DOI:
10.1039/D2SU00071G
(Critical Review)
RSC Sustain., 2023,
1, 418-431
Devising a people-friendly test kit for overcoming challenges in the assessment of water quality and analysis of water pollution in the river Ganga
Received
11th October 2022
, Accepted 22nd March 2023
First published on 22nd March 2023
Abstract
The Ganga is the most sacred Indian river, and it influences the lifestyle of ∼400 million people. However, owing to several anthropogenic activities, biotic and abiotic pollutants have increased substantially. The water quality assessment of the Ganga river has indicated that the water is not potable along most of its stretch. Most of the pollutants originate from a lack of responsible waste dumping from industrial, farming, sewage sectors and religious activities and cremation practices. The level of dissolved oxygen and biological oxygen could be the reason for serious concerns about aquatic life and human health risks. In this context, the challenges associated with formulating a standard testing kit for the Ganga water from people friendly viewpoint and utilising their knowledge base is presented. Overall identified data from available sources have been classified in terms of sources, such as industrial, farming, various religious activities, cremation practices, and their possible effects on aquatic life and human health. The present level of pollution from all sources and accepted standards are discussed. Further, we have highlighted the need for understanding pollution from the people's perspective involved in various activities and postulated the information regarding the sensors that have been developed to identify a variety of pollutants in the water.
Sustainability spotlight
Water is one of the most exquisite parts of life that is required for proper sustentation and function of our body. Degraded water quality can destructively affect human health. Having your water tested before consumption makes impeccable sagacity that can save thousands of lives. Ganges is a major source of water in India, and the water quality assessment of the river Ganga will provide knowledge to empower decisions and behaviour of people towards its purity. This review provides an overview of the contamination scenarios, its causes, and detection methods available to identify the water quality index of the most sacred river of India, i.e., “Ganga”. The work aligns to target the SDGs clean water and sanitation (SDG6).
|
1. Introduction
Ganga is among the largest rivers of the Indian sub-continent, which originates from the Gaumukh ice cave of the Gangotri Glacier and drains into the Bay of Bengal while traversing about 2525 km through Uttarakhand, Uttar Pradesh, Bihar, Jharkhand, and West Bengal.1,2 The lives of more than 400 million people depend on this river for their daily needs. It is also considered as the most sacred river in India, embodied as a living goddess and symbolized by Mother Ganga. It is believed to purify the soul and has been reported to have rich medicinal and antibacterial properties.3–5 In India, it covers around 861
404 km2 of the drainage basin and almost 26.2% of the geographical area that includes the major ancient cities including Haridwar, Kanpur, Allahabad, Varanasi, Patna, and Kolkata. Starting from its origin in Gaumukh to Rishikesh, it flows on the Himalayan hills, thereafter, it reaches the Gangetic plain.6 While traveling from hills to plain, Ganga unites with its tributaries, i.e., Yamuna, Ghaghara, Gandak, Kosi, Burhi Gandak, Gomati, Tons, Sone, and Punpun. While these tributaries increase the volume of water in the Ganga, they also bring several polluting elements that deteriorate its water quality. The stretch of the river flowing from Kannauj to Varanasi is intensely vulnerable due to abiotic sources of pollution such as industrial discharge, manure release, and agricultural runoff. Thus, due to the increased level of pollution, the consumption of Ganga water is reported to cause hazardous health effects such as water-borne diseases and cancer. Several studies have reported the deterioration in water quality and increased pollution levels by analyzing the physico-chemical, biological, and toxicological features of river Ganga.2,7–9
Industrial discharge has increased the level of inorganic and organic pollutants in the river. Inorganic pollutants such as metals like cadmium, iron, lead, chromium, mercury, and zinc have been released from electroplating industries and thermal power plants.10–14 Organic waste, chlorinated organics, and dioxins have entered the river due to effluents discharged from pulp and paper mills. The petrochemical industry is also responsible for discharging a lot of oils and phenols. Additionally, the Gangetic plane is among the most fertile agricultural land in the country; therefore, large amounts of pesticides that are used for agriculture also end up in the river due to runoff.15,16 Most of the pollutants present in the river, either organic or inorganic, are known to be carcinogens and mutagens in nature and can cause numerous biological disorders.17,18 Due to the high prevalence of these metals in the river, it gets accumulated in fish via biomagnification.8,11,19–21 Several other toxic elements are also biomagnified and bioaccumulated by aquatic animals.11,19–21 The accumulation of toxins by aquatic animals can cause significant health risks to the human population consuming sea foods. One study by the National Cancer Registry Program has reported that the number of cancer patients (especially gall bladder cancer) has augmented around the Ganga basin.22 The monitoring of the Ganga river's water quality has been mostly carried out by checking the physico-chemical characteristics and maximum count of fecal coliform present.23
The government of India has taken several steps to control the level of pollution in the Ganga,24 such as the Ganga Action Plan (GAP) launched in 1986 to reduce sewage discharge directly into the river, the establishment of the National Ganga River Basin Authority (NGRBA) to clean and conserve the Ganga under the Section 3(3) of Environment Protection Act, 1986. Nevertheless, after so many years, the quality of the Ganga did not improve as expected and continues to deteriorate. Here, we have reviewed the various pollution sources classified into biotic and abiotic factors that negatively affect the water quality of the Ganga river. Secondly, we reviewed the various detection methods available for water quality monitoring.24 Further, we argue for the need for a people-friendly test kit to identify and monitor the existing water quality for real-time action and awareness among people.
2. The Ganga pollution and the adverse health hazard to its inhabitants
Ganga is the largest river basin of India. The main river stream of Ganga homesteads a high density of population. The negligence in maintaining the hygiene level, effluents from industries, household waste, and irrigation runoff turns Ganga into a polluted river. The section here explains the sources responsible for pollution in the Ganga river along with the hazardous effects it can cause to living beings.
2.1 Sources
Around 20% of wastewater discharge in the Ganga emerges from industrial sources.25 However, it is enough to elevate the toxin levels in the river. A major part of industrial pollution, around 55% source is from Uttar Pradesh, followed by Haryana, Madhya Pradesh, Rajasthan, West Bengal, and other states.24 The majority of polluting industries located along the river basin are tanneries in Kanpur, textile and locomotive industries in Varanasi, and various engineering enterprises in Prayagraj (formerly Allahabad). The tributaries of the Ganga are also surrounded by various small and large-scale industries like paper, sugar, distilleries, fertilizers, etc., in the areas of Haryana, Uttar Pradesh, and Madhya Pradesh. Apart from these, various industries that are located in residential areas of almost all the cities and villages also directly discharge their waste into the river.25
This untreated discharge is one of the major causes of the deterioration of the water quality of the Ganga. The major toxic constituents are heavy metals, pesticides, pathogens, and polyfluoroalkyl substances dissolved in matter.26,27 The studies have shown that the Ganga river's upper stretch at Rishikesh does not qualify for the parameters of potable water,28 while at lower stretches of the river, the water is not even suitable for bathing and other livelihood activities.29,30 This continuous discharge has affected the physicochemical properties and overall microbial counts of river water. Household discharge and sewage are other major sources of pollution in the river. An increasing level of urbanization along the Ganga basin has deteriorated the quality of river water. The level of dissolved oxygen (DO) and biological oxygen demand (BOD) has also increased in recent years. As per American Public health Association (APHA), clean and fresh water should have BOD of less than 5 ppm, and DO above 6.5–8 ppm.31 The level of ions of nitrate, nitrite, sulfate, phosphate, and hardness was found highest in Uttar Pradesh, followed by Bihar and Uttarakhand, excluding nitrate, which was found to be higher in West Bengal after Uttar Pradesh.32 The fecal and total coliform count is higher in West Bengal, followed by Uttar Pradesh.32 Due to a large number of industries present, Kanpur and Varanasi in Uttar Pradesh are the most contaminated sites, with 93
000 and 50
000 MPN per 100 mL of fecal coliform, respectively.32 While in West Bengal, the most contaminated sites are Dakshineswar and Howrah, with 425
313 and 237
059 MPN per 100 mL of fecal coliform, respectively.32 The maximum permissible limits of fecal coliform are found to be higher for drinking (50 MPN per 100 mL) and bathing water (500 MPN per 100 mL, CPCB, 2009). The report of the Central Pollution Control Board (CPCB) in 2009 revealed that the Ganga water is not suitable for drinking, even from its origin, at Gangotri.
2.1.1 Industrial effluents.
Hundreds of industries, out of which 956 are only situated in Uttar Pradesh, like tanneries, textiles, sugar mills, paper and pulp, thermal power plants, electro processing, dairies, pesticides, and fertilizer industries discharge various types of waste into the river.32,33 As per the study by Trivedi 2010, around 2500 MLD of industrial waste has been generated in the Ganga basin.33 The CPCB 2016 report has listed 764 grossly polluting industries that are situated in Uttar Pradesh, discharging their wastewater directly into the river without any treatment. The tannery industry constitutes the highest proportion of pollution among all the grossly polluting industries, due to which there exists an elevated level of chromium in the Ganga stretch along Kanpur and Kolkata. The level of toxic elements present in the Ganga basin has been summarized in Table 1.
Table 1 Changes in the level of inorganic pollution (trace and toxic elements) in Ganga water in different states24 (reused with permission)
Sl. no. |
Name of trace and toxic elements |
|
Level (mg L−1) in Ganga water in different states |
Uttarakhand |
Uttar Pradesh |
Bihar |
West Bengal |
A
|
Trace elements
|
1 |
Fe |
After 2009 |
0.03–2.61 |
0.0–7.0 |
— |
0.0–5.5 |
Before 2009 |
0.3–5.99 |
0.02–3 |
0.08–0.36 |
0.35–2.35 |
2 |
Zn |
After 2009 |
ND-13 |
ND-106 |
— |
ND-64 |
Before 2009 |
0.015–0.16 |
0.01–0.60 |
0.04–0.4 |
0.04–0.69 |
3 |
Cu |
After 2009 |
ND-0.18 |
ND-36 |
— |
ND-38 |
Before 2009 |
0.01–0.02 |
0.01–2.0 |
0.02–0.15 |
0.003–0.322 |
4 |
Mn |
After 2009 |
0.002–0.16 |
0.04–2.8 |
— |
ND-2.7 |
Before 2009 |
0.03–0.08 |
0.03–0.12 |
0.10–0.25 |
0.085–0.712 |
5 |
Co |
After 2009 |
0.002–0.02 |
ND-0.02 |
— |
— |
Before 2009 |
— |
0.01–0.05 |
— |
— |
![[thin space (1/6-em)]](https://www.rsc.org/images/entities/char_2009.gif) |
B
|
Toxic elements
|
1 |
Cr |
After 2009 |
0.003–0.2 |
0.0–52 |
— |
0.0–9.5 |
Before 2009 |
— |
ND-1.09 |
ND-0.13 |
0.01–0.39 |
2 |
Cd |
After 2009 |
0.0–0.7 |
0.00–13 |
— |
ND-1.4 |
Before 2009 |
ND-0.011 |
0.003–0.033 |
ND-0.10 |
ND-0.003 |
3 |
Pb |
After 2009 |
0.00–5.0 |
0.0–27 |
— |
ND-7.9 |
Before 2009 |
— |
0.03–0.19 |
ND-0.37 |
0.00004–0.076 |
4 |
As |
After 2009 |
0.003–0.01 |
ND-0.01 |
— |
0.0–4.7 |
Before 2009 |
— |
0.007–0.03 |
ND-0.01 |
— |
5 |
Hg |
After 2009 |
ND-0.0004 |
ND-0.0008 |
— |
0.0–0.69 |
Before 2009 |
0.0–0.000081 |
ND-0.002 |
ND |
0.00001–0.95 |
6 |
Ni |
After 2009 |
0.0–0.004 |
0.0–1.12 |
— |
0.0–9.7 |
Before 2009 |
0.01–0.05 |
0.03–0.9 |
ND-0.01 |
0.03–0.05 |
2.1.2 Agricultural waste.
The Indo-Gangetic Plain (IGP) is one of the most extensive fertile plains in the world.34 In India, nearly 13% of the IGP area is present in the states of Uttarakhand, Uttar Pradesh, Bihar, Jharkhand, and West Bengal. Around 50% of the food grains are produced in this region, which feeds about 40% of the country's population.34 India has agricultural importance, and therefore, the use of pesticides is also high. India produces ∼85
000 MT year−1 of pesticides and ranks fourth in the world after the US, Japan, and China.35,36 Annually, India uses 60
000 MT of pesticides, of which the highest consumption is in the Ganga basin.36,37 Pesticides and fertilizers used in agriculture could easily penetrate into the river via runoff streams and tributaries. Apart from the usual agricultural practice, the dry bed of the Ganga basin is also used for the cultivation of vegetables and fruits, further adding pesticides to the river after monsoon runoff. Organophosphorus pesticides (OPPs) and Organochlorine (OCPs) are the most consumed pesticides in India. These pesticides contribute to the increase in Persistent Organic Pollutants (POPs). POPs are carcinogenic and are of human and environmental health concern.37,38 Few pesticides, such as DDT, aldrin, etc., are banned by the Government of India, but due to low cost and easy availability, these pesticides are still being used for agricultural practices.39,40
Several researchers have monitored various types of pesticides in the Ganga basin, and most of them were reported to be at elevated levels, beyond the permissible limit of the World Health Organization (WHO).41,42 Severe pesticide contamination has been observed in Uttar Pradesh, particularly in Kanpur and Varanasi cities.42,43 The level of pesticides in the Ganga basin in different states has been listed in Table 2.
Table 2 Changes in the level of organic pollution (pesticides) in Ganga water in different states24 (reused with permission)
Sl. no. |
Name of pesticides |
|
Level (ng L−1) in Ganga water in different states |
Uttarakhand |
Uttar Pradesh |
Bihar |
West Bengal |
A
|
Organochlorines
|
1 |
Σ-HCH |
After 2009 |
5.2–7.24 |
0.1–3.5 |
ND-74.04 |
0.0–0.039 |
Before 2009 |
ND-153 |
1–99,517 |
11–2597 |
0–18,650 |
2 |
Σ-DDT |
After 2009 |
ND-1.01 |
0.05–2.21 |
ND-489 |
|
Before 2009 |
2.0–365 |
ND-143226 |
19–1663 |
0–6000 |
3 |
Σ-Endosulfan |
After 2009 |
ND-0.92 |
ND-85.4 |
ND-739 |
— |
Before 2009 |
ND-66 |
ND-66516 |
— |
0–3620 |
4 |
Σ-Aldrin |
After 2009 |
0.12–2.3 |
ND-2.2 |
ND-489 |
0.0–0.009 |
Before 2009 |
ND-46 |
ND-3340 |
ND-800 |
10–900 |
5 |
Σ-Hepta |
After 2009 |
0.06–0.32 |
ND-0.2 |
ND-11.8 |
0.0–0.026 |
Before 2009 |
— |
— |
— |
— |
6 |
2-4D |
After 2009 |
— |
— |
— |
— |
Before 2009 |
— |
ND-39 |
— |
— |
![[thin space (1/6-em)]](https://www.rsc.org/images/entities/char_2009.gif) |
B
|
Organophosphorus
|
1 |
Dimethoate |
After 2009 |
— |
— |
— |
— |
Before 2009 |
— |
0–2694 |
— |
0–1940 |
2 |
Malathion |
After 2009 |
— |
— |
— |
— |
Before 2009 |
— |
ND-6982 |
— |
0–4830 |
3 |
Methyl parathion |
After 2009 |
— |
— |
ND |
— |
Before 2009 |
— |
ND-500 |
— |
0–3050 |
4 |
Ethion |
After 2009 |
— |
— |
— |
— |
Before 2009 |
— |
0–1995 |
— |
— |
2.1.3 Religious activities.
As per the Hindu religious belief, the river Ganga is a soul purifier or “Mokshdayini”, which means ritual bathing in the river can help you escape the cycle of death and rebirth. The most important pilgrim centers of the Ganga basin are Rishikesh, Haridwar, Garhmukteshwar, Allahabad, Gangasagar, Mirzapur, Varanasi, and Kannauj, where numerous religious activities occur on the bank of the Ganga. On various festive occasions, people come to these pilgrim cities and take a dip called “Ganga Snan” in river.5 Devotees not only take a dip, but they also engage in various ritualistic offerings that include flowers, leaves, discarded remains of books and idols, earthen lamps, etc. People also perform the last rites of their deceased ones at the banks of Ganga. Occasionally, dead human bodies and dead animals are also drowned in the river as per the beliefs of people. All these different religious practices also contribute to the water quality of the Ganga river, which has been summarized here.
2.1.3.1 Religious bathing.
Millions of people take a bath in the Ganga every day; however, on auspicious days, rituals of mass bathing occur. The main festival of this ritualistic bathing is “Kumbh”, which takes place mainly at four places: Nasik, Allahabad, Ujjain, and Haridwar. Kumbh festival is celebrated once every 12 years in each of the four places, and it continues for one and a half months. During this period, billions of people take a bath in the Ganga.44 This mass gathering depreciates the water quality as people carelessly use detergents and discard waste, food, and clothes into the river. Hence, these ritualistic activities also end up contributing to the pollution levels of the sacred Gange River. The effect of Kumbh on the water quality of the Ganga has been reported by various researchers.44–46 The BOD, COD, and fecal coliform count have been reported to increase significantly after the Kumbha snan.47
2.1.3.2 Immersion of idol.
The immersion of idols is also one of the practices responsible for increasing numerous toxins in the Ganga river. During many festivities such as Durga Puja, Lakshmi Puja, and Ganesh Chaturthi that are celebrated in West Bengal, Uttar Pradesh, and Bihar, immersion of idols takes place on a large scale. These idols are made up of a variety of materials that includes clay, metals, plaster of Paris, and cloths. These are further decorated with plastic and polystyrene, reported to deteriorate the water quality.47 The materials used for the decoration of these idols contain heavy metals like arsenic, cadmium, lead, and chromium, which are carcinogens as per WHO recommendation.48 The plaster of Paris used for making the idol structure contains sulfur, phosphorus, magnesium, and gypsum. The immersion of these idols increases the acidity and heavy metal levels of the water.49–52 Approximately, thousands of liters of paint and hundreds of kilograms of toxic synthetic materials are immersed in the river with the idols.52 Plastic and polystyrene are not soluble; therefore, it also gets accumulated at the banks of the river and hampers the natural flow of water. These materials not only deteriorate the water quality of rivers but also affect aquatic life by killing the fish and damaging the phytoplankton.
2.1.3.3 Waste generated through the temple and religious activities.
Every year thousands of tons of flowers and garlands are thrown in the Ganga as an offering. At prominent places like Haridwar, Varanasi, etc., grand evening prayers are held, where devotees offer earthen lamps, flowers, and food items in the river. These practices are common and occur in large numbers. In Kashi Vishwanath temple of Varanasi, an estimated 20 quintals of floral waste are disposed of in the river daily.24 This amount increases four to five times in the months of Shrawan (July–August). Furthermore, the religious wastes generated by households also end up in Ganga, leading to a greater pollution load in Ganga every day.
2.1.4 Cremation of the dead body.
The religious belief prescribes that dying and cremation near the Ganga, particularly in Varanasi, releases the soul from the process of rebirth and directs the soul to heaven. On average, around thirty to forty thousand dead bodies are cremated every year, and the number increased during the recent COVID-19 pandemic.53 The ashes and unburnt or half-burnt body parts are thrown into the river, and even the ashes of bodies cremated elsewhere are also transported and thrown into the Ganga. Further, the dead body of thousands of people that are not burnt is directly dumped into the river. These practices degrade the water quality of the river to a great extent.
2.1.5 Contamination by pathogens.
The contamination of pathogens in the water bodies is a serious issue, which makes its recognition essential. The level of the pathogen is usually detected through indicator organisms like water-borne pathogens such as E. coli and fecal coliforms. It is a common approach to quantify the pathogenic load over the water bodies by estimating the indicator organisms. The main sources of the entry of these pathogens are sewage discharge, runoff from vegetated areas, and discharge from commercial and industrial effluents. These pathogens are known to cause diarrhea and gastrointestinal illness.54
2.2 Degradation in water quality
In ancient times, Ganga water was used to treat various diseases, and people believed bathing in Ganga will cure their illnesses. The antimicrobial property of Ganga was identified long back in 1896 by Ernest Hankin, the British bacteriologist. The research reports indicated that pathogenic microbes were not able to thrive in the Ganga.3,54 The high oxygen retention rate of Ganga allowed it to remain fresh even after long storage.7 The extent of various polluting activities has changed the water quality and diminished the self-cleansing ability of the Ganga.
2.3 Accumulation of toxic substances by aquatic life
Hundreds of fish varieties are found in the Ganga basin, including the most exotic species.55 The river also supports the country's economy by benefiting and providing fisheries resources. These fish markets have commercial importance and serve as sources of livelihood for the local people residing near the bank of Ganga. Increased level of pollution has decreased the water volume and affected the health and diversity of fish in the river. Several species of fish in the Ganga are under threat, including the national aquatic animal, the Dolphin. The species number of commercially important fish has declined significantly.55 The pesticides introduced in the river water accumulate in the fish and cause metabolic disruption.56,57 Various metals and metalloids also accumulate in the organs of fish, like in the gills, brain, liver, kidney, and skin, therefore altering various metabolic pathways.58 The toxins accumulate and magnify in the fishes in two ways either through gills, which are the main sites to uptake toxicants and then get absorbed in the blood or through consumption of contaminated food and phytoplankton with high metal concentrations.10,59
2.4 Human health risks and diseases associated with water
Degradation in the water quality of Ganga due to a high level of pesticides, toxic chemicals, and heavy metals highly affects human health by entering through the food chain.24 Consumption of contaminated seafood may cause serious health hazards, predominantly in the states where fish is a subsistence economy. The guidelines for drinking water and the permissible limit of pesticides and heavy metals have been established by WHO (1993) and other health and environmental protection agencies, which referred to the level of maximum toxicant uptake as “acceptable daily intake” (ADI).60
The level of most of the elements, along with pesticides and heavy metals, are higher than the ADI values in the Ganga. The water of the Ganga is unsuitable for direct utilization or drinking purposes. The water is first treated with disinfectant and then subjected to a conventional treatment process and then supplied for domestic usage. High levels of heavy metal contamination (arsenic, cadmium, chromium, and nickel) present in the river may increase the risk of cancer, as per International Agency for Research on Cancer.61 These heavy metals are classified as group 1 toxicants, and the pesticides such as DDT, HCH, and lead are categorized in group 2.61 Therefore, consumption of contaminated toxic food and water poses a high risk of human cancer. Apart from cancer, contaminated water intake can also lead to various other water-borne diseases such as cholera, diarrhea, hepatitis A, typhoid, food poisoning, and polio. The polluted water contains viruses and bacteria as well like E. coli, hepatitis A and E, which can easily pass from one living being to another and affect health. Though the previous studies show the elevated level of toxic chemicals in the water, the regular monitoring system and lack of people's interest in knowing and remediating the pollution level of Ganga indicates the precarious fate of Ganga.24
3. People's perception of measuring the water quality of Ganga
People's perception of the water quality of a river is derived from one or a combination of the following factors: sensorial, contextual, scientific, heuristics, or culture and belief.62 For river Ganga, culture and belief are of particular importance given its iconic cultural status and reverence among people as an embodiment of a living goddess. People's perception of water quality gives us insights into the community's attitude toward water management, their conception of ‘polluted’ and ‘potable’ water, and thus, their risk perception related to water usage.63,64 Social norms play an important role in determining people's beliefs, attitudes, and perceptions, which in effect, influence users' behavioral intent.65 While attitude is an individual trait, norms are the collective traits shared by members of a given group or society that drives the social behavior of the public.66,67 Rich qualitative data generated from the investigation of people's perception of water quality offers the potential for the co-production of knowledge through engagement with diverse groups of stakeholders, thereby creating new knowledge. Such equitable processes address the needs of different communities and facilitate their ability to participate meaningfully in water management. It will also allow for the identification of different levels of risk perceptions amongst different groups, thus leading to the effective allocation of resources for the mitigation of risks.68
Public perception of water quality drives the user's assessment of water suitability for different purposes, the feasibility of water reuse, and their actions towards resource conservation.69 Integrating this information into public policy can make environmental management strategies more robust by creating resource ownership, wider community support, and enhanced implementation.
Public perception highlights the user's perception of perceived risks, and their responses and also informs their dialogue with government officials and various other stakeholders. However, in several cases, public perception and expert's classification of differentials risks might differ, thereby creating challenges for effective risk communication. In the context of the Ganga river, the community's perception of its water quality is heavily guided by their religious beliefs, which prescribe the ‘sacredness’ and ‘self-cleansing’ ability of Ganga, thus rendering it immune to any polluting activity.70 Such beliefs and attitudes can diminish the perception of hazards due to pollutants, thereby negatively impacting environmental decision-making.
4. Available methods and test kits for monitoring the water quality of river Ganga
A low-cost real-time water detection monitoring system that can be applied in remote sensing for water bodies has been developed. The sensor is a wireless electrochemical sensor controlled by a microcontroller. The device is designed to detect the temperature of the water, its pH, and dissolved oxygen at a particular time interval. The sensor gathers the information and presents it in graphical and tabular form through the designed user interface on the pre-registered mobile phones.71
The first sensor developed for monitoring water was a pH sensor with a glass electrode, developed around 1930. Since then, the pH has been tested as the primary parameter for identifying the water quality in most sensor devices. It is important to analyze various other parameters to evaluate the water quality, for which multiple sensors are needed.
The world has become cautious about the conservation of natural resources, especially water, as it is a basic need of life. The contamination in the water can cause various diseases in the community. Therefore, the real-time monitoring of toxin levels is the need of the hour. Several systems were developed, among which some focused on the detection of the initiation of a contamination event. The first such kind of system was Canary, built by Sandia National Laboratories, and this work was funded by Environmental Protection Agency (EPA), National Homeland Security Research Center. This system is currently being used at Greater Cincinnati Water Works (GCWW). It provides online monitoring of several contaminants to analyze the water quality, for which it employs multiple direct and interlinked sensors. It has artificial intelligence programming that provides users to update their algorithms.72
Electrochemical sensors are the best-suited sensors for rapid, sensitive, and on-site analysis. A recent report by Cai and co-workers presented a rechargeable microbial electrochemical sensor with bioanode and biocathode. Acetate, formate, and hydrogen were used as charge carriers, with coulombic efficiency and energy efficiency of 38% ± 18% and 2.2% ± 0.7%, respectively. The sensors demonstrated good reusability due to their rechargeable nature. The microbes Acetobacterium, Acetoanaerobium, and Geobacter act as biorecognition elements.73 To design microscale sensors, microfluidic microscale technologies have also been exploited.
Microfluidic-based designs have been reported for the detection of various biological and non-biological analytes such as heavy metals, pesticides, and phosphates with an improved limit of detection. E. coli O157 and Salmonella typhimurium have been successfully used for biosensor fabrication.74 A study by Schwartz demonstrated the use of high-concentration labeled antimicrobial peptides (AMPs) in microfluidic devices to achieve a low detection limit (105 cfu mL−1) with a rapid response time of 2 min.75 Briefly, the AMPs were mixed with electrolytes and focused to travel in a vacuum-driven flow, and the vacuum line was also subjected to contaminated water. Any bacteria present in the water moves along with high AMPs concentrations. The bound bacteria from AMPs continue downstream while free AMPs remain in the stationary zone. These fluorescent signals were then recorded by the detector (Fig. 1). The microfluidic device chip layout has been demonstrated in Fig. 2.
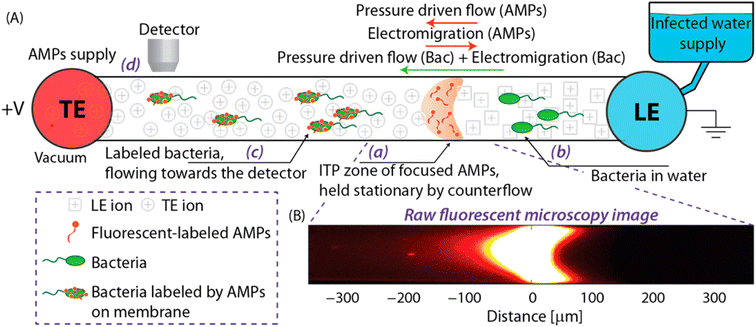 |
| Fig. 1 (A) Schematic representation of the assay using a single straight channel, (B) fluorescently labelled E. coli at the highest concentration area.75 | |
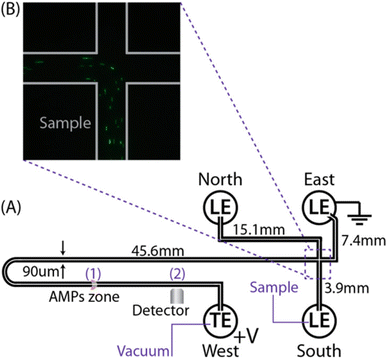 |
| Fig. 2 Representation of the microfluidic chip-based experimental setup.75 (A) The chip is a commercially available design (NS-12A, PerkinElmer) made of isotropically etched soda lime glass with dimensions of 90 μm (width) × 20 μm (depth). Also shown are the length dimensions of each intersected channel. A finite amount of AMPs is injected through the West reservoir, focused by cationic ITP, and remains confined and stationary at point (1) by negative pressure applied at the West reservoir. Electric field is applied on the channel by setting a constant voltage or current between East and West reservoirs, oriented for cationic ITP propagation from the West to the East. Detection of the fluorescent signal is obtained by a camera located at point (2), 4 mm downstream from the labeling zone. (B) Raw fluorescence image of the channel intersection showing an E. coli sample prelabeled with SYTO9, initially mixed in the South reservoir, flowing into the main channel, toward the labeling site. | |
The micro-scale sensor designed by Jiang et al. has also been customized to identify the concentration of bacteria in drinking water.76 The system was based on electrochemical impedance spectroscopy, where the presence of bacteria in the sample hinders the electrochemical system of the sensor (Fig. 3).
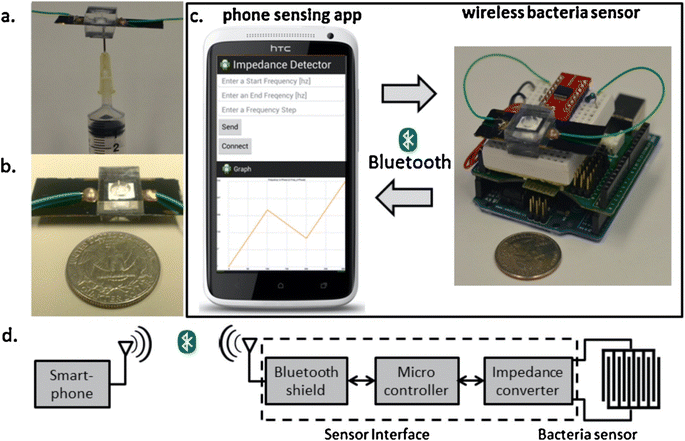 |
| Fig. 3 Smartphone based wireless bacteria sensing device (a) syringe to inject testing sample in the senor, (b) EIS based bacteria sensing (c) communication between smartphone and sensor device for bacteria sensing, (d) wireless circuit system.76 | |
To detect the chloride ion concentration in water, a microfluidic device using long-period fiber grating (LPFG) was designed by Wang in the year 2011.77 Apart from that, the microfluidic device integrated with optical transducers was also reported to measure several chemical and biological toxicants.78 A droplet sensor entrenched on an electrowetting-on-dielectric microfluidic system was reported by Zengerle and co-workers in the year 2012.79 The sampling requirement for analysis in the system is as less as 100 nL. The sensor was designed in a way that reduces power consumption, which makes the device useful for onsite detection. The microfluidic analytical platform can detect the contaminant at a very low level. The microfluidic platform has also been demonstrated for identifying the nanomaterials and their assembly, and this feature can also be useful to extricate the nanoparticle characterization.80 The experimental setup of the microfluidics device has been depicted in Fig. 4.
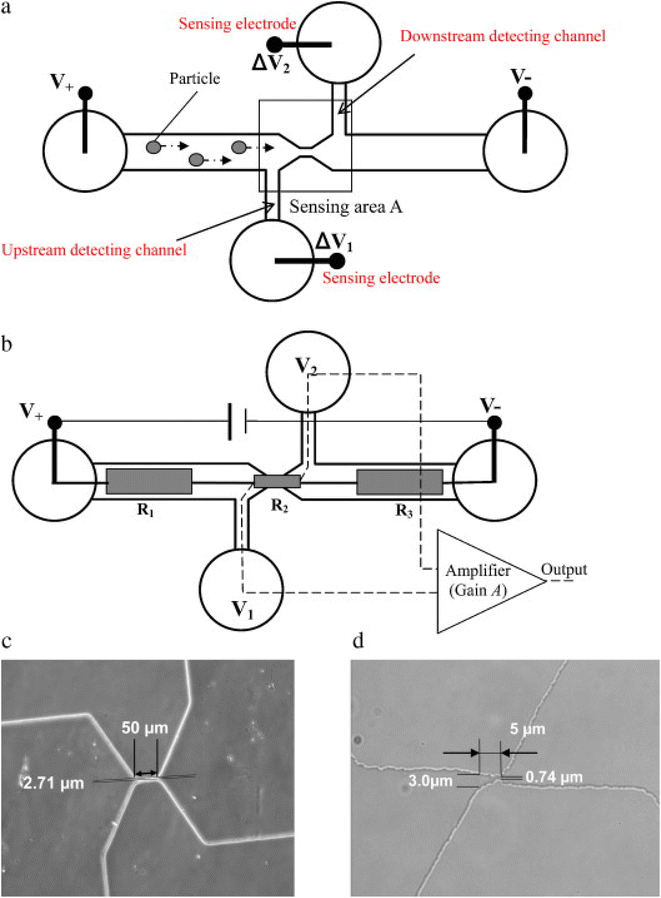 |
| Fig. 4 (a) Representation of a microfluidic setup for the detection of nanoparticles (b) circuit model of the sensor, (c) detection of 490 nm sized nanoparticle, and (d) detection of 220 nm sized nanoparticle.80 | |
Micro electro-mechanical system (MEMS) and polymer micromachining techniques have gained the interest of researchers for sensor development. MEMS provides the advantage of making the devices in the micron size to make the device compatible with a small volume of samples. Another advantage of MEMS based devices is their mass production, which permits low-cost components with high precision and reliability. Combining the electrochemical system with MEMS can provide more potential devices. Various other advancements in the sensor for water analysis have been made, such as using nanomaterials. Nanomaterials are well known for their good catalytic, mechanical, and electrical properties. Among the various metal nanomaterials, magnetic nanomaterials attribute extra advantage to capture targets and molecules of complex structure.81 The magnetic property of these nanomaterials is useful for their recovery after accumulation in the experiment. Magnetic nanomaterials have been reported for the detection of E. coli, where the magnetic beads were conjugated with bacteriophage (Fig. 5).82 In another study, aptamer-based biosensors have been reported to use gold-coated magnetic microdisks, which have an analysis time of fewer than 45 min.83 To detect pesticides in the water sample, a hybrid nanocomposite of Fe3O4@mSiO2 was reported by Xie and co-workers.84,93 Another study where graphene oxide (GO) coated with polyvinyl alcohol (PVA) was used to detect organophosphorus residues in the water was also reported.85 In another study, the detection of N-methylcarbamate in the surface water by temperature-handled ionic liquid amalgamated by magnetic multi-walled carbon nanotubes (MWCNTs) was reported.86 A report on electrochemical nanosensors was also published where a thiol and magnetic polymer was used for simultaneous detection of Cu(II) and Pb(II) in the natural water using differential-pulse voltammetry (DPV) analysis method.87 Few other information about sensors available for pollutant detection has been summarized in Table 3.
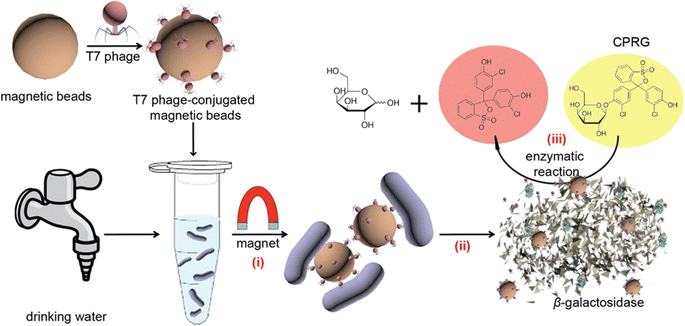 |
| Fig. 5 Schematic representation of the sensing mechanism to detect Escherichia coli in drinking water using T7 bacteriophage-conjugated magnetic probe. (i) T7 bacteriophage-conjugated magnetic probe-based E. coli separation, (ii) release of β-gal due to T7 bacteriophage infection, (iii) β-gal mediated CPRG hydrolysis that generates colorimetric readout82 (reprinted (adapted) with permission from {Chen, J.; Alcaine, S. D.; Jiang, Z.; Rotello, V. M.; Nugen, S. R. Detection of Escherichia coli in drinking water using T7 bacteriophage-conjugated magnetic probe. Anal. Chem. 2015, 87 (17), 8977-8984}. Copyright {2023} American Chemical Society). | |
Table 3 Sensors for monitoring water quality
Target |
Method |
Detection limit |
Reference |
E. coli and B. subtillis |
Electrochemical impedance spectroscopy (EIS) |
6 × 103 CFU mL−1 |
83
|
S. aureus
|
Differential pulse voltammetry (DPV) |
103 CFU mL−1 |
84
|
S. typhimurium
|
EIS |
10 CFU mL−1 |
85
|
C. parvum
|
Chrono-potentiometry; o-phenylenediamine/hydrogen peroxide potentiometry |
500 oocysts mL−1 |
86
|
Middle East respiratory syndrome corona virus (MERS-CoV) |
Square wave voltammetry (SWV) |
400 fg mL−1 |
87
|
Mercury (Hg2+) ions |
Electrochemical |
0.098 ppb |
88
|
Cadmium (Cd2+) ions |
Stripping voltammetry |
20.7 ppb |
88
|
Lead (Pb2+) ions |
Square wave anodic stripping voltammetry (SWASV) |
1.8 ppb |
88
|
Arsenic |
SWASV |
8 × 10−4 ppb |
89
|
Chromium |
Cyclic voltammetry (CV) |
0.03 μM |
90
|
Zinc |
CV |
7.5 μg L−1 |
91
|
Copper |
CV |
0.635 ppm |
92
|
5. Fate of Ganga water estimation and way forward
The rapid increase in industrialization and unplanned urbanization have increased the disposal of harmful pollutants in the river, leading to the degradation of natural water resources. This has detrimental effects on aquatic as well as human health. Water is a basic need of life and livelihood; therefore, the quality of water must be monitored regularly, and detailed information about water pollution should be documented. Recent developments in sensing applications have shown many advantages of providing rapid and accurate monitoring of water contaminants. Biomaterials functionalized with magnetic and metallic materials are also being utilized for effective detection processes due to their efficient conductivity, biocompatibility, high surface-to-volume ratio, magnetic properties, and easy fabrication.94 The water quality assessment of Ganga through these sensing techniques proved that water in Ganga is highly polluted and thus not appropriate for drinking. Regular monitoring and strong management strategies should be implemented by regulatory authorities for effective water quality management. There are innumerable approaches for improving the water quality of the Ganga, and a few options are (i) efficient irrigation and agricultural practices, (ii) quality check in the inflow and outflow streams of the Ganga using effective sensing technologies, (iii) control of effluent discharge from the industries, (iv) rational practice of cremation and other religious rituals should be followed. With renewed time, some rational thinking and behavioral approach should be practiced to improve the current scenario. The coming years will be the indication of the applicable measures and efficacy taken by the government and society's collective effort to conserve the Ganga and transform it into a healthy river.95
6. Conclusions
The sacred Indian river Ganga covers more than 2500 km and influences more than 400 million human life and millions of many other forms of life. Apart from that, the river Ganga also provides an equilibrium of biotic and abiotic balance throughout all seasons over centuries of its existence. However, during the last century, anthropogenic activities transformed this river into one of the most polluted rivers in the world. Reversing this equilibrium to an acceptable quality of water has become one of the main challenges in India politically, scientifically, socially, and culturally. This study has examined available data sources from different angles and dimensions of pollutants and pinpointed the following key aspects to be considered for coherently integrating to formulate a scope for quality water by Participation, Policy, Procedure, Process, and Practices (5P approach):
• Participation: people's participation in identifying and formulating an acceptable knowledge base to make informed decisions.
• Policies: needs to be formulated with the engagement of all stakeholders involved in polluting activities, such as industry, agriculture, religious activities, sewage, etc., to formulate strict public norms.
• Procedure: to identify norms to develop a scale and people-friendly test kit.
• Process: of engagement could be based on the norms identified and formulate sustainable actions of engagement.
• Practice: is for achieving acceptable water quality in the Ganga river and could be a cooperative venture willingly undertaken by all stakeholders based on a knowledge base and norms created above.
Overall, the water quality of river Gange and the future of the river has heavy dependence on the suggested 5P approach for actions and creating a people-friendly knowledge base, test kit, and norms of engagement with the river Ganga water by all stakeholders. This review provides an inclusive understanding of the factors responsible for the water quality deterioration of Ganga, how people should be more acquainted with the activities harming the water, and what test kits are available to determine the toxic pollutants.
Conflicts of interest
There are no conflicts to declare.
Acknowledgements
RKG acknowledges financial assistance from the Department of Science and Technology (DST), India, through Grant No. DST/TM/WTI/2K16/23(G).
References
-
A. K. Basu, Ecological and Resource Study of the Ganga Delta, Bagchi, Calcutta, 1992 Search PubMed.
- M. Singh and A. K. Singh, Bibliography of environmental studies in natural characteristics and anthropogenic influences on the Ganga river, Environ. Monit. Assess., 2007, 129, 421–432, DOI:10.1007/s10661-006-9374-7.
- C. S. Nautiyal, Scientific validation of incorruptible self-purificatory characteristic of Ganga water, Asian Agrihist., 2009, 13, 53–56 Search PubMed.
- C. S. Nautiyal, Self-purification Ganga water facilitates death of pathogenic Escherichia coli O157:H7, Curr. Microbiol., 2009, 58, 25–29, DOI:10.1007/s00284-008-9260-3.
- B. Rai, Pollution and conservation of Ganga river in modern India, j. sci. res. publ., 2013, 3(4), 1–4 Search PubMed.
- D. M. Joshi, A. Kumar and N. Agrawal, Assessment of the irrigation water quality of river Ganga in Haridwar district Rasayan, J. Chem., 2009, 2, 285–292 CAS.
- D. Mukherjee, M. Chattopadhaya and S. C. Lahiri, Water quality of the River Ganga (the Ganges) and some of its physicochemical properties, Environmentalist, 1993, 13, 199–210, DOI:10.1007/BF01901382.
- A. Kumari, R. K. Sinha and K. Gopal, Concentration of organochlorine pesticide residues in Ganga water in Bihar, India, J. Ecol. Environ., 2001, 19(2), 351–356 CAS.
- M. Singh, G. Muller and I. B. Singh, Heavy metals in freshly deposited stream sediments of rivers associated with urbanization of the Ganga plain, India, Water Air Soil Pollut., 2002, 141, 35–54, DOI:10.1023/A:1021339917643.
- S. Dwivedi, R. D. Tripathi, U. N. Rai, S. Srivastava, S. Mishra, M. K. Shukla, A. K. Gupta, S. Sinha, V. S. Baghel and D. K. Gupta, Dominance of algae in Ganga water polluted through fly–ash leaching: metal bioaccumulation potential of selected algal species, Bull. Environ. Contam. Toxicol., 2006, 77, 427–436, DOI:10.1007/s00128-006-1083-y.
- R. K. Sinha, S. K. Sinha, D. K. Kedia, A. Kumari, N. Rani, G. Sharma and K. Prasad, A holistic study on mercury pollution in the Ganga river system at Varanasi, India, Curr. Sci., 2007, 92, 1223–1228 CAS.
- Deepali and K. K. Gangwar, Metals concentration in textile and tannery effluents, associated soils and ground water N. Y., J. Sci., 2010, 3, 82–89 Search PubMed.
- P. K. Rai, A. Mishra and B. D. Tripathi, Heavy metal and microbial pollution of the River Ganga: a case study of water quality at Varanasi, Aquat. Ecosyst. Health Manag., 2010, 13, 352–361, DOI:10.1080/14634988.2010.528739.
- S. Katiyar, Impact of tannery effluent with special reference to seasonal variation on physico–chemical characteristics of river water at Kanpur (U.P). India, J. Anal. Toxicol., 2011, 1, 1–7, DOI:10.4172/2161-0525.1000115.
- P. K. Mutiyar and A. K. Mittal, Status of organochlorine pesticides in Ganga river basin: anthropogenic or glacial?, Drink. Water Eng. Sci., 2013, 6, 69–80, DOI:10.5194/dwes-6-69-2013.
-
Central Pollution Control Board (CPCB) Bulletin Vol-I, July 2016, http://www.cpcb.nic.in/upload/Latest/Latest_123_SUMMARY_BOOK_FS.pdf, 2016 Search PubMed.
- S. Ejaz, W. Akram, C. W. Lim, J. J. Lee and I. Hussain, Endocrine disrupting pesticides: a leading cause of cancer among rural people in Pakistan, Exp. Oncol., 2004, 26, 98–105 CAS.
- M. Pal, S. Ghosh, M. Mukhopadhyay and M. Ghosh, Methyl mercury in fish – a case study on various samples collected from Ganges river at West Bengal, Environ. Monit. Assess., 2012, 184, 3407–3414, DOI:10.1007/s10661-011-2193-5.
- A. K. Bhattacharya, S. N. Mandal and S. K. Das, Heavy metals accumulation in water, sediment and tissues of different edible fishes in upper stretch of Gangetic West Bengal, Trends Appl. Sci. Res., 2008, 3, 61–68, DOI:10.3923/tasr.2008.61.68.
- A. Mitra, R. Chowdhury and K. Banerjee, Concentrations of some heavy metals in commercially important finfish and shellfish of the river Ganga, Environ. Monit. Assess., 2012, 184, 2219–2230, DOI:10.1007/s10661-011-2111-x.
- S. U. Sudhakar, Effect of pollutants on the fishes of Ganga and Sai River of Raebareli District in Uttar Pradesh in India, Res. J. Anim. Vet. Fishery Sci., 2014, 2, 1–6 Search PubMed.
- K. Jain, V. Sreenivas, T. Velpandian, U. Kapil and P. K. Garg, Risk factors for gallbladder cancer: a case–control study, Int. J. Cancer, 2013, 132, 1660–1666, DOI:10.1002/ijc.27777.
- Z. S. Ahammad, T. R. Sreekrishnan, C. L. Hands, C. W. Knapp and D. W. Graham, Increased Waterborne blaNDM-1 resistance gene abundances associated with seasonal human pilgrimages to the upper Ganges river, Environ. Sci. Technol., 2014, 48, 3014–3020, DOI:10.1021/es405348h.
- S. Dwivedi, S. Mishra and R. D. Tripathi, Ganga water pollution: a potential health threat to inhabitants of Ganga basin, Environ. Int., 2018, 117, 327–338, DOI:10.1016/j.envint.2018.05.015.
- M. Roy and F. Shamim, Research on the impact of industrial pollution on River Ganga: A Review, Int. J. Prev. Control Ind. Pollut., 2020, 6(1), 43–51 Search PubMed.
- M. L. Magnuson, S. C. Allgeier, B. Koch, R. D. Leon and R. Hunsinger, Responding to water contamination threats, Environ. Sci. Technol., 2005, 39(7), 153A–159A CrossRef CAS PubMed.
- V. Franke, M. Ullberg, P. McCleaf, M. Wålinder, S. J. Köhler and L. Ahrens, The price of really clean water: Combining nanofiltration with granular activated carbon and anion exchange resins for the removal of per-and polyfluoralkyl substances (PFASs) in drinking water production, ACS ES&T Water, 2021, 1(4), 782–795, DOI:10.1021/acsestwater.0c00141.
- T. Shukla, I. S. Sen, S. Boral and S. Sharma, A time-series record during COVID-19 lockdown shows the high resilience of dissolved heavy metals in the Ganga River, Environ. Sci. Technol. Lett., 2021, 8(4), 301–306, DOI:10.1021/acs.estlett.0c00982.
- N. K. Mishra and S. C. Mohapatra, Effect of Gangetic pollution on water borne diseases in Varanasi: a case study, Indian J. Prev. Soc. Med., 2009, 40, 39–42 Search PubMed.
- S. Hamner, S. C. Broadaway, V. B. Mishra, A. Tripathi, R. K. Mishra, E. Pulcini, B. H. Pyle and T. E. Ford, Isolation of potentially pathogenic Escherichia coli O157:H7 from the Ganges river, Appl. Environ. Microbiol., 2007, 73, 2369–2372, DOI:10.1128/AEM.00141-07.
-
R. B. Baird, A. D. Eaton and L. S. Clesceri, Standard Methods for the Examination of Water and Wastewater, ed. E. W. Rice, American public health association, Washington, DC, 2012, vol. 10 Search PubMed.
- S. Pandey and L. Prasad, Pollution impact on Ganga river and fisheries, J. Vet. Sci. Technol., 2014, 5, 160 Search PubMed.
- R. C. Trivedi, Water quality of the Ganga River – an overview, Aquat. Ecosyst. Health Manage., 2010, 13(4), 347–351, DOI:10.1080/14634988.2010.528740.
- D. K. Pal, T. Bhattacharyya, P. Srivastava, P. Chandran and S. K. Ray, Soils of the Indo-Gangetic Plains: their historical perspective and management, Curr. Sci., 2009, 96, 1193–1202 CAS.
-
B. N. Sampathkumar, A Study on Brand Preference of Pesticides Used in Tomato Production in Kolar Taluk Master Thesissubmitted to University of Agricultural Sciences, Bengaluru, 2014, vol. 45 Search PubMed.
- S. P. Mohapatra, V. T. Gajbhiye, N. P. Agnihotri and M. Raina, Insecticide pollution of India rivers, Environmentalist, 1995, 15, 41–44, DOI:10.1007/BF01888888.
- B. Kumar, V. K. Verma, A. K. Naskar, P. Chakraborty, S. Kumar and D. Mukherjee, Human health risk from hexachlorocyclohexane and dichlorodiphenyltrichloroethane pesticides, through consumption of vegetables: estimation of daily intake and hazard quotients, J. Xenobiot., 2013, 3, 29–35, DOI:10.4081/xeno.2013.e6.
- S. Ejaz, W. Akram, C. W. Lim, J. J. Lee and I. Hussain, Endocrine disrupting pesticides: a leading cause of cancer among rural people in Pakistan, Exp. Oncol., 2004, 26, 98–105 CAS.
- P. C. Abhilash and N. Singh, Pesticide use and application: an Indian scenario, J. Hazard. Mater., 2009, 165, 1–12, DOI:10.1016/j.jhazmat.2008.10.061.
- J. Vijgen, P. C. Abhilash, Y. Li, R. Lal, M. Forter, J. Torres, S. Nandita, M. Yunus, C. Tian, A. Schaffer and R. Weber, Hexachlorocyclohexane (HCH) as new Stockholm Convention POPs-a global perspective on the management of lindane and its waste isomers, Environ. Sci. Pollut. Res., 2011, 18, 152–162, DOI:10.1007/s11356-010-0417-9.
- L. Guzzella, C. Roscioli, L. Viganò, M. Saha, S. K. Sarkar and A. Bhattacharya, Evaluation of the concentration of HCH, DDT, HCB, PCB and PAH in the sediments along the lower stretch of Hugli estuary, West Bengal, northeast India, Environ. Int., 2005, 31, 523–534, DOI:10.1016/j.envint.2004.10.014.
- P. K. Mutiyar and A. K. Mittal, Status of organochlorine pesticides in Ganga river basin: anthropogenic or glacial?, Drink. Water Eng. Sci., 2013, 6, 69–80, DOI:10.5194/dwes-6-69-2013.
- N. Semwal and P. Akolkar, Water quality assessment of sacred Himalayan rivers of Uttaranchal, Curr. Sci., 2006, 91, 486–496 CAS.
- V. K. Tyagi, A. Bhatia, R. Z. Gaur, A. A. Khan, M. Ali, A. Khursheed, A. A. Kazmi and S. L. Lo, Impairment in water quality of Ganges river and consequential health risks on account of mass ritualistic bathing, Desalination Water Treat., 2013, 51, 2121–2129, DOI:10.1080/19443994.2013.734677.
- N. Joshi and V. Sati, Assessment of water quality of river Ganges at Haridwar during Kumbh Mela–2010, Rep. opinion, 2011, 3, 30–36 Search PubMed.
- P. Srivastava, A. Burande and N. Sharma, Fuzzy environmental model for evaluating water quality of Sangam zone during Maha Kumbh 2013, Appl. Comput. Intell., 2013, 2013, 1–7, DOI:10.1155/2013/265924.
- H. Kulshrestha and S. Sharma, Impact of mass bathing during Ardhkumbh on water quality status of river Ganga, J. Environ. Biol., 2006, 27, 437–440 CAS.
-
WHO, Guidelines for Drinking-Water Quality, World Health Organization, Geneva, 3rd edn, 2008, vol. 1, pp. 306–308b, Incorporating 1st and 2nd Addenda, Recommendations Search PubMed.
- K. K. Das, T. Panigrahi and R. B. Panda, Idol immersion activities cause heavy metal contamination in river Budhabalanga, Balasore, Odisha, India, Int. j. mod. eng. res., 2012, 2, 4540–4542 Search PubMed.
- B. J. Kaur, M. P. George and S. Mishra, Water quality assessment of river Yamuna in Delhi stretch during Idol immersion, Int. J. Environ. Sci., 2013, 3, 2122–2130, DOI:10.6088/ijes.2013030600028.
- S. Bhattacharya, A. Bera, A. Dutta and U. C. Ghosh, Effects of idol immersion on the water quality parameters of Indian water bodies: environmental health perspectives, Int. lett. chem. phys. astron., 2014, 20, 234–263 Search PubMed.
- G. Mohini, A. Ekhalak and S. Ranjana, Pollution load assessment of Tapi river during Ganesh festival, India, Octa J. Environ. Res., 2014, 2, 310–313 Search PubMed.
-
B. D. Tripathi and S. Tripathi, Issues and challenges of river Ganga, Our National River Ganga: Life of Millions, ed. R. Sanghi, Springer International Publishing, Switzerland, 2014, pp. 211–221 Search PubMed.
- P. K. Pandey, P. H. Kass, M. L. Soupir, S. Biswas and V. P. Singh, Contamination of water resources by pathogenic bacteria, AMB Express, 2014, 4(1), 1–16, DOI:10.1186/s13568-014-0051-x.
- U. K. Sarkar, A. K. Pathak, R. K. Sinha, K. Sivakumar, A. K. Pandian, A. Pandey, V. K. Dubey and W. S. Lakra, Freshwater fish biodiversity in the River Ganga (India): changing pattern, threats and conservation perspectives, Rev. Fish Biol. Fish., 2012, 22, 251–272, DOI:10.1007/s11160-011-9218-6.
- P. B. Singh and V. Singh, Bioaccumulation of hexachlorocyclohexane, dichlorodiphenyltrichloroethane, and estradiol–17b in catfish and carp during the pre–monsoon season in India, Fish Physiol. Biochem., 2008, 34, 25–36, DOI:10.1007/s10695-007-9142-9.
- M. W. Aktar, M. Paramasivam, D. Sengupta, S. Purkait, M. Ganguly and S. Banerjee, Impact assessment of pesticide residue in fish of Ganga river around Kolkata in West Bengal, Environ. Monit. Assess., 2009, 157, 97–104, DOI:10.1007/s10661-008-0518-9.
- H. Vaseem and T. K. Banerjee, Contamination of the river Ganga and its toxic implication in the blood parameters of the major carp Labeo rohita (Ham), Environ. Sci. Pollut. Res., 2013, 20, 5673–5681, DOI:10.1007/s11356-013-1570-8.
- S. Dwivedi, S. Srivastava, S. Mishra, A. Kumar, R. D. Tripathi, U. N. Rai, R. Dave, P. Tripathi, D. Chakrabarty and P. K. Trivedi, Characterization of native microalgal strains for their chromium bioaccumulation potential: phytoplankton response in polluted habitats, J. Hazard. Mater., 2010, 173, 95–101, DOI:10.1016/j.jhazmat.2009.08.053.
-
WHO Guidelines for Drinking-Water Quality, 2nd edn, 1993, vol. 1, Recommendations, Geneva Search PubMed.
-
IARC, Agents Classified by the IARC Monographs, vol. 1–120, 2017 Search PubMed.
- M. de França Doria, Factors influencing public perception of drinking water quality, Water Policy, 2010, 12(1), 1–19, DOI:10.1186/s13643-019-1013-9.
- N. J. Bennett, Using perceptions as evidence to improve conservation and environmental management: Perceptions and Conservation, Conserv. Biol., 2016, 30(3), 582–592, DOI:10.1111/cobi.12681.
- F. Tarannum, A. Kansal and P. Sharma, Understanding public perception, knowledge and behaviour for water quality management of the river Yamuna in India, Water Policy, 2018, 20(2), 266–281, DOI:10.2166/wp.2018.134.
- I. Ajzen and M. Fishbein, A Bayesian analysis of attribution processes, Psychol. Bull., 1975, 82(2), 261, DOI:10.1037/h0076477.
-
C. Bicchieri, The Grammar of Society: the Nature and Dynamics of Social Norms. Cambridge University Press, 2005 Search PubMed.
- H. P. Young, The evolution of social norms, Econ., 2015, 7(1), 359–387, DOI:10.1146/annurev-economics-080614-115322.
-
USEPA, Development of User Perception Surveys to Protect Water Quality from Nutrient Pollution: A Primer on Common Practices and Insights, EPA 823-R-21-001, U.S. Environmental Protection Agency, Washington, DC, 2021 Search PubMed.
- M. Okumah, A. S. Yeboah and S. K. Bonyah, What matters most? Stakeholders' perceptions of river water quality, Land use policy, 2020, 99, 104824, DOI:10.1016/j.landusepol.2020.104824.
- S. Sachdeva, The influence of sacred beliefs in environmental risk perception and attitudes, Environ. Behav., 2017, 49(5), 583–600 CrossRef.
- A. T. Demetillo, M. V. Japitana and E. B. Taboada, A system for monitoring water quality in a large aquatic area using wireless sensor network technology, Sustain. Environ. Res., 2019, 29(1), 1–9, DOI:10.1186/s42834-019-0009-4.
- U. Ahmed, R. Mumtaz, H. Anwar, S. Mumtaz and A. M. Qamar, Water quality monitoring: from conventional to emerging technologies, Water Supply, 2020, 20(1), 28–45, DOI:10.2166/ws.2019.144.
- J. Cai, H. Huang, Z. Li, Y. Gao, Q. Liang, X. Chen, N. Chu, W. Hao, D. Wang, Y. Jiang and R. J. Zeng, A rechargeable microbial electrochemical sensor for water biotoxicity monitoring, Biosens. Bioelectron., 2022, 10, 100132, DOI:10.1016/j.biosx.2022.100132.
- C. G. Koh, W. Tan, M. Q. Zhao, A. J. Ricco and Z. H. Fan, Integrating polymerase chain reaction, valving, and electrophoresis in a plastic device for bacterial detection, Anal. Chem., 2003, 75(17), 4591–4598, DOI:10.1021/ac0343836.
- O. Schwartz and M. Bercovici, Microfluidic assay for continuous bacteria detection
using antimicrobial peptides and isotachophoresis, Anal. Chem., 2014, 86(20), 10106–10113, DOI:10.1021/ac5017776.
- J. Jiang, X. Wang, R. Chao, Y. Ren, C. Hu, Z. Xu and G. L. Liu, Smartphone based portable bacteria pre-concentrating microfluidic sensor and impedance sensing system, Sens. Actuators B Chem., 2014, 193, 653–659, DOI:10.1016/j.snb.2013.11.103.
- J. N. Wang, A microfluidic long-period fiber grating sensor platform for chloride ion concentration measurement, Sensors, 2011, 11(9), 8550–8568, DOI:10.3390/s110908550.
- D. Sparks, R. Smith, M. Straayer, J. Cripe, R. Schneider, A. Chimbayo, S. Anasari and N. Najafi, Measurement of density and chemical concentration using a microfluidic chip, Lab Chip, 2003, 3(1), 19–21, 10.1039/B211429A.
-
R. Zengerle, J. Hoffmann, G. Roth, O. Strohmeier, A. R. Fiebach, L. Drechsel, S. Zhang, A. Kloke, N. Paust, D. Mark and F. Von Stetten, Microfluidic “Apps” on Standard Lab-Instruments, in 16th International Conference on Miniaturized Systems for Chemistry and Life Sciences (μTAS), Okinawa, Japan, 2012, vol. 28, No. 01.11 Search PubMed.
- Y. Song, H. Zhang, C. H. Chon, X. Pan and D. Li, Nanoparticle detection by microfluidic resistive pulse sensor with a submicron sensing gate and dual detecting channels-two stage differential amplifier, Sens. Actuators B Chem., 2011, 155(2), 930–936, DOI:10.1016/j.snb.2011.01.004.
- H. Karimi-Maleh, C. T. Fakude, N. Mabuba, G. M. Peleyeju and O. A. Arotiba, The determination of 2-phenylphenol in the presence of 4-chlorophenol using nano-Fe3O4/ionic liquid paste electrode as an electrochemical sensor, J. Colloid Interface Sci., 2019, 554, 603–610, DOI:10.1016/j.jcis.2019.07.047.
- J. Chen, S. D. Alcaine, Z. Jiang, V. M. Rotello and S. R. Nugen, Detection of Escherichia coli in drinking water using T7 bacteriophage-conjugated magnetic probe, Anal. Chem., 2015, 87(17), 8977–8984 CrossRef CAS PubMed.
- N. M. Saucedo, S. Srinives and A. Mulchandani, Electrochemical biosensor for rapid detection of viable bacteria and antibiotic screening, J. Anal. test, 2019, 3(1), 117–122, DOI:10.1007/s41664-019-00091-2.
- M. Divagar, R. Sriramprabha, S. Sornambikai, N. Ponpandian and C. Viswanathan, Surface imprinted Ag decorated MnO2 thin film electrodes for the synergic electrochemical detection of bacterial pathogens, J. Electrochem. Soc., 2019, 166(2), G1, DOI:10.1149/2.0711902jes.
- D. Wilson, E. M. Materon, G. Ibanez-Redin, R. C. Faria, D. S. Correa and O. N. Oliveira Jr, Electrical detection of pathogenic bacteria in food samples using information visualization methods with a sensor based on magnetic nanoparticles functionalized with antimicrobial peptides, Talanta, 2019, 194, 611–618, DOI:10.1016/j.talanta.2018.10.089.
- O. Lazcka, F. J. Del Campo and F. X. Munoz, Pathogen detection: A perspective of traditional methods and biosensors, Biosens. Bioelectron., 2007, 22(7), 1205–1217, DOI:10.1016/j.bios.2006.06.036.
- L. A. Layqah and S. Eissa, An electrochemical immunosensor for the corona virus associated with the Middle East respiratory syndrome using an array of gold nanoparticle-modified carbon electrodes, Microchim. Acta, 2019, 186(4), 224, DOI:10.1007/s00604-019-3345-5.
- A. Nigam, N. Sharma, S. Tripathy and M. Kumar, Development of semiconductor based heavy metal ion sensors for water analysis: A review, Sens. Actuator A Phys., 2021, 330, 112879, DOI:10.1016/j.sna.2021.112879.
- C. Gao, X. Y. Yu, S. Q. Xiong, J. H. Liu and X. J. Huang, Electrochemical detection of arsenic (III) completely free from noble metal: Fe3O4 microspheres-room temperature ionic liquid composite showing better performance than gold, Anal. Chem., 2013, 85(5), 2673–2680, DOI:10.1021/ac303143x.
- W. Jin, G. Wu and A. Chen, Sensitive and selective electrochemical detection of chromium (VI) based on gold nanoparticle-decorated titania nanotube arrays, Analyst, 2014, 139(1), 235–241, 10.1039/C3AN01614E.
- M. Moyo, Horseradish peroxidase biosensor to detect zinc ions in aqueous solutions, Open J. Appl. Biosens., 2014, 3, 1–7, DOI:10.4236/ojab.2014.31001.
- B. Feier, I. Băjan, I. Fizeşan, D. Floner, C. Cristea, F. Geneste and R. Săndulescu, Highly selective electrochemical detection of copper (II) using N, N’-bis (acetylacetone) ethylenediimine as a receptor, Int. J. Electrochem. Sci., 2015, 10, 121–139 Search PubMed.
- J. Xie, T. Liu, G. Song, Y. Hu and C. Deng, Simultaneous analysis of organophosphorus pesticides in water by magnetic solid-phase extraction coupled with GC–MS, Chromatographia, 2013, 76(9), 535–540, DOI:10.1007/s10337-013-2408-8.
- A. Hojjati-Najafabadi, M. Mansoorianfar, T. Liang, K. Shahin and H. Karimi-Maleh, A review on magnetic sensors for monitoring of hazardous pollutants in water resources, Sci. Total Environ., 2022, 824, 153844, DOI:10.1016/j.scitotenv.2022.153844.
- N. Kaushal, S. Babu, A. Mishra, N. Ghosh, V. Tare, R. Kumar, P. K. Sinha and R. U. Verma, Towards a healthy ganga—improving river flows through understanding trade-offs, Front. Environ. Sci., 2019, 7, 83, DOI:10.3389/fenvs.2019.00083.
|
This journal is © The Royal Society of Chemistry 2023 |
Click here to see how this site uses Cookies. View our privacy policy here.