DOI:
10.1039/D3TC00558E
(Paper)
J. Mater. Chem. C, 2023,
11, 11834-11841
Automated corona discharge (CD) for efficient and broad-spectrum surface and air sterilization†
Received
15th February 2023
, Accepted 17th July 2023
First published on 19th July 2023
Abstract
In the past three years, the COVID-19 pandemic has caused serious health, environmental, societal, and economic challenges globally. Sterilization is one of the most efficient methods to mitigate the spread of infectious viruses like SARS-CoV-2. However, extreme sterilization practices can cause serious health and environmental problems worldwide. Heat, ultraviolet C (UVC), and chemical disinfectants require high energy consumption and can cause health concerns, environmental pollution, and chemical overuse. In this paper, we evaluated the efficiency of corona discharge (CD) as an environmentally and energy-friendly sterilization method on different surfaces for sterilization. It was confirmed that CD is an efficient sterilization process for most surfaces and personal protective equipment (PPE). CD allows for a reduction in disinfectant use, addresses the PPE shortage problem, and reduces plastic pollution and biowaste. The air sterilization effect of CD creates a promising option to reduce airborne pathogens. To address the safety concerns of CD, the heat, UVC, and ozone emissions of CD were confirmed to be within a safe range. A wireless, affordable CD robot was developed with the capability to scan along pre-designed paths while having the capability to avoid static and moving obstacles. Automated CD devices and robots can be favorable sterilization solutions for the future as a contactless, more environmentally friendly, efficient, affordable, and portable solution.
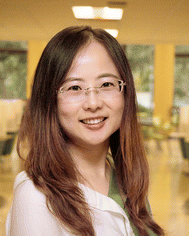
Ying Zhong
| Dr Ying Zhong is currently a professor at School of Materials Science and Engineering at Harbin Institute of Technology at Shenzhen. She received her PhD degree from University of California at San Diego in 2017. When she served as an assistant professor at University of South Florida from 2019 to 2022, she secured three NSF grants, including the NSF CAREER Award. Dr Zhong is specialized in innovations of manufacturing techniques through understanding and taking advantage of surface and interface behaviors. To address mask shortage problems during the COVID-19 pandemic, she developed an efficient technology for safe mask reuse. She also invented an ultra-fast electrostatic printing technique. |
Introduction
As of May 2023, there have been 770 million confirmed cases of COVID-19 worldwide. More than 6.9 million people have lost their lives in this three-year-long epidemic.1 As an airborne infectious respiratory disease, COVID-19 spreads through contaminated air and surfaces.2,3 SARS-CoV-2 has been shown to persist on a variety of surfaces (3–7 days on non-porous plastics, metals, and ceramics,4,5 3 days on clothes,5 and 7 days on nitrile gloves6); it can also be airborne for hours.7,8 Sterilization practices are one of the most powerful ways to limit the spread of viruses like SARS-CoV-2.9,10 The COVID-19 pandemic led to a rise in consumption of household disinfectants, including quaternary ammonium compounds (QACs), hydrogen peroxide, bleach (sodium hypochlorite), alcohols, acids, and phenolic compounds. In 2020, online sales of hand sanitizer and wipes grew by about 5678% compared to the previous year.11 While protecting people from infection, the significant increase in disinfectant exposure has brought serious health and environmental concerns. Inappropriate use of disinfectants can cause indoor air pollution, dermatitis, allergic rhinitis, respiratory problems, or increase asthma.12,13 According to the CDC, from January to March 2020, poison centers received 45
550 exposure calls related to the misuse of cleaners and disinfectants, representing an overall increase of 20.4% from last year.14 Mixing chemicals inappropriately or taking them by swallowing can cause poisoning and may result in death.15 Using massive quantities of disinfectant poses a threat to the environment, bodies of water, soil, wildlife, and biodiversity.16 Efficient, affordable, accessible, and environmentally friendly sterilization solutions for surfaces and air that can reduce or prevent excessive use of disinfectants are highly desirable.
In addition, large amounts of disposable personal protective equipment (PPE), including masks, face shields, coveralls, and gloves, are consumed globally, causing a serious environmental burden. Since the start of the COVID-19 pandemic, more than 8.4 million tons of pandemic-associated plastic waste have been generated globally, with more than 25
000 tons entering the ocean.17 Due to the lack of efficient, affordable, and user-friendly PPE sterilization solutions, PPE is used between patients without proper sterilization around the world, risking cross-infection.18 If managed improperly, an infection caused by medical waste may spread further.19 Therefore, proper sterilization solutions can prevent the spread of infection, decrease the use of PPE, and reduce their environmental impact. Chemical disinfectants, heating, UVC radiation, and/or X-ray radiation are the most commonly used sterilization methods. However, heating consumes a large amount of energy, damages heat-sensitive surfaces, and can only treat limited sizes;20 UVC and X-rays are carcinogenic, which requires extra protection components for the users, leading to higher costs and lower efficiency. Even though UVC sterilization robots have been deployed in many hospitals because of their automation advantages, they typically cost over $80
000 and have a height of over 1.5 meters, limiting their broad application and the areas where they can be used.21,22 The ideal sterilization process is one that is environmentally friendly, effective, affordable, uses little energy, is simple to use, is not harmful to the user, and can be automated.
The sanitization of indoor air is essential to suppress the spread of airborne diseases. The use of hydrogen peroxide spray or a UVC lamp for 60 minutes is some of the recommended practices to prevent cross-infection in hospitals. But these are time-consuming and inefficient, as rooms cannot be used during this process.23 For homes and other indoor public facilities, the current choices are mostly limited to natural ventilation and filtering through the HVAC system. However, the effectiveness is quite limited, and the HVAC system may spread the virus further, especially when filtration is less effective.24 Therefore, continuous, low-cost, and efficient air sterilization solutions that allow the presence of users are highly desirable.
In our previous research, we have confirmed the capability of corona discharge (CD) for the safe reuse of N95 respirators by disinfecting and recharging them simultaneously.25 This technology can address the mask shortage problem and reduce the environmental impact of disposing of used masks. Additionally, we observed cell membrane rupturing and tested DNA damage in the sterilization mechanism of CD. Reactive nitrogen species (RNS) and reactive oxygen species (ROS) species and ozone are generated by the following chemical reactions, which include but are not limited to:
e + O2 → e + O(3P) + O(3P) O + O2 + M → O3 + M |
The OH radical can weaken the barrier function of the unsaturated fatty acid lipid bilayer on the cell membrane, allowing ions and polar compounds to enter. The proteins embedded in the lipid bilayers that can control the passage of various compounds are also susceptible to oxidation when exposed to the ROS and radical-rich environment in the corona, leading to additional damage to the cell membrane. With the integrity of the cell membrane damaged and paths opened, the reactive species generated by corona can directly interact with subcellular biomaterials in the microorganisms, leading to their destruction and inactivation.26
CD has benefits such as non-contact, low energy consumption, affordable, and reusable. In the current research, the use of CD is further explored to sterilize different types of surfaces and the air. The targeted surfaces include both conductive and non-conductive surfaces, with an emphasis on PPE materials to reduce the use of disinfectants, the production of medical waste, and the spread of infectious diseases in healthcare facilities. The broad-spectrum sterilization capability of CD was investigated by testing its impact on E. coli and spores. CD generated in different atmospheres were compared to evaluate the necessity of gas input to enhance the sterilization effect. To evaluate the surface treatment efficiency of CD for large-area sterilization, the generation speed and area covered by it were simulated. The air sterilization capability of CD was tested to evaluate its capability to purify the air. The ozone emission, UVC emission, and temperature increase caused by CD were tested and measured at a safe level, confirming its potential to be used as an environmentally friendly sterilization solution.
Materials and methods
Corona discharge (CD) system and treatment
The CD system was set up as shown in Fig. 1a. The voltage and current applied to the discharge needle were provided by a high-voltage DC power supply (XP Glassman Co., Ltd., FJ Series, 120 W) with a controllable discharge voltage (V) range up to ±60 kV and a current range up to 0.20 mA. The diameter of the discharge tungsten needle electrode tip was approximately 100 μm. Based on our previous results in ref. 25, the discharge voltage and current were respectively set at 25 kV and 0.05 mA, meaning the output power is as low as 1.25 W. The tested surfaces include cloth masks, face shields made of polyethylene terephthalate (PET), coveralls made of polypropylene (PP), alumina, which represents most ceramic surfaces, and stainless steel, which represents conductive surfaces. The specimens were cut into 5 cm by 5 cm squares and then mounted on the ground electrode made from a stainless-steel plate. The needle was set vertically above the center of the specimen at 3.5 cm. As SARS-CoV-2 virus is less resilient against sterilization procedures than most bacteria,27 to improve experiment efficiency and prove the broad-spectrum sterilization capability of CD, Gram-negative bacteria E. coli, as well as Gram-positive bacteria spores, and yeast were selected as the major sterilization efficiency testing subjects in this paper.
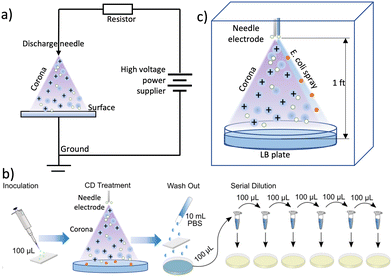 |
| Fig. 1 (a) Schematic of the CD treatment setup; (b) procedure of the sterilization experiments, including inoculation with microorganisms, CD treatment, washing out surviving colonies, serial dilution using PBS, and plating; (c) schematic of CD air sterilization test setup. | |
Sterilization of E. coli
E. coli (DH5- derivative, New England Biolabs Inc.) was chosen as the major microorganism in most sterilization tests to increase experiment efficiency due to the relatively short culturing time and low biosafety concern. As schematized in Fig. 1b, the E. coli solution was spread evenly to form a uniform layer covering the entire surface area of the specimens. Then CD was applied to the samples for 7.5 minutes with the parameters mentioned above. In some experiments, the CD treatment was conducted for more than one cycle. Between each cycle, 5 minutes of resting time were added. After CD treatment, the surviving colonies were washed from the sample with phosphate-buffered saline (PBS). 100 μL of this solution was then added to 900 μL of PBS. This process was repeated to get six serial dilutions. Then 100 μL of each dilution was smeared onto a lysogeny broth (LB) agar plate for incubation at 37 °C. After 20 hours, the surviving colony-forming units (CFU) were counted. All experiments were conducted in triplicate. Log reduction R was used as a measure of sterilization efficacy and calculated by: | Log reduction (R) = log(initial colonies) − log(survived colonies) | (1) |
Broad-spectrum sterilization
To test the broad-spectrum sterilization efficacy of CD, we conducted sterilization experiments on Geobacillus stearothermophilus (which produces heat-resistant spores), which possesses thicker cell walls than E. coli, meaning better tolerance against membrane rupture and DNA leakage. G. stearothermophilus cells were prepared using 100 mL of nutrient broth (DifcoTM nutrient broth for the cultivation of non-fastidious microorganisms, 3 grams of beef extract, and 5 grams of peptone per liter) and incubated overnight at 50 °C at 120 rpm (reaching an initial concentration of 104 CFU mL−1). The same CD treatment against E. coli was applied to evaluate the sterilization of CD against G. stearothermophilus.
Atmosphere control and air sterilization
The atmosphere of the CD process was controlled by initiating sterilization when a controllable gas flow was turned on. The CD treatment using either nitrogen or oxygen gas was conducted within a hermetically sealed acrylic chamber to ensure a controlled environment. The gas cylinder was connected to a pipe, which was regulated by an inlet valve. At the opposite end of the pipe, a corona discharge needle was positioned, pointing downward towards the sample being treated. The CD needle was concentric and positioned adjacent to the gas inlet, allowing for overlap to generate the plasma. The chamber was firstly vacuumed to ∼100 Pa. To initiate the treatment, the gas inlet was opened for a few minutes to allow the chamber to fill with gas, with a flow pressure set at 20 psi. Subsequently, the CD was activated for 7.5 minutes to perform the sterilization treatment. This process took place in the closed chamber under a nitrogen and oxygen environment, respectively. The CD parameters employed for this treatment were consistent with those described in the “Corona Discharge (CD) system and treatment” section of this study. To evaluate the impact of humidity, the humidity in the chamber was controlled by injecting mist from a humidifier and monitored by a humidity sensor (Sensirion, SHT4). To evaluate the capability of CD for air sterilization, an E. coli solution with a concentration of 103 CFU was sprayed as aerosols along the direction of CD, as shown in Fig. 1c. The distance between the spray nozzle and the LB plate was set at 30 cm. The aerosols were generated using a spray nozzle with an average droplet size of 5 to 20 μm. The CD was generated immediately after the aerosols were introduced. According to our previous study, it takes about 10 ms to generate cold plasma and hence this ensures that most of the aerosols were exposed to corona during the treatment.28 The spray zone was enclosed in a transparent chamber to avoid leakage or contamination of the surroundings.
Characterization of corona discharge
The OES spectrum of CD was acquired using a spectrometer (AvaSpec-ULS2048CL-EVO, Avantes). The fiber optic cable was positioned at horizontal distances of 5.5 cm, 7.5 cm, and 9.5 cm from the needle tip. The ion density from the discharge was measured using an air ion counter (AIC2, Alpha Lab Inc.). The measurements were conducted using positive polarity, and the device was positioned at a parallel distance of 7.5 cm from the needle tip. Ozone generation was measured using an ozone meter (Forensics Detectors, model FD-90A-O3, 0.1% resolution) at 0 to 5 cm horizontal distances away from the center of the corona before it was turned on and after it had been working for 2 minutes, 4 minutes, 6 minutes, and 8 minutes.
Simulation of corona discharge
COMSOL Multiphysics 6.0 COMSOL (Application Gallery ID 14035: Atmospheric Pressure Corona Discharge) was utilized to simulate the CD process. A two-dimensional symmetrical setup sharing the same dimensions as the experiment was used. A voltage of 25 kV was applied at the tip of the needle to simulate the CD process.
Assembly of programming of the CD robot
Two VEX robotics kits, three VEX ultrasonic range finders (3 cm range), one portable high voltage transformer (Shihon Power Supply, 12 V DC input, 25 kV, and 0.05 mA output), one 12 V DC battery, one tungsten needle, and some connection cables were used to assemble the CD robot. Python is the programming language for the autonomy of the CD robot. The final product was constructed by combining the two bases of the robotic kits. A static arm was attached to the front of the robot to hold the needle with its two claws. The two wheels on each side of the robot connect to a large gear of 3.5 inches, which spins adjacent to a smaller gear of 0.5 inches attached to the motor. This allows the motor to run at full power while maintaining a slow speed of 0.2 ft s−1 for scanning. Three VEX ultrasonic range finder sensors were attached to the left, front, and right sides of the robot to detect any obstacles in its path and measure the distance of the surrounding objects to the robot. The 12-volt battery works with the high-voltage transformer to create CD through the needle. The battery and transformers were mounted to the robot in 3D-printed containers. The design is compact and fits within the frame.
Results and discussion
Sterilization of different surfaces
We evaluated the sterilization effectiveness of CD on surfaces including cotton cloth masks, PET face shields, PP coveralls, alumina, and stainless steel. The results are elaborated in Fig. 2a. It was learned that after 2 cycles of CD treatment, the average R for E. coli on N95 masks reaches as high as 3.37. Interestingly, the R for cloth masks turned out to be lower than for N95 masks, with an average value of 2.54. It is caused by the hydrophilic property and the thinner thickness of the cotton cloth. The E. coli solution-soaked part of the cloth material, which gathered at the surface of the substrate underneath, led to less exposure of E. coli to CD.
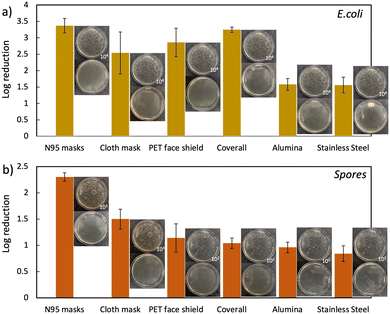 |
| Fig. 2 The R of CD on different surfaces when contaminated with (a) E. coli and (b) G. stearothermophilus. The insets are images of the LB plates before and after CD treatment. | |
An average R of 2.54 is already better than regular washing, the most common cleaning method for cloth masks. Additionally, compared with washing, CD treatment is a noncontact and dry process, skipping the long-time drying procedure and reducing the waiting time from hours to minutes between each use. If commercialized, CD devices may provide immediate mask sterilization solutions for people. It can reduce the use of detergents and water, address the shortage of masks, and provide immediate mask sterilization whenever needed.
Face shields and coveralls are the other two important PPEs for protecting healthcare workers from infectious diseases. Face shields are normally reused, which requires going through a sterilization process such as alcohol spraying or UVC radiation, which may cause material degradation; washing, which consumes a large amount of water and detergent; or hydrogen peroxide vapor (HPV), whose high temperature may cause damage to PET material. Here, we confirm that CD treatment can achieve an average R of 2.86 as a contactless, heatless solution without impacting the transmittance of the face shield. It is a promising solution for quick and safe face shield reuse. Coveralls are the largest piece of PPE used on the front lines of infectious disease combat. Their massive use is the largest concern for non-degradable plastic pollution and biowaste-caused infections. The R of 3.25 on coveralls provided by CD and its large area scanning capability provide the possibility to disinfect large-size PPE and biowaste at the industrial level.
In addition to PPE made by polymers, we also conducted sterilization experiments on ceramics and metals by using alumina and stainless steel as representatives. However, the contact angle between the E. coli solution and the smooth alumina and stainless-steel surfaces was large. It was difficult to minimize the droplet size, resulting in droplets with diameters larger than 1 mm and limiting the exposure of E. coli to CD on those tested 5 cm × 5 cm samples. The tested average R for millimeter droplets on alumina and stainless steel was 1.58 and 1.56, respectively, which is still good enough for the sterilization of daily-used surfaces. But in real applications, the droplet sizes are only in the micrometer range, much smaller than what we tested on these two types of surfaces, meaning higher R can be achieved.
Broad spectrum sterilization of corona discharge
G. stearothermophilus, a type of Gram-positive bacterium that is widely distributed in soil, hot springs, and ocean sediment, is the cause of the flat, sour spoilage of canned liquid foods.29 The G. stearothermophilus spore is one of the most disinfection-resistant spores of aerobic microorganisms, highly resistant to many physical treatments and chemical agents, including heat, drying, radiation, and chemicals. They are often used as biological indicators to evaluate the effectiveness of sterilization processes.30 The R of CD against spores on different surfaces was evaluated, as shown in Fig. 2b. It was confirmed that R for G. stearothermophilus on N95 masks can reach as high as 2.3 on average. The R on cloth masks is around 1.5, impacted by the soaking of large droplets. Face shields and coveralls were sterilized at R of 1.14 and 1.04, respectively. The R on alumina and stainless-steel surfaces is also around 1. Again, as the surfaces of the face shields, coveralls, alumina, and stainless steel are all much smoother compared with the porous masks, the droplets of the microorganism solutions are normally larger than 1 mm in diameter because of surface tension. It is expected that R in the real application when the droplet size is in the micrometer range can be higher as the microorganisms can be better exposed to CD. We also tested the sterilization effect of CD on Pichia pastoris SMD1163, which is another challenging Gram-positive bacterium for sterilization. The R of approximately 1.5 on most surfaces was reached. As a result, it is safe to say that CD is an effective broad-spectrum sterilization solution for the majority of surfaces. More attractively, the regular sterilization practice for G. stearothermophilus requires a 15 minute autoclave treatment at 121 °C, which is a time- and energy-consuming process that risks damaging the material because of the long-time heating. In comparison, CD is a contactless, non-chemical, and low-energy consumption method (down to 1.25 W), which makes it very promising for addressing sterilization challenges in soil, food, and other surfaces.31
Sterilization effectiveness at different atmospheres
As corona is operated in an ambient environment, the surrounding atmosphere may significantly impact its sterilization effectiveness. It is meaningful to evaluate whether injecting N2 or O2 gas can help increase sterilization effectiveness. As shown in Fig. 3a, N2 and O2 gas injected corona has comparable sterilization efficacy to air corona. The spectra data in Fig. 3b and c did show higher intensities of NO2, NO−, N2O3 as RNS and O3 as ROS, respectively. Until now, the role of RNS and ROS in sterilization has remained ambiguous. As long as the number of species is enough, it does not matter what type of corona is generated. Therefore, to reduce the cost, corona generated in the air is preferable. Humidity is another aspect that may impact the strength and sterilization effectiveness of corona. In Fig. 3a, we compared R at 50% relative humidity (RH) and 75% RH. It turned out that the R difference is minor. Therefore, the sterilization effectiveness of corona is relatively resilient against changes in gas content or humidity level. Also, the corona generated in the ambient air is more cost-effective, portable, and convenient to use, making it the best choice for daily surface sterilization applications.
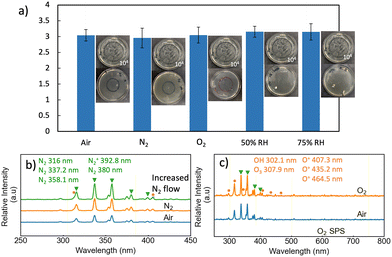 |
| Fig. 3 (a) CD sterilization effectiveness of E. coli on N95 masks at different atmospheres; (b) spectrum generated by CD under N2 flow; (c) spectrum generated by CD under O2 flow. | |
In situ air sterilization with corona discharge
To evaluate the air sterilization effectiveness of CD, we sprayed E. coli solution onto the LB plate at a distance of 30 cm, with the setup schematized in Fig. 1c. It was confirmed that when spraying E. coli solution with CD turned on, the contaminated air can be disinfected with R of approximately 0.9 (Fig. 4a and b). As it is difficult to experimentally evaluate the effective range of corona sterilization, COMOSL simulation was utilized to address this problem. As elaborated in Fig. 4c and Video 1, the corona was generated from the tip of the needle. The size of the corona increases rapidly with time until it reaches the ground substrate. The bell-shaped corona was fully established in a time as short as 10 ms, which means when needed, corona is an “instant” sterilization solution once turned on.
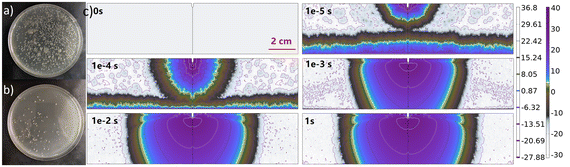 |
| Fig. 4 Cultured LB plates of E. coli-contaminated air (a) without and (b) after 7.5 minutes of CD treatment at 25 kV and 0.05 mA; (c) the electron density distribution map changed over time after the corona was turned on. | |
When the distance between the discharge needle and the targeted surface is 3.5 cm, the covered area can have a diameter of 7 cm. With the increase in needle-surface distance, the entire size of the 3D bell-shaped corona will increase, and the surface area covered by it will increase accordingly. Also, the CD can be generated by multiple needles, thin wires, and 3D-shaped electrodes. Therefore, corona can be used as an “instant” sterilization solution for a large volume of air and large surface areas. CD can potentially overcome the problems of conventional air filters used in ventilating systems, which cannot prevent direct infection between people or require a high cost for filter replacement.
Safety concerns of corona discharge
The heat, UVC, and ozone emitted by corona are the three major health concerns when considering its daily applications for surface and air sterilization, especially when the users are present. All three of them are measured while the corona is turned on for different durations of time. Firstly, an infrared camera was utilized to evaluate the temperature change around the corona, especially on the treated surface. Based on Fig. 5a–c, the temperature changes before and after 7.5 minutes of corona treatment were very minor, indicating the heat impact of corona on the treated surfaces is negligible. Secondly, the carcinogenic UVC represents a wavelength in the range of 100 nm to 290 nm. The spectrum data in Fig. 5d shows that the UVC emission from the corona is also negligible. And the waves in the range of 290 nm to 400 nm decrease with the increase in distance from the corona. Therefore, the UVC radiation hazards are low enough to allow the user to use corona without extra protection. Lastly, the ozone emission around the corona at different distances was plotted in Fig. 5e. The ozone concentration reduces dramatically from approximately 1.5 ppm to 0 when the distance away from the center of the corona (the horizontal distance from the discharge needle) is increased from 0 to 20 mm. As simulated in Fig. 4c, it is still within the range of corona. Therefore, the ozone emission from CD is also acceptable for daily use while the user is present. Overall, the thermal, UVC, and ozone impacts of corona are all within the safe range for daily use, overcoming the limitations of UVC, ozone, and chemical treatments, as well as dry and wet heating. A portable, battery-powered, high-voltage power supply that costs $20 can provide the required voltage of 1.25 W. Therefore, compared with most other sterilization solutions, including heat, UVC, and chemical disinfectants, CD is an efficient, non-contact, low-temperature, non-toxic, and user-friendly sterilization solution that allows for daily and repeated use, leading to negligible material damage and impact on the user. No extra protection precautions are needed, which makes it more convenient and affordable for the users. It can also increase space efficiency by allowing users to share a room with the sterilization system.
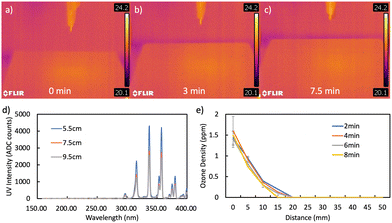 |
| Fig. 5 Corona environmental impact: heat impact on the surface (a) before the corona is turned on, after corona treatment for (b) 3 minutes and (c) 7.5 minutes; (d) UV emission spectroscopy when the horizontal detection distance ranges from 5.5 cm to 9.5 cm away. | |
Automated corona discharge robot
We designed, assembled, and programmed a foot-sized wireless CD robot capable of autonomous sterilization. The robot can move at designed speeds (e.g., 3 cm s−1) to slowly sterilize the surface. Three main Python programs assured the robot could complete all required tasks: follow a designated path, avoid static and moving obstacles, and continue back on the original path after avoiding the obstacles. To test and validate this program, an obstacle course was created (Fig. 6 and Video 2). The course was designed with many different turns and obstacles, specifically one static and one moving obstacle. Once the robot detected an obstacle with its ultrasonic rangefinder sensors, it was programmed to move around the obstacle. Once the obstacle was cleared, the robot continued along its original path. We plan on expanding this system by attaching cameras to the robot. This will allow the robot to traverse rougher terrain, more accurately scan its surroundings, and automatically adjust itself if it begins to deviate from the path and create more complex paths. The manufacturing cost of this CD robot is less than $500, which is much lower than the UVC robots that mostly cost around $800,000. When combined with the benefits of CD mentioned above, low-cost, and user-friendly CD robots have the potential to be widely used in hospitals, public areas, schools, and even homes. They can work while the users are present, significantly increasing space use and work efficiency.
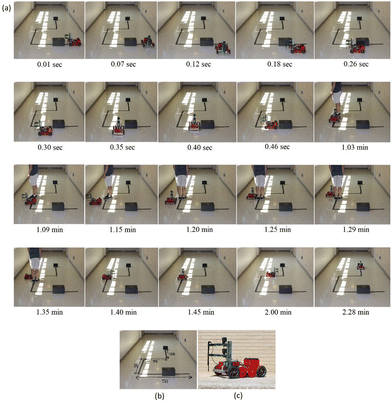 |
| Fig. 6 (a) Timed snapshots of the robot completing an obstacle course; (b) an overview of the obstacle course with measurements overlayed; (c) an isometric view of the CD robot. | |
Conclusion
This paper confirms the effectiveness of CD for broad-spectrum sterilization of different types of surfaces, including most types of PPEs and other conductive and non-conductive surfaces. The log reduction of CD on E. coli-contaminated PPE is around or higher than 3, empowering its promising application in addressing the PPE shortage, mitigating infection, and reducing white pollution. CD can also provide a non-contact, non-thermal, non-chemical, and reusable sterilization solution for all other surfaces to reduce the use of chemical disinfectants and other invasive sterilization solutions such as heat and UVC. The sterilization effectiveness of CD against E. coli, spores, and yeasts indicated the broad-spectrum sterilization capability of CD. The sterilization effect of CD is also resilient against changes in the atmosphere. CD is also effective for air sterilization. The heat, UVC, and ozone emissions of the corona are within a safe range, indicating that CD can actively decontaminate the air while people are present, thereby reducing the need for extra protection and increasing space utilization efficiency. Empowered by assembling the wireless CD components with a programmable foot-size robot, we can automatically scan the designated areas with the capabilities to avoid static and dynamic obstacles and return to its original path. By designing the CD system into automatic, portable, and handheld devices, combined with robotics or mass production solutions, we expect the CD to become a user- and environment-friendly, affordable, and convenient sterilization solution for mitigating future pandemics. CD will be a powerful alternative to conventional sterilization solutions to mitigate the spread of infectious diseases and reduce the environmental burden.
Author contributions
Sriram S. K. S. Narayanan: validation, methodology, formal analysis, investigation. Zijian Weng: validation, methodology, formal analysis, investigation, software, methodology, data curation, writing – original draft. Andrew Tenjo: methodology, investigation, writing – original draft. Marcelo Farfan: validation, investigation. Parinitha Giridharan: software. Xudong Wang: validation, methodology, Valerie Zapata: writing – review & editing. Xing Ma: writing – review & Editing. Libin Ye: conceptualization. Ehsan Sheybani: conceptualization, writing – review & editing, supervision, project administration, funding acquisition. Ying Zhong: conceptualization, resources, writing – original draft, supervision, project administration, funding acquisition.
Conflicts of interest
The authors declare that they have no known competing financial interests or personal relationships that could have appeared to influence the work reported in this paper.
Acknowledgements
All participants included in the present study and all members of our research group are greatly acknowledged. The authors would like to thank Dr Michael Celestin for his help in measuring the heat emission from corona; Dr Rob Knight, Dr Pedro Belda-Ferre, Dr Shi Huang, Dr Yu Qiao, and Dr Rui Kou at University of California at San Diego (UCSD) for their suggestions and support for this work.
References
- WHO Coronavirus (COVID-19) Dashboard, https://covid19.who.int, (accessed May 2023).
- A. Lopez and J. Srigley, Lancet, 2022, 399, 519 CrossRef CAS PubMed.
- K. A. Prather, L. C. Marr, R. T. Schooley, M. A. McDiarmid, M. E. Wilson and D. K. Milton, Science, 2020, 370, 303–304 CrossRef.
- CDC, https://www.cdc.gov/coronavirus/2019-ncov/more/science-and-research/surface-transmission.html (accessed February 2023).
- L. Owen, M. Shivkumar and K. Laird, mSphere, 2021, 6 DOI:10.1128/msphere.00316-21.
- S. B. Kasloff, A. Leung, J. E. Strong, D. Funk and T. Cutts, Sci. Rep., 2021, 11, 1–7 CrossRef.
- M. Ehsanifar, Environ. Res., 2021, 200, 111752 CrossRef CAS.
- US EPA, https://www.epa.gov/coronavirus/indoor-air-and-coronavirus-covid-19 (accessed February 2023).
-
CDC, https://www.cdc.gov/hygiene/cleaning/facility.html (accessed February 2023).
- WHO, https://www.who.int/news-room/questions-and-answers/item/coronavirus-disease-covid-19-cleaning-and-disinfecting-surfaces-in-non-health-care-settings, (accessed February 2023).
- Statista, https://www.statista.com/statistics/1104540/impact-of-coronavirus-on-drug-store-sales-values-of-health-and-hygiene-products-in-the-us/ (accessed February 2023).
- H. M. Dewey, J. M. Jones, M. R. Keating and J. Budhathoki-Uprety, ACS Chem. Health Saf., 2022, 29, 27–38 CrossRef CAS.
- J. Guo, M. Liao, B. He, J. Liu, X. Hu, D. Yan and J. Wang, J. Environ. Chem. Eng., 2021, 9, 106168 CrossRef CAS PubMed.
- A. Chang, A. H. Schnall, R. Law, A. C. Bronstein, J. M. Marraffa, H. A. Spiller, H. L. Hays, A. R. Funk, M. Mercurio-Zappala, D. P. Calello, A. Aleguas, D. J. Borys, T. Boehmer and E. Svendsen, Morb. Mortal. Wkly. Rep., 2020, 69, 496–498 CrossRef CAS PubMed.
- S. Koksoy Vayisoglu and E. Oncu, Int. J. Clin. Pract., 2021, 75, e14534 CAS.
- P. Srivastava, S. Dhyani, M. A. Emmanuel and A. S. Khan, Environ. Sustainability, 2021, 4, 649–670 CrossRef CAS.
- Y. Peng, P. Wu, A. T. Schartup and Y. Zhang, Proc. Natl. Acad. Sci. U. S. A., 2021, 118, e2111530118 CrossRef CAS.
- J. H. Kang, E. J. Kim, J. H. Choi, H. K. Hong, S. H. Han, I. S. Choi, J. Kim, J. Y. Kim, E. S. Park and P. G. Choe, Am. J. Infect. Control, 2021, 49, 713–720 CrossRef PubMed.
-
K. K. Padmanabhan and D. Barik, in Health Hazards of Medical Waste and Its Disposal, ed. D. Barik, Woodhead Publishing, Sawston, United Kingdom, 2019, 1st edn, ch. 8, pp. 99–118 Search PubMed.
- Centers for Disease Control and Prevention, https://www.cdc.gov/infectioncontrol/guidelines/disinfection/index.html (accessed February 2023).
- M. Diab-El Schahawi, W. Zingg, M. Vos, H. Humphreys, L. Lopez-Cerero, A. Fueszl, J. R. Zahar and E. Presterl, Antimicrob. Resist. Infect. Control., 2021, 10, 1–3 CrossRef PubMed.
- C. McGinn, R. Scott, N. Donnelly, K. L. Roberts, M. Bogue, C. Kiernan and M. Beckett, Front. Rob. AI, 2021, 7, 193 Search PubMed.
- Infection Control Today, https://www.infectioncontroltoday.com/view/infection-preventionists-spearhead-sars-cov-2-vaccine-efforts-trusted-leaders (accessed February 2023).
- E. A. Nardell, Photochem. Photobiol., 2021, 97, 493–497 CrossRef CAS PubMed.
- S. S. K. S. Narayanan, X. Wang, J. Paul, V. Paley, Z. Weng, L. Ye and Y. Zhong, Environ. Sci. Technol., 2021, 55, 15351–15360 CrossRef.
-
K. H. Becker, U. Kogelschatz, K. H. Schoenbach and R. J. Barker, Non-Equilibrium Air Plasmas at Atmospheric Pressure, CRC Press, Boca Raton, 2005 Search PubMed.
- C. P. Viana Martins, C. S. F. Xavier and L. Cobrado, J. Hosp. Infect., 2022, 119, 84–117 CrossRef CAS PubMed.
- L. Wang, R. Kou, Z. Shang, Z. Weng, C. Zhu and Y. Zhong, ACS Appl. Mater. Interfaces, 2021, 13, 45966–45976 CrossRef CAS.
-
J. E. Thwaite and H. S. Atkins, in Bacillus: Anthrax; food poisoning, Medical Microbiology, ed. D. Greenwood, M. Barer, R. Slack, W. Irving, Churchill Livingstone, Edinburgh, UK, 2012, 18th edn, pp. 237–244 Search PubMed.
- T. Watanabe, S. Furukawa, J. Hirata, T. Koyama, H. Ogihara and M. Yamasaki, Appl. Environ. Microbiol., 2003, 69, 7124 CrossRef CAS PubMed.
- T. Von Woedtke, M. Laroussi and M. Gherardi, Plasma Sources Sci. Technol., 2022, 31, 054002 CrossRef.
|
This journal is © The Royal Society of Chemistry 2023 |
Click here to see how this site uses Cookies. View our privacy policy here.