Ultrathin and flexible polyimide/Ti3C2TX MXene composite films for electromagnetic interference shielding with harsh environment tolerance†
Received
23rd August 2023
, Accepted 28th November 2023
First published on 29th November 2023
Abstract
Two-dimensional MXene materials show great potential for electromagnetic interference (EMI) shielding application because of their superior electrical conductivity. However, the susceptibility to oxidation and the mechanical brittleness of MXenes greatly limit their extensive application. Herein, an ultrathin flexible polyimide (PI)/Ti3C2TX composite film with a nacre-like lamellar structure is successfully prepared using a simple vacuum filtration and thermal imidization process. Owing to the addition of PI, the composite film exhibits excellent mechanical properties with a tensile strength of 114.9 MPa and a fracture strain of 3.0%. Moreover, the nacre-like lamellar structure is conducive to multiple internal reflections, giving rise to the absorption of electromagnetic waves. The PI/Ti3C2TX composite film exhibits an outstanding EMI shielding effectiveness of 37 dB in the X-band range with an ultrathin thickness of only 15 μm. Furthermore, PI serves as an antioxidation coating, and its EMI shielding performance is maintained even when the composite film is exposed to various harsh environments. Therefore, the resulting composite film exhibits excellent EMI shielding stability and durability, which enables the potential application of high-performance EMI shielding materials against harsh environments.
Introduction
With the development of 5G technology, a rising number of high-frequency and high-power electronic devices are widely used in different aspects of life, resulting in increasingly serious electromagnetic interference (EMI) or radiation.1,2 However, a large number of electromagnetic waves will seriously influence normal working and service life of sophisticated electronic equipment and pose a certain risk to human health.3,4 Therefore, high-performance EMI shielding materials must be developed to address this issue.5,6
Traditional metal shielding materials, such as copper, iron, and silver, have been widely used in the prevention of electromagnetic pollution due to their good electrical conductivity. However, high density, difficult processing, and non-corrosion resistance of these materials limit their application.7–11 In recent years, carbon-based nanomaterials, such as carbon black,12 carbon nanotubes,13 reduced graphene oxide,14–16 and graphene,17–20 have been regarded as excellent EMI shielding materials due to their corrosion resistance and low density.21,22 However, the difficulties in dispersion and low EMI shielding capabilities limit the extensive application of these materials.23,24 In contrast to metal and carbon materials, two-dimensional transition metal carbides and/or nitrides (MXenes) are expected to be the most promising candidate of high-performance EMI shielding materials and have received extensive attention, owing to their excellent electrical conductivity, hydrophilicity, and chemical activity.25–27 However, the inherent poor mechanical properties and high susceptibility to oxidation of MXene materials hinder their practical application.28–31
The most common method for improving the mechanical properties of MXenes is to introduce a polymer matrix to compensate for their deficiencies through the excellent mechanical properties of the polymer itself.32–40 Gogotsi et al.41 fabricated polyvinyl alcohol (PVA)/Ti3C2TX composite films with excellent mechanical properties by vacuum-assisted filtration. When the PVA content is 60%, the ultimate tensile strength and fracture strain are 91 ± 10 MPa and 4.0 ± 0.5% for the composite films, respectively, which are 4–5 times higher than those of the pure Ti3C2TX film. Xie et al.42 chose aramid nanofibers (ANFs) as the polymer matrix, and when ANF was added at 80%, the ANF/Ti3C2TX composite film had the best tensile strength of 197.4 MPa and a fracture strain of 9.8%. In addition, reasonable structural design is also beneficial to the mechanical and electromagnetic shielding performance of MXene-based electromagnetic shielding materials.43 Sun et al.33 prepared MXene@polystyrene (PS) nanocomposites with a segregated structure by electrostatic self-assembly and pressing. This structure allows the conductive filler to be isolated within the insulating polymer matrix, forming a conductive network with a low volume fraction, while enhancing the electromagnetic shielding capability. The MXene@PS nanocomposite film has an EMI shielding effectiveness (EMI SE) of 62 dB with only 1.9 vol % Ti3C2TX. Xu et al.35 reported MXene/PVA composite foam with porous structure by the freeze-drying method and rolled the foam to obtain the corresponding MXene/PVA porous composite film. The design of the porous structure produces multiple interfaces, which increases the propagation path of the electromagnetic waves and helps to attenuate them within the material. The MXene/PVA porous composite film has an EMI SE of 26 dB at a thickness of 100 μm. Zhou et al.44 prepared alternating multilayer CNF@MXene composite films by a simple alternating vacuum filtration method. The design of the alternating multilayer structure can produce large internal scattering at internal interfaces due to the presence of different impedance phases, which helps to attenuate electromagnetic waves. The CNF@MXene composite film has an ultimate tensile strength of 112.5 MPa and an EMI SE of 40 dB at a thickness of 35 μm. Relatively, the most practical method is the construction of nacre-like lamellar, in which the polymer acts as a bonding agent for the two-dimensional MXene nanosheets, thus enhancing the mechanical strength and flexibility of the composite film. Moreover, this method facilitates multiple internal reflections, resulting in the absorption of electromagnetic waves. Using the vacuum filtration-induced self-assembly technique, Cao et al.45 prepared a MXene/CNF composite paper with a nacre-like lamellar structure. The tensile strength and fracture strain of the composite paper are 135.4 MPa and 16.7%, respectively. Moreover, the composite paper exhibited a relatively good absolute shielding efficiency of 2647 dB cm2 g−1 at 47 μm thickness. In addition, bacterial cellulose,46 epoxy resins,34 polyvinylidene fluoride,32 and sodium alginate47,48 can also be used as polymeric substrates to prepare MXene-based electromagnetic shielding materials. However, the EMI SE of these polymers is not stable in harsh environments, which can significantly limit their application. Among the polymeric materials, polyimide (PI) can be used as an ideal candidate substrate because of its excellent mechanical properties, high and low temperature resistances, and flame retardancy,49–54 which will widen its applicability as an electromagnetic shielding material.
Herein, an ultrathin and flexible PI/Ti3C2TX composite film with a nacre-like lamellar structure was successfully designed and fabricated using a simple vacuum filtration and thermal imidization process. The design of nacre-like lamellar structures allows PI to join the Ti3C2TX nanosheets together well, which plays a mechanically reinforcing role. This design also facilitates multiple reflections of electromagnetic waves between adjacent layers, acting as an internal loss. In addition, the PI/Ti3C2TX composite film can maintain a high and stable EMI SE under various harsh environments (high temperature (250 °C), low temperature (−196 °C), ultrasonic, and hygrothermal environment). Compared with other MXene composites, the PI/Ti3C2TX composite film has a simple preparation process with excellent EMI SE, even in harsh environments, providing good application prospects in the field of flexible wearable electronic devices and aerospace fields.
Experimental
Materials and chemicals
3,3′,4,4′-Biphenyltetracarboxylic dianhydride (BPDA, ≥97%), 4,4′-diaminodiphenyl ether (ODA, ≥98%), and lithium fluoride (LiF, ≥99%) were obtained from Shanghai Aladdin Biochemical Technology Co. Triethylamine (TEA, ≥99%), N,N-dimethylacetamide (DMAc, ≥99%) and hydrochloric acid (HCl, AR) were purchased from Shanghai Titan Technology Co. Titanium aluminum carbide (Ti3AlC2, 200 mesh) was provided by Beijing Huawei Ruike Chemical Co. All chemicals and materials were used as received.
Preparation of Ti3C2TX MXene nanosheets
Ti3C2TX nanosheets were prepared based on the modified minimally intensive layer delamination method.55,56 In general, 1.6 g of lithium fluoride was dissolved in 20 mL of hydrochloric acid (9 mol L−1) for 10 min to prepare the in situ HF solution. Then, 1.0 g of Ti3AlC2 was added to the aforementioned HF solution and reacted at 40 °C for 24 h. The final reaction mixture was washed 4–5 times with deionized water (by centrifugation at 8000 rpm for 5 min) until the pH of the supernatant reached above 6. Finally, the resulting swollen deposits diluted with deionized water were treated by sonication under argon protection for 1 h and then centrifuged at 3500 rpm for 1 h. The supernatant was obtained as the required few-layer Ti3C2TX at a concentration of approximately 2 mg mL−1.
Preparation of water-soluble polyamide acid (PAA)
Triethylamine-capped polyamide acid (TEA-PAA), the water-soluble PI precursor was synthesized by the polycondensation method reported previously,17,57 and can play a good protective role similar to other water-based polymers.58–60 Typically, 4.0796 g of ODA was first dissolved in 30 g of DMAc solution, then 5.9204 g of BPDA was dissolved in 60 g of DMAc solution, and they were mechanically stirred in an ice-water bath for 6 h to obtain a transparent yellowish viscous solution. Subsequently, the solution with a solid content of 10 wt% was dropped into deionized water at 0 °C for precipitation. Finally, the precipitate was repeatedly washed 5 times, and the solid PAA was obtained after freeze-drying. Approximately 1 g of the aforementioned PAA was dissolved in 98.525 g of aqueous solution containing 0.475 g of TEA and stirred for 3 h until dissolved to obtain a TEA-PAA solution with a 1 wt% concentration.
Preparation of PI/Ti3C2TX composite films
The PI/Ti3C2TX composite films were fabricated by vacuum-assisted filtration and thermal imidization process (Fig. 1). Specifically, 15 mL of Ti3C2TX aqueous dispersion (2 mg mL−1) was added dropwise to the 1 wt% TEA-PAA solution with different qualities under continuous agitation. Subsequently, the mixture was sonicated for 10 min and stirred for 3 h to form a homogeneous suspension. Thereafter, PAA/Ti3C2TX films were prepared by vacuum-assisted filtration. Finally, PI/Ti3C2TX composite films were prepared by thermal imidation in a vacuum oven at 100, 150, 200 and 250 °C for 1 h, respectively. The mass ratios of PI to Ti3C2TX were 10
:
90, 20
:
80, 30
:
70, and 40
:
60 and denoted as PM-1, PM-2, PM-3, and PM-4, respectively.
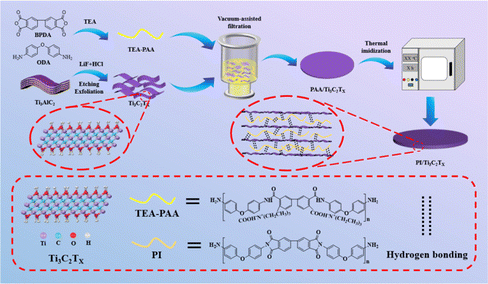 |
| Fig. 1 Schematic illustration for the fabrication of flexible conductive PI/Ti3C2TX composite films. | |
Characterization and measurements
A transmission electron microscope (TEM, JEM-2100, Japan) and a field emission scanning electron microscope (SEM, S-4800, Japan) were used to characterize the microstructure and morphology of the samples. The phase compositions of the samples were analyzed using X-ray diffractometry (XRD, D/max2550VB/PC, Japan). Dynamic light scattering (DLS, Zetasizer Nano Series, USA) was conducted to characterize the size distribution of Ti3C2TX nanosheets. X-ray photoelectron spectroscopy (XPS, ESCALAB 250Xi, UK) was performed to analyze the surface chemistry of the samples. The thermal properties were characterized using a thermogravimetric analyzer (TGA, Netzsch TG209, Germany) in nitrogen from 30 °C to 800 °C at a heating rate of 10 °C min−1. The infrared spectra of the samples were measured using Fourier transform infrared spectroscopy (FTIR, Nicolet is50, USA). The tensile properties were measured on an electronic universal testing machine (E42.503, China) by cutting each sample into 30 mm × 5 mm strips at a loading rate of 1 mm min−1. Conductivity was measured by using a four-point probe test system (RTS-8). EMI SE was measured based on the waveguide method with a vector network analyzer (Keysight, N5222B, USA) in the frequency range of 8.2 to 12.4 GHz (X-band). The power coefficients for reflection (R), absorption (A), transmission (T), and the total EMI shielding efficiency (EMI SET) are calculated from the scattering parameters (S11 and S21) according to the following equations:19,45,61,62 | SER = −10 log(1 − R) | (4) |
| 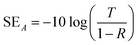 | (5) |
where SER is the microwave reflection, SEA is the microwave absorption, and SEM is the microwave multiple internal reflection. When SET ≥ 15 dB, SEM can be neglected.19,45,61,62
Results and discussion
Characterizations of PI/Ti3C2TX composite films
First, the microstructure and morphology of Ti3C2TX nanosheets was characterized. The SEM result shows that Ti3AlC2 has a tightly packed structure (Fig. 2(a)), while the etched Ti3C2TX has an accordion-like multilayer structure (Fig. 2(b)). The size of the Ti3C2TX nanosheets ranging from 500 nm to 2 μm can be confirmed by using TEM (Fig. 2(c)) and DLS (Fig. 2(d)). Meanwhile, owing to the nanometer dimension of Ti3C2TX nanosheets, a typical “Tyndall effect” can be observed (Fig. 2(e)). In addition, the successful preparation of Ti3C2TX nanosheets can be directly demonstrated by the XRD pattern (Fig. 2(f)). The shift of the (002) peak from 9.5° to 7.1° and the significant reduction or disappearance of the diffraction peaks confirm the elimination of Al after etching and the increase in the Ti3C2TX layer spacing after exfoliation. The XPS results show the introduction of a large amount of fluorine on the Ti3C2TX surface after etching (Fig. 2(g)) and the disappearance of the peak on the Al 2p orbital (Fig. 2(h)), further confirming that Ti3C2TX nanosheets are prepared successfully.
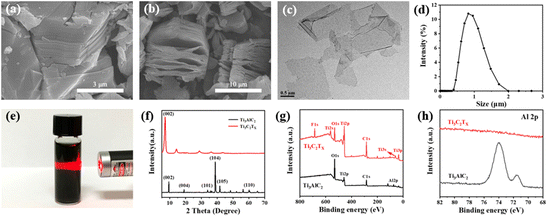 |
| Fig. 2 (a) SEM image of Ti3AlC2. (b) SEM image, (c) TEM image, (d) DLS image, and (e) the Tyndall effect of Ti3C2TX. (f) XRD image, (g) XPS image, and (h) Al 2p spectra of Ti3AlC2 and Ti3C2TX. | |
PM composite films with different PI contents were obtained by solution blending Ti3C2TX aqueous dispersions with different masses of water-soluble PAA, followed by vacuum filtration and thermal imidization (Fig. S1, ESI†). Herein, PI resins act as a binder to improve the mechanical properties and as antioxidant coatings to protect Ti3C2TX, and the resulting PM composite films have good flexibility (Fig. S2, ESI†). The TGA curves of PM composite films with different PI contents are shown in Fig. S3 (ESI†). Because of the loss of a certain amount of PI and Ti3C2TX in the process of vacuum filtration, the content of PI in the PM composite films changes when compared with the nominal content of PI.
Fig. 3(a) and (b) show the microstructure of pure Ti3C2TX and PM composite films, respectively. Without the addition of PI, the pure Ti3C2TX film shows a tightly packed lamellar structure. In contrast, the PM composite film shows a relatively loose layered structure due to the polymer insertion, which allows the Ti3C2TX nanosheets to be effectively separated and avoids restacking, but nonetheless is still sufficiently connected to form a continuous conductive network. In addition, EDS mapping images show that the Ti3C2TX nanosheets are uniformly distributed in the PM composite film (Fig. 3(c) and (d)). This structure is similar to the structure of a pearl shell, with the Ti3C2TX nanosheets playing the role of inorganic “bricks” and PI playing the role of organic “mortar”, and the biomimetic structure not only enhances the mechanical properties, but also facilitates the reflection and absorption of electromagnetic waves of the PM composite film.45
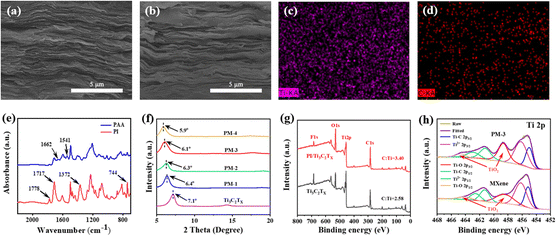 |
| Fig. 3 (a) Cross-sectional SEM image of pure Ti3C2TX films. (b) Cross-sectional SEM and (c) and (d) EDS mapping images of PM composite films. (e) FTIR spectra of PAA and PI. (f) XRD patterns of the PM composite film with different PI contents. (g) XPS survey spectrum of pure Ti3C2TX films and PM composite films. (h) Ti 2p spectra of Ti3C2TX and PM-3. | |
The FTIR spectra confirm the successful conversion of PAA to PI after thermal imidization (Fig. 3(e)). The absorption peaks at 1662 cm−1 (amidic acid, C
O) and 1541 cm−1 (amidic acid, C–N) appearing in PAA are no longer observed after thermal imidization. These peaks are replaced by absorption peaks at 1717 and 1778 cm−1, which are the symmetric and asymmetric stretching vibration absorption peaks of the carbonyl group on the imide ring of PI, respectively. In addition, absorption peaks at 744 and 1372 cm−1 are observed, which are assigned to carbonyl bending and imide bond stretching vibrations, respectively. These peaks provide sufficient evidence for the presence of an aromatic imide structure. The XRD spectral results show that the (002) peak shifts from 2θ = 7.1° to 5.9° with the increase in PI content (Fig. 3(f)), and the layer spacing between Ti3C2TX nanosheets in the PM composite film increases, indicating that PI is successfully embedded in the interlayer space of Ti3C2TX nanosheets. Meanwhile, the chemical compositions of the pure Ti3C2TX film and the PM composite film have been analyzed by XPS (Fig. 3(g)). It can be found that the surface of the PM composite film is rich in F- and O-groups, which can be well hydrogen bonded with the polymer. Also, due to the introduction of PI, the atomic ratio of C/Ti in the PM composite film (3.40) is higher than that of the pure Ti3C2TX film (2.58). Furthermore, the XPS Ti 2p spectra of Ti3C2TX and PM-3 (Fig. 3(h)) show a slight increase in the TiO2 percentage from 24.85% for Ti3C2TX to 27.91% for PM-3, which is mainly due to oxidation of Ti3C2TX during high temperature thermal imidization. Notably, the introduction of PI coating well prevents further oxidation of Ti3C2TX nanosheets and effectively enhances their stability,63 which can also be fully demonstrated in later application in harsh environments.
Mechanical properties of PI/Ti3C2TX composite films
The mechanical strength and toughness of the MXene-based electromagnetic shielding film can be improved by the design of the “brick-and-mortar” structure.41Fig. 4(a) presents the tensile stress–strain curves of pure Ti3C2TX and PM composite films, and the detailed mechanical properties are listed in Table S1 and Fig. S4 (ESI†). As expected, the PM composite films exhibit excellent mechanical properties (Fig. 4(b)). The pure Ti3C2TX film shows a tensile strength of 12.9 ± 0.5 MPa and a fracture strain of 0.58 ± 0.01%. With increasing PI content from 10 wt% to 40 wt%, the tensile strength of the PM composite films show a trend of first increasing and then decreasing. In particular, when the PI content reached 30 wt%, the corresponding tensile strength and fracture strain are 114.9 ± 1.6 MPa and 3.02 ± 0.12%, which are 9 and 5 times higher than those of the pure Ti3C2TX film, respectively.
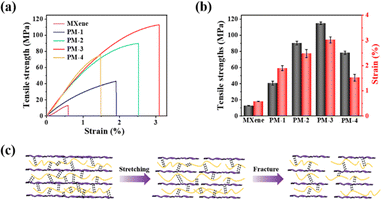 |
| Fig. 4 (a) Tensile stress–strain curves of the PM composite films with different PI contents. (b) Tensile stresses and strains of the PM composite films with different PI contents. (c) Schematic illustration of the fracture mechanism of the PM composite film. | |
The schematic fracture mechanism is proposed to explore the synergistic toughening effect of Ti3C2TX nanosheets and PI (Fig. 4(c)). The hydrogen bondings between the Ti3C2TX nanosheets and PI48,63 are broken at first when stretching starts, and the nanosheets slip in the stretching direction. Meanwhile, PI can afford additional frictional energy loss between adjacent nanosheets as a way to resist the sliding effect until cracks occur. Subsequently, to avoid crack propagation, PI chains are stretched and more energy is consumed until the material is completely pulled off.45,47
EMI shielding performance of PI/Ti3C2TX composite films
The EMI shielding performance of conductive materials is closely related to their electrical conductivity. As shown in Fig. 5(a), the pure Ti3C2TX film exhibits the highest conductivity of 1865 S cm−1. However, the conductivity of PM composite films decreases accordingly with increasing PI content. Fortunately, when the PI content reaches 40%, the conductivity of the PM composite film still reaches 667 S cm−1, which is much higher than 1 S m−1 for the practical application of EMI shielding materials. Meanwhile, the EMI SE of PM composite films with different PI contents in the X-band region is shown in Fig. 5(b). With increasing PI content, the EMI SE of PM composite films gradually decrease, the average EMI SE of pure Ti3C2TX film can reach 56 dB, and the average EMI SE of PM-1 to PM-4 are 44, 41, 37 and 28 dB, respectively, all of which meet the requirements of commercial grade EMI shielding applications (15 dB).
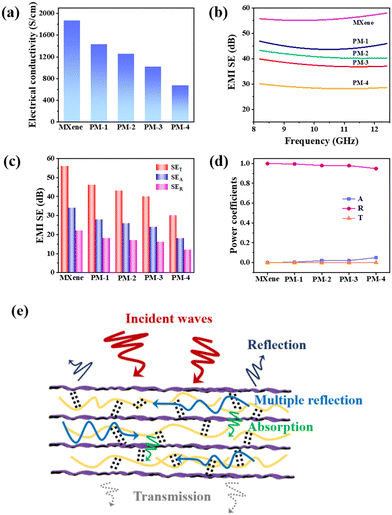 |
| Fig. 5 (a) Electrical conductivity of PM composite films with different PI contents. (b) The EMI SE of PM composite films with different PI contents in the X-band region. (c) Comparison of SET, SEA, and SER at a frequency of 12.4 GHz of the PM composite films with different PI contents. (d) Average power coefficients of A, R, and T. (e) Schematic illustration of the EMI shielding mechanism of the PM composite film. | |
SET, SEA, and SER of PM composite films with different PI contents at 8.2 GHz are investigated to further explore the EMI shielding mechanism of the PM composite film. As shown in Fig. 5(c), SEA and SER have the same trend as SET, both decreasing with increasing PI and decreasing MXene contents. Meanwhile, the contribution of SEA to SET is greater than that of SER, indicating that the absorption mechanism plays a dominant role within the material. Fig. 5(d) shows the average power coefficients of different PM composite films. It is clear that the value of R is much higher than that of A, revealing that most of the incident waves are attenuated by reflection for the whole PM composite films. Therefore, the main shielding mechanism of the PM composite films is predominantly reflection, and a small fraction of the incident electromagnetic waves can penetrate into the composite films and be absorbed. Additionally, the R values of the PM composite films slightly decrease with the increase in the PI content, while the A values slightly increase. The R-value of PM-4 is 0.95 while the A-value is only 0.05. In all PM composite films, the sum of the R-value and A-value is very close to 100%, indicating that almost all penetrating electromagnetic waves can be absorbed. Therefore, the PM composite films can provide a very good electromagnetic shielding effect.
Fig. 5(e) depicts the transmission process of electromagnetic waves through the PM composite film to better explain the EMI shielding mechanism. First, most of the electromagnetic waves are immediately reflected at the surface of the shielding material because of the impedance mismatch between the surface of the shielding material and the air. The remaining electromagnetic waves then enter the interior of the material and interact with the high charge density Ti3C2TX nanosheets, resulting in a loss of energy from the electromagnetic waves. Meanwhile, the nacre-like lamellar structure of the PM composite films facilitates multiple reflections of electromagnetic waves inside, promoting energy dissipation and absorption of electromagnetic waves, and the resulting composite film can effectively shield more than 99.99% of the electromagnetic waves, playing a very good electromagnetic shielding effect.63,64
Summary and comparison of the properties of PI/Ti3C2TX composite films
In order to compare the various properties of the PM composite films, we have facilitated this comparison by plotting their mechanical properties, electrical conductivity and EMI SE as radar plots (Fig. 6(a)). Clearly, when the PI content increases, the tensile strength of PM composite films shows a trend of increasing and then decreasing, while the EMI shielding performance and electrical conductivity decrease. Considering all the properties, the PM-3 composite films exhibit excellent mechanical properties, with a tensile strength of 114.9 MPa, and outstanding electromagnetic shielding properties, with an EMI SE of 37 dB at a thickness of only 15 μm.
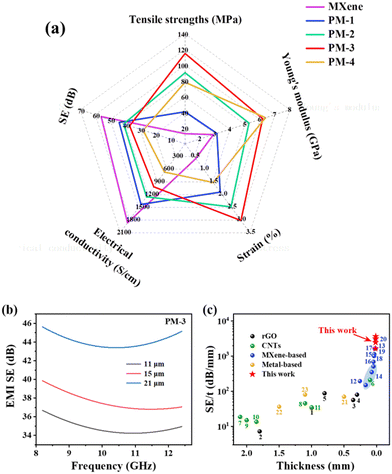 |
| Fig. 6 (a) A radial plot comparing the tensile strength, strain, Young's modulus, electrical conductivity, and EMI SE for MXene and PI/Ti3C2TX composite films. (b) EMI SE of PM-3 composite films with different thicknesses. (c) Comparison of SE/t of PM composite films with literature studies. The numbers inside Fig. 6(c) are sample numbers listed in Table S2 (ESI†). | |
The PI/Ti3C2TX composite films in this study are compared with those reported in other studies to highlight their excellent EMI shielding properties. Given that the EMI SE of PM composite films are dependent on thickness (Fig. 6(b)), thicker PM composite films exhibit higher EMI SE. Therefore, to provide a fair comparison, EMI SE is normalized by thickness (SE/t) herein. As shown in Table S2 (ESI†) and Fig. 6(c), PM composite films prepared herein show an ultrathin thickness as well as a high SE/t, ranking high in the graph compared with other shielding materials, and can be used as efficient EMI shielding materials.
Flame retardancy and EMI shielding performance in harsh environments of PI/Ti3C2TX composite films
Due to the combination of excellent mechanical properties and electromagnetic shielding properties, the PM-3 composite film is able to show good promise for use in high-performance EMI shielding materials. In particular, for practical applications, its flame retardancy and EMI shielding performance under harsh conditions are critical. As shown in Fig. 7(a), the PM-3 composite film was burned with the flame of an alcohol lamp for half a minute and the whole process was recorded with a smartphone (Video S1, ESI†). As a result, the PM-3 composite film was never ignited during the whole burning process, which is a great indication of its good flame retardancy. In addition, the PM-3 composite film was treated at high temperature (250 °C) and low temperature (−196 °C) for 1 h (Video S2, ESI†) to check the change in EMI shielding performance before and after the treatment. As shown in Fig. 7(b) and (c), the EMI shielding performance of the PM-3 composite film is almost unchanged after 1 h of treatment at 250 °C, while the EMI shielding performance is slightly reduced after 1 h of treatment at a low temperature of −196 °C, indicating that the material is well suited for application in high and low temperature environments. In addition, the bonding stability of the Ti3C2TX nanosheets and the PI matrix also affects the stability of the EMI shielding performance due to the weak hydrogen bonding and van der Waals interaction.65 Based on the comparison of the EMI shielding performance of the PM-3 composite film before and after 1 h of ultrasonic (240 W, 40 kHz) treatment (Fig. 7(d)), obviously, the EMI shielding performance remains almost stable, indicating a strong bonding between the Ti3C2TX nanosheets and the PI matrix. To better understand the long durability of the material in practical application, the change in EMI shielding performance of the PM-3 composite film was observed after 7 days of storage at 70% RH, 70 °C. As shown in Fig. 7(e), the EMI shielding performance of the material only slightly decreases after 3 and 7 days. Moreover, the decrease is insignificant and remains around 1–3 dB. Clearly, the material still maintains good EMI shielding performance. Therefore, the high EMI shielding PI/Ti3C2TX composite film prepared in this study exhibits broad application prospects in harsh external environments and is expected to be used in aerospace and other fields.
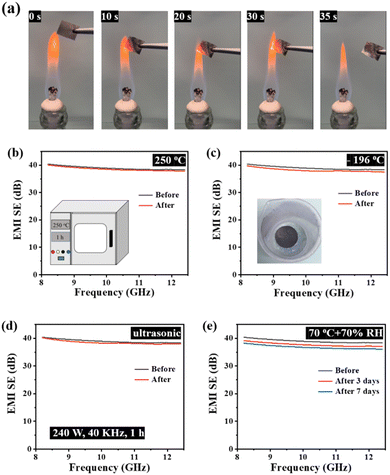 |
| Fig. 7 The flame retardancy of the PM-3 composite film and the stable EMI shielding performance after resisting harsh environments. (a) Images of the combustion process at different time. (b) EMI SE of the PM-3 composite film before and after treatment at 250 °C for 1 h. (c) EMI SE of the PM-3 composite film before and after treatment in liquid N2 (−196 °C) for 1 h. (d) EMI SE of the PM-3 composite film before and after ultrasonic cleaning for 1 h. (e) EMI SE of the PM-3 composite film treated in a hygrothermal environment. | |
Conclusions
Ultrathin and flexible PI/Ti3C2TX composite films have been designed and prepared by a simple vacuum filtration and thermal imidization process. Through the design of the nacre-like lamellar structure, PI is well intercalated between Ti3C2TX nanosheets, playing a role in mechanical reinforcement. Significantly, when the PI content is 30%, the composite film exhibits excellent mechanical properties, with a tensile strength of 114.9 MPa and a fracture strain of 3.0%, which are 9 and 5 times higher than those of pure Ti3C2TX films, respectively. Moreover, the design of the nacre-like lamellar structure also facilitates the multiple reflections of electromagnetic waves between adjacent layers and promotes the absorption of electromagnetic waves. The as-prepared PI/Ti3C2TX composite film (30 wt% PI) has a 37 dB EMI shielding effect in the X-band range when the thickness is only 15 μm. In addition, owing to the high/low temperature resistance of the PI matrix, the PI/Ti3C2TX composite films can be well adapted to various harsh environments and maintain a stable EMI shielding effect; PI plays the role of an anti-oxidation coating and at the same time has good flame retardancy. Therefore, the ultrathin flexible PI/Ti3C2TX composite film exhibits excellent mechanical performance and stable EMI shielding properties. Thus, this film is expected to be used as a high-performance EMI shielding material to resist harsh environments and provides potential application prospects in flexible wearable electronic devices and aerospace fields.
Conflicts of interest
There are no conflicts to declare.
Acknowledgements
This work was supported by the National Nature Science Foundation of China (51503066, 51873063), the National Basic Research Program of China (2013CB035505), the Shanghai Sailing Program (14YF1404900), and the China Postdoctoral Science Foundation (2019M660082).
References
- T. Bai, Y. Guo, D. Wang, H. Liu, G. Song, Y. Wang, Z. Guo, C. Liu and C. Shen, A resilient and lightweight bacterial cellulose-derived C/rGO aerogel-based electromagnetic wave absorber integrated with multiple functions, J. Mater. Chem. A, 2021, 9, 5566–5577 RSC.
- J. Yang, X. Liao, G. Wang, J. Chen, W. Tang, T. Wang and G. Li, Fabrication of lightweight and flexible silicon rubber foams with ultra-efficient electromagnetic interference shielding and adjustable low reflectivity, J. Mater. Chem. C, 2020, 8, 147–157 RSC.
- Y. Han, K. Ruan and J. Gu, Janus (BNNS/ANF)-(AgNWs/ANF) thermal conductivity composite films with superior electromagnetic interference shielding and Joule heating performances, Nano Res., 2022, 15, 4747–4755 CrossRef CAS.
- L. Zhang, J. Luo, S. Zhang, J. Yan, X. Huang, L. Wang and J. Gao, Interface sintering engineered superhydrophobic and durable nanofiber composite for high-performance electromagnetic interference shielding, J. Mater. Sci. Technol., 2022, 98, 62–71 CrossRef CAS.
- G. Yang, X. Zhang, D. Pan, W. Zhang, Y. Shang, F. Su, Y. Ji, C. Liu and C. Shen, Highly Thermal Conductive Poly(vinyl alcohol) Composites with Oriented Hybrid Networks: Silver Nanowire Bridged Boron Nitride Nanoplatelets, ACS Appl. Mater. Interfaces, 2021, 13, 32286–32294 CrossRef CAS.
- S. Luo, T. Xiang, J. Dong, F. Su, Y. Ji, C. Liu and Y. Feng, A double crosslinking MXene/cellulose nanofiber layered film for improving mechanical properties and stable electromagnetic interference shielding performance, J. Mater. Sci. Technol., 2022, 129, 127–134 CrossRef CAS.
- C. Liang, P. Song, A. Ma, X. Shi, H. Gu, L. Wang, H. Qiu, J. Kong and J. Gu, Highly oriented three-dimensional structures of Fe3O4 decorated CNTs/reduced graphene oxide foam/epoxy nanocomposites against electromagnetic pollution, Compos. Sci. Technol., 2019, 181, 107683 CrossRef CAS.
- J. Li, X. Zhang, Y. Ding, S. Zhao, Z. Ma, H. Zhang and X. He, Multifunctional carbon fiber@NiCo/polyimide films with outstanding electromagnetic interference shielding performance, Chem. Eng. J., 2022, 427, 131937 CrossRef CAS.
- J. Ma, M. Zhan and K. Wang, Ultralightweight Silver Nanowires Hybrid Polyimide Composite Foams for High-Performance Electromagnetic Interference Shielding, ACS Appl. Mater. Interfaces, 2015, 7, 563–576 CrossRef CAS PubMed.
- J. Ruan, Z. Chang, H. Rong, T. Alomar, D. Zhu, N. AlMasoud, Y. Liao, R. Zhao, X. Zhao, Y. Li, B. Xu, Z. Guo, Z. El-Bahy, H. Li, X. Zhang and S. Ge, High-conductivity nickel shells encapsulated wood-derived porous carbon for improved electromagnetic interference shielding, Carbon, 2023, 213, 118208 CrossRef CAS.
- H. Cheng, L. Xing, Y. Zuo, Y. Pan, M. Huang, A. Alhadhrami, M. Ibrahim, Z. El-Bahy, C. Liu, C. Shen and X. Liu, Constructing nickel chain/MXene networks in melamine foam towards phase change materials for thermal energy management and absorption-dominated electromagnetic interference shielding, Adv. Compos. Hybrid. Mater., 2022, 5, 755–765 CrossRef CAS.
- D. Kong, J. Li, A. Guo and X. Xiao, High temperature electromagnetic shielding shape memory polymer composite, Chem. Eng. J., 2021, 408, 127365 CrossRef CAS.
- X. Tang, J. Li, Y. Wang, Y. Weng and M. Wang, Controlling distribution of multi-walled carbon nanotube on surface area of Poly(epsilon-caprolactone) to form sandwiched structure for high-efficiency electromagnetic interference shielding, Composites, Part B, 2020, 196, 108121 CrossRef CAS.
- H. Yang, Z. Li, H. Zou and P. Liu, Preparation of porous polyimide/in-situ reduced graphene oxide composite films for electromagnetic interference shielding, Polym. Adv. Technol., 2017, 28, 233–242 CrossRef.
- S. Zhao, H. Zhang, J. Luo, Q. Wang, B. Xu, S. Hong and Z. Yu, Highly Electrically Conductive Three-Dimensional Ti3C2TX MXene/Reduced Graphene Oxide Hybrid Aerogels with Excellent Electromagnetic Interference Shielding Performances, ACS Nano, 2018, 12, 11193–11202 CrossRef CAS.
- P. Song, H. Qiu, L. Wang, X. Liu, Y. Zhang, J. Zhang, J. Kong and J. Gu, Honeycomb structural rGO-MXene/epoxy nanocomposites for superior electromagnetic interference shielding performance, Sustainable Mater. Technol., 2020, 24, e00153 CrossRef CAS.
- Q. Wei, S. Pei, X. Qian, H. Liu, Z. Liu, W. Zhang, T. Zhou, Z. Zhang, X. Zhang, H. Cheng and W. Ren, Superhigh Electromagnetic Interference Shielding of Ultrathin Aligned Pristine Graphene Nanosheets Film, Adv. Mater., 2020, 32, 1907411 CrossRef CAS.
- H. Liu, Q. Li, Y. Bu, N. Zhang, C. Wang, C. Pan, L. Mi, Z. Guo, C. Liu and C. Shen, Stretchable conductive nonwoven fabrics with self-cleaning capability for tunable wearable strain sensor, Nano Energy, 2019, 66, 104143 CrossRef CAS.
- J. Yan, C. Ren, K. Maleski, C. Hatter, B. Anasori, P. Urbankowski, A. Sarycheva and Y. Gogotsi, Flexible MXene/Graphene Films for Ultrafast Supercapacitors with Outstanding Volumetric Capacitance, Adv. Funct. Mater., 2017, 27, 1701264 CrossRef.
- Z. Yu, T. Dai, S. Yuan, H. Zou and P. Liu, Electromagnetic Interference Shielding Performance of Anisotropic Polyimide/Graphene Composite Aerogels, ACS Appl. Mater. Interfaces, 2020, 12, 30990–31001 CrossRef CAS.
- Y. Xu, Z. Lin, Y. Yang, H. Duan, G. Zhao, Y. Liu, Y. Hu, R. Sun and C. Wong, Integration of efficient microwave absorption and shielding in a multistage composite foam with progressive conductivity modular design, Mater. Horiz., 2022, 9, 708–719 RSC.
- Y. Li, D. Zhang, B. Zhou, C. He, B. Wang, Y. Feng and C. Liu, Synergistically enhancing electromagnetic interference shielding performance and thermal conductivity of polyvinylidene fluoride-based lamellar film with MXene and graphene, Composites, Part A, 2022, 157, 106945 CrossRef CAS.
- A. Iqbal, P. Sambyal and C. Koo, 2D MXenes for Electromagnetic Shielding: A Review, Adv. Funct. Mater., 2020, 30, 2000883 CrossRef CAS.
- S. Chen, Y. Zheng, B. Zhang, Y. Feng, J. Zhu, J. Xu, C. Zhang, W. Feng and T. Liu, Cobalt, Nitrogen-Doped Porous Carbon Nanosheet-Assembled Flowers from Metal-Coordinated Covalent Organic Polymers for Efficient Oxygen Reduction, ACS Appl. Mater. Interfaces, 2019, 11, 1384–1393 CrossRef CAS PubMed.
- A. Lipatov, M. Alhabeb, M. Lukatskaya, A. Boson, Y. Gogotsi and A. Sinitskii, Effect of Synthesis on Quality, Electronic Properties and Environmental Stability of Individual Monolayer Ti3C2 MXene Flakes, Adv. Electron. Mater., 2016, 2, 1600255 CrossRef.
- M. Naguib, V. Mochalin, M. Barsoum and Y. Gogotsi, 25th Anniversary Article: MXenes: A New Family of Two-Dimensional Materials, Adv. Mater., 2014, 26, 992–1005 CrossRef CAS PubMed.
- P. Song, B. Liu, H. Qiu, X. Shi, D. Cao and J. Gu, MXenes for polymer matrix electromagnetic interference shielding composites: A review, Compos. Commun., 2021, 24, 100653 CrossRef.
- G. Lee, T. Yun, H. Kim, I. Kim, J. Choi, S. Lee, H. Lee, H. Hwang, J. Kim, D. Kim, H. Lee, C. Koo and S. Kim, Mussel Inspired Highly Aligned Ti3C2TX MXene Film with Synergistic Enhancement of Mechanical Strength and Ambient Stability, ACS Nano, 2020, 14, 11722–11732 CrossRef CAS.
- J. Cai, X. Tang, X. Chen and M. Wang, Temperature and strain-induced tunable electromagnetic interference shielding in polydimethylsiloxane/multi-walled carbon nanotube composites with temperature-sensitive microspheres, Composites, Part A, 2021, 140, 106188 CrossRef CAS.
- C. Zhang, S. Pinilla, N. McEyoy, C. Cullen, B. Anasori, E. Long, S. Park, A. Seral-Ascaso, A. Shmeliov, D. Krishnan, C. Morant, X. Liu, G. Duesberg, Y. Gogotsi and V. Nicolosi, Oxidation Stability of Colloidal Two-Dimensional Titanium Carbides (MXenes), Chem. Mater., 2017, 29, 4848–4856 CrossRef CAS.
- Z. Lin, X. Li, H. Zhang, B. Xu, P. Wasnik, H. Li, M. Singh, Y. Ma, T. Li and Z. Guo, Research progress of MXenes and layered double hydroxides for supercapacitors, Inorg. Chem. Front., 2023, 10, 4358–4392 RSC.
- K. Rajavel, S. Luo, Y. Wan, X. Yu, Y. Hu, P. Zhu, R. Sun and C. Wong, 2D Ti3C2TX MXene/polyvinylidene fluoride (PVDF) nanocomposites for attenuation of electromagnetic radiation with excellent heat dissipation, Composites, Part A, 2020, 129, 105693 CrossRef CAS.
- R. Sun, H. Zhang, J. Liu, X. Xie, R. Yang, Y. Li, S. Hong and Z. Yu, Highly Conductive Transition Metal Carbide/Carbonitride(MXene)@ polystyrene Nanocomposites Fabricated by Electrostatic Assembly for Highly Efficient Electromagnetic Interference Shielding, Adv. Funct. Mater., 2017, 27, 1702807 CrossRef.
- L. Wang, L. Chen, P. Song, C. Liang, Y. Lu, H. Qiu, Y. Zhang, J. Kong and J. Gu, Fabrication on the annealed Ti3C2TX MXene/Epoxy nanocomposites for electromagnetic interference shielding application, Composites, Part B, 2019, 171, 111–118 CrossRef CAS.
- H. Xu, X. Yin, X. Li, M. Li, S. Liang, L. Zhang and L. Cheng, Lightweight Ti2CTX MXene/Poly(vinyl alcohol) Composite Foams for Electromagnetic Wave Shielding with Absorption-Dominated Feature, ACS Appl. Mater. Interfaces, 2019, 11, 10198–10207 CrossRef CAS PubMed.
- X. Wu, B. Han, H. Zhang, X. Xie, T. Tu, Y. Zhang, Y. Dai, R. Yang and Z. Yu, Compressible, durable and conductive polydimethylsiloxane-coated MXene foams for high-performance electromagnetic interference shielding, Chem. Eng. J., 2020, 381, 122622 CrossRef CAS.
- L. Wang, H. Qiu, P. Song, Y. Zhang, Y. Lu, C. Liang, J. Kong, L. Chen and J. Gu, 3D Ti3C2TX MXene/C hybrid foam/epoxy nanocomposites with superior electromagnetic interference shielding performances and robust mechanical properties, Composites, Part A, 2019, 123, 293–300 CrossRef CAS.
- B. Dai, Y. Ma, F. Dong, J. Yu, M. Ma, H. Thabet, S. El-Bahy, M. Ibrahim, M. Huang, I. Seok, G. Roymahapatra, N. Naik, B. Xu, J. Ding and T. Li, Overview of MXene and conducting polymer matrix composites for electromagnetic wave absorption, Adv. Compos. Hybrid. Mater., 2022, 5, 704–754 CrossRef.
- S. Yang, C. Shi, K. Qu, Z. Sun, H. Li, B. Xu, Z. Huang and Z. Guo, Electrostatic self-assembly cellulose nanofibers/MXene/nickel chains for highly stable and efficient seawater evaporation and purification, Carbon Lett., 2023, 33, 2063–2074 CrossRef.
- D. Kong, Z. El-Bahy, H. Algadi, T. Li, S. El-Bahy, M. Nassan, J. Li, A. Faheim, A. Li, C. Xu, M. Huang, D. Cui and H. Wei, Highly sensitive strain sensors with wide operation range from strong MXene-composited polyvinyl alcohol/sodium carboxymethylcellulose double network hydrogel, Adv. Compos. Hybrid. Mater., 2022, 5, 1976–1987 CrossRef CAS.
- Z. Ling, C. Ren, M. Zhao, J. Yang, J. Giammarco, J. Qiu, M. Barsoum and Y. Gogotsi, Flexible and conductive MXene films and nanocomposites with high capacitance, Proc. Natl. Acad. Sci. U. S. A., 2014, 111, 16676–16681 CrossRef CAS PubMed.
- F. Xie, F. Jia, L. Zhuo, Z. Lu, L. Si, J. Huang, M. Zhang and Q. Ma, Ultrathin MXene/aramid nanofiber composite paper with excellent mechanical properties for efficient electromagnetic interference shielding, Nanoscale, 2019, 11, 23382–23391 RSC.
- C. Liang, Z. Gu, Y. Zhang, Z. Ma, H. Qiu and J. Gu, Structural Design Strategies of Polymer Matrix Composites for Electromagnetic Interference Shielding: A Review, Nano-Micro Lett., 2021, 13, 1–29 Search PubMed.
- B. Zhou, Z. Zhang, Y. Li, G. Han, Y. Feng, B. Wang, D. Zhang, J. Ma and C. Liu, Flexible, Robust, and Multifunctional Electromagnetic Interference Shielding Film with Alternating Cellulose Nanofiber and MXene Layers, ACS Appl. Mater. Interfaces, 2020, 12, 4895–4905 CrossRef CAS.
- W. Cao, F. Chen, Y. Zhu, Y. Zhang, Y. Jiang, M. Ma and F. Chen, Binary Strengthening and Toughening of MXene/Cellulose Nanofiber Composite Paper with Nacre-Inspired Structure and Superior Electromagnetic Interference Shielding Properties, ACS Nano, 2018, 12, 4583–4593 CrossRef CAS PubMed.
- Y. Wan, P. Xiong, J. Liu, F. Feng, X. Xun, F. Gama, Q. Zhang, F. Yao, Z. Yang, H. Luo and Y. Xu, Ultrathin, Strong, and Highly Flexible Ti3C2TX MXene/Bacterial Cellulose Composite Films for High-Performance Electromagnetic Interference Shielding, ACS Nano, 2021, 15, 8439–8449 CrossRef CAS PubMed.
- F. Shahzad, M. Alhabeb, C. Hatter, B. Anasori, S. Hong, C. Koo and Y. Gogotsi, Electromagnetic interference shielding with 2D transition metal carbides (MXenes), Science, 2016, 353, 1137–1140 CrossRef CAS PubMed.
- S. Wan, X. Li, Y. Wang, Y. Chen, X. Xie, R. Yang, A. Tomsia, L. Jiang and Q. Cheng, Strong sequentially bridged MXene sheets, Proc. Natl. Acad. Sci. U. S. A., 2020, 117, 27154–27161 CrossRef CAS.
- D. Liaw, K. Wang, Y. Huang, K. Lee, J. Lai and C. Ha, Advanced polyimide materials: Syntheses, physical properties and applications, Prog. Polym. Sci., 2012, 37, 907–974 CrossRef CAS.
- Y. Ding, H. Hou, Y. Zhao, Z. Zhu and H. Fong, Electrospun polyimide nanofibers and their applications, Prog. Polym. Sci., 2016, 61, 67–103 CrossRef CAS.
- Y. Cao, M. Weng, M. Mahmound, A. Elnaggar, L. Zhang, I. Azab, Y. Chen, M. Huang, J. Huang and X. Sheng, Flame-retardant and leakage-proof phase change composites based on MXene/polyimide aerogels toward solar thermal energy harvesting, Adv. Compos. Hybrid. Mater., 2022, 5, 1253–1267 CrossRef CAS.
- K. Zhang, L. Zheng, M. Aouraghe and F. Xu, Ultra-light-weight kevlar/polyimide 3D woven spacer multifunctional composites for high-gain microstrip antenna, Adv. Compos. Hybrid. Mater., 2022, 5, 872–883 CrossRef CAS.
- X. Zhang, J. Dong, D. Pan, G. Yang, F. Su, Y. Ji, C. Liu and C. Shen, Constructing dual thermal conductive networks in electrospun polyimide membranes with highly thermally conductivity but electrical insulation properties, Adv. Compos. Hybrid. Mater., 2021, 4, 1102–1112 CrossRef CAS.
- M. Clausi, M. Zahid, A. Shayganpour and I. Bayer, Polyimide foam composites with nano-boron nitride (BN) and silicon carbide (SiC) for latent heat storage, Adv. Compos. Hybrid. Mater., 2022, 5, 798–812 CrossRef CAS.
- M. Alhabeb, K. Maleski, B. Anasori, P. Lelyukh, L. Clark, S. Sin and Y. Gogotsi, Guidelines for Synthesis and Processing of Two-Dimensional Titanium Carbide (Ti3C2TX MXene), Chem. Mater., 2017, 29, 7633–7644 CrossRef CAS.
- S. Wan, X. Li, Y. Chen, N. Liu, Y. Du, S. Dou, L. Jiang and Q. Cheng, High-strength scalable MXene films through bridging-induced densification, Science, 2021, 374, 96–99 CrossRef CAS.
- X. Chen, H. Liu, Y. Zheng, Y. Zhai, X. Liu, C. Liu, L. Mi, Z. Guo and C. Shen, Highly Compressible and Robust Polyimide/Carbon Nanotube Composite Aerogel for High-Performance Wearable Pressure Sensor, ACS Appl. Mater. Interfaces, 2019, 11, 42594–42606 CrossRef CAS.
- J. Cai, V. Murugadoss, J. Jiang, X. Gao, Z. Lin, M. Huang, J. Guo, S. Alsareii, H. Algadi and M. Kathiresan, Waterborne polyurethane and its nanocomposites: a mini-review for anti-corrosion coating, flame retardancy, and biomedical applications, Adv. Compos. Hybrid. Mater., 2022, 5, 641–650 CrossRef CAS.
- Z. Yan, S. Wang, J. Bi, Q. He, H. Song, I. Azab, S. El-Bahy, A. Elnaggar, M. Huang, M. Mahmoud, J. Wang and Q. Shao, Strengthening waterborne acrylic resin modified with trimethylolpropane triacrylate and compositing with carbon nanotubes for enhanced anticorrosion, Adv. Compos. Hybrid. Mater., 2022, 5, 2116–2130 CrossRef CAS.
- X. He, S. Li, R. Shen, Y. Ma, L. Zhang, X. Sheng, Y. Chen, D. Xie and J. Huang, A high-performance waterborne polymeric composite coating with long-term anti-corrosive property based on phosphorylation of chitosan-functionalized Ti3C2TX MXene, Adv. Compos. Hybrid. Mater., 2022, 5, 1699–1711 CrossRef CAS.
- Z. Zeng, H. Jin, M. Chen, W. Li, L. Zhou and Z. Zhang, Lightweight and Anisotropic Porous MWCNT/WPU Composites for Ultrahigh Performance Electromagnetic Interference Shielding, Adv. Funct. Mater., 2016, 26, 303–310 CrossRef CAS.
- Z. Chen, C. Xu, C. Ma, W. Ren and H. Cheng, Lightweight and Flexible Graphene Foam Composites for High-Performance Electromagnetic Interference Shielding, Adv. Mater., 2013, 25, 1296–1300 CrossRef CAS.
- K. Sun, F. Wang, W. Yang, H. Liu, C. Pan, Z. Guo, C. Liu and C. Shen, Flexible Conductive Polyimide Fiber/MXene Composite Film for Electromagnetic Interference Shielding and Joule Heating with Excellent Harsh
Environment Tolerance, ACS Appl. Mater. Interfaces, 2021, 13, 50368–50380 CrossRef CAS PubMed.
- W. Cao, C. Ma, S. Tan, M. Ma, P. Wan and F. Chen, Ultrathin and Flexible CNTs/MXene/Cellulose Nanofibrils Composite Paper for Electromagnetic Interference Shielding, Nano-Micro Lett., 2019, 11, 1–17 Search PubMed.
- Y. Cheng, X. Li, Y. Qin, Y. Fang, G. Liu, Z. Wang, J. Matz, P. Dong, J. Shen and M. Ye, Hierarchically porous polyimide/Ti3C2TX film with stable electromagnetic interference shielding after resisting harsh conditions, Sci. Adv., 2021, 7, eabj1663 CrossRef CAS.
|
This journal is © The Royal Society of Chemistry 2024 |
Click here to see how this site uses Cookies. View our privacy policy here.