DOI:
10.1039/D3SU00075C
(Critical Review)
RSC Sustainability, 2023,
1, 746-762
Bio-based agricultural products: a sustainable alternative to agrochemicals for promoting a circular economy
Received
2nd March 2023
, Accepted 16th April 2023
First published on 21st April 2023
Abstract
The renewable and sustainable nature of bio-based products has made them increasingly popular in commercial and industrial applications. Bio-based products are not only environmentally friendly, but they also offer economic benefits, promoting sustainability and creating opportunities for businesses and consumers. As a result, bio-based products have become an appealing choice in the agrochemical market. In this review, we present the latest research findings on bio-based agricultural products as viable substitutes for agrochemicals, along with an overview of the current state of the agrochemicals market. The review's main objective is to highlight a range of bio-based products and their applications, with a particular focus on the potential of waste streams to drive the development of a circular economy. We observe that integrating biowaste into the circular economy can significantly boost the production of sustainable bioproducts and bioenergy, ultimately contributing to the fulfillment of the United Nations' Sustainable Development Goal #12 of promoting sustainable consumption and production.
Sustainability spotlight
This review looks at the potential of bio-based products to support the transition to a sustainable bioeconomy. It examines the current state of bio-based agro solutions, their prospective benefits and applications, and the challenges that need to be addressed to maximize their potential. The sustainability spotlight covers UN SDG (SDG 12: sustainable consumption and production), sustainable and green chemistry and circular economy principles. Certainly, bio-based waste streams offer a wide range of possibilities for developing a global front-line circular economy.
|
1. Introduction
Due to competing goals, agricultural practices have become increasingly challenging in recent years. The need to feed the world's growing population while using less arable land requires increased efficiency, while also promoting organic and sustainable practices that create “bio” products, which have grown in popularity among buyers despite their sometimes poorer aesthetic quality.1 Lawmakers must consider the negative effects of conventional and synthetic agrochemicals, such as the risk of residues to consumers and environmental concerns such as biodegradability and threats to biodiversity. Some bio-based products could serve as safer replacements for currently used agrochemicals, while others could complement them effectively.
Increasing demand for energy and chemicals as a result of population growth and development is prompting the search for alternate resources.2 The rapid depletion of fossil reserves, natural gas, and oil sources leads to an increase in price and the discharge of greenhouse gases (GHGs). A variety of organic waste (OW) can be used to produce bioenergy and chemicals, and scientists are attempting to develop novel and viable recycling technologies to address these issues. These initiatives seek to reduce garbage going to landfills while balancing supply and demand. Agro-industries, municipalities, food processing firms, and the dairy industry are all potential sources of OW.3 It's vital to remember that by polluting the environment, these contaminants can impair human and ecological health. As a result, biorefineries may be a viable option for producing bio-based goods from OW. Biofuels, biopolymers, biopesticides, biofertilizers, and biosurfactants are some of the bioproducts that can be produced from OW. These bioproducts could be made using multistep sequential methods. Most research has been conducted on anaerobic digestion and OW fermentation to produce bioenergy and biochemicals.4,5 The biorefinery process seeks and promotes the decrease of global GHG emissions by effectively recycling OW into bioproducts.6
Every year, 100 billion tons of biomass resources are produced.7 The amount of agricultural residue produced is not tracked systematically, and the amounts stated are estimated depending on the yield of the crop harvested. The waste residue is frequently carried to dump sites to decompose and/or be burned after harvesting. Dumping, on the other hand necessitates space.8 As these leftovers degrade, they take up space that could otherwise be used for something else. Conversely, burning agricultural waste produces superfluous carbon dioxide (CO2) and frequently results in the emission of pollutants such as polycyclic aromatic hydrocarbons and dioxins. Nonetheless, this trash could be both useful and profitable.9 Agricultural waste leftovers are also a good source of carbohydrates and can thus be used as a viable feedstock for the development of bio-based products. The second method of turning agricultural waste into something useful is better because it is viable for the environment and makes crops last longer. However, to maximize the quality of the resulting bio-based products, intensive washing and pretreatment of agricultural feeds are typically needed. During the washing and pre-treatment processes, the chemical makeup of the agricultural feedstock can change, depending on the method used.10
Biobased treatment is becoming more popular as a good alternative to physicochemical treatments for producing organic products out of waste.11 Some types of biowaste can be burned directly as fuel; however, this generates pollution in the air when it is carried out. As a result, it is vital to transform biowaste into other high-value products, such as biofuel. Product planning, strategy modifications, product life extension, waste management, and the expansion of the market for auxiliary materials such as food, pulverization, development, organic goods, and biohydrogen are all part of the circular economy's growth.12 In this review, biobased processes that use lignocellulosic waste and chemicals that can be made from biowaste in biorefineries have been summed up. The relevance of recent regulations that may hinder the growth of the circular economy due to their effects on the utilization of lignocellulosic waste has also been explained. The objective of this review is to identify a variety of bio-based products and energy sources, as well as their applications. Table 1 presents a comparison between the current review and previously published reviews.
Table 1 Comparison of topics in this review with previously published reviews on biobased agro solutionsa
Comparative analysis |
Circular economy significance |
Practical implications |
Patent landscape |
Perspective |
Reference |
√ – included.
|
√ |
√ |
|
|
√ |
13
|
√ |
√ |
|
|
|
14
|
√ |
√ |
|
|
√ |
15
|
√ |
√ |
√ |
|
√ |
16
|
√ |
√ |
√ |
√ |
√ |
Present |
2. Biological waste in the context of the global economy
The increase in population, urbanization, and industrialization, as well as alterations in consumption patterns, all contribute to the huge volume of municipal biomass waste. According to the data provided by the World Bank, the world generates 2.01 billion tonnes of municipal solid waste every year, with at least 33% of that not being managed in an environmentally safe manner. Waste generated per person per day in the world averages 0.74 kilograms but varies greatly, ranging from 0.11 to 4.5 kilograms. Despite having only 16% of the world's population, high-income countries generate approximately 34% of the world's waste, or 683 million tonnes. It is worth mentioning that biomass from trash will account for 19% in high-income countries by 2050 and increase to 40% or more for countries with middle- and low-income ranges.17 The countries that make up the Organization for Economic Cooperation and Development (OECD) are responsible for producing 533.8 kg per capita of the world's municipal solid waste.18
Methods of thermochemical transformation, including gasification, combustion, and pyrolysis, have been employed to improve understanding of the process by which non-food biomass assets can be converted into a variety of forms.19 In developing and developed nations, converting biomass to biofuel can be performed using a variety of biochemical and thermochemical processes. A few examples of thermochemical processes are combustion, pyrolysis, gasification, and liquid catalytic cracking. An example of a biochemical process is esterification and anaerobic digestion.20 Thus, biowaste has a lot of potential to be converted into valuable products.21 Biopesticides could be produced using the digestate of biowaste. Solid fermentation is used in the manufacturing of biobased commodities such as biosurfactants, hydrolytic chemicals, and biopesticides.22 Having a suitable reactor structure is critical for creating a modern solid-form fermentation method for biopesticide formulations based on biowaste digestate.23 Biopolymers, including biocosmetics, biopharmaceuticals, biochemicals, bionutrients, biofertilizers, and biomaterials, can be produced by combusting, gasifying, or aging biomass.24 Chemical methods such as ammonia pressurization and depressurization or acid–base pretreatment can be employed to reduce the price of enzymes before hydrolysis.25 Processes such as pyrolysis, combustion, and gasification, in addition to linked digestion, are examples of these types of reactions.26
3. Bio-based products and renewable energy
The term “bio-based products” is sometimes misunderstood by the general public to indicate chemicals, materials, or things that neither hurt nor affect the environment. Companies use the word “bio-based” to market their products, interpreting the term to mean “without affecting the environment”. By one interpretation of the term “bio-based products”, these are wares that have been manufactured using non-exhaustible primary materials obtained from food or farming businesses.27 Bio-based products, according to the European Union (EU) Commission, are those that are manufactured entirely or partially from biological sources, as opposed to those that are anchored in geological formations or have been fossilized. In the Farm Security and Rural Investment Act of 2002, the United States (US) secretary of agriculture classified bio-based products as follows: a commercial or industrial product (other than food or feed) that is made up entirely or in part of biological products, renewable domestic agricultural materials (including plant, animal, and marine materials), forestry materials, or an intermediate feedstock is referred to as a “bio-based product”. The US Department of Agriculture started the bio preferred program in 2002. Its goal is to get more people to buy and use bio-based products. Agriculture spray adjuvants, compost activators, and energizers as well as pest control products such as dethatchers, erosion management, and foliar sprays are some of the goods that belong under this parasol area.28,29
Bio-based products will affect agricultural commodity economics, politics, and trade all across the world. An abundance of information on novel conversion methods, cutting-edge technology, and creative applications of these products is fueling the revolution that is taking place in the use of agricultural raw materials in the production of chemicals, bioenergy, polymers, and other new products.30 This revolution is being powered by information and the information can be found in a plethora of various online sources. The National Association of State Universities and Land-Grant Colleges and Experiment Station Committee on Organization and Policy in their report “A science roadmap for agriculture” stressed the importance of creating “a large technical data source that producers, shippers, exporters, rural communities, government agencies, and institutions can use to make informed decisions”.31 Based on the above discussion, the representation of the divisions of biobased products is depicted in Fig. 1.
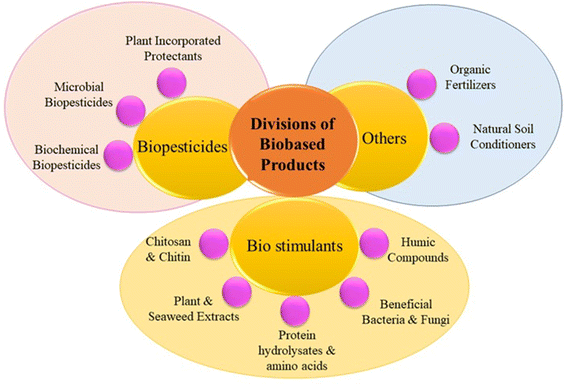 |
| Fig. 1 Schematic representation of the divisions of biobased products. Redrawn from ref. 29. | |
Bio-based raw materials are already utilized to make a huge range of industrial products, such as biomaterials, fuels, and biochemicals. These products can be placed into three main groups which are biomaterials, energy fuels, and bio-chemicals as depicted in Fig. 2.
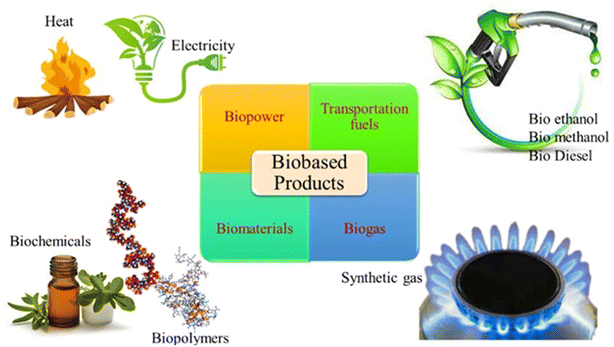 |
| Fig. 2 Schematic representation of biobased products. | |
3.1 Biomaterials
The production of biomaterials is possible using a wide variety of biomass resources.32,33 These include whole plants, parts of plants (such as seeds and stalks), other plant parts (such as starch, lipids, proteins, and fiber), processed byproducts (such as distillers' grains and maize solubles), marine materials (such as chitin, seaweed, and so on), and animal byproducts (e.g., gelatin, albumen, etc.). Before being used in the production of biomaterials there is a possibility that these resources could be utilized “as is” in the production of new biomaterials; however, it is more likely that they will be treated. A thorough understanding of the composition of raw materials whether they are whole plants, plant components, or byproducts will be necessary to obtain the desired functional elements for biomaterial manufacturing. This fundamental understanding will allow for the recovery of other potentially usable components (biofuels and biochemicals) from these raw materials, which is equally important.34
3.2 Energy fuels
For a variety of reasons, biomass is an appealing energy source. One of the major advantages of biomass energy is that it is renewable. Unlike fossil fuels, biomass energy is generated from renewable sources such as plants, agricultural waste, and forestry residues. This means that biomass energy is not depleted over time, unlike fossil fuels. In addition to this, biomass is more widely dispersed over the world than conventional sources of energy which are limited in quantity, and it may be utilized with technology that is friendlier to the environment.35,36 Biomass can improve energy self-sufficiency on a global scale, at all levels of government, including the community, regional, and national levels. By converting raw materials, also known as feedstocks, into a form that can be used, it is possible to extract the energy that is contained in the biomass. Biochemical or thermochemical techniques are used to create transportation fuels from biomass. Ethanol, methanol, biodiesel, biocrude, and methane are examples of biofuels.37
3.3 Biochemicals
Biobased chemicals are molecules that are used in commercial and industrial biobased products and are generated from biomass feedstocks.38 Biobased chemicals are derived from plant and animal sources as well as microbial sources and are renewable alternatives to petroleum-based chemicals. Common biobased chemicals include bioalcohols, such as ethanol and methanol, and bio-derived acids, such as lactic and succinic acid. Biobased chemicals are used in many industries, such as pharmaceuticals, cosmetics, food, and agriculture. Biobased chemicals have a number of advantages over their petroleum-based counterparts, such as being more sustainable, having fewer environmental impacts, and being more cost-effective. Furthermore, biobased chemicals are often biodegradable, making them a more environmentally friendly option.
Bioconversion of OW into value-added chemicals offers a lot of potential as a replacement for their traditional counterparts.39 According to the National Research Council (NRC), biobased industrial products would account for significant industrial production, respectively, compared to existing levels for liquid fuels, organic compounds, and materials.40 The use of biobased products and bioenergy will increase several fold.41
Probably, the fact that nobody knows how long the world's easily accessible petroleum resources will survive is the single most serious problem surrounding the current situation of our transportation fuel.42 Domestic petroleum resources in the US are insufficient to supply the country's energy demands. Nearly two-thirds of the oil reserves that have been discovered on the planet are located in the region that surrounds the Persian Gulf. In addition, the US gets the majority of its oil from the Persian Gulf, which accounts for more than 50% of the country's total oil consumption. Oil imports are responsible for more than half of the US trade deficit, and they have a considerable influence on both the growth of the economy and the number of jobs that are created. A nation's economy is also subject to price increases due to supply disruptions as a result of a large trade imbalance resulting from reliance on foreign energy. By creating a better market for biofuels made in the US, it is possible to reduce the negative effects of trade deficit and help the US transportation sector grow economically.43,44
4. The significance of agrochemicals in modern agriculture
Agrochemicals are chemicals used in agriculture to improve crop production. They are used to protect crops from pests, diseases, and weeds, as well as to promote growth and increase yield. Agrochemicals can include insecticides, fungicides, herbicides, and fertilizers, as well as growth regulators, hormones, and nutrient supplements.45 Agrochemicals are essential for modern agriculture, as they help maximize crop yields and reduce the impacts of pests and diseases. They play an important role in increasing food production, and their use has been linked to higher yields and improved quality. They can also help reduce the environmental impacts of modern agriculture, such as soil erosion and water pollution.
The current crop production strategy is built around two pillars: fertilizer use and chemical protection. The addition of a third pillar – bio-products – might be important to examine.46 Agriculture has recently morphed into a technical endeavor. Mineral fertilizers are used extensively in field crops as well as comprehensive chemical plant protection. Comprehensive chemical plant protections are widely used, as they provide essential nutrients for plants and can help reduce the risk of pest and disease infestations. Soil degradation may result because of this. Degraded soils are not a good place for plants to grow. This will have the opposite impact of what is anticipated: a decrease in production yield. It is crucial to keep in mind that soil has its own unique ecosystem, which calls for the maintenance of a balance among its mineral, organic, and biological components. Soil also contains a variety of organic matter, such as decaying plant and animal remains, which is used as a source of nutrition for the organisms in the soil. The biological components of soil play a key role in maintaining soil fertility, as they promote nutrient cycling and soil structure. Crop production would be impossible without the use of fertilizers.47 Harvested crops must be used to replenish the constituents extracted from soil. Plant cultivation is challenging today without the usage of extensive chemical protection. Furthermore, agriculture has come to rely on these preparations. Resistant pests to synthetic agrochemicals have become a problem. Antibiotic resistance in pathogenic bacteria is an issue comparable to one that has just been uncovered in human medicine. This necessitates the development of new agricultural products.48
Bio-products are any naturally occurring goods that are not only completely biodegradable but also non-toxic to both plants and consumers. Bio-products come in a range of different forms. These include plant-based products such as essential oils, beeswax and honey; animal-based products such as wool, leather and feathers; and microbial-based products such as enzymes and probiotics. Bio-products are increasingly being used in various industries, from cosmetics and food to textiles and fuel. The environmental benefits of bio-products make them an attractive option for businesses looking to reduce their carbon footprint and embrace sustainable practices. In addition to being environmentally friendly, bio-products are often less expensive than their synthetic counterparts and can provide superior performance. As a direct consequence of this, there are no problems associated with toxicity or ecotoxicity, hazardous residues, environmental fate, or behavior. The operator of the dispensing agent is safe with these formulae. Bio-products are produced by extracting or homogenizing microorganisms or macroorganisms.49 Semiochemicals are complementary components of insecticide approaches in various integrated pest management strategies.50 Semiochemical chemicals, such as a semiochemical biopesticide for the control of the cotton pest Helicoverpa spp., are substances that are used to communicate across species.51 Biopesticides, biostimulants, and biofertilizers are the three types of agro bio-products. They are distinct in terms of their intended function and mode of action. Biopesticides are biological agents used to control pests and diseases, caused by viruses, bacteria, fungi, or insects. Biostimulants are materials that enhance plant growth and development, such as certain hormones, enzymes, or vitamins. Biofertilizers are organic materials that supply nutrients to plants, such as manure, compost, or fish meal. Their distinct roles are crop protection, enhancement, and nutrition.
4.1 Biopesticides
Pesticides can be replaced by biopesticides, which are derived from materials such as bacteria, fungi, baculoviruses, and nematode-associated bacteria that fight invertebrate pests in agricultural ecosystems.52 They work by interfering with the life cycle of pests, such as by introducing predators or parasites that feed on the target pest, by producing natural toxins, or by competing with the pest for food or habitat. An example of a biopesticide is Bacillus thuringiensis.53 Additionally, pest control strategies such as crop rotation, intercropping, and mechanical cultivation can be employed to reduce the need for chemical pesticides. These biopesticides are effective even in low doses, although their therapeutic window is quite narrow. Pheromones form as a result of chemicals released into the environment that benefit both the receiver and the sender.54 A pheromone-based bio-insecticide, for example, that is aggressive in stopping the spread of a specific insect-pest species is only effective against that pest species.55 Other pests aren't affected by it. It is vital to remember that synthetic chemicals are dangerous xenobiotics, even though they offer a far broader spectrum of activity.56 In this regard, biopesticides are preferable. One example of this would be using biofungicides to prevent powdery mildew from spreading on cucurbit rootstock seedlings.57 An occurrence of powdery mildew on Punica granatum was first observed in Italy in June 2015. Better disease management strategies are suggested to prevent yield losses.58 Chemical pesticides can be used to protect plants; however, their effectiveness and resistance can be compromised over time, as pests become resistant to chemicals. Additionally, there are environmental risks associated with chemical pesticides, as they can pollute soil and water, and some can harm beneficial insects. Furthermore, the usage of chemical pesticides has the potential to disrupt the natural balance as well as human health. These pesticides can be toxic to both beneficial and harmful insects, aquatic life, and humans. In addition, the long-term use of chemical pesticides can lead to the development of insect populations that are resistant to chemicals, rendering pesticides ineffective. Biopesticides are a viable option in this regard. Biopesticides are compounds that are used to protect plants from pests.59 Usually, biopesticides are non-toxic to the environment, and microorganisms have a lower resistance to them.60,61 Although there are challenges, the microbial biopesticide market has plenty of room for growth in agriculture and public health.62
4.1.1 Microbial biopesticides.
Endophytic bacteria, which are bacteria that grow on plants but do not harm the plant, can be used as a biopesticide because they can either assist in combating infections by creating antibiotics or induce resistance by releasing bioactive metabolites.63 Plant bacteria with biocontrol potential include Pseudomonas, Bacillus, and Streptomyces.64,65 Additionally, some of these bacteria are able to promote plant growth by producing hormones and other compounds that help the plant absorb nutrients, increase root growth, and improve water and nutrient uptake from the soil. Bacteria are the microorganisms that prevent diseases from spreading and causing disease in their hosts. For example, biosurfactants and lytic enzymes can all be synthesized in the lab. Rhizobiotic bacteria can compete for resources such as nutrition or the environment, and in some cases, they can outcompete native bacteria. Rhizobiotic bacteria can also provide beneficial services to the host, such as nitrogen fixation. It makes use of the host plant to fix atmospheric nitrogen and convert it into useful organic compounds, which benefits both the bacteria and the plant. The bacteria can also help improve soil fertility and reduce the need for costly fertilizer applications.66 Other bacteria, such as plant pathogens, can be controlled with better adaptation. Bacteria can also operate as bioinsecticides, in addition to biofungicides and bio bactericides.59 Microbiological insecticides, such as those based on Bacillus thuringiensis, are the most widely used bio-insecticides. It is popular because it is a natural product, has low toxicity to non-target organisms, and is effective against a wide range of pests.67 Furthermore, Bacillus thuringiensis strains account for approximately 90% of the biopesticide market.68
A recent study shows that antifungal capabilities are possessed by a strain of Bacillus safensis bacteria inaccessible from the fruiting tree osmanthus. Both in vitro and in vivo tests were carried out to see how well these antifungal abilities worked. B. safensis produced activities that were harmful to cells as well as those that limit the growth of bacteria and fungus as a result of its synthesis of metabolites such as antibiotics and biosurfactants. These activities were also capable of preventing the growth of bacteria and fungi.69
4.1.2 Biochemical pesticides.
Microbes, as well as the substances they create, can influence other, often harsh, microorganisms. Considering the influence of the wheat-borne fungus Magnaporthe oryzae Triticum on the antifungal properties of oligomycin B and oligomycin F, both of which are produced by the bacteria, the growth of fungal mycelium was inhibited by both oligomycins. In comparison to oligomycin B, oligomycin F inhibited fungus hyphal development more effectively. They completely inhibited the fungal mycelium from forming conidia on an agar plate when they were applied. Compounds of oligomycin considerably cut down on fungal conidia germination and significantly improved lysis. They also halted the spread of wheat blast disease.70 It has been demonstrated that the amount of bioactive secondary metabolites produced by an endophytic fungus has a positive impact on agriculture. Different taxa have been studied to see if compounds produced by the endophytic fungal strain Fusarium sambucinum are effective.71,72
Both plants and microorganisms are sources of important secondary metabolites that have the potential to act as biopesticides and biotherapeutics. Flavonoids, quinines, phenols, tannins, coumarins, alkaloids, saponins, terpenoids, and sterols are examples of metabolites. Plant secondary metabolites such as terpenoids and flavonoids can act as herbicides, insecticides, fungicides, and nematicides. Microbial secondary metabolites such as peptides and antibiotics can be used to control insects, weeds, fungi, and bacteria. Both plants and microorganisms can also produce metabolites that can be used to produce drugs and other therapeutic compounds. Plant chemicals have antifeedant, repellant, growth regulator, and poisonous properties that are hazardous to insects. Furthermore, the biopesticide actions of chemicals from a variety of plants affect not just insects but also hazardous microbes. In a study, the potato tuber moth, Phthorimaea operculella, was exposed to as many as 12 different ethanol plant extracts while they were being stored. Zygophyllum, colocynth, solanum, arnoglosse, jasmine, coriander, senna, geranium, basil, chamomile, harmel, and mint extracts were studied. Some of them prevented potato tuber infestation or lowered the total number of eggs that were laid. Others impose a limit on the number of eggs a single female can lay. Others made an effort to reduce the total number of adult specimens by delaying the hatching of larvae from eggs for as long as they could.73
In a recent study, the biopesticide function of plant chemicals was documented. The essential oils of two different plants, known respectively as summer savory and lesser calamint, were used. Caryophyllene, terpinene, carvacrol, p-cymene, and thymol acetate are some of the components that contribute to the savory flavor. Calamine contains piperitone oxide, limonene, cisperitone epoxide, and terpinen-4-ol. The most prevalent types of bacteria investigated were Pseudomonas, Xanthomonas, Erwinia, Agrobacterium, Clavibacter, Enterobacter, Bacillus, and Ralstonia. It was discovered that the natural oils from these plants might inhibit the growth of these bacteria. Calamint oil exhibited a stronger antibacterial impact than savory oil, even though both had an inhibitory effect on phytopathogenic microorganisms.29
4.1.3 Plant-based antioxidants.
It is possible to change the genes of farmed plants so that they are less likely to be eaten by insects. This can be carried out through genetic modification, which involves introducing new genes to the plant. This is in addition to the administration of exogenous biopesticides, which is another viable option. To combat hazardous insects, it is feasible to inject deoxyribonucleic acid (DNA) from soil bacteria into the insects to make them harmless. This type of biopesticide, called a microbial insecticide, takes advantage of the bacteria's natural ability to produce toxins that harm insects. A recent study reveals that agrobacterium could transform the callus of a soybean cultivar by using materials from Bacillus thuringiensis. This led to the creation of eight transgenic lines of soybeans, each of which expressed genes similar to cry8 and produced harmful proteins. The soybean plants were found to be more resistant to Coeloptera insects, in insect feeding trials than the unmodified plant. The highest concentration of Cry8 was discovered in soybean leaves, which were used to feed adult insects. When dangerous insects are larvae and feed on the roots of altered species of plants, they have a high fatality rate.74 In another study, it was observed that Cry1Ab/Ac, a Bacillus thuringiensis endotoxin, affects genes produced in jute plants. Also, Agrobacterium tumefaciens was used to transfer the genetic material, resulting in five lines of resistant jute. The plants' tissues displayed varying levels of cry endotoxin expression to varying degrees depending on the tissue.75
4.2 Biostimulants
Biostimulants are a type of bio-based substance used in agriculture. Biostimulants are chemical compounds and other types of substances that, when applied to plants, seeds, or other parts of the plant's growth cycle in the form of a solution, assist the plant in enhancing its capacity for growth, development, and stress resistance. Microorganisms have a bioaugmentation impact, as shown in Fig. 3. Biostimulation factors are frequently found in nature and are made up of a variety of bio-stimulating ingredients. What's more, their action is based on the use of very low doses. After application to the surface of a plant or soil, a stimulating effect may be obtained.76 Biostimulants are compounds that assist plants in coping with abiotic stress, such as high salt levels or drought, generated by the environment. Because of the wide variety of roles that natural plant products play in agricultural production, they can help reduce the quantity of man-made chemical compounds that are released into the environment. These items are thought to be environmentally friendly. Biopesticides protect the plant against biotic stress (insect attack), whereas biostimulants protect it from abiotic stress (drought). When comparing the efficiency of various agronomic components on experimental plots to control and reference plots, procedures including root mass measurement, photosynthetic intensity, and the extent of harvested quantity can be used. These are examples of methods that can be used. When abiotic stress conditions develop, biostimulants have a very apparent effect. It's possible that the alterations won't be noticed if stress isn't present. In the case of abiotic stress, biostimulants act as a form of protection for the plant.77
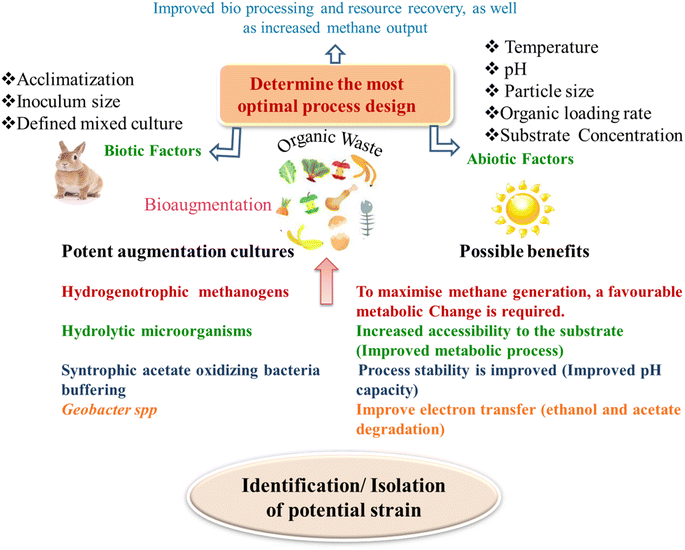 |
| Fig. 3 Schematic representation of bioaugmentation for methane production. | |
4.3 Biofertilizers
The phrase “living fertilizers” is probably the most correct way to refer to biofertilizers. Biofertilizers help increase the availability of essential nutrients, such as nitrogen and phosphorus, to plants, and they can also improve the soil structure and organic matter content. They are made from living organisms, such as bacteria, fungi, and algae, and act as a natural source of plant nutrients. Biofertilizers can also help reduce the need for chemical fertilizers by providing a sustainable alternative. They are beneficial for both the environment and for farmers, as they can increase crop yields, reduce costs and help reduce soil erosion and water contamination caused by chemical fertilizers. Mycorrhizal fungi are one example that could be employed as biofertilizers.78 This makes it possible for plants to receive nitrogen even when a nitrogen fertilizer has not been applied directly to them. The use of mycorrhizal fungi has been shown to boost soil productivity and produce quality while lowering environmental costs.78 Another example is phosphate biofertilizers, which are soil-based phosphate solubilizing bacteria that dissolve phosphorus soil deposits that are unavailable to plants and make them into a form that is usable for the plants. This type of fertilizer helps the plants take up more phosphorus from the soil and improve their growth.79
5. Pretreatment of organic waste before its conversion into bioproducts
To weaken the cell walls that are present in organic materials, pretreatment of OW is required. This process, which started in the early 1920s with the treatment of lignocellulosic biomass with steam and acid for the production of bioenergy, has been around for a long time. There is a range of pretreatment methods available, as indicated in Fig. 4 including mechanical, thermal, physiochemical, and biological. Although OW pretreatment is widely regarded as the most costly phase, it is also the most critical for enhancing the bioproduct output. To produce bioproducts, decentralized pretreatment and a centralized biorefinery of treated OW may prove to be the most cost-efficient approaches. The physical, chemical and biological properties of OW are altered during the pretreatment process.80 This makes it possible for OW to be easily converted into a wide variety of bioproducts, while also improving the efficiency of the process and lowering the costs of downstream processing and the biorefinery as a whole. Pretreatment should change microbial surface qualities and break down bigger OW molecules into simple monomers.81 By reducing the size of the initial feed material and altering the structural and physical characteristics of OW, mechanical and physical pretreatment procedures can increase bulking density and decrease the volume to surface ratio. The surface area and pore size of OW are indeed improved by mechanical and physical pretreatments, enabling more rapid microbial conversion to a variety of bioproducts.82 These approaches, however, are still energy-intensive. High-pressure homogenization is the most popular biomass pretreatment method.83 On the one hand, chemical treatments are used to prevent OW and are based on the use of chemical agents that disrupt OW.
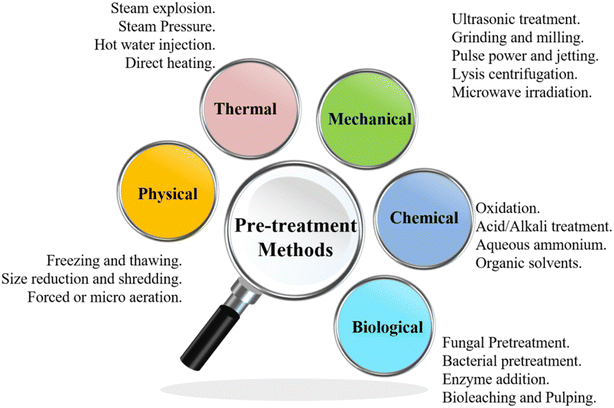 |
| Fig. 4 Schematic representation of various pretreatment methods. | |
Thermal pretreatment technologies are also considered for OW pretreatments because they are cost-effective and environmentally benign. They are composed of a range of processes that can be separated into two categories depending on the structural characteristics of OW, which are dry OW (torrefaction) and wet OW (hydrothermolysis). The torrefaction process is a thermal one that depolymerizes and dries OW at temperatures between 200 and 300 °C with or without the presence of ambient nitrogen.84 Hydrothermolysis is performed at 150°C–210 °C for a period (10–20 minutes), which accelerates the rate of OW degradation.85
6. Commercial implementation of bioproducts: potential and challenges
A few bio-products are commercially available. The oldest is a seed biofungicide based on Bacillus subtilis that works against Fusarium, Rhizoctonia, Alternaria, and Aspergillus fungal infections.86 Only 0.1% of the created formulations have been placed on the market, even though bio-based products provide new potential, are fully safe to use, and have been studied for more than 50 years. The reasons for this are complex, but they can be summed up as follows: the current climate of the agrochemical market; the registration procedures; and the distinct operational mechanisms that distinguish these products from conventional agrochemicals. Bio-products, unlike chemically manufactured products, lack a defined active component with definable properties. There are several compounds in bio-products that work together synergistically. However, the precise mechanism of action of bio-products is still unknown.87
From the moment the active ingredient is discovered until it is released into the market, it is predicted that it will take 10 years. Product development and registration research can cost tens of millions of euros, if not hundreds of millions. The purpose of limited registration procedures was to learn as much as possible about the qualities of the substance before it was released on the market. The perception that has been created, however, is quite different. A tiny number of biologically active substances are available on the agrochemical market. The majority of these chemicals were created more than 30 years ago. There aren't enough innovative products on the market. The US, Japan, and China are top three countries that produce agrochemicals, with China producing 90% of the world's agrochemicals.88 Small and medium-sized firms in other nations find it difficult to acquire entry into the agrochemical market because of this stance. As a result, bio-product manufacturers began to band together to form consortia. The European Biostimulant Industry Council was established to improve bio-product registration procedures based on their uniqueness. In the US, this issue has been mostly resolved, with the time between the discovery of a substance and its registration reduced from ten to three years. Simultaneously, the costs of product development were dramatically cut. EU countries are still adhering to Regulation 1107/2009 on agrochemicals.89 Bio-products (biopesticides and biostimulants) must be registered to comply with the law. This includes submitting a “registration dossier” to the appropriate European agencies, such as the European Food Safety Authority. The dossier includes a summary of study findings on physicochemical parameters. Additionally, documentation on toxicity, ecotoxicology, environmental fate and behavior, and residues must be submitted. Examining the properties of these bio-products, on the other hand, is pointless because they do not pose a threat to ecotoxicology. Reports on agronomic effectiveness should also be available through the application. Within the registration dossier is the characterization of a validated analytical methodology. This methodology is used to determine the active component in the product, as well as in plants, and in the environment.
7. Advancements in biowaste refining & circular economy's significance
Throughout the twentieth century, biowaste was used to make a variety of industrial products. Biowaste refining is the process of turning waste into bioproducts that can be used in better ways in the near future. This is different from using fossil fuels, which are a resource that does not regenerate on its own. Several different biobased along with thermal revolution methodologies can be utilized to turn different types of biowaste into viable forms of biofuel.90 By enhancing the productivity of specific biowaste-consuming bacteria, methods for delivering higher-quality bioproducts that are required for bioeconomy firms to be financially sustainable can be developed.
An integrated biorefinery gives us a once-in-a-lifetime chance to bring a whole manufacturing division back to life by making huge amounts of chemicals, energy, pharmaceuticals, and energy from a renewable resource. This is a once-in-a-lifetime opportunity that will not be repeated. Historically, chitosan was extracted from shrimp shells, which are a waste product of the fishing industry.91 Other sources of chitosan have now been discovered. The synthesis of methane from CO2-rich gases is critical to modern economies. This is because the creation of methane from CO2 rich gases has an advantageous effect on lowering global warming potential as well as the consumption of energy sources that are not renewable. In addition, the infrastructure for producing methane that already exists in many countries has made it possible to produce methane from gases that are rich in CO2.
7.1 Anaerobic digestion/co-digestion produces bioenergy
Sustainable biogas production by anaerobic fermentation, such as biohydrogen and biomethane, has recently received a lot of attention.92 It has been demonstrated that the biowaste solid-state anaerobic digestion of yard waste (YW) is a feasible method for the production of bioenergy. Biowaste co-digestion with YW microwave pretreatment can produce a substantial amount of methane (431 mL g−1 V−1) at a food to microorganism ratio of around 1.5.93 The growth of attapulgite could increase methane generation by 8.9–37.3% while lowering the amount of energy needed to manufacture methane. It is possible to produce an additional 210.4 mL of methane per gram of volatile solids when attapulgite is combined with an expansion stacking of 10 g L−1.94
The authors noted that although a six-day hydraulic retention time (HRT) resulted in the highest hydrogen generation of 0.676 mol H2 per mol of carbs eaten, a three-day HRT is required for acidogenic fermentation of dairy products to minimize instability caused by lactate aggregation. Even though a three-day HRT is required, this is the case. Pasteurization of slaughterhouse waste had little impact on anaerobic digestion, even if increasing the nutritional ratio of dairy products boosted the methane output in individual schemes (up to 37.6% growth). Surprisingly, anaerobic digestion was not suppressed even at the highest ammonia concentrations (about 4 g L−1).16 In another study, the authors observed that co-digesting waste with waste-activated sludge can result in a steady-state methane generation rate of up to 0.27 N m3 kg−1 volatile solid fed when the organic loading rate is equal to 1.7 gVS per Ld. This can be accomplished by mixing trash with the sludge.95
7.2 Composting/co-composting
It is possible to increase the monetary worth of waste biomass by composting it, as well as sterilizing & stabilizing it, and reducing its mass through the process of composting. Composting is a process by which an organic material is decomposed and recycled as a natural fertilizer and soil amendment. The compost can be used as a soil amendment to improve soil fertility, improve soil structure, and reduce soil erosion. Composting also helps reduce the environmental impact of waste biomass by reducing the amount of waste sent to landfills. Additionally, composting can be used to generate energy, either through anaerobic digestion or by burning the compost in a power plant. Composted biomass can be used as a source of biogas, which can be used to generate electricity, heat, and other forms of energy. The incorporation of biochar during the composting and vermin composting of biowastes would result in an improvement in the physicochemical properties of the compost mixture. On the other hand, natural matter biodegradation and microbial activities result in a reduction in nitrogen loss and GHG emissions.96
7.3 Hydrothermal and thermochemical technologies
The manufacture of hydrogen containing syngas from trash is commonly accomplished by combining two well-established processes: hydrothermal carbonization (HTC) and gasification. Gasifier tar from hydrochars has half the value of biowastes, but the amount of hydrogen and methane in syngas increases. In the meantime, this transition from biowaste to fuel encourages gasification and makes it possible to create similar advancements in a variety of fields. These include an improvement in syngas quality brought using hydrochars and a reduction in the amount of gasified tar arrangement by hydrochar.16 When aromaticity increases, the apex of the change value in the HTC method is pushed to a higher temperature. The production of fuel with a greater hydrogen to carbon ratio can be accomplished by the process of water gasification, which replaces the drying of biomass with the use of water as a reaction solvent and makes use of water as a reaction solvent. And, hydrogenation, also known as hydrogasification, is the substitution of hydrogen for carbon to produce a fuel with a higher hydrogen-to-carbon (H/C) ratio. After HTC, biowastes' energy characteristics are comparable to those of lignite or even bituminous coal. The conversion of –C–H/C–O to aromatic –C–C/CC is beneficial for fuel combustion. The percentage of a fuel that is composed of biomass is directly correlated to the amount of carbon that can be converted via co-gasification. The overall amount of hydrogen via co-gasification is greater than what was anticipated based on the gasification outcomes of the fuels that were investigated.16 The carbonaceous assemblage in hydrochars could be converted into bitumite or even anthracite when HTC temperatures were elevated from 120 to 300 °C. At both the laboratory and pilot scales, a thorough investigation of the integrated process is required. The lower heating value of biodiesel could increase from 36
080 to 36
992 kJ kg−1, and the cetane number increases from 47.7 to 58.4.16
The terms “circular economy” and “bioeconomy” are often used interchangeably. Both of these concepts are rolled into one under the umbrella phrase “circular economy”, with the focus being placed on bioproducts and biofuels to differing degrees. The viability of the circular bioeconomy is in jeopardy.16,97 Not only in the US, but also in countries all over the world, the concept of a circular economy is getting significant support from policymakers and business leaders. Some of the most important barriers are (1) the large environmental and social impacts of landfilling, (2) the strong dependence of national economies and (3) the rapid expansion of business strategies to urban residents that compete with conventional recycling industries. In recent years, there has been a lot of interest in a biowaste refinery as a potential biorefinery alternative. The process of generating high-value bioproducts and bioenergy from biowaste is known as biorefining. This process is typically carried out in a facility known as a biowaste refinery, as shown in Fig. 5.
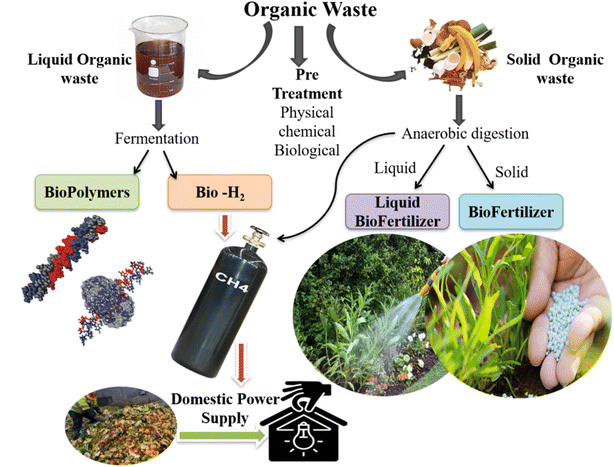 |
| Fig. 5 Biowaste refinery to generate high-value bioproducts and bioenergy. | |
Several parties involved in the value chain, from those involved in product design to those involved in waste disposal, must be thoroughly aware of the ramifications of using biomass if they are to construct a circular bioeconomy. The model was found to have benefited financially from environmental tax money. 1 unit change in gross domestic product (GDP) will increase environmental quality by 0.52 units.98 In other words, the amount of money collected from environmental taxes is a key indicator of economic growth due to the fact that these taxes have a positive correlation with the growth of the economy.99 In the EU, municipal garbage recycling rates were found to be high, and this had a positive impact on GDP per capita.100 As a surrogate for both social and economic implications, this variable was chosen. As a consequence of this, the socioeconomical aspects of the recycling economy have statistical significance and are of the utmost significance for the expansion of the economy.101
8. Practical implications
Sustainable agriculture seeks to achieve a balance between environmental stewardship, economic profitability, and social and economic equity. To achieve this balance, farmers must adopt sustainable practices that ensure the protection of natural resources and the health of the environment while also providing economic opportunities to their communities. Sustainable agriculture, green chemistry, and the recovery and cradle-to-cradle re-use of macronutrients from digestate derivatives can all advance together. Macronutrients, such as nitrogen, phosphorus, and potassium, are essential for crop growth and development. By recovering macronutrients from digestate derivatives, such as manure, municipal wastewater, and food waste, the need for chemical fertilizers can be reduced. Additionally, these macronutrients can be reused in the form of fertilizers, soil amendments, and soil conditioners.102 This reduces the amount of nutrients lost through runoff and leaching, ultimately helping to reduce water pollution. This also promotes sustainable nutrient management and reduces the need for additional chemical inputs. Finally, by reusing macronutrients in a cradle-to-cradle fashion, it can help reduce the amount of waste that is sent to landfills and incinerators, which can help reduce GHGs.
For example, concentrates from reverse osmosis membrane filtration have the potential to be green N–K fertilizers, whereas wastewater from acidic air scrubbers has the potential to be a green N–S fertilizer.96 Particularly for livestock farmers, the salt content, sodium adsorption ratio, and potassium content could be significant barriers to the agricultural re-use of concentrates. The pH, salt concentration, and caustic qualities of acidic air scrubber water could constitute bottlenecks for agricultural re-use. Theoretically, substituting synthetic fertilizers with acidic air scrubber water or membrane filtration concentrates will always produce substantial economic and environmental benefits for the farmer. These new green fertilizers are currently being evaluated in the field to determine their effect on soil and crop production.
The circular economy concepts of a biorefinery are exerting a significant influence on global economic development, with particular emphasis on the biowaste circular economy. This model is well-suited to address the increasing need for eco-friendly management of organic materials. Given its inherent properties, biowaste is rich in energy content. The vast majority of times, the contributions of social scientists to circular economy survey are limited to directing approach conflicts. Consolidation of current efforts on administrative adjustments and policies related to the circular economy is imperative to determine their potential for facilitating the shift towards sustainability.103 A subjective evaluation is suggested to establish the criteria and limitations required for developing a feasible supply chain in the provincial bioproduct circular economy. However, factors such as the novelty of pertinent technical limitations, the accessibility of financial resources, and the preferences of end-users create challenges in obtaining circular economy benefits and increasing the carbon efficiency of industrial operations. To advance circular economy ethics and policy, citizen involvement, collaborative strategies, and the participation of numerous firms are essential.96,104
9. Patent landscape
Patent landscape analysis is carried out for biomass as biogas, biomass as energy resources, biomass as biopolymesr, biomass as biochemicals and biomass as biofuel (Tables 2–4). The total count is 253 as per the record for the keyword EN_ALL: (“biomass production” “energy resource”). The total count is 498 as per the record for the keyword EN_ALL: (“biomass production” “biogas”). The total count is 486 as per the record for the keyword EN_ALL: (“biomass production” “biopolymer”). The total count is 3381 as per the record for the keyword EN_ALL: (“biomass production” “ biochemical”). The total count is 1829 as per the record for the keyword EN_ALL: (“Biomass production” “Biofuel”). In all the above cases, the option was enabled to filter the same patent filed in multiple countries.
Table 2 Patent landscape based on year
Year |
Energy resource |
Biogas |
Biopolymer |
Biochemical |
Biofuel |
As on 28 Feb 2023.
|
2014 |
17 |
43 |
30 |
232 |
174 |
2015 |
17 |
50 |
36 |
197 |
153 |
2016 |
1 |
24 |
35 |
197 |
125 |
2017 |
7 |
19 |
17 |
153 |
110 |
2018 |
4 |
30 |
25 |
127 |
98 |
2019 |
5 |
30 |
32 |
144 |
107 |
2020 |
10 |
34 |
27 |
128 |
82 |
2021 |
9 |
29 |
29 |
123 |
87 |
2022 |
13 |
36 |
38 |
134 |
104 |
2023a |
4 |
9 |
8 |
24 |
12 |
Table 3 Patent landscape based on IPC
International patent classification (IPC) code |
Energy resource |
Biogas |
Biopolymer |
Biochemical |
Biofuel |
A01G |
— |
— |
— |
— |
89 |
A01H |
82 |
34 |
66 |
751 |
221 |
A01N |
21 |
— |
48 |
241 |
— |
A01P |
12 |
— |
— |
— |
— |
A61K |
— |
— |
26 |
234 |
— |
B01D |
— |
43 |
— |
— |
|
C02F |
— |
103 |
31 |
— |
86 |
C05F |
11 |
24 |
— |
— |
— |
C07H |
— |
— |
— |
216 |
— |
C07K |
66 |
33 |
58 |
678 |
171 |
C10B |
11 |
— |
— |
— |
— |
C10G |
— |
— |
— |
— |
88 |
C10L |
18 |
40 |
39 |
— |
160 |
C12M |
17 |
124 |
33 |
222 |
325 |
C12N |
149 |
169 |
234 |
2305 |
937 |
C12P |
19 |
165 |
157 |
942 |
531 |
C12Q |
— |
|
— |
186 |
— |
C12R |
— |
20 |
28 |
148 |
92 |
Table 4 Patent landscape based on country
Country |
Energy resource |
Biogas |
Biopolymer |
Biochemical |
Biofuel |
EPO – European Patent Office.
PCT – Patent Co-operation Treaty.
|
Australia |
— |
4 |
6 |
20 |
7 |
Canada |
— |
4 |
11 |
37 |
49 |
China |
— |
4 |
— |
— |
4 |
EPOa |
3 |
20 |
18 |
55 |
34 |
Finland |
— |
1 |
— |
— |
— |
German |
— |
2 |
— |
— |
— |
India |
4 |
15 |
22 |
62 |
41 |
Israel |
— |
— |
— |
3 |
— |
Netherlands |
— |
— |
1 |
— |
— |
New Zealand |
— |
— |
— |
4 |
— |
PCTb |
182 |
278 |
269 |
1835 |
937 |
Philippines |
— |
— |
1 |
— |
2 |
South Africa |
— |
— |
4 |
36 |
— |
Republic of Korea |
— |
— |
— |
— |
4 |
UK |
2 |
4 |
5 |
6 |
10 |
USA |
62 |
159 |
148 |
1314 |
735 |
The patent landscape analysis shows that the growth of biomass production-based biosolutions is remarkably significant. Year-wise consolidation reveals that there is consistent growth by numbers, especially in particular areas and further analysis needs to be conducted.105 When using the IPC system to classify patents based on their technical subject matter, researchers can effectively narrow their search to focus on a specific area of interest. For instance, by focusing on the “C12N” international patent classification code, researchers can limit their search to patents related to microorganisms. C12N specifically pertains to patents and patent applications concerning the extraction, cultivation, alteration, and exploitation of microorganisms, along with enzymes and other biologically active compounds that originate from these microorganisms. Most biomass derivatives consist of microorganisms, enzymes, and their combinations. The country wherein more extensive research is conducted is the US, as evidenced by its position as the leader of the entire derivative.
10. Conclusions and future perspectives
To achieve both environmental sustainability and public health, it is imperative to invest in appropriate waste management infrastructure and promote responsible waste management practices. Bio-based products have the potential to revolutionize modern agriculture by providing sustainable and eco-friendly alternatives to traditional chemical-based products. However, significant legislative barriers hinder their entry into the market. One of the key challenges is the registration process, which aims to ensure the safety and efficacy of products sold to farmers. While traditional chemical-based products are relatively easy to register, with a well-defined active ingredient that can be easily tested and evaluated, bio-products often contain complex mixtures of naturally occurring compounds, making it more challenging to identify a specific active ingredient. To overcome this challenge, regulatory agencies need to develop new registration requirements that consider the unique nature of bio-products. This may involve implementing more flexible testing protocols that can accommodate the complexity of these products, as well as adopting new approaches to risk assessment that recognize the inherent variability in natural products. Another critical factor is the need for greater education and outreach to farmers and other stakeholders. Many farmers may be hesitant to adopt bio-products due to a lack of understanding about their benefits and how they work. By providing more information and resources, regulatory agencies can help build confidence in these products and encourage their widespread adoption.
The future of bio-products in modern agriculture looks bright. With the right regulatory frameworks in place, these innovative products can help support sustainable farming practices and promote a healthier environment for all. By promoting the use of bio-products in agriculture, we can contribute to reducing GHG emissions, enhancing soil health and fertility, and reducing our dependence on traditional chemical-based products. The emergence of new technologies based on renewables offers exciting opportunities for bioeconomic transformation. By leveraging these innovations, we can make significant strides towards achieving the United Nations' Sustainable Development Goal #12 of promoting sustainable consumption and production.
Abbreviations
CO2 | Carbon dioxide |
DNA | Deoxyribonucleic acid |
EU | European Union |
EPO | European patent office |
GDP | Gross domestic product |
GHG | Greenhouse gas |
H/C | Hydrogen-to-carbon ratio |
HRT | Hydraulic retention time |
HTC | Hydrothermal carbonization |
IPC | International patent classification |
NRC | National research council |
OECD | Organization for economic cooperation and development |
OW | Organic waste |
PCT | Patent co-operation treaty |
SDG | Sustainable Development Goal |
US | The United States |
YW | Yard waste |
Conflicts of interest
There are no conflicts to declare.
References
- A. Muller, C. Schader, N. El-Hage Scialabba, J. Brüggemann, A. Isensee, K.-H. Erb, P. Smith, P. Klocke, F. Leiber and M. Stolze,
et al.). Strategies for feeding the world more sustainably with organic agriculture, Nat. Commun., 2017, 8, 1290, DOI:10.1038/s41467-017-01410-w
.
- S. E. Ibitoye, T.-C. Jen, R. M. Mahamood and E. T. Akinlabi, Densification of agro-residues for sustainable energy generation: an overview, Bioresour. Bioprocess., 2021, 8, 75, DOI:10.1186/s40643-021-00427-w
.
- B. Koul, M. Yakoob and M. P. Shah, Agricultural waste management strategies for environmental sustainability, Environ. Res., 2022, 206, 112285, DOI:10.1016/j.envres.2021.112285
.
- L. C. Ampese, W. G. Sganzerla, H. Di Domenico Ziero, A. Mudhoo, G. Martins and T. Forster-Carneiro, Research progress, trends, and updates on anaerobic digestion technology: A bibliometric analysis, J. Cleaner Prod., 2022, 331, 130004, DOI:10.1016/j.jclepro.2021.130004
.
- S. Y. Lee, R. Sankaran, K. W. Chew, C. H. Tan, R. Krishnamoorthy, D.-T. Chu and P.-L. Show, Waste to bioenergy: a review on the recent conversion technologies, BMC Energy, 2019, 1, 4, DOI:10.1186/s42500-019-0004-7
.
- K. Huang, X. Peng, L. Kong, W. Wu, Y. Chen and C. T. Maravelias, Greenhouse Gas Emission Mitigation Potential of Chemicals Produced from Biomass, ACS Sustainable Chem. Eng., 2021, 9, 14480–14487, DOI:10.1021/acssuschemeng.1c04836
.
- A. I. Osman, M. Farghali, I. Ihara, A. M. Elgarahy, A. Ayyad, N. Mehta, K. H. Ng, E. M. Abd El-Monaem, A. S. Eltaweil and M. Hosny,
et al.). Materials, fuels, upgrading, economy, and life cycle assessment of the pyrolysis of algal and lignocellulosic biomass: a review, Environ. Chem. Lett., 2023 DOI:10.1007/s10311-023-01573-7
.
- A. M. Abdulaziz, Planning in a dynamic society: A case of Makkah, Saudi Arabia, J. Adv. Res. Dyn. Control Syst., 2020, 12, 105–110, DOI:10.5373/JARDCS/V12SP1/20201052
.
- M. Xu, M. Yang, H. Sun, M. Gao, Q. Wang and C. Wu, Bioconversion of biowaste into renewable energy and resources: A sustainable strategy, Environ. Res., 2022, 214, 113929, DOI:10.1016/j.envres.2022.113929
.
-
L. Tabil, P. Adapa and M. Kashaninejad, Biomass Feedstock Pre-Processing – Part 1: Pre-Treatment, in Biofuel's Engineering Process Technology, ed. M. A. dos Santos Bernardes, IntechOpen, 2011 Search PubMed
.
- F. Fazzino, A. Pedullà and P. S. Calabrò, Boosting the circularity of waste management: pretreated mature landfill leachate enhances the anaerobic digestion of market waste, Biofuel Res. J., 2023, 10, 1764–1773, DOI:10.18331/BRJ2023.10.1.2
.
- A. T. Ubando, W.-H. Chen, D. A. Hurt, A. Conversion, S. Rajendran and S.-L. Lin, Biohydrogen in a circular bioeconomy: A critical review, Bioresour. Technol., 2022, 366, 128168, DOI:10.1016/j.biortech.2022.128168
.
- P. Sharma, V. K. Gaur, R. Sirohi, S. Varjani, S. H. Kim and J. W. C. Wong, Sustainable processing of food waste for production of bio-based products for circular bioeconomy, Bioresour. Technol., 2021, 325, 124684 CrossRef CAS PubMed
.
- S. A. Afolalu, E. Y. Salawu, T. S. Ogedengbe, O. O. Joseph, O. Okwilagwe, M. E. Emetere, O. O. Yusuf, A. A. Noiki and S. A. Akinlabi, Bio-Agro Waste Valorization and its Sustainability in the Industry: A Review, IOP Conf. Ser.: Mater. Sci. Eng., 2021, 1107, 12140, DOI:10.1088/1757-899X/1107/1/012140
.
- P. D. Cuadrado-Osorio, J. M. Ramírez-Mejía, L. F. Mejía-Avellaneda, L. Mesa and E. J. Bautista, Agro-industrial residues for microbial bioproducts:
A key booster for bioeconomy, Bioresour. Technol. Rep., 2022, 20, 101232, DOI:10.1016/j.biteb.2022.101232
.
- A. Jain, S. Sarsaiya, M. Kumar Awasthi, R. Singh, R. Rajput, U. C. Mishra, J. Chen and J. Shi, Bioenergy and bio-products from bio-waste and its associated modern circular economy: Current research trends, challenges, and future outlooks, Fuel, 2022, 307, 121859, DOI:10.1016/j.fuel.2021.121859
.
-
A. Demirbas, Trends in Solid Waste Management, https://datatopics.worldbank.org/what-a-waste/trends_in_solid_waste_management.html Search PubMed
.
-
OECD, Municipal Waste, 2023, DOI:10.1787/89d5679a-en, accessed on 26 February 2023.
-
Thermochemical Conversion Processes, in Biofuels: Securing the Planet's Future Energy Needs, Springer, London, 2009, pp. 261–304 Search PubMed.
- K. Adekunle and J. Okolie, A Review of Biochemical Process of Anaerobic Digestion, Adv. Biosci. Biotechnol., 2015, 6, 205–212, DOI:10.4236/abb.2015.63020
.
- E. Álvarez-Castillo, M. Felix, C. Bengoechea and A. Guerrero, Proteins from agri-food industrial biowastes or co-products and their applications as green materials, Foods, 2021, 10, 981 CrossRef
.
- A. Cerda, L. Mejias Torrent, P. Rodriguez, A. Rodriguez, A. Artola, X. Font Segura, T. Gea and A. Sánchez, Valorisation of digestate from biowaste through solid-state fermentation to obtain value added bioproducts: A first approach, Bioresour. Technol., 2019, 271, 409–416, DOI:10.1016/j.biortech.2018.09.131
.
-
X. Ge, J. Vasco-Correa and Y. Li, 13 - Solid-State Fermentation Bioreactors and Fundamentals, in Current Developments in Biotechnology and Bioengineering, ed. C. Larroche, M. Á. Sanromán, G. Du and A. Pandey, Elsevier, 2017, pp. 381–402 Search PubMed
.
- M. Hassan, J. Bai and D.-Q. Dou, Biopolymers; Definition, Classification and Applications, Egypt, J. Chem., 2019, 62(9), 1725–1737, DOI:10.21608/EJCHEM.2019.6967.1580
.
- Y. Zheng, Y.-S. Cheng, C. Yu, R. Zhang, B. M. Jenkins and J. S. VanderGheynst, Improving the efficiency of enzyme utilization for sugar beet pulp hydrolysis, Bioprocess Biosyst. Eng., 2012, 35, 1531–1539, DOI:10.1007/s00449-012-0743-z
.
-
R. P. Kumar, E. Gnansounou, J. K. Raman and B. Gurunathan, Refining Biomass Residues for Sustainable Energy and Bioproducts: Technology, Advances, Life Cycle Assessment, and Economics, Academic Press, 2019 Search PubMed
.
- M. Pylak, K. Oszust and M. Frąc, Review report on the role of bioproducts, biopreparations, biostimulants and microbial inoculants in organic production of fruit, Rev. Environ. Sci. Bio/Technol., 2019, 18, 597–616 CrossRef CAS
.
-
C. M. Galanakis, Biobased Products and Industries, Elsevier, 2020 Search PubMed
.
-
M. Sojka and A. Saeid, Chapter 10 - Bio-Based Products for Agriculture, in Smart Agrochemicals for Sustainable Agriculture, ed. K. Chojnacka and A. Saeid, Academic Press, 2022, pp. 279–310, ISBN 9780128170366, DOI:10.1016/B978-0-12-817036-6.00001-7
.
-
T. R. K. C. Doddapaneni and T. Kikas, Thermochemical and biochemical treatment strategies for resource recovery from agri-food industry wastes, in Valorization of Agri-Food Wastes and By-Products, Elsevier, 2021, pp. 787–807 Search PubMed
.
- A Science Roadmap for Agriculture, https://agsci.oregonstate.edu/sites/agsci.oregonstate.edu/files/main/roadmap2.pdf.
- A. Haile, G. G. Gelebo, T. Tesfaye, W. Mengie, M. A. Mebrate, A. Abuhay and D. Y. Limeneh, Pulp and paper mill wastes: utilizations and prospects for high value-added biomaterials, Bioresour. Bioprocess., 2021, 8, 35, DOI:10.1186/s40643-021-00385-3
.
-
S. Irmak, Biomass as Raw Material for Production of High-Value Products, in Biomass Volume Estimation and Valorization for Energy, ed. J. S. Tumuluru, IntechOpen, 2017 Search PubMed
.
- N. Ali, Q. Zhang, Z.-Y. Liu, F.-L. Li, M. Lu and X.-C. Fang, Emerging technologies for the pretreatment of lignocellulosic materials for bio-based products, Appl. Microbiol. Biotechnol., 2020, 104, 455–473 CrossRef CAS PubMed
.
- A. E. M. Fodah, M. K. Ghosal and D. Behera, Microwave-assisted pyrolysis of agricultural residues: current scenario, challenges, and future direction, Int. J. Environ. Sci. Technol., 2022, 19, 2195–2220, DOI:10.1007/s13762-020-03099-9
.
- Y. Tian and H. Zhang, Producing biogas from agricultural residues generated during phytoremediation process: Possibility, threshold, and challenges, Int. J. Green Energy, 2016, 13, 1556–1563, DOI:10.1080/15435075.2016.1206017
.
- R. A. Diltz, H. R. Luckarift and G. R. Johnson, Sustainable land use for bioenergy in the 21 st century, Ind. Biotechnol., 2011, 7, 437–447, DOI:10.1089/ind.2011.0019
.
- Biobased Chemicals, https://www.acs.org/greenchemistry/research-innovation/biobased-chemicals.html.
- S. Chavan, B. Yadav, A. Atmakuri, R. D. Tyagi, J. W. C. Wong and P. Drogui, Bioconversion of organic wastes into value-added products: A review, Bioresour. Technol., 2022, 344, 126398 CrossRef CAS PubMed
.
-
National Research Council, Biobased Industrial Products: Priorities for Research and Commercialization, The National Academies Press, Washington, DC, 2000, DOI:10.17226/5295
.
-
T. Mehmood, F. Nadeem, S. A. Qamar, M. Bilal and H. Iqbal, Bioconversion of agro-industrial waste into value-added compounds, in Sustainable Bioconversion of Waste to Value Added Products, Springer, 2021, pp. 349–368 Search PubMed
.
-
L. Lopez, F. Alagna, L. Bianco, I. De Bari, C. Fasano, F. Panara and G. Perrella, Plants: a sustainable platform for second-generation biofuels and biobased chemicals, in Handbook of Biofuels, Elsevier, 2022, pp. 47–72 Search PubMed
.
-
A. Duarte, Introduction to the Citrus Waste Valorisation, 2019, pp. 7–20 Search PubMed.
-
V. Pérez Martínez, A. Pascual, A. Rodrigo, M. García Torreiro, M. Latorre Sánchez, C. Coll Lozano, A. D. Moreno, J. M. Oliva Dominguez, A. Serna Maza and N. Herrero García, Integrated Innovative Biorefinery for the Transformation of Municipal Solid Waste into Biobased Products, 2020 Search PubMed
.
-
J. G. Speight, Chapter 4 - Sources and Types of Organic Pollutants, in Environmental Organic Chemistry for Engineers, ed. J. G. Speight, Butterworth-Heinemann, 2017, pp. 153–201 Search PubMed
.
- N. Dhakal and B. Acharya, Syngas Fermentation for the Production of Bio-Based Polymers: A Review, Polymers, 2021, 13, 3917 CrossRef CAS PubMed
.
-
P. S. Kumar, A. Saravanan, R. Jayasree and S. Jeevanantham, Food industry waste biorefineries, in Refining Biomass Residues for Sustainable Energy and Bioproducts, Elsevier, 2020, pp. 407–426 Search PubMed
.
-
M. K. Mann, M. J. Biddy, C. R. Augustine, Q. Nguyen, H. Hu, M. Ebadian and E. Webb, Evaluation of Agricultural Equipment Manufacturing for a Bio-Based Economy, National Renewable Energy Lab. (NREL), Golden, CO (United States), 2019 Search PubMed
.
- M. Kordi, R. Salami, P. Bolouri, N. Delangiz, B. Asgari Lajayer and E. D. van Hullebusch, White biotechnology and the production of bio-products, Syst. Microbiol. Biomanuf., 2022, 1–17 Search PubMed
.
- N. El-Ghany, Semiochemicals for controlling insect pests, J. Plant Prot. Res., 2019, 59, 1–11, DOI:10.24425/jppr.2019.126036_rfseq1
.
- R. Mensah, C. Moore, N. Watts, M. Deseo, P. Glennie and A. Pitt, Discovery and development of a new semiochemical biopesticide for cotton pest management: Assessment of extract effects on the cotton pest Helicoverpa spp, Entomol. Exp. Appl., 2014, 152(1), 1–15, DOI:10.1111/eea.12198
.
- L. Ruiu, Microbial Biopesticides in Agroecosystems, Agronomy, 2018, 8(11), 235, DOI:10.3390/agronomy8110235
.
- H. S. Salama and S. A. Salem, Bacillus thuringiensis and neem seed oil (Azadirachta indica) effects on the potato tuber moth Phthorimaea operculella zeller in the field and stores, Arch. Phytopathol.
Plant Prot., 2000, 33, 73–80, DOI:10.1080/03235400009383329
.
-
T. D. Wyatt, Pheromones and Other Chemical Communication in Animals, in Reference Module in Neuroscience and Biobehavioral Psychology, Elsevier, 2017 Search PubMed
.
- H.-L. Wang, B.-J. Ding, J.-Q. Dai, T. J. Nazarenus, R. Borges, A. Mafra-Neto, E. B. Cahoon, P. Hofvander, S. Stymne and C. Löfstedt, Insect pest manageent with sex pheromone precursors from engineered oilseed plants, Nat. Sustain., 2022, 5, 981–990, DOI:10.1038/s41893-022-00949-x
.
- Y. Zhu, A. Boye, M. Body-Malapel and J. Herkovits, The Toxic Effects of Xenobiotics on the Health of Humans and Animals, BioMed Res. Int., 2017, 4627872, DOI:10.1155/2017/4627872
.
- A. P. Keinath and V. B. DuBose, Controlling powdery mildew on cucurbit rootstock seedlings in the greenhouse with fungicides and biofungicides, Crop Prot., 2012, 42, 338–344, DOI:10.1016/j.cropro.2012.06.009
.
- S. Pollastro, D. Gerin, S. Marullo, M. de miccolis and F. Faretra, First report of Erisiphe sp. as a causal agent of powdery mildew on Punica granatum L, in Italy, Plant Dis., 2016, 100(9), 1949, DOI:10.1094/PDIS-03-16-0267-PDN
.
- M. Ahmad, L. Pataczek, T. H. Hilger, Z. A. Zahir, A. Hussain, F. Rasche, R. Schafleitner and S. Solberg, Perspectives of microbial inoculation for sustainable development and environmental management, Front. Microbiol., 2018, 9 DOI:10.3389/fmicb.2018.02992
.
- L. Bhatia, P. K. Sarangi, A. K. Singh, A. Prakash and K. P. Shadangi, Lignocellulosic waste biomass for biohydrogen production: future challenges and bio-economic perspectives, Biofuels Bioprod. Biorefin., 2022, 16(3), 838–858 CrossRef CAS
.
-
M. Razia, V. U. M. Nallal and S. Sivaramakrishnan, Agro-based sugarcane industry wastes for production of high-value bioproducts, in Biovalorisation of Wastes to Renewable Chemicals and Biofuels, Elsevier, 2020, pp. 303–316 Search PubMed
.
- O. K. Opender Koul, Microbial biopesticides: opportunities and challenges, CABI Rev., 2011, 1–26 Search PubMed
.
- I. Afzal, Z. K. Shinwari, S. Sikandar and S. Shahzad, Plant beneficial endophytic bacteria: Mechanisms, diversity, host range and genetic determinants, Microbiol. Res., 2019, 221, 36–49, DOI:10.1016/j.micres.2019.02.001
.
- A. Alrobaian, V. Rajasekar and A. Alagumalai, Critical insight into biowaste-derived biocatalyst for biodiesel production, Environ. Prog. Sustainable Energy, 2020, 39, e13391, DOI:10.1002/ep.13391
.
- D. Fira, I. Dimkić, T. Berić, J. Lozo and S. Stanković, Biological control of plant pathogens by Bacillus species, J. Biotechnol., 2018, 285, 44–55, DOI:10.1016/j.jbiotec.2018.07.044
.
- M. E. Hibbing, C. Fuqua, M. R. Parsek and S. B. Peterson, Bacterial competition: surviving and thriving in the microbial jungle, Nat. Rev. Microbiol., 2010, 8, 15–25, DOI:10.1038/nrmicro2259
.
-
L. de Souza and S. Shivakumar, Conversion of Agro-industrial Wastes for the Manufacture of Bio-based Plastics, in Bioplastics for Sustainable Development, Springer, 2021, pp. 177–204 Search PubMed
.
-
N. Moazami, 3.61 - Biological Control, in Comprehensive Biotechnology, ed. M. Moo-Young, Pergamon, 3rd edn, 2019, pp. 772–784 Search PubMed
.
- S. Rong, H. Xu, L. Li, R. Chen, X. Gao and Z. Xu, Antifungal activity of endophytic Bacillus safensis B21 and its potential application as a biopesticide to control rice blast, Pestic. Biochem. Physiol., 2020, 162, 69–77, DOI:10.1016/j.pestbp.2019.09.003
.
- M. Chakraborty, N. U. Mahmud, A. N. M. Muzahid, S. M. F. Rabby and T. Islam, Oligomycins inhibit Magnaporthe oryzae Triticum and suppress wheat blast disease, PLoS One, 2020, 15, 1–16, DOI:10.1371/journal.pone.0233665
.
- T. Bouslama, I. Chaieb, A. Rhouma and A. Laarif, Evaluation of a Bacillus thuringiensis isolate-based formulation against the pod borer, Helicoverpa armigera Hübner (Lepidoptera: Noctuidae). Egypt, J. Biol. Pest Control, 2020, 30, 16, DOI:10.1186/s41938-020-00218-z
.
- R. M. K. Toghueo, Bioprospecting endophytic fungi from Fusarium genus as sources of bioactive metabolites, Mycology, 2020, 11, 1–21, DOI:10.1080/21501203.2019.1645053
.
- A. M. F. Sharaby, M. A. Gesraha and S. A. B. Fallatah, Botanical extracts against the potato tuber moth, Phthorimaea operculella (Zeller 1873) (Lepidoptera: Gelechiidae), during storage conditions, Egypt. J. Biol. Pest Control., 2020, 30, 93, DOI:10.1186/s41938-020-00294-1
.
- D. Qin, X.-Y. Liu, C. Miceli, Q. Zhang and P. Wang, Soybean plants expressing the Bacillus thuringiensis cry8-like gene show resistance to Holotrichia parallela, BMC Biotechnol., 2019, 19, 66, DOI:10.1186/s12896-019-0563-1
.
- S. Majumder, K. Datta, S. Satpathy and S. K. Datta, Development and evaluation of lepidopteran insect resistant jute expressing the fused Bt-Cry1Ab/Ac toxin driven by CaMV35S promoter, Ind. Crops Prod., 2020, 156, 112873, DOI:10.1016/j.indcrop.2020.112873
.
- L. Yang, X.-C. Wang, M. Dai, B. Chen, Y. Qiao, H. Deng, D. Zhang, Y. Zhang, C. M. V. B. de Almeida and A. S. F. Chiu, Shifting from fossil-based economy to bio-based economy: Status quo, challenges, and prospects, Energy, 2021, 228, 120533 CrossRef
.
- P. R. Yaashikaa, P. S. Kumar, A. Saravanan, S. Varjani and R. Ramamurthy, Bioconversion of municipal solid waste into bio-based products: A review on valorisation and sustainable approach for circular bioeconomy, Sci. Total Environ., 2020, 748, 141312 CrossRef CAS PubMed
.
-
H. M. S. P. Madawala, Chapter 7 - Arbuscular mycorrhizal fungi as biofertilizers: Current trends, challenges, and future prospects, in Biofertilizers, ed. A. Rakshit, V. S. Meena, M. Parihar, H. B. Singh, and A. K. Singh, Woodhead Publishing, 2021, pp. 83–93 Search PubMed
.
-
G. Mohammadi, Phosphate Biofertilizers as Renewable and Safe Nutrient Suppliers for Cropping Systems: A Review BT - Probiotics and Plant Health, ed. V. Kumar, M. Kumar, S. Sharma, and R. Prasad, Springer Singapore, 2017, pp. 113–130 Search PubMed
.
-
D. Barik, Chapter 3 - Energy Extraction From Toxic Waste Originating From Food Processing Industries, in Woodhead Publishing Series in Energy, ed. D. B. T.-E. from T. O. W. for H. and P. G. Barik, Woodhead Publishing, 2019, pp. 17–42 Search PubMed
.
- D. A. Peguero, M. Gold, D. Vandeweyer, C. Zurbrügg and A. Mathys, A Review of Pretreatment Methods to Improve Agri-Food Waste Bioconversion by Black Soldier Fly Larvae, Front. Sustain. Food Syst., 2022, 5 DOI:10.3389/fsufs.2021.745894
.
-
M. N. Aftab, I. Iqbal, F. Riaz, A. Karadag and M. Tabatabaei, Different Pretreatment Methods of Lignocellulosic Biomass for Use in Biofuel Production, in Biomass for Bioenergy, ed. A. E.-F. Abomohra, IntechOpen, 2019 Search PubMed
.
-
A. A. Shah, T. H. Seehar, K. Sharma and S. S. Toor, Chapter 7 - Biomass pretreatment technologies, in Hydrocarbon Biorefinery, ed. S. K. Maity, K. Gayen and T. K. B. T.-H. B. Bhowmick, Elsevier, 2022, pp. 203–228 Search PubMed.
-
W.-H. Chen, Chapter 10 - Torrefaction, in Pretreatment of Biomass, ed. A. Pandey, S. Negi, P. Binod and C. Larroche, Elsevier, 2015, pp. 173–192 Search PubMed
.
- M. M. Gomes, C. A. B. S. Rabelo, I. K. Sakamoto, E. L. Silva and M. B. A. Varesche, Methane Production Using Brewery Spent Grain: Optimal Hydrothermolysis, Fermentation of Waste and Role of Microbial Populations, Waste Biomass Valorization, 2022, 13, 1179–1194, DOI:10.1007/s12649-021-01564-1
.
- S. Ben Khedher, B. Mejdoub-Trabelsi and S. Tounsi, Biological potential of Bacillus subtilis V26 for the control of Fusarium wilt and tuber dry rot on potato caused by Fusarium species and the promotion of plant growth, Biol. Control, 2021, 152, 104444, DOI:10.1016/j.biocontrol.2020.104444
.
- Y. Kourkoutas, K. A. G. Karatzas, V. P. Valdramidis and N. Chorianopoulos, Bioactive Natural Products: Facts, Applications, and Challenges, Biomed. Res. Int., 2015, 2015, 684109, DOI:10.1155/2015/684109
.
-
https://www.fnbnews.com/Top-News/china-produces-90-of-worlds-agrochemical-raw-materials-66813
.
-
https://eur-lex.europa.eu/legal-content/EN/TXT/?uri=CELEX%3A32009R1107
.
- S. Gajula and C. R. K. Reddy, More sustainable biomass production and biorefining to boost the bioeconomy, Biofuels Bioprod. Biorefin., 2021, 15, 1221–1232 CrossRef CAS
.
- A. M. Sixto-Berrocal, M. Vázquez-Aldana, S. P. Miranda-Castro, M. A. Martínez-Trujillo and M. R. Cruz-Díaz, Chitin/chitosan extraction from shrimp shell waste by a completely biotechnological process, Int. J. Biol. Macromol., 2023, 230, 123204, DOI:10.1016/j.ijbiomac.2023.123204
.
-
K. Stamatelatou, G. Antonopoulou and P. Michailides, 15 - Biomethane and biohydrogen production via anaerobic digestion/fermentation, in Advances in Biorefineries, ed. K. Waldron, Woodhead Publishing, 2014, pp. 476–524 Search PubMed
.
- S. Panigrahi, H. B. Sharma and B. K. Dubey, Anaerobic co-digestion of food waste with pretreated yard waste: A comparative study of methane production, kinetic modeling and energy balance, J. Cleaner Prod., 2020, 243, 118480, DOI:10.1016/j.jclepro.2019.118480
.
- Y. Liang, L. Xu, J. Bao, K. A. Firmin and W. Zong, Attapulgite enhances methane production from anaerobic digestion of pig slurry by changing enzyme activities and microbial community, Renewable Energy, 2020, 145, 222–232, DOI:10.1016/j.renene.2019.06.037
.
- B. Tonanzi, C. M. Braguglia, A. Gallipoli, D. Montecchio, P. Pagliaccia, S. Rossetti and A. Gianico, Anaerobic digestion of mixed urban biowaste: The microbial community shift towards stability, New Biotechnol., 2020, 55, 108–117, DOI:10.1016/j.nbt.2019.10.008
.
- C. Vaneeckhaute, E. Meers, E. Michels, J. Buysse and F. M. G. Tack, Ecological and economic benefits of the application of bio-based mineral fertilizers in modern agriculture, Biomass Bioenergy, 2013, 49, 239–248, DOI:10.1016/j.biombioe.2012.12.036
.
- H. L. Bos and J. Broeze, Circular bio-based production systems in the context of current biomass and fossil demand, Biofuels, Bioprod. Biorefin., 2020, 14, 187–197 CrossRef CAS
.
- M. Chen, W. Jiandong and H. Saleem, The role of environmental taxes and stringent environmental policies in attaining the environmental quality: Evidence from OECD and non-OECD countries, Front. Environ. Sci., 2022, 10 DOI:10.3389/fenvs.2022.972354
.
- M. Ma, Z. Xiao, M. Liu and X. Huang, Combining the role of green finance and environmental sustainability on green economic growth: Evidence from G-20 economies, Renewable Energy, 2023, 207, 128–136, DOI:10.1016/j.renene.2023.02.046
.
- L. Levaggi, R. Levaggi, C. Marchiori and C. Trecroci, Waste-to-Energy in the EU: The Effects of Plant Ownership, Waste Mobility, and Decentralization on Environmental Outcomes and Welfare, Sustainability, 2020, 12, 5743, DOI:10.3390/su12145743
.
- F. Gatto and I. Re, Circular bioeconomy business models to overcome the valley of death. A systematic statistical analysis of studies and projects in emerging bio-based technologies and trends linked to the SME instrument support, Sustainability, 2021, 13, 1899 CrossRef
.
- H. Bamdad, S. Papari, G. Lazarovits and F. Berruti, Soil amendments for sustainable agriculture: Microbial organic fertilizers, Soil Use Manag., 2022, 38, 94–120, DOI:10.1111/sum.12762
.
- G. Sharma, M. Kaur, S. Punj and K. Singh, Biomass as a sustainable resource for value-added modern materials: a review, Biofuels, Bioprod. Biorefin., 2020, 14, 673–695 CrossRef CAS
.
- J.-M. Arauzo-Carod, I. Kostakis and K. P. Tsagarakis, Policies for supporting the regional circular economy and sustainability, Ann. Reg. Sci., 2022, 68, 255–262, DOI:10.1007/s00168-022-01124-y
.
-
https://patentscope.wipo.int/search/en/structuredSearch.jsf, accessed on 28th Feb 2023, the keywords should be entered accordingly to get the respective data.
Footnote |
† Contributed equally. |
|
This journal is © The Royal Society of Chemistry 2023 |
Click here to see how this site uses Cookies. View our privacy policy here.