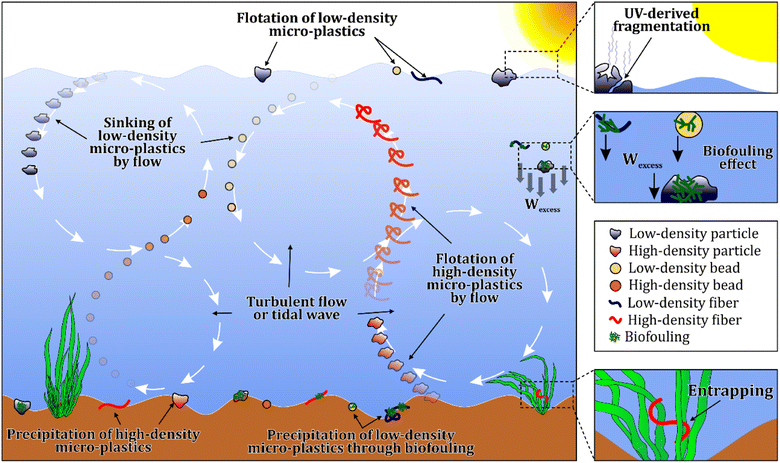 |
| Fig. 4 Schematic of MP settling behavior affected by different parameters. | |
Like water sampling methods, the procedures for MPs collection in sediments are selective, bulk, and volume-reduced sampling. The leading equipment for selective sampling of sediments is tweezers, and this procedure is efficient for detecting larger MPs.53 Further, from riverbanks/lakeshores, samples can be collected using stainless steel shovels, spoons, and spatulas,54 while sampling from the bottom of lakes can be done using different grabbers, corers, and samplers.39,55 The primary sampling equipment is Ekman, Van Veen grab, and the Box Corer. For sediment sampling from depth, a columnar sampler (inner diameter = 7 cm, height = 100 cm) is required.51 Corer-based methods are also preferable when the bottom of the water bodies is hard, leading to the malfunction of grab-based methods, which cannot close efficiently.56 However, corer-based methods are ineffective when the risk of core freezing is high. The number of sample replicates is another factor affecting the accuracy of sediment sampling which is reported to be varying from 2 to 5 times.57 The sampling depth is also important, and it is noted that most MPs occur in the top 5 cm of the sediment layers, however, some recent studies included the collection of deeper sediments for vertical analysis of MP appearance in the samples.1,58,59 Further, a recent study proved that MPs abundance decreases with an increase in sediment depth.60 Another study showed that bigger MPs (4–5 mm) are more abundant in shallower layers, but the number of MPs raised by an increase in depth for smaller MPs (<2 mm). The number was 40 items per kg of wet sediment (items per kg WW) in 0–10 cm and 110 items per kg WW in 40–50 cm.61
3. Sample storage, preservation, and contamination control
After obtaining samples, they are often transported to the laboratories and stored before analysis to prevent physicochemical and microbial reactions.56 This process may take time to transfer samples from sampling sites to the labs, altering the quality of obtained samples. As a result, optimizing the storage conditions, storage containers, temperature, and preservation chemicals becomes imperative.
3.1 Sample storage materials
The type of containers being used for microplastic sample storage is an important parameter to consider, as they can influence the stability and contamination of some analytes. The use of non-plastic containers, such as glass, steel or aluminum-made vessels, is preferable since these containers reduce unwanted plastic pollution.42,62 However, some studies have also used polymer-based vessels, such as PE bags,63 PET jars,64 PP bags,65,66 and Whirl-Pak or zip-lock bags.67,68 Unlike glass containers, which can break due to falling, especially while sampling on boats, plastic-based vessels can be used in sampling aboard ships and boats. Nonetheless, due to the plastic abrasion, particularly by sediment grains, the above materials might cross-contaminate the samples with MPs, hence is not recommended to store sediment samples.56 If it is not feasible to avoid storage of samples in polymeric containers, appropriate blanks should be considered in different stages of analysis, namely sampling, pre-analysis, and analysis phases. By subtracting the findings MPs from blank data, the overestimation caused by the abrasion of plastic materials could be hindered. Field and laboratory blanks in the study of MP abundance in Lake Simcoe show 1 to 27 particles, which were subtracted from MP numbers before characterization analysis.63 A study on different containers for storing food and water revealed that various factors such as physical stress and squeezing, scissoring, tearing, and cutting the bottles expand the abundance of MPs in the stored samples. These studies revealed that plastic containers could be a source of MP overestimation/cross-contamination.69,70 Besides, the aging process could increase plastic cross-contamination, another attribute of plastic containers.71 In terms of recycling/non-recyclable plastic containers, it is proved that recyclable plastic-made containers release MPs into the samples 8 times higher than single-use plastic containers, showing the effect of plastic aging on the leachate of MPs into the samples.70Table 2 provides more information about the storage condition governed in different freshwater studies. It can be seen that only very few studies used polymeric storage containers to store the water and sediment materials, which is mainly related to avoiding adding unwanted plastic contamination due to the abrasion of polymeric containers. Researchers have used glass containers in most of the mentioned studies in Table 2. However, in conditions that sways related to the transportation of samples will happen, glass materials may fall and break. Therefore, metal-based containers, stainless steel or aluminum, should be considered to avoid the risk of glass breaking.
Table 2 Details of MP sampling and preservation in different studies
Location |
Sampling method |
Storage, preservation, and pretreatment methods |
Abundance |
Reference |
Lake water
|
(1) Taihu Lake, China; (2) three lakes, Poland; (3) Lake Naivasha, Kenya; (4) Lakes Mead and Mohave, U.S. (5) Veeranam Lake, South India; (6) four lakes, Italy; (7) Dimon Lake, Carnic Alps; (8) Nine Lakes, Argentine; (9) Lake Guaíba, Brazil; (10) Red Hills Lake, India |
Net sampling |
Storage and preservation: (1, 10) 1 L glass container containing 5% methyl aldehyde; (2) 100 mL screw-capped vials; (3) poured in glass bottles and kept at 4 °C (4) glass jars containing isopropyl alcohol (5) a 250 mL amber bottle; (6) kept at 4 °C in glass vials containing 30% hydrogen peroxide; (7) frozen at −20 °C in plastic-free containers; (8) glass bottles at −20 °C; (9) 250 mL glass vial, stored at 0 °C |
(1) 3.4–25.8 items per L; (2) 4930 fibres per m3; (3) 0.183 ± 0.017–0.633 ± 0.067 items per m2; (4) 0.44–9.7 items per m3; (5) 28 items per km2; (6) 100 036 MPs per km2; (7) absence of MPs; (8) 0.9 items per m3; (9) 11.9 ± 0.6–61.2 ± 6.1 items per m3; (10) 5.9 items per L |
88, 91 and 100–106 |
Pretreatment: (1, 5, 8) 30% H2O2 digestion + drying; (2) 69% nitric acid + 30% H2O2 digestion; (3) 30% H2O2 and Fenton digestion + drying; (4) sieving + 30% H2O2 digestion and Fenton reagents + sieving + density separation (lithium metatungstate); (5, 7); (6) density separation (NaCl); (9) sieving + 35% H2O2 digestion and Fenton reagents + 35% H2O2 digestion + density separation (NaI); (10) sieving + drying + 30% H2O2 digestion and Fenton reagents + drying + density separation (NaCl) |
(1) Lake; Simcoe, Ontario; (2) Rawal Lake, Pakistan; (3) La Salada lake, Argentina; (4) Remote; Mountain Lake, Switzerland |
Grab sampling |
Storage and preservation: (1) stored in pre-cleaned polyethylene or PET jars and preserved in 20% ethanol solution; (2) the glass bottles held at 4 °C; (3) 4% formalin |
(1) 0–0.7 items per L (grab); 0.4–1.3 items per m3 (trawl); (2) 1.4 items per L (3) 140–180 items per m3; (4) 2.6 microplastics and 4.4 fibres per litre |
63 and 107–110 |
Pretreatment: (1) sieving + rinsing; (2, 3) 30% H2O2 digestion + drying |
(1) Lake Donghu, China; (2) Ox-bow Lake, Nigeria |
Pump sampling |
Storage and preservation: (1) stored in 50 mL brown glass jars containing 5% methyl aldehyde and at 4 °C; (2) stored in a clean beaker and preserved at −3 °C |
(1) 7.4–29.6 items per L; (2) 1004–8329 items per m3 (dry season); 201–8369 items per m3 (wet season) |
111 and 112 |
Pretreatment: (1, 2) 30% H2O2 digestion + drying |
River water
|
(1, 3) Gave de Pau River, France; (2) Milwaukee Rivers, USA; (4) Illinois, USA; (5) Vistula River, Poland; (6) North Saskatchewan River, Canada; (7) Three Manitoban Rivers; (8) Ganges River, India; (9) Citarum River, Indonesia; (10) Upper Guayllabamba River Basin, Ecuador; (11) Rogue River, USA; (12) Rhône and Têt Rivers, France; (13) Thames River, UK; (14) Magdalena River, Colombia; (15) Surabaya River, Indonesia; (16) Ofanto river, Italy; (17) Tamsui River, Taiwan |
Net sampling |
Storage and preservation: (1, 2, 3, 9, 12, 14); (5) glass containers containing formaldehyde; (4, 6, 8, 10, 11; 16) glass jar, storing at 4 °C; (7; 15) stored in non-plastic containers and preserved with 70% ethanol; (13) 4% formalin; (17) zip-lock bag and preserved at 3 °C |
(1) 100.6 ± 99.9 fibers per m3; (2) 0.42–2.71 particles per m3; (3) 3.34 ± 0.20 particles per m3; (4) 2.355 ± 0.375–5.733 ± 0.850 particles per m3; (5) 1.6–2.55 particles per L; (6) 26.2 ± 18.4 particles per m3; (7) 113 888–1241 085 particles per km2; (8) 380–684 particles per 1000 m3; (9) 0.0574 ± 0.025 particles per m3; (10) 1584 particles per m3; (11) 0.001–0.249 particles per m3; (12) 11.6 ± 17.7 particles per m3; (13) 14.2–24.8 particles per m3; (14) 0.097–0.135 fibers per L; (15) 0.76–43.11 particles per m3; (16) 0.9–13 particles per m3 |
18, 58, 59, 67, 89, 90, 92 and 113–122 |
Pretreatment: (1) enzymatic digestion (biozyme F + biozyme SE) + 30% H2O2 digestion + drying + density separation (ZnCl2); (2, 3, 7) 30% H2O2 digestion and Fenton reagents + sieving; (4, 8, 10) sieving + heating + 30% H2O2 digestion + density separation (NaCl); (5, 17) sieving + 30% H2O2 digestion + drying; (6) sieving + heating + density separataion (ZnCl2) + 30% H2O2 digestion and Fenton reagents + sieving; (9) sieving + 30% H2O2 digestion and Fenton reagents + heating + density separation (ZnCl2); (11) sieving + digestion (KOH) + density separation (NaCl); (12) sieving + 40% H2O2 digestion + drying; (13) sieving + 40% KOH digestion + drying; (14) sieving + drying + density separation (NaCl); (15, 16) sieving + drying + 30% H2O2 digestion and Fenton reagents + density separation (NaCl) |
(1) The Manas River, China; (2, 3) Ottawa River, Canada; (4) St. Lawrence River, Canada; (5) Netravathi River, India; (6) Fengshan River, Taiwan; (7) three rivers, UK; (8) Yellow River, China; (9) Maozhou River, China |
Grab sampling |
Storage and preservation: (1, 2; 6; 9) kept at 4 °C; (3) stored in Whirl-Pak bags; (4) Whirl-Pak bags and kept at −20 °C; (5, 7) stored in a glass container; (8) amber bottles with foil-lined caps, preserved in 4 °C |
(1) 32 particles per m3: (2) 1.35 particles per m3; (3) 0.11 particles per L; (4) 0.12 ± 0.01–0.16 ± 0.02 particles per L; (5) 288 particles per m3; (6) 334–1058 particles per m3; (7) 0.4 particles per L; (8) 497–930 particles per L; (9) 4–25.5 particles per m3 |
68 and 123–130 |
Pretreatment: (1) Sieving + 30% H2O2 digestion and Fenton reagents; (2, 3, 7; 9) 30% H2O2 digestion; (4) oil separation + 30% H2O2 digestion + heating; (5, 6) sieving + drying + 30% H2O2 digestion and Fenton reagents + density separation (ZnCl2); (8) centrifuging + density separation (NaCl) + 30% H2O2 digestion |
(1) Ganges River, India; (2) Haihe River, China; (3) Yongjiang River, China; (4) Qin River, China |
Pump sampling |
Storage and preservation: (1) double-wrapped in foil and placed in polypropylene bags; (2) stored in glass jars covered with aluminum foils and preserved at 4 °C; (3) stored in a glass bottle and preserved in 5% methyl aldehyde; (4) stored in polypropylene containers in ice boxes |
(1) 0.038 particles per L; (2) 0.69–74.95 particles per m3; (3) 500–7700 particles per m3; (4) — |
24, 65, 131 and 132 |
Pretreatment: (1); (2) sieving + 30% H2O2 digestion + density separation (NaCl) + drying; (3) 30% H2O2 digestion and Fenton reagents; (4) 30% H2O2 digestion + density separation (NaCl) |
Lake sediment
|
(1) Three Gorges Reservoir, China; (2) Yangtze River Basin, China; (3) Taihu Lake, China; (4) Lake Simcoe, Canada; (5) Lake Victoria, Africa; (6) Lakes Mead and Mohave, U.S; (7) Veeranam Lake, South India; (8) Dimon Lake, Italy; (9) Ox-bow Lake, Nigeria; (10) Red Hills Lake, India; (11) Vembanad Lake, India |
Grab sampling |
Storage and preservation: (1, 3) non-plastic container, preserved at 4 °C; (2) glass jar containing 5% formalin and then preserved at 4 °C; (4) amber glass bottles; (5, 8, 9) non-plastic bottle and frozen; (6) glass jars and preserved with isopropyl alcohol and stored at room temperature; (7, 10, 11) - |
(1) 25–300 particles per kg ww; (2) 50–580 particles per kg; (3) 11.0–234.6 particles per kg dw; (4) 8.3–1070 particles per kg; (5) 0–1102 particles per kg dw; (6) 87.5–1010 particles per kg dw; (7) 309 particles per kg; (8) no MPs; (9) 347–4031 particles per kg; (10) 27 particles per kg; (11) 252.8 particles per m2 |
63, 88, 100, 103, 105, 110, 111 and 133–136 |
Pretreatment: (1) 30% H2O2 digestion + drying + two steps density separation (NaCl + NaI); (2) 30% H2O2 digestion + drying + density separation (NaCl); (3) density separation (NaCl) + 30% H2O2 digestion; (4) sieved + drying + density separation (CaCl2); (5) density separation (NaCl) + 30% H2O2 digestion and Fenton reagents + density separation (NaCl) + drying; (6) drying + density separation (lithium metatungstate) + sieving + 30% H2O2 digestion and Fenton reagents + sieving + density separation (lithium metatungstate); (7) sieving + drying +30% H2O2 digestion + density separation (Milli Q deionized water) + density separation (ZnCl2); (8) density separation (NaCl) + drying; (10) sieving + drying + 30% H2O2 digestion and Fenton reagents + density separation (NaCl) + drying; (11) sieving + drying + 30% H2O2 digestion + density separation (NaCl) + drying |
(1) Yangtze Estuary, China; (2) Fuhe River Estuary, China; (3) Lake Ontario, Canada |
Core sampling |
Storage and preservation: (1) non-plastic containers, kept at 4 °C; (2); (3) PET bottles, stored at −25 °C |
(1) 10–60 particles per kg dw; (2) 1049 particles per kg; (3) 760 particles per kg dw |
51, 64 and 137 |
Pretreatment: (1, 2) drying + density separation (NaCl) + 30% H2O2 digestion; (3) drying + density separation (sodium polytungstate) + density separation (deionized water) |
River sediment
|
(1) Yangtze River, China; (2) Karnaphuli River, Bangladesh |
Grab sampler |
Storage and preservation: (1, 2) stored in a non-plastic container and frozen |
(1) 34 particles per kg; (2) 22.3–59.5 items per kg dw |
138 and 139 |
Pretreatment: (1) density separation (ZnCl2) + drying; (2) drying + density separation (ZnCl2) + sieving + 30% H2O2 digestion and Fenton + density separation (NaCl) + sieving |
3.2 Sample storage temperature and preservation chemicals
Besides container type, maintaining the temperature of samples while transferring from sites to laboratories and storing them in laboratories to avoid sample deterioration, mainly caused by biological reactions, is essential.56,72 Although microplastics are designed to be durable, recent reports have unveiled the presence of potential microbes that can degrade microplastics.73–76 Sediment and soil microorganisms in marine and terrestrial ecosystems have been reported to biodegrade debris and MPs.76–78 For instance, commercial synthetic organic polymers, such as polyesters polylactic acid (PLA), polycaprolactone (PCL), polybutylene adipate terephthalate (PBAT) and polyhydroxybutyrate (PHB) contain polyester bonds, which can be degraded by enzymes (esterase), released by ubiquitous bacteria and fungi.76 These potential plastic-eating microbes can attach to the surface of the polymer (formation of biofilms) and release extracellular enzymes, such as lipase, laccase, polyesterase, and lignin peroxidase, resulting in depolymerization (polymer to oligomers and dimers) and ultimately mineralization (carbon dioxide and water). Different synthetic MPs have different degrees of biodegradability and require suitable conditions-pH, temperature, nutrients, and humidity.
Although the process is very slow, to maintain the integrity of the MPs in collected samples, it becomes necessary to contain the microbial action and prevent biodegradation of the polymeric chains by providing unfavorable conditions, such as cold temperatures. It is a common practice to store environmental samples including water, soil, sediment, and sludge at 4 °C until further use and is often used for MPs as well. However, as 4 °C only slows down the bacterial activity, it is effective for only a short period (a few weeks, less than a month), as suggested for sludge and sediment samples.79,80 For long-term storage, cryopreservation-freezing or ultra-freezing conditions (−20/−80 °C) are advised to suppress both biological and chemical reactions, which can otherwise affect the composition of the samples.81 The schematic of sample and MPs deterioration caused by bacterial growth and activity is illustrated in Fig. 5. As seen in the figure, long-term storage of MPs without proper preservation and storage could, directly and indirectly, affect the quality of MPs contained samples. In other words, plastic-ingesting organisms may affect the MPs in the water and sediment, changing their characteristics and shapes and decreasing the accuracy of sample analysis (the right side of the figure).82 Apart from plastic-ingesting organisms, algae, fungi, etc., could grow inside the samples. As a result, the samples would need extensive pretreatment processes, particularly the digestion process, to eliminate the growth organisms, which increases the risk of the negative impact of digestion chemicals on the particles. Without extensive pretreatment methods, some particles, especially transparent MPs, could hide behind these impurities, adding error to the analysis process.
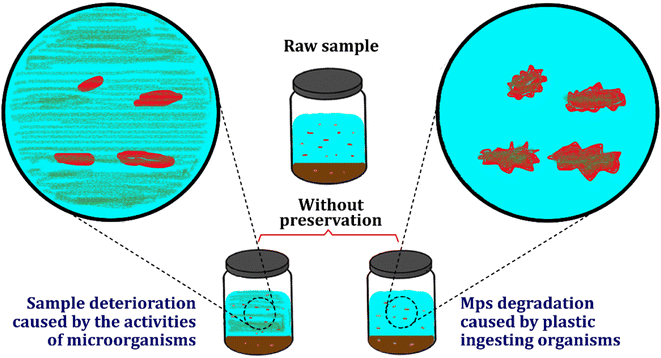 |
| Fig. 5 Schematic of bacterial effect on unpreserved samples. | |
When storing the samples in ultra-freezing temperatures, it becomes necessary to study the effect of freeze–thaw cycles on the MPs. Recent reports have revealed that continuous freeze–thaw cycles can accelerate the aging of MPs and affect their physicochemical properties. Aged MPs are reported to have smaller sizes, increased specific surface area (SSA), and more oxygen-containing surface functional groups, leading to increased adsorption sites for co-existing contaminants, such as heavy metals and organic matter. A recent study by Sun et al. (2022) reported that the −25/+25 °C freeze–thaw cycle increased the SSA, pore size and pore volume of PVC. They have also reported that freeze–thaw cycle aging of PE results in increased pore size (from 29.8 nm to 123.4 nm) and pore volume due to local rupture of the microplastic surface due to water penetration during thawing.83 Also, freeze–thaw cycling can destroy the amorphous region of the polymers, thereby increasing the crystallinity and making the polymer more brittle. A few reports also reveal the formation of stable aggregates in MPs suspension after exposure to 10 freeze–thaw cycles.84,85 Barb and Mikucki (1959) reported that frozen suspensions of 50–100 nm polystyrene latex particles remained agglomerated even after thawing at room temperature.84 Therefore, from these reports, it is evident that continuous freeze–thaw cycles can affect the characteristics of the MP sample and must be avoided to introduce any deviations in the results. One way to avoid continuous freeze–thaw cycles is to store the samples in aliquots. Another prospective course of action is lyophilization, as suggested recently by.86 The collected samples can be subjected to centrifugal force to separate clear liquid and solid fractions containing the MPs. The liquid fraction can be filtered, and MPs collected on filter paper or discarded, and the solid fraction can be frozen overnight, followed by lyophilization or freeze-drying. The dry samples can then be passed through molecular sieves of desired sizes to obtain the MPs of interest. Freeze drying of sludge is a frequent practice and can be extrapolated to sediments containing MPs.87 The lyophilization of samples will eliminate the storage of large amounts of samples/space requirements. Since the method does not use any solvent or elevated temperatures, the samples are supposed to retain their physicochemical and mechanical properties and can be stored at room temperature, or 4 °C, for long periods.
However, in some cases, freeze-drying may not kill all bacteria and it is safe to use a preservative, such as 5% methyl aldehyde, 20–70% alcohol, and formalin, to further inhibit microbial growth in free-dried samples. These are industrial disinfectants and have been commonly used for the preservation of various samples to retard both biological and natural chemical changes, which can affect the sample characteristics.56,63,88–90 For instance, formalin 4% and formaldehyde were used to preserve lakes and rivers water samples with MPs ranging from 32 to 5000 μm.90–92 Further, a recent investigation into the identification of fiber microplastic in Lake Simcoe used 20% ethanol to preserve water samples.63 In other studies, samples collected by manta trawling were preserved in 70% ethanol solutions.59,89Table 2 expands further on the sampling and preservation of the collected samples from lakes, rivers, and sediments in recent studies. As can be observed in Table 2, a large number of lake and river water samples have been stored at low temperatures, 4 °C and 0 °C. However, in some of the studies, and based on the organic load of the samples, samples could be kept in freezers. Regarding the preservative chemicals, few studies have preserved their samples in chemical solutions, which could end up with inaccuracies in the results due to possible alteration of sample structures.
The selection of the preservative, however, depends on the chemical compatibility of the solvent and the chemical makeup of the MPs of interest. The most important factors that should be considered are the solubility condition of polymers and chemicals and the polarity condition of these materials. When polymers and chemicals have the highest difference in solubility and polarity states, the polymers will stay in a compatible condition. Otherwise, the situation is considered incompatible, causing changes in the polymer structure.93 Since most of the MPs detected in freshwater compartments are attributed to PE, PP, PS, and PET, the chemical compatibility of these materials with solvents, such as alcohols, aldehydes, and formaldehyde, is summarized in Table 3. It should be noted that, as mentioned earlier, there is a giant gap in the analyzed research papers about the potential effects of different environmental and experimental conditions on the found microplastics. Therefore, the table is provided based on the chemical compatibility charts provided by polymer industries and polymer handbooks. It is evident that PP is highly resistant to solvents and chemicals and does not show any damage from exposure to them. To strengthen and cite, a recent study that evaluated PP samples under different solvers has shown that the mass, dimensions, or thermal properties of the samples remained unchanged.94 Further, as per the results from the literature, PS, PP, and PET polymers have good compatibility in an ethanol solvent.94,95 Solvolytic reactions, like alcoholysis (methanolysis), hydrolysis, transesterification, and glycolysis, generally attack the C–X bonds (where X is the heteroatom, such as O, N, Cl, in the polymeric chain), for example, polyesters (PET) or polyamides. Transesterification of PET using alcohols is a common technique for chemical recycling; however, it requires supercritical conditions, higher temperatures (180–220 °C), an inert atmosphere, and catalysts.96–98 Therefore, preserving MP samples in alcohols, such as methanol, at 4 °C or under ambient conditions does not affect the chemistry of the polymer PET even after 30 days of constant exposure and can be used for the preservation of MP samples containing PET. Similarly, LDPE, HDPE, and PS show little to no damage, after 30 days of exposure, when preserved in ethanol, isopropanol or formaldehyde. Whereas PVC is susceptible to nucleophilic attack-both substitution, and elimination, followed by dichlorination, as suggested by.99 The conjugate base of alcohols, or the alkoxides (R–O−), are good nucleophiles and can attack the polymeric chain to alter its properties within 30 days of constant exposure (Table 3). The mentioned table is obtained based on the chemical compatibility of polymers at room temperature. More details regarding the storage, preservation, and precautions required for maintaining the quality of samples over longer times are given in Table 2. However, it is essential to notice that in almost all of the reviewed articles, the effect of preservation methods on MPs has yet to be analyzed, which may cause the misidentification of MPs since different conditions could cause changes in plastic physical and chemical characteristics. Therefore, it should be of the most important factors to be considered in the upcoming microplastic studies. Thermoplastic polyesters and Nylon-66 become brittle even in water after two months,93 which shows the vital role of this missed part of microplastic research. Therefore, we strongly recommend devising spiking tests evaluating the effect of preservation/lack of preservation on the obtained results.
Table 3 Chemical compatibility of common polymers with preservation chemicalsa,b,c
Solvent |
MPs |
LDPE |
HDPE |
PP |
PS |
PVC |
PET |
E: 30 days of constant exposure causes no damage. Plastic may tolerate for years.
G: little or no damage after 30 days of constant exposure to the reagent.
B: some effect after 7 days to the reagent. The effect may be crazing, cracking, loss of strength or discoloration.
There is no conclusive evidence on the effect of formaldehyde on PET.
|
Ethanol |
E |
E |
E |
E |
G |
E |
Methanol |
E |
E |
E |
G |
G |
G |
Isopropyl alcohol |
E |
E |
F |
B |
B |
B |
Formaldehyde 10% |
E |
E |
E |
E |
E |
—d |
3.3 Quality assurance/quality control (QA/QC)
Besides storage and preservation methods, quality assurance is imperative for a more accurate MP analysis. Apart from sampling sites and laboratory storage, all the equipment should be cleaned before usage and be covered with aluminum or glass lids when applicable.56,62 All surfaces should be rinsed with either Milli-Q water or alcohol and working under laminar airflow in laboratories and fume hoods would be preferable.140 Although laminar flow hoods draw air through high-efficiency particulate air (HEPA) filters to make laminar airflow, preventing the entrance of uncontrolled air, all labs may not have access to this advanced equipment.141 Also, bright colour sponges and other clothes can be used to ease the detection of potential secondary contaminations.142 To avoid fiber contamination, researchers should wear cotton clothes and latex gloves, and the exposure of samples should be minimized by isolating them.56 Considering specific spaces in labs that are not in laboratory traffic zones, pre-filtering all utilized solutions, and conducting blanks in different steps should also be considered.62 Moreover, working solutions used in the laboratory processes, including preservation, preparation and extraction procedures, should be pre-filtered and kept in suitable containers.62 Also, water sampler materials, i.e., nets, pumps, and grabs should be selected meticulously to decrease the risk of unwanted secondary contamination originating from samplers. To do so, Karlsson et al. (2019) suggested the use of a net with an aluminum frame and nylon net. Apart from nets, non-plastic pumps should be used for water sampling via pumps, and they recommended using stainless steel pumps, filters, and ropes for submerging the pump from the boat.143 Heating, cooling, or rinsing of the used lab instrument, including beakers, flasks, filtration systems should be done before sampling and analytical investigations.62
4. Sample preparation (extraction and pre-treatment)
After being transported to the laboratories, samples should be prepared for the next steps of MP analysis preparation. This is an essential and critical step in MP's quantitative and qualitative analysis.144 Based on the environmental sampling parameters such as the load of organic/inorganic particles, and the size of impurities in samples, morphology of water/sediment surface, bottom gradient, vegetation, etc., different steps, including but not limited to digestion and extraction are used for the preparation step of both water and sediments.145,146
4.1 Sample heating
To obtain an accurate picture of MP presence in water and sediment samples and eliminate the effects of humidity, various studies applied heating as the first step of the preparation procedure. The most frequent method for this purpose is drying samples at high temperatures (between 35 °C to 100 °C) until reaching a constant weight.105,147,148 However, to avoid probable MP deformation, it is suggested to put samples in an oven under 60 °C.39 It is imperative to acknowledge that permeation is a factor that can compromise the structural integrity of polymers. The rate of permeation of a polymer can be augmented by an increase in temperature, due to two primary reasons. Firstly, the solubility of the permeant in the polymer becomes more pronounced at elevated temperatures. Secondly, the polymer chains exhibit more extensive movements at higher temperatures, facilitating the diffusion of the permeant. Hence, it is vital to consider the heating temperature within a range that does not jeopardize the polymer structure.93
4.2 Sample digestion
Another pre-treatment step is to purify samples that indeed contain organic matrix. Two main water/sediment sample purification strategies are enzymatic and chemical digestion/oxidation of the organic matrix. Chemicals that are mainly added to digest organic matter can be divided into three main categories, acid, alkaline, and hydrogen peroxide-based oxidation.140 It is proven that using acid and alkaline as digestion materials can result in the degradation and damage of MP structures.149 Therefore, hydrogen peroxide-based methods have been widely used in studies as a digestive reagent in both water and sediment samples.39,150 It is proven that the number and size of MPs are not affected by adding hydrogen peroxide, although they may be discolored.148 Acidic digestion shows weight loss in PE, PET, PP, and PS structures since acids could dissolve these polymers and cause shifts in their functional group's spectroscopy. The alkaline process shows a significant surface change in PET films since this polymer consists of carbonate and ester linkages, which makes them susceptible to alkaline hydrolysis of these functional groups.151 Enzymatic degradation could also be employed for sediments containing high amounts of planktons.152 However, this method is mainly used when the study aims to evaluate MP presence in biota tissues. Besides, enzymatic methods are expensive, the preservation of enzymes is challenging, and different enzymes are required for degrading various organic compounds.39 Another method for degrading organic compounds presenting in water and sediment samples is using Fenton reagents. This method is based on the reaction between hydrogen peroxide and Fe2+ ions to generate hydroxyl radicals, oxidizing the organic contaminants.153 Using Fenton reagents combined with peroxidation is a method recommended by National Oceanic and Atmospheric Administration (NOAA).153 In a study, 98% of organic load reduction and recovery rate with no alteration in polymer structures were achieved using the Fenton oxidation method.154 30% H2O2 was used for water samples' digestion in urban surface water in Wuhan, China, for 24 h and at room temperature, and the abundance of MPs was up to 8925 ± 1591 particles per m3.155 The combination of sediment samples drying, followed by digesting using 50% HCl and 30% H2O2 was utilized for water and sediment preparation, and 90.6 ± 2.9% to 93.5 ± 5.7% recovery rates for water samples and 96.8 ± 4.3% to 104.5 ± 3.7% for sediment were achieved for PET microplastics. As the authors mentioned, more than 100% of the recovery rate for sediment samples is related to errors that happened during the experiments.156 Based on the quantity of organic matter present in samples, adding hydrogen peroxide to samples under temperatures and purity attracts the researcher's attention and is the method that has been widely used in studies related to freshwater sediment purification.112,133,139,148,157 Based on the mentioned reasons, hydrogen peroxide-based methods are shown to be the best method for freshwater water and sediment samples due to their higher recovery rate and ease of application, which is following the provided literature review depicted in Table 2.
4.3 Density separation
The separation of MPs from inorganic compounds such as sands and silts is another important extraction method. This method is mainly used when the sample contains fine and coarse grains that may cause the entrapment of MPs in these ambiances.39 The density of most MPs existing in freshwater bodies varies between 0.01–2.3 g cm−3 (Table 2). Some particles are buoyant with densities higher than ambient water, and others are negatively buoyant and will sink into a quiescent fluid.30,47 It has been mentioned that only 46% of MPs float on the marine water's surface.144 It should be noted that since the density of freshwater is lower than saline water, these values may be lower in freshwater environments. Therefore, density separation methods are used to separate entrapped MPs from water and sediment samples. However, it is mentioned that density separation is rarely used for extracting MPs from water samples.57 Density separation methods are based on the floatation of lighter particles on the surface of high-density salt solutions (varies from 1.2 to 1.8 g cm−3).158 With all this considered, based on the density of the solution used for density separation, different types of MPs with various densities are extracted from the sediment samples.42
Different solutions and liquids are used in the density separation stage, the most frequently used of which is NaCl solution. These various materials and chemicals have merits and demerits listed in Table 4. In addition, some studies conducted two-step density separation to assure the highest separation of MPs. First, the sample bulks were separated using high-density NaCl to reduce bulk volume. Then, the residues were density-separated using denser solutions such as NaI to have broader spectra of size. The main reason for the mentioned method is to reduce the sample volume, leading to a decrease in the amount of required NaI, which is an expensive material.57,133,157 In terms of the recovery rate of MPs using different salt solutions, saturated NaCl shows the lowest recovery rate (75.5%). To evaluate the effect of different density separation solutions on distinct polymers, NaCl, CaCl2, ZnCl2, and NaI have been used to separate six types of commonly used polymers (PP, PE, HDPE, PS, PVC, and PET). The obtained results revealed that lighter MPs (PP, PE, HDPE, and PS) were highly recovered with all the density separation solutions (up to 97% of recovery rate) due to their high ability to float with any of the density separation solutions. On the other hand, PVC showed the lowest recovery rate with all the mentioned solutions, varying from 28% to 62%. This is also related to the harder floatation of heavier MPs on the surface of the solutions, decreasing the recovery rate.159
Table 4 Advantages and disadvantages of different salt solutions used in density separation
Density separation solution |
Density (g cm−3) |
Advantage |
Disadvantage |
Deionized water (DI water) |
1 |
Non-toxic, highly available, able to afloat lighter MPs |
Unable to afloat high-density polymers |
NaCl |
1.2 |
Low cost, non-toxic, highly available, high recovery rate |
Unable to afloat high-density polymers such as PET and PVC |
CaCl2 |
1.4 |
Low-cost, highly available |
Unable to afloat high-density polymers, less efficiency in high organic load matrices |
Sodium metatungstate (SPT) |
1.4 |
Non-toxic, able to afloat high-density MPs |
Expensive |
NaI |
1.5–1.8 |
Separation of very high-density polymers |
Expensive, hard handling, time-consuming separation process |
ZnCl2 |
1.5–1.7 |
Toxic, separation of high-density polymers |
Harmful, corrosive, blackening filter papers |
Despite the wide applicability of density separation methods using salts, these methods suffer from deficiencies. The two most significant disadvantages are the inability to separate smaller particles (<400 μm) of plastic particles and the generation of large amounts of waste.160,161 This results are in accordance with another study's finding which showed the higher recovery rate of different types of MPs for larger particles.162 Therefore, some researchers have proposed applying oil-based solutions.163,164 These methods are based on the lipophilic characteristics of plastic compounds, which lead to the formation of a less hazardous alternative to salt-based methods.39 The application of canola oil163 and olive oil164 for density separation has been suggested in various studies, which shows a promising recovery rate (92–97%) for light and heavy MPs. Combining some drops of oil with a salt solution has also been recommended for the density separation process.165 The main advantages of using oils are their low cost and their high ability to separate light or heavy MPs. However, it has been proven that the hydrophilicity of polymeric particles may be affected through organic particle attachment to their surface, leading to the inaccuracy of separation using oils.166 Considering the different preparation (pre-treatment and extraction) steps mentioned in the previous sections, the flowchart of sample preparation is illustrated in Fig. 6.
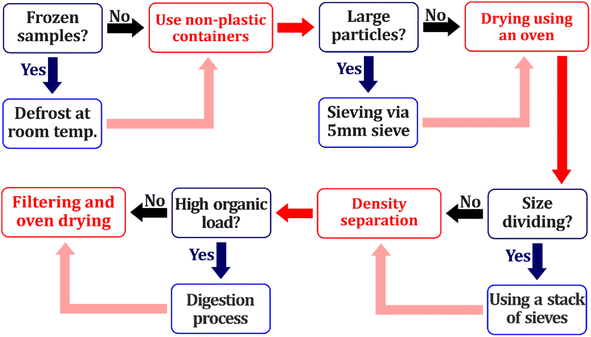 |
| Fig. 6 A flowchart showing different pre-treatment and extraction steps. | |
5. Conclusion and recommendations
In this review paper, besides sampling and preparation (pre-treatment and extraction) methods and for the first time, different preservation and storing methods were discussed. The pros and cons of various methods have been investigated. Based on the reviewed papers regarding MP studies in freshwater compartments, plastic-free materials, either glass or metal-based materials, are recommended to be utilized as container materials. In other words, using non-polymeric materials as containers decreases the risk of secondary contamination through the abrasion of plastic-made containers, leading to the overestimation and inaccuracy of MP studies. When using non-plastic materials is not accessible or feasible, using less abundant polymeric materials such as PVC to facilitate the detection of abrasive materials is recommended. In terms of sample preservation, using alcohol-based materials is suggested due to their lower toxicity and higher availability. When the long-term storage of the materials is desired, freezing and ultra-freezing temperatures are preferable since samples can be stored with minor changes in their structures. However, samples' thawing and freezing cycles, especially those with high ratios of organic loads such as tissues, should be considered to avoid the deterioration of samples. In the short-term storage of samples, on the other hand, keeping samples at refrigerator temperature (4 °C) is enough to maintain the quality of samples. Sampling, preparation and pretreatment, were other pre-analysis steps that have been mentioned in this review paper. Based on our study, different sampling and preparation methods could be applied based on the research goals, studied MPs size range, type of the matrix (either water or sediment), and sample organic and inorganic loads based on which different digestion and density separation methods should be applied.
It goes without mentioning that despite the importance of sample preservation and storage in MP studies, to the best of the authors' knowledge, none of the studies evaluate the importance of these parameters. Therefore, it is strongly advised that MPs researchers consider the impact of preservation and storage methods on their studies to make the data more reliable. The following hints are recommended to be in researchers' minds once conducting microplastic research:
• Devise parallel studies utilizing synthetic polymers to find the recovery rate of the studies while using different preservatives and containers.
• Conduct blank experiments in various stages of MP studies to reduce the impact of secondary contamination and determine the effect of different preservation and storage methods.
• Do the polymer characterization tests before and after using preservation methods to see any probable effect of preservation materials on microplastic structures. We strongly recommend devising experiments before and after applying any chemicals or heat to see if there are any changes in the polymer characteristics.
• For long-term storage, cryopreservation-freezing or ultra-freezing conditions (−20/−80 °C) are advised to suppress both biological and chemical reactions.
By considering these precautions in different pre-analysis phases of MP research – that is, sampling, preservation and storage, and preparation steps – the obtained results will be more reliable to give a more accurate picture of MP presence in freshwater environments.
Conflicts of interest
There are no conflicts to declare.
Acknowledgements
This research was funded by the Natural Sciences and Engineering Research Council (Alliance Grants Option-1, ALLRP-560377-20, Discovery Grant 23451). We would also like to thank James and Joanne Love Chair in Environmental Engineering.
References
- C. Jiang, L. Yin, Z. Li, X. Wen, X. Luo and S. Hu,
et al., Microplastic pollution in the rivers of the Tibet Plateau, Environ. Pollut., 2019, 249, 91–98 CrossRef CAS PubMed
.
- L. Lebreton and A. Andrady, Future scenarios of global plastic waste generation and disposal, Palgrave Commun., 2019, 5(1), 1–11 CrossRef
.
- C. Schmidt, R. Kumar, S. Yang and O. Büttner, Microplastic particle emission from wastewater treatment plant effluents into river networks in Germany: Loads, spatial patterns of concentrations and potential toxicity, Sci. Total Environ., 2020, 737, 139544 CrossRef CAS PubMed
.
- C. Guerranti, S. Cannas, C. Scopetani, P. Fastelli, A. Cincinelli and M. Renzi, Plastic litter in aquatic environments of Maremma Regional Park (Tyrrhenian Sea, Italy): Contribution by the Ombrone river and levels in marine sediments, Mar. Pollut. Bull., 2017, 117(1–2), 366–370 CrossRef CAS PubMed
.
-
R. Singh, R. Kumar and P. Sharma, Microplastic in the subsurface system: Extraction and characterization from sediments of River Ganga near Patna, Bihar, Advances in Remediation Techniques for Polluted Soils and Groundwater, 2022, pp. 191–217 Search PubMed
.
- P. Kasamesiri and W. Thaimuangpho, MICROPLASTICS INGESTION BY FRESHWATER FISH IN THE CHI RIVER, THAILAND, Int. J. GEOMATE, 2020, 18(67), 114–119 Search PubMed
.
- N. Von Moos, P. Burkhardt-Holm and A. Köhler, Uptake and effects of microplastics on cells and tissue of the blue mussel Mytilus edulis L. after an experimental exposure, Environ. Sci. Technol., 2012, 46(20), 11327–11335 CrossRef CAS PubMed
.
- I. E. Napper, A. Bakir, S. J. Rowland and R. C. Thompson, Characterisation, quantity and sorptive properties of microplastics extracted from cosmetics, Mar. Pollut. Bull., 2015, 99(1–2), 178–185 CrossRef CAS PubMed
.
- R. Kumar, P. Sharma, C. Manna and M. Jain, Abundance, interaction, ingestion, ecological concerns, and mitigation policies of microplastic pollution in riverine ecosystem: A review, Sci. Total Environ., 2021, 782, 146695 CrossRef CAS
.
- J. Duan, N. Bolan, Y. Li, S. Ding, T. Atugoda and M. Vithanage,
et al., Weathering of microplastics and interaction with other coexisting constituents in terrestrial and aquatic environments, Water Res., 2021, 196, 117011 CrossRef CAS PubMed
.
- C. A. Peters and S. P. Bratton, Urbanization is a major influence on microplastic ingestion by sunfish in the Brazos River Basin, Central Texas, USA, Environ. Pollut., 2016, 210, 380–387 CrossRef CAS PubMed
.
- S. Gao, Q. Wu, M. Peng, J. Zeng, T. Jiang and Y. Ruan,
et al., Rapid urbanization affects microplastic communities in lake sediments: A case study of Lake Aha in southwest China, J. Environ. Manage., 2023, 338, 117824 CrossRef CAS PubMed
.
- A. A. Horton, C. Svendsen, R. J. Williams, D. J. Spurgeon and E. Lahive, Large microplastic particles in sediments of tributaries of the River Thames, UK – Abundance, sources and methods for effective quantification, Mar. Pollut. Bull., 2017, 114(1), 218–226 CrossRef CAS PubMed
.
- P. Kay, R. Hiscoe, I. Moberley, L. Bajic and N. McKenna, Wastewater treatment plants as a source of microplastics in river catchments, Environ. Sci. Pollut. Res., 2018, 25(20), 20264–20267 CrossRef CAS PubMed
.
- P. Jan Kole, A. J. Löhr, F. Van Belleghem and A. Ragas, Wear and Tear of Tyres: A Stealthy Source of Microplastics in the Environment, Int. J. Environ. Res. Public Health, 2017, 14(10), 1265 CrossRef PubMed
.
- K. Zhang, W. Gong, J. Lv, X. Xiong and C. Wu, Accumulation of floating microplastics behind the Three Gorges Dam, Environ. Pollut., 2015, 204, 117–123 CrossRef CAS PubMed
.
- X. Cai, H. Chen, B. Huang and J. Lu, Analysis on advances and characteristics of microplastic pollution in China's lake ecosystems, Ecotoxicol. Environ. Saf., 2022, 232, 113254 CrossRef CAS PubMed
.
- P. Martínez Silva and M. Nanny, Impact of Microplastic Fibers from the Degradation of Nonwoven Synthetic Textiles to the Magdalena River Water Column and River Sediments by the City of Neiva, Huila (Colombia), Water, 2020, 12, 1210 CrossRef
.
- A. A. Shah, F. Hasan, A. Hameed and S. Ahmed, Biological degradation of plastics: A comprehensive review, Biotechnol. Adv., 2008, 26(3), 246–265 CrossRef CAS PubMed
.
- E. S. Gruber, V. Stadlbauer, V. Pichler, K. Resch-Fauster, A. Todorovic and T. C. Meisel,
et al., To Waste or Not to Waste: Questioning Potential Health Risks of Micro- and Nanoplastics with a Focus on Their Ingestion and Potential Carcinogenicity, Exposure Health, 2022, 15(1), 33–51 CrossRef PubMed
.
- F. Yu, Y. Li, G. Huang, C. Yang, C. Chen and T. Zhou,
et al., Adsorption behavior of the antibiotic levofloxacin on microplastics in the presence of different heavy metals in an aqueous solution, Chemosphere, 2020, 260, 127650 CrossRef CAS PubMed
.
- N. Naqash, S. Prakash, D. Kapoor and R. Singh, Interaction of freshwater microplastics with biota and heavy metals: a review, Environ. Chem. Lett., 2020, 18(6), 1813–1824 CrossRef CAS
.
- B. He, A. Goonetilleke, G. A. Ayoko and L. Rintoul, Abundance, distribution patterns, and identification of microplastics in Brisbane River sediments, Australia, Sci. Total Environ., 2020, 700, 134467 CrossRef CAS PubMed
.
- Y. Liu, J. D. Zhang, C. Y. Cai, Y. He, L. Y. Chen and X. Xiong,
et al., Occurrence and characteristics of microplastics in the Haihe River: An investigation of a seagoing river flowing through a megacity in northern China, Environ. Pollut., 2020, 262, 114261 CrossRef CAS PubMed
.
- S. L. Wright, R. C. Thompson and T. S. Galloway, The physical impacts of microplastics on marine organisms: A review, Environ. Pollut., 2013, 178, 483–492 CrossRef CAS PubMed
.
- Y. Wang, B. Zhou, H. Chen, R. Yuan and F. Wang, Distribution, biological effects and biofilms of microplastics in freshwater systems – A review, Chemosphere, 2022, 299, 134370 CrossRef CAS PubMed
.
- Y. Meng, F. J. Kelly and S. L. Wright, Advances and challenges of microplastic pollution in freshwater ecosystems: A UK perspective, Environ. Pollut., 2020, 256, 113445 CrossRef CAS PubMed
.
- L. C. M. Lebreton, J. Van Der Zwet, J. W. Damsteeg, B. Slat, A. Andrady and J. Reisser, River plastic emissions to the world's oceans, Nat. Commun., 2017, 8(1), 1–10 CrossRef PubMed
.
- A. Tursi, M. Baratta, T. Easton, E. Chatzisymeon, F. Chidichimo and M. De Biase,
et al., Microplastics in aquatic systems, a comprehensive review: origination, accumulation, impact, and removal technologies, RSC Adv., 2022, 12(44), 28318–28340 RSC
.
- A. Shamskhany, Z. Li, P. Patel and S. Karimpour, Evidence of Microplastic Size Impact on Mobility and Transport in the Marine Environment: A Review and Synthesis of Recent Research, Front. Mar. Sci., 2021, 8, 760649 CrossRef
.
- D. Kaiser, N. Kowalski and J. J. Waniek, Effects of biofouling on the sinking behavior of microplastics, Environ. Res. Lett., 2017, 12(12), 124003 CrossRef
.
- T. J. Hoellein, A. J. Shogren and J. L. Tank, Microplastic deposition velocity in streams follows patterns for naturally occurring allochthonous particles, Sci. Rep., 2019, 9, 3740 CrossRef PubMed
.
- A. E. Schwarz, T. N. Ligthart, E. Boukris and T. van Harmelen, Sources, transport, and accumulation of different types of plastic litter in aquatic environments: A review study, Mar. Pollut. Bull., 2019, 143, 92–100 CrossRef CAS PubMed
.
- J. Gerritse, H. A. Leslie, C. A. de Tender, L. I. Devriese and A. D. Vethaak, Fragmentation of plastic objects in a laboratory seawater microcosm, Sci. Rep., 2020, 10(1), 1–16 CrossRef PubMed
.
- S. Estahbanati and N. L. Fahrenfeld, Influence of wastewater treatment plant discharges on microplastic concentrations in surface water, Chemosphere, 2016, 162, 277–284 CrossRef CAS PubMed
.
- W. Luo, L. Su, N. J. Craig, F. Du, C. Wu and H. Shi, Comparison of microplastic pollution in different water bodies from urban creeks to coastal waters, Environ. Pollut., 2019, 246, 174–182 CrossRef CAS PubMed
.
- F. Mendrik, R. Fernández, C. R. Hackney, C. Waller and D. R. Parsons, Non-buoyant microplastic settling velocity varies with biofilm growth and ambient water salinity, Commun. Earth Environ., 2023, 4(1), 1–9 CrossRef
.
- M. N. Issac and B. Kandasubramanian, Effect of microplastics in water and aquatic systems, Environ. Sci. Pollut. Res., 2021, 28(16), 19544–19562 CrossRef CAS PubMed
.
- L. Yang, Y. Zhang, S. Kang, Z. Wang and C. Wu, Microplastics in freshwater sediment: A review on methods, occurrence, and sources, Sci. Total Environ., 2021, 754, 141948 CrossRef CAS PubMed
.
- V. Hidalgo-Ruz, L. Gutow, R. C. Thompson and M. Thiel, Microplastics in the marine environment: A review of the methods used for identification and quantification, Environ. Sci. Technol., 2012, 46(6), 3060–3075 CrossRef CAS PubMed
.
- W. Wang and J. Wang, Investigation of microplastics in aquatic environments: An overview of the methods used, from field sampling to laboratory analysis, TrAC, Trends Anal. Chem., 2018, 108, 195–202 CrossRef CAS
.
- C. B. Crawford and B. Quinn, Microplastic Pollutants, Microplast. Pollut., 2016, 1–315 Search PubMed
.
- G. Fytianos, E. Ioannidou, A. Thysiadou, A. C. Mitropoulos and G. Z. Kyzas, Microplastics in Mediterranean Coastal Countries: A Recent Overview, J. Mar. Sci. Eng., 2021, 9(1), 98 CrossRef
.
- Z. Harrold, M. M. Arienzo, M. Collins, J. M. Davidson, X. Bai and S. Sukumaran,
et al., A Peristaltic Pump and Filter-Based Method for Aqueous Microplastic Sampling and Analysis, ACS ES&T Water, 2022, 2(2), 268–277 Search PubMed
.
- C. M. Rochman, F. Regan and R. C. Thompson, On the harmonization of methods for measuring the occurrence, fate and effects of microplastics, Anal. Methods, 2017, 9(9), 1324–1325 RSC
.
- E. Miller, M. Sedlak, D. Lin, C. Box, C. Holleman and C. M. Rochman,
et al., Recommended best practices for collecting, analyzing, and reporting microplastics in environmental media: Lessons learned from comprehensive monitoring of San Francisco Bay, J. Hazard. Mater., 2021, 409, 124770 CrossRef CAS PubMed
.
-
J. Frias, E. Pagter, R. Nash, I. O'Connor, O. Carretero, A. Filgueiras, et al., Standardised protocol for monitoring microplastics in sediments, Deliverable 4.2, 2018 Search PubMed
.
- S. Uddin, S. W. Fowler, M. F. Uddin, M. Behbehani and A. Naji, A review of microplastic distribution in sediment profiles, Mar. Pollut. Bull., 2021, 163, 111973 CrossRef CAS PubMed
.
- L. Miao, P. Wang, J. Hou, Y. Yao, Z. Liu and S. Liu,
et al., Distinct community structure and microbial functions of biofilms colonizing microplastics, Sci. Total Environ., 2019, 650, 2395–2402 CrossRef CAS PubMed
.
- J. Dusaucy, D. Gateuille, Y. Perrette and E. Naffrechoux, Microplastic pollution of worldwide lakes, Environ. Pollut., 2021, 284, 117075 CrossRef CAS PubMed
.
- Z. Zhou, P. Zhang, G. Zhang, S. Wang, Y. Cai and H. Wang, Vertical microplastic distribution in sediments of Fuhe River estuary to Baiyangdian Wetland in Northern China, Chemosphere, 2021, 280, 130800 CrossRef CAS PubMed
.
- Y. Li, X. Wang, W. Fu, X. Xia, C. Liu and J. Min,
et al., Interactions between nano/micro plastics and suspended sediment in water: Implications on aggregation and settling, Water Res., 2019, 161, 486–495 CrossRef CAS PubMed
.
- P. L. Corcoran, T. Norris, T. Ceccanese, M. J. Walzak, P. A. Helm and C. H. Marvin, Hidden plastics of Lake Ontario, Canada and their potential preservation in the sediment record, Environ. Pollut., 2015, 204, 17–25 CrossRef CAS PubMed
.
- S. Abidli, H. Toumi, Y. Lahbib and N. Trigui El Menif, The First Evaluation of Microplastics in Sediments from the Complex Lagoon-Channel of Bizerte (Northern Tunisia), Water, Air, Soil Pollut., 2017, 228(7), 1–10 CrossRef CAS
.
- F. C. Alam, E. Sembiring, B. S. Muntalif and V. Suendo, Microplastic distribution in surface water and sediment river around slum and industrial area (case study: Ciwalengke River, Majalaya district, Indonesia), Chemosphere, 2019, 224, 637–645 CrossRef CAS PubMed
.
- Y. Adomat and T. Grischek, Sampling and processing methods of microplastics in river sediments – A review, Sci. Total Environ., 2021, 758, 143691 CrossRef CAS PubMed
.
- K. Zhang, A. H. Hamidian, A. Tubić, Y. Zhang, J. K. H. Fang and C. Wu,
et al., Understanding plastic degradation and microplastic formation in the environment: A review, Environ. Pollut., 2021, 274, 116554 CrossRef CAS PubMed
.
- A. Bruge, M. Dhamelincourt, L. Lanceleur, M. Monperrus, J. Gasperi and B. Tassin, A first estimation of uncertainties related to microplastic sampling in rivers, Sci. Total Environ., 2020, 718, 137319 CrossRef CAS PubMed
.
- P. Lestari, Y. Trihadiningrum, B. A. Wijaya, K. A. Yunus and M. Firdaus, Distribution of microplastics in Surabaya River, Indonesia, Sci. Total Environ., 2020, 726, 138560 CrossRef CAS PubMed
.
- R. Mao, J. Song, P. Yan, Z. Ouyang, R. Wu and S. Liu,
et al., Horizontal and vertical distribution of microplastics in the Wuliangsuhai Lake sediment, northern China, Sci. Total Environ., 2021, 754, 142426 CrossRef CAS PubMed
.
- L. Niu, Y. Li, Y. Li, Q. Hu, C. Wang and J. Hu,
et al., New insights into the vertical distribution and microbial degradation of microplastics in urban river sediments, Water Res., 2021, 188, 116449 CrossRef CAS PubMed
.
- S. M. Brander, V. C. Renick, M. M. Foley, C. Steele, M. Woo and A. Lusher,
et al., Sampling and Quality Assurance and Quality Control: A Guide for Scientists Investigating the Occurrence of Microplastics Across Matrices, Appl. Spectrosc., 2020, 74(9), 1099–1125 CrossRef CAS PubMed
.
- M. E. L. Felismino, P. A. Helm and C. M. Rochman, Microplastic and other anthropogenic microparticles in water and sediments of Lake Simcoe, J. Great Lakes Res., 2021, 47(1), 180–189 CrossRef CAS
.
- A. Ballent, P. L. Corcoran, O. Madden, P. A. Helm and F. J. Longstaffe, Sources and sinks of microplastics in Canadian Lake Ontario nearshore, tributary and beach sediments, Mar. Pollut. Bull., 2016, 110(1), 383–395 CrossRef CAS PubMed
.
- I. E. Napper, A. Baroth, A. C. Barrett, S. Bhola, G. W. Chowdhury and B. F. R. Davies,
et al., The abundance and characteristics of microplastics in surface water in the transboundary Ganges River, Environ. Pollut., 2021, 274, 116348 CrossRef CAS PubMed
.
- Z. Zhang, C. Deng, L. Dong, L. Liu, H. Li and J. Wu,
et al., Microplastic pollution in the Yangtze River Basin: Heterogeneity of abundances and characteristics in different environments, Environ. Pollut., 2021, 287, 117580 CrossRef CAS PubMed
.
- G. Wong, L. Löwemark and A. Kunz, Microplastic pollution of the Tamsui River and its tributaries in northern Taiwan: Spatial heterogeneity and correlation with precipitation, Environ. Pollut., 2020, 260, 113935 CrossRef CAS PubMed
.
- A. Crew, I. Gregory-Eaves and A. Ricciardi, Distribution, abundance, and diversity of microplastics in the upper St. Lawrence River, Environ. Pollut., 2020, 260, 113994 CrossRef CAS PubMed
.
- Z. Sobhani, Y. Lei, Y. Tang, L. Wu, X. Zhang and R. Naidu,
et al., Microplastics generated when opening plastic packaging, Sci. Rep., 2020, 10(1), 1–7 CrossRef PubMed
.
- D. Schymanski, C. Goldbeck, H. U. Humpf and P. Fürst, Analysis of microplastics in water by micro-Raman spectroscopy: Release of plastic particles from different packaging into mineral water, Water Res., 2018, 129, 154–162 CrossRef CAS PubMed
.
- B. E. Oßmann, G. Sarau, H. Holtmannspötter, M. Pischetsrieder, S. H. Christiansen and W. Dicke, Small-sized microplastics and pigmented particles in bottled mineral water, Water Res., 2018, 141, 307–316 CrossRef PubMed
.
-
M. G. J. Löder and G. Gerdts, Methodology used for the detection and identification of microplastics—a critical appraisal, Marine Anthropogenic Litter, 2015, pp. 201–227 Search PubMed
.
- J. Gong, T. Kong, Y. Li, Q. Li, Z. Li and J. Zhang, Biodegradation of Microplastic Derived from Poly(ethylene terephthalate) with Bacterial Whole-Cell Biocatalysts, Polymers, 2018, 10(12), 1326 CrossRef PubMed
.
- A. R. Othman, H. A. Hasan, M. H. Muhamad, N. ’I. Ismail and S. R. S. Abdullah, Microbial degradation of microplastics by enzymatic processes: a review, Environ. Chem. Lett., 2021, 19(4), 3057–3073 CrossRef CAS
.
- H. S. Zurier and J. M. Goddard, Biodegradation of microplastics in food and agriculture, Curr. Opin. Food Sci., 2021, 37, 37–44 CrossRef CAS
.
- S. Miri, R. Saini, S. M. Davoodi, R. Pulicharla, S. K. Brar and S. Magdouli, Biodegradation of microplastics: Better late than never, Chemosphere, 2022, 286, 131670 CrossRef CAS PubMed
.
- S. M. Satti, A. A. Shah, T. L. Marsh and R. Auras, Biodegradation of Poly(lactic acid) in Soil Microcosms at Ambient Temperature: Evaluation of Natural Attenuation, Bio-augmentation and Bio-stimulation, J. Polym. Environ., 2018, 26(9), 3848–3857 CrossRef CAS
.
- F. Kawai, T. Kawabata and M. Oda, Current knowledge on enzymatic PET degradation and its possible application to waste stream management and other fields, Appl. Microbiol. Biotechnol., 2019, 103(11), 4253–4268 CrossRef CAS PubMed
.
-
C. S. Wong and S. Coffin, Standard Operating Procedures for Extraction and Measurement by Infrared Spectroscopy of Microplastic Particles in Drinking Water, 2021 Search PubMed
.
-
POW Sludge Sampling and Analysis Guidance Document, 1989 Search PubMed.
- A. Rain-Franco, G. P. de Moraes and S. Beier, Cryopreservation and Resuscitation of Natural Aquatic Prokaryotic Communities, Front. Microbiol., 2021, 11, 597653 CrossRef PubMed
.
- V. Gambarini, O. Pantos, J. M. Kingsbury, L. Weaver, K. M. Handley and G. Lear, Phylogenetic Distribution of Plastic-Degrading Microorganisms, mSystems, 2021, 6(1), e01112 CrossRef CAS PubMed
.
- S. Sun, H. Sui, L. Xu, J. Zhang, D. Wang and Z. Zhou, Effect of freeze–thaw cycle aging and high-temperature oxidation aging on the sorption of atrazine by microplastics, Environ. Pollut., 2022, 307, 119434 CrossRef CAS PubMed
.
- W. G. Barb and W. Mikucki, On the coagulation of polymer latices by freezing and thawing, J. Polym. Sci., 1959, 37(132), 499–514 CrossRef CAS
.
- O. S. Alimi, J. Farner Budarz, L. M. Hernandez and N. Tufenkji, Microplastics and Nanoplastics in Aquatic Environments: Aggregation, Deposition, and Enhanced Contaminant Transport, Environ. Sci. Technol., 2018, 52(4), 1704–1724 CrossRef CAS PubMed
.
- J. Qian, C. Mo, H. Yang, J. Zhang, S. Zhu and F. Gong,
et al., Preparation of myricetin nanoliposomes using film-ultrasonic dispersion method and characterization, Appl. Nanosci., 2022, 13(5), 3263–3272 CrossRef
.
- P. Campo, A. Holmes and F. Coulon, A method for the characterisation of microplastics in sludge, MethodsX, 2019, 6, 2776–2781 CrossRef PubMed
.
- A. K. Baldwin, A. R. Spanjer, M. R. Rosen and T. Thom, Microplastics in Lake Mead National Recreation Area, USA: Occurrence and biological uptake, PLoS One, 2020, 15(5), e0228896 CrossRef CAS PubMed
.
-
S. Warrack, J. K. Challis, M. L. Hanson and M. D. Rennie, Microplastics Flowing into Lake Winnipeg: Densities, Sources, Flux, and Fish Exposures, Proceedings of Manitoba’s Undergraduate Science and Engineering Research, 2017, vol. 3 Search PubMed
.
- K. H. Rowley, A. C. Cucknell, B. D. Smith, P. F. Clark and D. Morritt, London's river of plastic: High levels of microplastics in the Thames water column, Sci. Total Environ., 2020, 740, 140018 CrossRef CAS PubMed
.
- M. B. Alfonso, F. Scordo, C. Seitz, G. M. Mavo Manstretta, A. C. Ronda and A. H. Arias,
et al., First evidence of microplastics in nine lakes across Patagonia (South America), Sci. Total Environ., 2020, 733, 139385 CrossRef CAS PubMed
.
- I. Sekudewicz, A. M. Dąbrowska and M. D. Syczewski, Microplastic pollution in surface water and sediments in the urban section of the Vistula River (Poland), Sci. Total Environ., 2021, 762, 143111 CrossRef CAS PubMed
.
-
W. Woishnis and S. Ebnesajjad, Chemical Resistance of Thermoplastics, Chemical Resistance of Thermoplastics, 2012 Search PubMed
.
- I. T. S. Heikkinen, C. Kauppinen, Z. Liu, S. M. Asikainen, S. Spoljaric and J. V. Seppälä,
et al., Chemical compatibility of fused filament fabrication-based 3-D printed components with solutions commonly used in semiconductor wet processing, Addit. Manuf., 2018, 23, 99–107 CAS
.
- E. Skidmore, Chemical compatibility of polymers, J. Fail. Anal. Prev., 2011, 11(4), 393–397 CrossRef
.
-
L. Bartolome, M. Imran, B. G. Cho, W. A. Al-Masry, D. H. Kim, L. Bartolome, et al., Recent Developments in the Chemical Recycling of PET, Material Recycling – Trends and Perspectives, 2012 Search PubMed
.
- L. Kárpáti, F. Fogarassy, D. Kovácsik and V. Vargha, One-Pot Depolymerization and Polycondensation of PET Based Random Oligo- and Polyesters, J. Polym. Environ., 2019, 27(10), 2167–2181 CrossRef
.
- P. Lozano-Martinez, T. Torres-Zapata and N. Martin-Sanchez, Directing Depolymerization of PET with Subcritical and Supercritical Ethanol to Different Monomers through Changes in Operation Conditions, ACS Sustainable Chem. Eng., 2021, 9(29), 9846–9853 CrossRef CAS
.
- T. Kameda, M. Ono, G. Grause, T. Mizoguchi and T. Yoshioka, Chemical modification of poly(vinyl chloride) by nucleophilic substitution, Polym. Degrad. Stab., 2009, 94(1), 107–112 CrossRef CAS
.
- L. Su, Y. Xue, L. Li, D. Yang, P. Kolandhasamy and D. Li,
et al., Microplastics in Taihu Lake, China, Environ. Pollut., 2016, 216, 711–719 CrossRef CAS PubMed
.
- A. Kaliszewicz, M. Winczek, K. Karaban, D. Kurzydłowski, M. Górska and W. Koselak,
et al., The contamination of inland waters by microplastic fibres under different anthropogenic pressure: Preliminary study in Central Europe (Poland), Waste Manage. Res., 2020, 38(11), 1231–1238 CrossRef CAS PubMed
.
- F. K. Migwi, J. A. Ogunah and J. M. Kiratu, Occurrence and Spatial Distribution of Microplastics in the Surface Waters of Lake Naivasha, Kenya, Environ. Toxicol. Chem., 2020, 39(4), 765–774 CrossRef CAS PubMed
.
- M. Srinivasan, D. Sedmak and S. Jewell, Effect of Fixatives and Tissue Processing on the Content and Integrity of Nucleic Acids, Am. J. Pathol., 2002, 161(6), 1961–1971 CrossRef CAS PubMed
.
- A. Binelli, L. Pietrelli, S. Di Vito, L. Coscia, M. Sighicelli and C. D. Torre,
et al., Hazard evaluation of plastic mixtures from four Italian subalpine great lakes on the basis of laboratory exposures of zebra mussels, Sci. Total Environ., 2020, 699, 134366 CrossRef CAS PubMed
.
- P. Pastorino, E. Pizzul, M. Bertoli, S. Anselmi, M. Kušće and V. Menconi,
et al., First insights
into plastic and microplastic occurrence in biotic and abiotic compartments, and snow from a high-mountain lake (Carnic Alps), Chemosphere, 2021, 265, 129121 CrossRef CAS PubMed
.
- C. Bertoldi, L. Z. Lara, F. A. de L. Mizushima, F. C. G. Martins, M. A. Battisti and R. Hinrichs,
et al., First evidence of microplastic contamination in the freshwater of Lake Guaíba, Porto Alegre, Brazil, Sci. Total Environ., 2021, 759, 143503 CrossRef CAS PubMed
.
- T. Irfan, S. Khalid, M. Taneez and M. Z. Hashmi, Plastic driven pollution in Pakistan: the first evidence of environmental exposure to microplastic in sediments and water of Rawal Lake, Environ. Sci. Pollut. Res., 2020, 27(13), 15083–15092 CrossRef CAS PubMed
.
- M. B. Alfonso, A. H. Arias and M. C. Piccolo, Microplastics integrating the zooplanktonic fraction in a saline lake of Argentina: influence of water management, Environ. Monit. Assess., 2020, 192(2), 1–10 CrossRef PubMed
.
- A. de J. N. Velasco, L. Rard, W. Blois, D. Lebrun, F. Lebrun and F. Pothe,
et al., Microplastic and Fibre Contamination in a Remote Mountain Lake in Switzerland, Water, 2020, 12(9), 2410 CrossRef CAS
.
- K. Gopinath, S. Seshachalam, K. Neelavannan, V. Anburaj, M. Rachel and S. Ravi,
et al., Quantification of microplastic in Red Hills Lake of Chennai city, Tamil Nadu, India, Environ. Sci. Pollut. Res., 2020, 27(26), 33297–33306 CrossRef CAS PubMed
.
- B. A. Oni, A. O. Ayeni, O. Agboola, T. Oguntade and O. Obanla, Comparing microplastics contaminants in (dry and raining) seasons for Ox- Bow Lake in Yenagoa, Nigeria, Ecotoxicol. Environ. Saf., 2020, 198, 110656 CrossRef CAS PubMed
.
- W. Xia, Q. Rao, X. Deng, J. Chen and P. Xie, Rainfall is a significant environmental factor of microplastic pollution in inland waters, Sci. Total Environ., 2020, 732, 139065 CrossRef CAS PubMed
.
- C. Campanale, F. Stock, C. Massarelli, C. Kochleus, G. Bagnuolo and G. Reifferscheid,
et al., Microplastics and their possible sources: The example of Ofanto river in southeast Italy, Environ. Pollut., 2020, 258, 113284 CrossRef CAS PubMed
.
- R. Dris, J. Gasperi, V. Rocher and B. Tassin, Synthetic and non-synthetic anthropogenic fibers in a river under the impact of Paris Megacity: Sampling methodological aspects and flux estimations, Sci. Total Environ., 2018, 618, 157–164 CrossRef CAS PubMed
.
- P. L. Lenaker, A. K. Baldwin, S. R. Corsi, S. A. Mason, P. C. Reneau and J. W. Scott, Vertical Distribution of Microplastics in the Water Column and Surficial Sediment from the Milwaukee River Basin to Lake Michigan, Environ. Sci. Technol., 2019, 53(21), 12227–12237 CrossRef CAS PubMed
.
- A. R. Mccormick, T. J. Hoellein, M. G. London, J. Hittie, J. W. Scott and J. J. Kelly,
et al., Microplastic in surface waters of urban rivers: concentration, sources, and associated bacterial assemblages, Ecosphere, 2016, 7(11), e01556 CrossRef
.
- T. Bujaczek, S. Kolter, D. Locky and M. S. Ross, Characterization of microplastics and anthropogenic fibers in surface waters of the North Saskatchewan River, Alberta, Canada, Facets, 2021, 6(1), 26–43 CrossRef
.
- N. Singh, A. Mondal, A. Bagri, E. Tiwari, N. Khandelwal and F. A. Monikh,
et al., Characteristics and spatial distribution of microplastics in the lower Ganga River water and sediment, Mar. Pollut. Bull., 2021, 163, 111960 CrossRef CAS PubMed
.
- E. Sembiring, A. A. Fareza, V. Suendo and M. Reza, The Presence of Microplastics in Water, Sediment, and Milkfish (Chanos chanos) at the Downstream Area of Citarum River, Indonesia, Water, Air, Soil Pollut., 2020, 231(7), 1–14 CrossRef
.
- J. M. Donoso and B. Rios-Touma, Microplastics in tropical Andean rivers: A perspective from a highly populated Ecuadorian basin without wastewater treatment, Heliyon, 2020, 6(7), e04302 CrossRef PubMed
.
- A. E. Valine, A. E. Peterson, D. A. Horn, K. M. Scully-Engelmeyer and E. F. Granek, Microplastic Prevalence in 4 Oregon Rivers Along a Rural to Urban Gradient Applying a Cost-Effective Validation Technique, Environ. Toxicol. Chem., 2020, 39(8), 1590–1598 CrossRef CAS PubMed
.
- M. Constant, W. Ludwig, P. Kerhervé, J. Sola, B. Charrière and A. Sanchez-Vidal,
et al., Microplastic fluxes in a large and a small Mediterranean river catchments: The Têt and the Rhône, Northwestern Mediterranean Sea, Sci. Total Environ., 2020, 716, 136984 CrossRef CAS PubMed
.
- A. K. Baldwin, S. R. Corsi and S. A. Mason, Plastic Debris in 29 Great Lakes Tributaries: Relations to Watershed Attributes and Hydrology, Environ. Sci. Technol., 2016, 50(19), 10377–10385 CrossRef CAS PubMed
.
- J. C. Vermaire, C. Pomeroy, S. M. Herczegh, O. Haggart and M. Murphy, Microplastic abundance and distribution in the open water and sediment of the Ottawa River, Canada, and its tributaries, Facets, 2017, 2(1), 301–314, DOI:10.1139/facets-2016-0070
.
- S. A. Forrest, L. Holman, M. Murphy and J. C. Vermaire, Citizen science sampling programs as a technique for monitoring microplastic pollution: results, lessons learned and recommendations for working with volunteers for monitoring plastic pollution in freshwater ecosystems, Environ. Monit. Assess., 2019, 191(3), 1–10 CrossRef PubMed
.
- K. Amrutha and A. K. Warrier, The first report on the source-to-sink characterization of microplastic pollution from a riverine environment in tropical India, Sci. Total Environ., 2020, 739, 140377 CrossRef CAS PubMed
.
- C. J. Tien, Z. X. Wang and C. S. Chen, Microplastics in water, sediment and fish from the Fengshan River system: Relationship to aquatic factors and accumulation of polycyclic aromatic hydrocarbons by fish, Environ. Pollut., 2020, 265, 114962 CrossRef CAS PubMed
.
- T. Stanton, M. Johnson, P. Nathanail, W. MacNaughtan and R. L. Gomes, Freshwater microplastic concentrations vary through both space and time, Environ. Pollut., 2020, 263, 114481 CrossRef CAS PubMed
.
- M. Han, X. Niu, M. Tang, B. T. Zhang, G. Wang and W. Yue,
et al., Distribution of microplastics in surface water of the lower Yellow River near estuary, Sci. Total Environ., 2020, 707, 135601 CrossRef CAS PubMed
.
- P. Wu, Y. Tang, M. Dang, S. Wang, H. Jin and Y. Liu,
et al., Spatial-temporal distribution of microplastics in surface water and sediments of Maozhou River within Guangdong-Hong Kong-Macao Greater Bay Area, Sci. Total Environ., 2020, 717, 135187 CrossRef CAS PubMed
.
- X. Zhang, Y. Leng, X. Liu, K. Huang and J. Wang, Microplastics' Pollution and Risk Assessment in an Urban River: A Case Study in the Yongjiang River, Nanning City, South China, Exposure Health, 2020, 12(2), 141–151 CrossRef CAS
.
- L. Zhang, J. Liu, Y. Xie, S. Zhong, B. Yang and D. Lu,
et al., Distribution of microplastics in surface water and sediments of Qin river in Beibu Gulf, China, Sci. Total Environ., 2020, 708, 135176 CrossRef CAS PubMed
.
- M. Di and J. Wang, Microplastics in surface waters and sediments of the Three Gorges Reservoir, China, Sci. Total Environ., 2018, 616–617, 1620–1627 CrossRef CAS PubMed
.
- L. Li, S. Geng, C. Wu, K. Song, F. Sun and C. Visvanathan,
et al., Microplastics contamination in different trophic state lakes along the middle and lower reaches of Yangtze River Basin, Environ. Pollut., 2019, 254, 112951 CrossRef CAS PubMed
.
- R. Egessa, A. Nankabirwa, R. Basooma and R. Nabwire, Occurrence, distribution and size relationships of plastic debris along shores and sediment of northern Lake Victoria, Environ. Pollut., 2020, 257, 113442 CrossRef CAS PubMed
.
- S. Sruthy and E. V. Ramasamy, Microplastic pollution in Vembanad Lake, Kerala, India: The first report of microplastics in lake and estuarine sediments in India, Environ. Pollut., 2017, 222, 315–322 CrossRef CAS PubMed
.
- Y. Li, Z. Lu, H. Zheng, J. Wang and C. Chen, Microplastics in surface water and sediments of Chongming Island in the Yangtze Estuary, China, Environ. Sci. Eur., 2020, 32(1), 1–12 CrossRef
.
- M. Refat Jahan Rakib, M. Belal Hossain, R. Kumar, M. Akram Ullah, S. Al Nahian and N. Naher Rima, Spatial distribution and risk assessments due to the microplastics pollution in sediments of Karnaphuli River Estuary, Bangladesh, Sci. Rep., 2022, 12, 8581 CrossRef PubMed
.
- X. Xiong, C. Wu, J. J. Elser, Z. Mei and Y. Hao, Occurrence and fate of microplastic debris in middle and lower reaches of the Yangtze River – From inland to the sea, Sci. Total Environ., 2019, 659, 66–73 CrossRef CAS PubMed
.
- N. N. Phuong, V. Fauvelle, C. Grenz, M. Ourgaud, N. Schmidt and E. Strady,
et al., Highlights from a review of microplastics in marine sediments, Sci. Total Environ., 2021, 777, 146225 CrossRef CAS
.
- J. C. Prata, V. Reis, J. P. da Costa, C. Mouneyrac, A. C. Duarte and T. Rocha-Santos, Contamination issues as a challenge in quality control and quality assurance in microplastics analytics, J. Hazard. Mater., 2021, 403, 123660 CrossRef CAS PubMed
.
- A. P. W. Barrows, C. A. Neumann, M. L. Berger and S. D. Shaw, Grab vs. neuston tow net: a microplastic sampling performance comparison and possible advances in the field, Anal. Methods, 2017, 9(9), 1446–1453 RSC
.
- T. M. Karlsson, A. Kärrman, A. Rotander and M. Hassellöv, Comparison between manta trawl and in situ pump filtration methods, and guidance for visual identification of microplastics in surface waters, Environ. Sci. Pollut. Res., 2020, 27(5), 5559–5571 CrossRef PubMed
.
- J. Lee and K. J. Chae, A systematic protocol of microplastics analysis from their identification to quantification in water environment: A comprehensive review, J. Hazard. Mater., 2021, 403, 124049 CrossRef CAS PubMed
.
- R. Vaughan, S. D. Turner and N. L. Rose, Microplastics in the sediments of a UK urban lake, Environ. Pollut., 2017, 229, 10–18 CrossRef CAS PubMed
.
- F. Stock, C. Kochleus, B. Bänsch-Baltruschat, N. Brennholt and G. Reifferscheid, Sampling techniques and preparation methods for microplastic analyses in the aquatic environment – A review, TrAC, Trends Anal. Chem., 2019, 113, 84–92 CrossRef CAS
.
- G. Peng, P. Xu, B. Zhu, M. Bai and D. Li, Microplastics in freshwater river sediments in Shanghai, China: A case study of risk assessment in mega-cities, Environ. Pollut., 2018, 234, 448–456 CrossRef CAS PubMed
.
- L. Hu, M. Chernick, D. E. Hinton and H. Shi, Microplastics in Small Waterbodies and Tadpoles from Yangtze River Delta, China, Environ. Sci. Technol., 2018, 52(15), 8885–8893 CrossRef CAS PubMed
.
- J. D. O’Connor, A. Marie Mahon, A. F. R. M. Ramsperger, B. Trotter, P. E. Redondo-Hasselerharm and A. A. Koelmans,
et al., Microplastics in Freshwater Biota: A Critical Review of Isolation, Characterization, and Assessment Methods, Global Challenges, 2020, 4(6), 1800118 CrossRef PubMed
.
- P. Kumar, Y. Inamura, P. N. Bao, A. Abeynayaka, R. Dasgupta and H. D. L. Abeynayaka, Microplastics in Freshwater Environment in Asia: A Systematic Scientific Review, Water, 2022, 14(11), 1737 CrossRef CAS
.
- I. Schrank, J. N. Möller, H. K. Imhof, O. Hauenstein, F. Zielke and S. Agarwal,
et al., Microplastic sample purification methods – Assessing detrimental effects of purification procedures on specific plastic types, Sci. Total Environ., 2022, 833, 154824 CrossRef CAS PubMed
.
- V. C. Shruti, M. P. Jonathan, P. F. Rodriguez-Espinosa and F. Rodríguez-González, Microplastics in freshwater sediments of Atoyac River basin, Puebla City, Mexico, Sci. Total Environ., 2019, 654, 154–163 CrossRef CAS PubMed
.
-
J. Masura, J. Baker, G. Foster and C. Arthur, Laboratory Methods for the Analysis of Microplastics in the Marine Environment: Recommendations for Quantifying Synthetic Particles in Waters and Sediments, 2015 Search PubMed
.
- S. S. Monteiro, T. Rocha-Santos, J. C. Prata, A. C. Duarte, A. V. Girão and P. Lopes,
et al., A straightforward method for microplastic extraction from organic-rich freshwater samples, Sci. Total Environ., 2022, 815, 152941 CrossRef CAS PubMed
.
- W. Wang, A. W. Ndungu, Z. Li and J. Wang, Microplastics pollution in inland freshwaters of China: A case study in urban surface waters of Wuhan, China, Sci. Total Environ., 2017, 575, 1369–1374 CrossRef CAS PubMed
.
- N. Peez, J. Becker, S. M. Ehlers, M. Fritz, C. B. Fischer and J. H. E. Koop,
et al., Quantitative analysis of PET microplastics in environmental model samples using quantitative 1H-NMR spectroscopy: validation of an optimized and consistent sample clean-up method, Anal. Bioanal. Chem., 2019, 411(28), 7409–7418 CrossRef CAS PubMed
.
- M. Di, X. Liu, W. Wang and J. Wang, Manuscript prepared for submission to environmental toxicology and pharmacology pollution in drinking water source areas: Microplastics in the Danjiangkou Reservoir, China, Environ. Toxicol. Pharmacol., 2019, 65, 82–89 CrossRef CAS PubMed
.
- K. Mattsson, E. Ekstrand, M. Granberg, M. Hassellöv and K. Magnusson, Comparison of pre-treatment methods and heavy density liquids to optimize microplastic extraction from natural marine sediments, Sci. Rep., 2022, 12(1), 1–9 CrossRef PubMed
.
- T. T. Duong, P. T. Le, T. N. H. Nguyen, T. Q. Hoang, H. M. Ngo and T. O. Doan,
et al., Selection of a density separation solution to study microplastics in tropical riverine sediment, Environ. Monit. Assess., 2022, 194(2), 1–17 CrossRef PubMed
.
- B. Nguyen, D. Claveau-Mallet, L. M. Hernandez, E. G. Xu, J. M. Farner and N. Tufenkji, Separation and Analysis of Microplastics and Nanoplastics in Complex Environmental Samples, Acc. Chem. Res., 2019, 52(4), 858–866 CrossRef CAS PubMed
.
- B. Quinn, F. Murphy and C. Ewins, Validation of density separation for the rapid recovery of microplastics from sediment, Anal. Methods, 2017, 9(9), 1491–1498 RSC
.
- M. A. Cashman, K. T. Ho, T. B. Boving, S. Russo, S. Robinson and R. M. Burgess, Comparison of microplastic isolation and extraction procedures from marine sediments, Mar. Pollut. Bull., 2020, 159, 111507 CrossRef CAS PubMed
.
- E. M. Crichton, M. Noël, E. A. Gies and P. S. Ross, A novel, density-independent and FTIR-compatible approach for the rapid extraction of microplastics from aquatic sediments, Anal. Methods, 2017, 9(9), 1419–1428 RSC
.
- C. Scopetani, D. Chelazzi, J. Mikola, V. Leiniö, R. Heikkinen and A. Cincinelli,
et al., Olive oil-based method for the extraction, quantification and identification of microplastics in soil and compost samples, Sci. Total Environ., 2020, 733, 139338 CrossRef CAS PubMed
.
- T. M. Karlsson, A. D. Vethaak, B. C. Almroth, F. Ariese, M. van Velzen and M. Hassellöv,
et al., Screening for microplastics in sediment, water, marine invertebrates and fish: Method development and microplastic accumulation, Mar. Pollut. Bull., 2017, 122(1–2), 403–408 CrossRef CAS PubMed
.
- D. He, X. Zhang and J. Hu, Methods for separating microplastics from complex solid matrices: Comparative analysis, J. Hazard. Mater., 2021, 409, 124640 CrossRef CAS PubMed
.
|
This journal is © The Royal Society of Chemistry 2023 |