DOI:
10.1039/D3SC04945K
(Edge Article)
Chem. Sci., 2023,
14, 13228-13234
An efficient and mild oxidative approach from thiols to sulfonyl derivatives with DMSO/HBr†
Received
20th September 2023
, Accepted 30th October 2023
First published on 1st November 2023
Abstract
A mild and practical method for synthesizing sulfonyl derivatives, which have a wide range of applications in pharmaceuticals, materials, and organic synthesis, was described through the oxidative functionalization of thiols with DMSO/HBr. The simple conditions, low cost and ready availability of DMSO/HBr, as well as the versatility of the transformations, make this strategy very powerful in synthesizing a variety of sulfonyl derivatives including sulfonamides, sulfonyl fluorides, sulfonyl azides, and sulfonates. Mechanistic studies revealed that DMSO served as the terminal oxidant, and HBr acted as both a nucleophile and a redox mediator to transfer the oxygen atom.
Introduction
Sulfonyl derivatives including sulfonyl halides, sulfonamides, sulfonyl azides etc. have a myriad of applications in pharmaceuticals, agrochemicals, and materials (Fig. 1).1 For instance, sulfonyl halides are vital protecting reagents, versatile partners for cross-coupling reactions, and important synthetic intermediates.2 Sulfonamides are some of the most important motifs in pharmaceuticals, and 25% of the FDA approved drugs from 2016 to 2018 contain the sulfonamide moiety.3 Many strategies for the synthesis of sulfonyl derivatives have been developed, including transition-metal catalyzed coupling reactions,4 electrochemical C–H activation,5 nucleophilic reaction of sulfinate salts,6 and the design of new sulfonylating reagents.7 These elegant strategies provide new protocols to access sulfonyl derivatives and push this field forward.
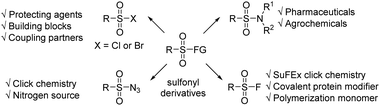 |
| Fig. 1 The application of sulfonyl derivatives. | |
Compared with the above strategies, the oxidative functionalization of commercially available thiols with nucleophiles represents one of the most convenient protocols to synthesize sulfonyl derivatives. In this transformation, the thiol motif couples with a nucleophile with two oxygen atoms introduced into the sulfur center simultaneously. Thus, the oxidant plays a dominant role in these reactions.8 Many oxidative functionalization reactions of thiols employed stoichiometric oxidants, such as oxone,8a Cu(OAc)2,8b PhI(OAc)2,8c I2O5,8d TBHP,8e selectfluor8fetc., which suffer from narrow substrate scope, low atom economy, and sometimes harsh reaction conditions8a (Scheme 1A). Recently, Noël9 and coworkers developed an elegant electrochemical oxidative functionalization of thiols with KF or amines for the efficient synthesis of sulfonyl fluorides and sulfonamides, which features broad substrate scope, high atom economy, and environmentally friendly reagents (Scheme 1B). Despite the significance of previous work, it is still highly desirable to develop a practical and efficient protocol to access sulfonyl derivatives with commercially available chemicals, mild reaction conditions, and broad substrate scope.
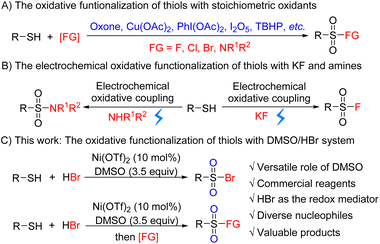 |
| Scheme 1 Oxidative functionalization of thiols. | |
DMSO, as a common solvent, could serve as an oxidant, catalyst, or reagent in organic synthesis.10 Compared with other solvents, DMSO could donate its O atom in many reactions to construct diverse C
O or C–OH bonds.11 However, it is very hard to donate the O atom of DMSO to heteroatoms. HBr, as the byproduct of the bromination industry, is inexpensive and readily available. The combination of DMSO and HBr could achieve many brominations12 and has been widely applied in the synthesis of natural products, drugs, and materials.13 Recently, Chen and coworkers reported a cyclization reaction enabling convenient construction of thiophene derivatives with DMSO/HBr.14 Based on our previous research on halogenation,15 herein, we disclose the Ni(OTf)2 catalyzed oxidative bromination of thiols with DMSO and HBr to afford sulfonyl bromides (Scheme 1C). The synthesis of other sulfonyl derivatives is also achieved by a one-pot procedure. Mechanistic studies reveal that HBr acts as both a nucleophile and a redox mediator to transfer the O atom of DMSO, which serves as the terminal oxidant.
Results and discussion
Compared with aromatic thiols, the oxidative bromination of aliphatic thiols is less documented. Thus, we chose octane-1-thiol 1 as the model substrate (Table 1). The oxidative bromination of 1 was performed with 48% aqueous HBr (1.5 equiv.) in DMSO at 40 °C for 12 hours under air, and no sulfonyl bromide 2 was obtained (entry 1). We then investigated the influence of solvent with 5 equiv. of DMSO as the oxidant (entries 2–4) and found that DCM was the best solvent to deliver 2 in 32% yield (entry 4). The study on the equivalent of DMSO revealed that 3.5 equiv. of DMSO as the oxidant afforded 2 in 57% yield (entries 4–6). The yield increased to 74% when acetic acid solution of HBr was employed instead of aqueous solution (entry 7). The Lewis acid catalyst was beneficial for present oxidative bromination (entries 8–10). With 10 mol% Ni(OTf)2 as the catalyst, this reaction delivered 2 in 91% yield (entry 8), and other Lewis acid catalysts such as AgOTf and Sc(OTf)3 were also suitable (entries 9 and 10). The oxidative bromination of 1 underwent smoothly under an argon atmosphere, which indicated that the molecular oxygen was not the oxidant (entry 11). Without DMSO, the sulfonyl bromide 2 was not detected, revealing the dominant role of DMSO in the present reaction (entry 12). This reaction could not proceed when HBr was replaced by NaBr, revealing that the acidic conditions were crucial for the transformation (entry 13). 40 °C is the optimal temperature for this reaction. The yield of 2 decreased to 74% at room temperature (entry 14).
Table 1 Optimization of the reaction conditionsa

|
Entry |
Catalyst |
HBr |
DMSO |
Solvent |
Yield of 2b |
The solution of 1 (0.50 mmol), catalyst, DMSO, and HBr (0.75 mmol) in anhydrous DCM (2.0 mL) was heated at 40 °C for 12 h.
Yields were determined by 1H-NMR using 1,1,2,2-tetrachloroethane as the internal standard.
Isolated yield.
Under an argon atmosphere.
At room temperature.
|
1 |
— |
48% HBr in H2O |
— |
DMSO |
0% |
2 |
— |
48% HBr in H2O |
5 equiv. |
MeCN |
0% |
3 |
— |
48% HBr in H2O |
5 equiv. |
EtOAc |
11% |
4 |
— |
48% HBr in H2O |
5 equiv. |
DCM |
32% |
5 |
— |
48% HBr in H2O |
3.5 equiv. |
DCM |
57% |
6 |
— |
48% HBr in H2O |
2 equiv. |
DCM |
27% |
7 |
— |
33% HBr in AcOH |
3.5 equiv. |
DCM |
74% |
8
|
Ni(OTf)2
|
33% HBr in AcOH
|
3.5 equiv.
|
DCM
|
91% (86%)c
|
9 |
AgOTf |
33% HBr in AcOH |
3.5 equiv. |
DCM |
84% |
10 |
Sc(OTf)3 |
33% HBr in AcOH |
3.5 equiv. |
DCM |
87% |
11d |
Ni(OTf)2 |
33% HBr in AcOH |
3.5 equiv. |
DCM |
87% |
12 |
Ni(OTf)2 |
33% HBr in AcOH |
— |
DCM |
0% |
13 |
Ni(OTf)2 |
NaBr in AcOH |
3.5 equiv. |
DCM |
0% |
14e |
Ni(OTf)2 |
33% HBr in AcOH |
3.5 equiv. |
DCM |
74% |
With the optimum conditions in hand, we explored the substrate scope of this oxidative bromination (Table 2). A broad range of aliphatic and (hetero)aromatic thiols were all converted to sulfonyl bromides smoothly with good to high yields. Several linear, branched and cyclic aliphatic thiols were valid substrates to deliver sulfonyl bromides 2–17 in satisfactory yields, regardless of the steric hindrance or chain length. The reactivity of secondary thiols (14–16) was lower than that of primary thiols, so the equivalent of DMSO and HBr should be enlarged to ensure the full conversion of substrates. Functional groups such as ether (8), ester (7, 9), arene (5), amide (13, 37) and sulfonamide (17) were tolerated in the reaction. Besides aliphatic thiols, aromatic thiols were also compatible with the current conditions (18–40). Para-substituted thiophenols bearing electron-donating groups (18–21) or weak electron-withdrawing groups (22–24) furnished the products in moderate to good yields. However, thiophenols bearing strong electron-withdrawing groups such as CN (26) or NO2 (27) suffered from a reduced reactivity, affording the corresponding disulfides as the byproduct. Meta- (28–30), ortho- (31–32), and di-substituted (34–35) thiophenols were also successfully converted to sulfonyl bromides in good yields. To our pleasure, the thioether group was unaffected under the standard conditions (33), although it's considered as a reductant motif. This result proves the good selectivity of the reaction. Besides thiophenols, 2-naphthalenethiol (36) and heteroaromatic thiols including N-oxide pyridine (38), thiophene (39), and furan (40) also worked well under the standard conditions, although the isolated yields were lower. This was probably because the products 38–40 were unstable and easily hydrolyzed to the corresponding sulfonic acids during the isolating process. Tertiary thiol failed to deliver the corresponding sulfonyl bromide under the standard conditions. Although the DMSO/HBr system showed high reactivity in bromination of electron-rich arenes,12a,c no aromatic bromination was detected in our present reaction. It is worth mentioning that without the Ni(OTf)2 catalyst, the oxidative bromination could also work smoothly to afford products with good yields in some cases.
Table 2 The oxidative bromination of thiolsa
The solution of thiol (0.50 mmol), Ni(OTf)2 (0.05 mmol), DMSO (1.75 mmol) and HBr (33% in AcOH, 0.75 mmol) in anhydrous DCM (2.0 mL) was heated at 40 °C for 12 h. Isolated yield.
DMSO (2.3 mmol) and HBr (33% in AcOH, 1 mmol) was added.
HBr (48% aqueous solution) in EtOAc (2.0 mL) was used.
DMSO (3.2 mmol) and HBr (33% in AcOH, 1.2 mmol) were used.
Without Ni(OTf)2.
|
|
As sulfonyl bromides are important intermediates to access sulfonamides. We speculated that the sulfonyl bromide generated could be converted to sulfonamide via a one-pot procedure. By adding the reaction mixture to the DCM solution of amines, the sulfonamides were prepared conveniently with good to high yields. We then evaluated different amine fragments under these conditions with octane-1-thiol or 2-phenylethane-1-thiol as model substrates. Aliphatic and aromatic amines, NH3, and bioactive molecules bearing amino group performed well in the reaction (Table 3). The chained amines including phenylethylamine (41), benzylamine (42), and cyclohexylamine (44), or cyclic amines including piperidine (46–50), azepane (51), azocane (52–53), piperazine (54–55), morpholine (56), and imidazole (57) were compatible with the standard conditions, delivering sulfonamides in moderate to good yields. α- or β-Amino acid derivatives which are common motifs in drug design were also suitable substrates (45, 58, and 60). The chained secondary amine was converted into the corresponding sulfonamide (61) with moderate yield. With the modification of reaction conditions, electron-rich anilines furnished the conversion (62–64) successfully with satisfactory yields. When NH3 was used as the nucleophile, primary sulfonamides (65–66) were obtained in moderate yields. Pleasingly, the present method was successfully applied in the late-stage sulfonylation of drugs bearing amino groups. Olefin, amide, or thioether groups (67–69) were all well tolerated. As a result, this approach provides a novel way to prepare a set of sulfonamide analogues, which are very important for drug discovery.16
Table 3 One-pot synthesis of sulfonamidesa
The solution of thiol (0.50 mmol), Ni(OTf)2 (0.05 mmol), DMSO (1.75 mmol) and HBr (33% in AcOH, 0.75 mmol) in anhydrous DCM (2.0 mL) was heated at 40 °C for 12 h. The reaction mixture was added dropwise into the solution of amine (1.5 mmol) and NEt3 (4 mmol) in DCM (1 mL) in an ice-salt bath, and then reacted for 8 h. Isolated yield.
The reaction mixture was added into the DCM (1 mL) solution of amines (5 mmol) and NEt3 (4 mmol).
The reaction mixture was added into the DCM (1 mL) solution of amine (1.5 mmol) and NEt3 (4 mmol) under a N2 atmosphere.
The reaction mixture was added into the DCM (1 mL) solution of NEt3 (4 mmol) under an NH3 atmosphere.
The reaction mixture was added into the THF (2 mL) solution of amine (5 mmol) and NEt3 (4 mmol).
|
|
Besides sulfonamides, the present oxidative bromination method was also successfully applied in the synthesis of other valuable sulfonyl derivatives (Table 4). With inorganic Bu4NF as the nucleophile, sulfonyl fluoride 70 was conveniently obtained in 60% yield. The sulfonyl azide 71 was prepared by the one-pot procedure in 77% yield with TMSN3 as the nucleophile. Several alcohols or phenols were successfully coupled with thiol to deliver sulfonates (72–76) in good yields. Olefin, ether, halogen, and ketone groups were tolerated under the reaction conditions.
Table 4 One-pot synthesis of sulfonyl derivativesa
The solution of thiol (0.50 mmol), Ni(OTf)2(0.05 mmol), DMSO (1.75 mmol) and HBr (33% in AcOH, 0.75 mmol) in anhydrous DCM (2.0 mL) was heated at 40 °C for 12 h. Then, for oxidative fluorination: the reaction mixture was added dropwise into the solution of TBAF (2.5 mmol) and NEt3 (3.5 mmol) in DCM (1 mL) in an ice-salt bath. The mixture was stirred at r.t. for 8 h. For oxidative azidation: the reaction mixture was added dropwise into the solution of TMSN3 (1.5 mmol) and NEt3 (4 mmol) in DCM (1 mL) in an ice-salt bath. The mixture was stirred at r.t. for 8 h. For oxidative esterification: the reaction mixture was added dropwise into the solution of alcohol (1.5 mmol) and NEt3 (4 mmol) in DCM (1 mL) in an ice-salt bath. The mixture was stirred at r.t. for 8 h. Isolated yield.
|
|
To test the scalability of this method, the synthesis of 18 was scaled up to 10 mmol with 72% yield (Scheme 2A). To further demonstrate the importance of our method, it was applied in the synthesis of tolbutamide, which is used in the management of type II diabetes mellitus.17 Oxidative functionalization of p-toluenethiol produced sulfonamide 77 in 71% yield, which underwent further acylation to deliver tolbutamide (Scheme 2B).18
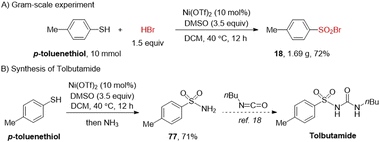 |
| Scheme 2 Gram-scale experiment and application. | |
To further understand the mechanism of the reaction, some control experiments were conducted. In order to detect the possible intermediates, we monitored the reaction at different times under standard conditions. The reaction afforded product 2 in 40% yield along with disulfide 78 in 55% yield at 1 h (Scheme 3A). As the reaction proceeded, the yield of 2 increased while disulfide 78 was consumed continuously, revealing that 78 was the intermediate of this reaction (Scheme 3A). When the reaction was performed with 0.2 equiv. of HBr, only 12% yield of 2 was obtained along with disulfide 78 (33%) and S-octyl octane-1-sulfonothioate 79 (54%) (Scheme 3B, eqn (a)). 75% yield of 78 and 23% yield of 2 were detected with 1.5 equiv. of DMSO as the oxidant (Scheme 3B, eqn (b)). These results indicate that DMSO and HBr both play important roles in this oxidative bromination reaction. Then we isolated the plausible intermediates disulfide 78 and S-octyl octane-1-sulfonothioate 79, which were subjected to the reaction system with different amounts of DMSO (Scheme 3C, eqn (c) and (d)). Disulfide 78 could not be converted to 2 without DMSO, and the yield of 2 increased with the addition of DMSO (eqn (c)). 79 could be converted to 2 (31%) and 78 (46%) by simply stirring with HBr without DMSO. An enhanced amount of 2 was produced with the addition of DMSO (eqn (d)). It should be noted that using 2 equiv. of DMSO gave the highest yield of 2. This result suggests that 78 and 79 are intermediates for the formation of 2. When DMSO18 was used in the standard reaction, the 18O-labeled product 46 was detected although the 18O-labeled ratio was low. The result reveals that DMSO participates in the oxygenation process (Scheme 3D, eqn (e)). Performing the reaction in the presence of H2O18 (5 equiv.) afforded 46 in a higher 18O-labeled ratio (eqn (f)). These results indicate that DMSO performs the oxygenation process via H2O as the intermediary, and HBr is the key mediator to transfer the oxygen atom of DMSO to the product.
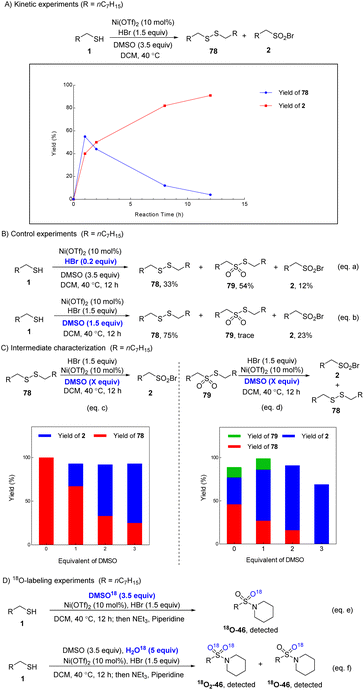 |
| Scheme 3 Mechanistic studies. | |
On the basis of the above results, a plausible mechanism was proposed (Scheme 4). Initially, HBr is oxidized by DMSO to afford H2O and DMS·Br2,12a,19 which disproportionates to produce HBrO, HBr and DMS. The Br+ species can oxidize thiol to afford disulfide and regenerate HBr simultaneously, which would be oxidized by DMSO again to deliver DMS·Br2 or HBrO. The disulfide is further oxidized to deliver R–S(O)–S(O)–R via R–S(O)–S–R by HBrO.20 As a result, HBrO serves as a direct oxidant while DMSO serves as a terminal oxidant. Next, the rearrangement of R–S(O)–S(O)–R produces R–SO2–SR through a free radical process.21 In the subsequent step, R–SO2–SR undergoes nucleophilic attack by HBr to afford the target product sulfonyl bromide and thiol with the assistance of Ni(OTf)2. The produced thiol enters a new reaction cycle to be converted to sulfonyl bromides by DMSO/HBr.
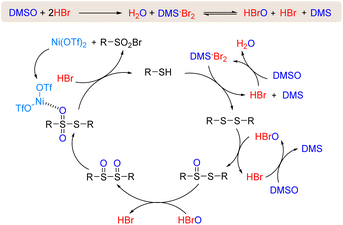 |
| Scheme 4 Proposed mechanism. | |
Conclusions
In summary, we describe a novel and general approach to access various sulfonyl derivatives through oxidative functionalization of thiol with DMSO and HBr. This method features mild reaction conditions, available reagents, and broad substrate scope, and thus proved useful in the synthesis of various sulfonyl derivatives. Mechanistic studies reveal that DMSO served as the terminal oxidant, and HBr acts as a nucleophile and a redox mediator to transfer the oxygen atom of DMSO.
Data availability
All of the necessary data had been included in the ESI.†
Author contributions
S. S. and H. W. conceived and designed the experiments; H. W. carried out most of experiments; S. S., H. W., Z. L., R. D. and N. J. analysed data; S. S. and H. W. wrote the paper; S. S. directed the project.
Conflicts of interest
There are no conflicts to declare.
Acknowledgements
Financial support from the National Natural Science Foundation of China (No. 22071005 and 22371007) and the Peking University Medicine Fund for world's leading discipline or discipline cluster development (BMU2022DJXK002).
Notes and references
-
(a) A. S. Barrow, C. J. Smedley, Q. Zheng, S. Li, J. Dong and J. E. Moses, Chem. Soc. Rev., 2019, 48, 4731–4758 RSC;
(b) B. R. Beno, K.-S. Yeung, M. D. Bartberger, L. D. Pennington and N. A. Meanwell, J. Med. Chem., 2015, 58, 4383–4438 CrossRef CAS PubMed;
(c) T. S.-B. Lou and M. C. Willis, Nat. Rev. Chem., 2022, 6, 146–162 CrossRef CAS PubMed;
(d) A. Narayanan and L. H. Jones, Chem. Sci., 2015, 6, 2650–2659 RSC.
-
(a) X.-Q. Chu, D.-H. Ge, Y.-Y. Cui, Z.-L. Shen and C.-J. Li, Chem. Rev., 2021, 121, 12548–12680 CrossRef CAS PubMed;
(b) L. Kvaerno, M. Werder, H. Hauser and E. M. Carreira, Org. Lett., 2005, 7, 1145–1148 CrossRef PubMed;
(c) S. R. Dubbaka and P. Vogel, Angew. Chem., Int. Ed., 2005, 44, 7674–7684 CrossRef CAS PubMed;
(d) S. R. Dubbaka and P. Vogel, J. Am. Chem. Soc., 2003, 125, 15292–15293 CrossRef CAS PubMed;
(e) X. Zhao, E. Dimitrijevic and V. M. Dong, J. Am. Chem. Soc., 2009, 131, 3466–3467 CrossRef CAS PubMed;
(f) O. Saidi, J. Marafie, A. E. W. Ledger, P. M. Liu, M. F. Mahon, G. Kociok-Koehn, M. K. Whittlesey and C. G. Frost, J. Am. Chem. Soc., 2011, 133, 19298–19301 CrossRef CAS PubMed.
-
(a) K. A. Scott and J. T. Njardarson, Top. Curr. Chem., 2018, 376, 1 CrossRef CAS PubMed;
(b) P. Bhutani, G. Joshi, N. Raja, N. Bachhav, P. K. Rajanna, H. Bhutani, A. T. Paul and R. Kumar, J. Med. Chem., 2021, 64, 2339–2381 CrossRef CAS PubMed.
-
(a) G. Qiu, K. Zhou and J. Wu, Chem. Commun., 2018, 54, 12561–12569 RSC;
(b) J. A. Andrews and M. C. Willis, Synthesis, 2022, 54, 1695–1707 CrossRef CAS;
(c) Y. Chen, P. R. D. Murray, A. T. Davies and M. C. Willis, J. Am. Chem. Soc., 2018, 140, 8781–8787 CrossRef CAS PubMed;
(d) V. T. Nguyen, G. C. Haug, V. D. Nguyen, N. T. H. Vuong, H. D. Arman and O. V. Larionov, Chem. Sci., 2021, 12, 6429–6436 RSC;
(e) Y. Chen and M. C. Willis, Chem. Sci., 2017, 8, 3249–3253 RSC;
(f) Y. Meng, M. Wang and X. Jiang, Angew. Chem., Int. Ed., 2020, 59, 1346–1353 CrossRef CAS PubMed.
-
(a) S. P. Blum, K. Hofman, G. Manolikakes and S. R. Waldvogel, Chem. Commun., 2021, 57, 8236–8249 RSC;
(b) S. P. Blum, T. Karakaya, D. Schollmeyer, A. Klapars and S. R. Waldvogel, Angew. Chem., Int. Ed., 2021, 60, 5056–5062 CrossRef CAS PubMed;
(c) S. P. Blum, D. Schollmeyer, M. Turks and S. R. Waldvogel, Chem.–Eur. J., 2020, 26, 8358–8362 CrossRef CAS PubMed;
(d) S. P. Blum, L. Schaffer, D. Schollmeyer and S. R. Waldvogel, Chem. Commun., 2021, 57, 4775–4778 RSC;
(e) Z. Li, L. Jiao, Y. Sun, Z. He, Z. Wei and W.-W. Liao, Angew. Chem., Int. Ed., 2020, 59, 7266–7270 CrossRef CAS PubMed;
(f) C. Zhang, M. Yang, Y. Qiu, M. Song, H. Wang, M. Yang, W. Xie, J. Wu and S. Ye, Chem. Sci., 2022, 13, 11785–11791 RSC.
-
(a) D. Kaiser, I. Klose, R. Oost, J. Neuhaus and N. Maulide, Chem. Rev., 2019, 119, 8701–8780 CrossRef CAS PubMed;
(b) D.-K. Kim, H.-S. Um, H. Park, S. Kim, J. Choi and C. Lee, Chem. Sci., 2020, 11, 13071–13078 RSC;
(c) Q. Zhou, A. Ruffoni, R. Gianatassio, Y. Fujiwara, E. Sella, D. Shabat and P. S. Baran, Angew. Chem., Int. Ed., 2013, 52, 3949–3952 CrossRef CAS PubMed;
(d) C. S. Richards-Taylor, D. C. Blackmore and M. C. Willis, Chem. Sci., 2014, 5, 222–228 RSC;
(e) P. S. Fier and K. M. Maloney, J. Am. Chem. Soc., 2019, 141, 1441–1445 CrossRef CAS PubMed;
(f) P. S. Fier, S. Kim and K. M. Maloney, J. Am. Chem. Soc., 2019, 141, 18416–18420 CrossRef CAS PubMed;
(g) G. K. S. Prakash, C. Ni, F. Wang, J. Hu and G. A. Olah, Angew. Chem., Int. Ed., 2011, 50, 2559–2563 CrossRef CAS PubMed ; for approachs generating sulfinate salts or sulfinate radicals in situ, see: ;
(h) M. Wang and X. Jiang, Chem. Rec., 2021, 21, 3338–3355 CrossRef CAS PubMed;
(i) S. Ye, M. Yang and J. Wu, Chem. Commun., 2020, 56, 4145–4155 RSC;
(j) T. Liu, D. Zheng, Z. Li and J. Wu, Adv. Synth. Catal., 2017, 359, 2653–2659 CrossRef CAS;
(k) X. Marset, J. Torregrosa-Crespo, R. M. Martinez-Espinosa, G. Guillena and D. J. Ramon, Green Chem., 2019, 21, 4127–4132 RSC;
(l) M. Magre and J. Cornella, J. Am. Chem. Soc., 2021, 143, 21497–21502 CrossRef CAS PubMed;
(m) E. J. Emmett, B. R. Hayter and M. C. Willis, Angew. Chem., Int. Ed., 2014, 53, 10204–10208 CrossRef CAS PubMed;
(n) M. W. Johnson, S. W. Bagley, N. P. Mankad, R. G. Bergman, V. Mascitti and F. D. Toste, Angew. Chem., Int. Ed., 2014, 53, 4404–4407 CrossRef CAS PubMed;
(o) A. Shavnya, K. D. Hesp, V. Mascitti and A. C. Smith, Angew. Chem., Int. Ed., 2015, 54, 13571–13575 CrossRef CAS PubMed;
(p) A. S. Deeming, C. J. Russell and M. C. Willis, Angew. Chem., Int. Ed., 2016, 55, 747–750 CrossRef CAS PubMed;
(q) T. S.-B. Lou, S. W. Bagley and M. C. Willis, Angew. Chem., Int. Ed., 2019, 58, 18859–18863 CrossRef CAS PubMed;
(r) P. K. T. Lo, Y. Chen and M. C. Willis, ACS Catal., 2019, 9, 10668–10673 CrossRef CAS;
(s) Y. Meng, M. Wang and X. Jiang, Angew. Chem., Int. Ed., 2020, 59, 1346–1353 CrossRef CAS PubMed;
(t) D. Zheng, Y. An, Z. Li and J. Wu, Angew. Chem., Int. Ed., 2014, 53, 2451–2454 CrossRef CAS PubMed;
(u) Y. Meng, M. Wang and X. Jiang, CCS Chem., 2021, 3, 17–24 CrossRef CAS;
(v) Y. Li, S. Chen, M. Wang and X. Jiang, Angew. Chem., Int. Ed., 2020, 59, 8907–8911 CrossRef CAS PubMed.
-
(a) T. Q. Davies and M. C. Willis, Org. Lett., 2020, 22, 9495–9499 CrossRef CAS PubMed;
(b) S. M. Hell and T. Noël, J. Am. Chem. Soc., 2020, 142, 720–725 CrossRef CAS PubMed;
(c) X. Nie, T. Xu, J. Song, A. Devaraj, B. Zhang, Y. Chen and S. Liao, Angew. Chem., Int. Ed., 2021, 60, 3956–3960 CrossRef CAS PubMed;
(d) P. Wang, H. Zhang, X. Nie, T. Xu and S. Liao, Nat. Commun., 2022, 13, 3370 CrossRef CAS PubMed;
(e) P. K. T. Lo and M. C. Willis, J. Am. Chem. Soc., 2021, 143, 15576–15581 CrossRef CAS PubMed;
(f) S. M. Hell, C. F. Meyer, A. Misale, J. B. I. Sap, K. E. Christensen, M. C. Willis, A. A. Trabanco and V. Gouverneur, Angew. Chem., Int. Ed., 2020, 59, 11620–11626 CrossRef CAS PubMed;
(g) T. Guo, G. Meng, X. Zhan, Q. Yang, T. Ma, L. Xu, K. B. Sharpless and J. Dong, Angew. Chem., Int. Ed., 2018, 57, 2605–2610 CrossRef CAS PubMed;
(h) H.-L. Qin, Q. Zheng, G. A. L. Bare, P. Wu and K. B. Sharpless, Angew. Chem., Int. Ed., 2016, 55, 14155–14158 CrossRef CAS PubMed;
(i) G. Meng, T. Guo, T. Ma, J. Zhang, Y. Shen, K. B. Sharpless and J. Dong, Nature, 2019, 574, 86–89 CrossRef CAS PubMed;
(j) J. E. Erchinger, R. Hoogesteger, R. Laskar, S. Dutta, C. Hümpel, D. Rana, C. G. Daniliuc and F. Glorius, J. Am. Chem. Soc., 2023, 145, 2364–2374 CrossRef CAS PubMed.
-
(a) S. Madabhushi, R. Jillella, V. Sriramoju and R. Singh, Green Chem., 2014, 16, 3125–3131 RSC;
(b) X. Huang, J. Wang, Z. Ni, S. Wang and Y. Pan, Chem. Commun., 2014, 50, 4582–4584 RSC;
(c) A. Tota, S. St John-Campbell, E. L. Briggs, G. O. Estevez, M. Afonso, L. Degennaro, R. Luisi and J. A. Bull, Org. Lett., 2018, 20, 2599–2602 CrossRef CAS PubMed;
(d) M. Zhu, W. Wei, D. Yang, H. Cui, L. Wang, G. Meng and H. Wang, Org. Biomol. Chem., 2017, 15, 4789–4793 RSC;
(e) J.-B. Feng and X.-F. Wu, Org. Biomol. Chem., 2016, 14, 6951–6954 RSC;
(f) M. Kirihara, S. Naito, Y. Ishizuka, H. Hanai and T. Noguchi, Tetrahedron Lett., 2011, 52, 3086–3089 CrossRef CAS;
(g) H. Veisi, R. Ghorbani-Vaghei, S. Hemmati and J. Mahmoodi, Synlett, 2011, 16, 2315–2320 CrossRef;
(h) C. Silva-Cuevas, C. Perez-Arrieta, L. A. Polindara-Garcia and J. Armando Lujan-Montelongo, Tetrahedron Lett., 2017, 58, 2244–2247 CrossRef CAS;
(i) K. Bahrami, M. M. Khodaei and M. Soheilizadt, J. Org. Chem., 2009, 74, 9287–9291 CrossRef CAS PubMed;
(j) P. Vedso, P. H. Olesen and T. Hoeg-Jensen, Synlett, 2004, 5, 892–894 CrossRef;
(k) K. Bahrami, M. M. Khodaei and M. Soheilizad, Synlett, 2009, 17, 2773–2776 CrossRef;
(l) T. Okada, H. Matsumuro, T. Iwai, S. Kitagawa, K. Yamazaki, T. Akiyama, T. Asawa, Y. Sugiyama, Y. Kimura and M. Kirihara, Chem. Lett., 2015, 44, 185–187 CrossRef;
(m) M. Jereb and L. Hribernik, Green Chem., 2017, 19, 2286–2295 RSC;
(n) M. Kirihara, S. Yamahara, T. Okada, H. Matsumuro, Y. Kinoshita, A. Kitajima, Y. Takamura, T. Odagiri, T. Asawa, Y. Sugiyama and Y. Kimura, Synthesis, 2022, 54, 4120–4128 CrossRef CAS;
(o) E. Hayashi, Y. Yamaguchi, Y. Kita, K. Kamata and M. Hara, Chem. Commun., 2020, 56, 2095–2098 RSC;
(p) A. K. Singh, H. Yi, G. Zhang, C. Bian, P. Pei and A. Lei, Synlett, 2017, 28, 1558–1563 CrossRef CAS.
-
(a) G. Laudadio, E. Barmpoutsis, C. Schotten, L. Struik, S. Govaerts, D. L. Browne and T. Noël, J. Am. Chem. Soc., 2019, 141, 5664–5668 CrossRef CAS PubMed;
(b) G. Laudadio, A. d. A. Bartolomeu, L. M. H. M. Verwijlen, Y. Cao, K. T. de Oliveira and T. Noël, J. Am. Chem. Soc., 2019, 141, 11832–11836 CrossRef CAS PubMed.
-
(a) X.-F. Wu and K. Natte, Adv. Synth. Catal., 2016, 358, 336–352 CrossRef CAS;
(b) Z. Tashrifi, M. M. Khanaposhtani, B. Larijani and M. Mahdavi, Adv. Synth. Catal., 2020, 362, 65–86 CrossRef CAS.
-
(a) N. Kornblum, J. W. Powers, G. J. Anderson, W. J. Jones, H. O. Larson, O. Levand and W. M. Weaver, J. Am. Chem. Soc., 1957, 79, 6562 CrossRef CAS;
(b) R. Xu, J. P. Wan, H. Mao and Y. Pan, J. Am. Chem. Soc., 2010, 132, 15531–15533 CrossRef CAS PubMed;
(c) Y. Ashikari, A. Shimizu, T. Nokami and J. Yoshida, J. Am. Chem. Soc., 2013, 135, 16070–16073 CrossRef CAS PubMed;
(d) Y. Ashikari, T. Nokami and J. Yoshida, J. Am. Chem. Soc., 2011, 133, 11840–11843 CrossRef CAS PubMed;
(e) R. N. Reddi, P. K. Prasad and A. Sudalai, Angew. Chem., Int. Ed., 2015, 54, 14150–14153 CrossRef CAS PubMed;
(f) Y.-F. Liang, X. Li, X. Wang, M. Zou, C. Tang, Y. Liang, S. Song and N. Jiao, J. Am. Chem. Soc., 2016, 138, 12271–12277 CrossRef CAS PubMed;
(g) S. Song, X. Huang, Y.-F. Liang, C. Tang, X. Li and N. Jiao, Green Chem., 2015, 17, 2727–2731 RSC;
(h) X. Wu, Q. Gao, S. Liu and A. Wu, Org. Lett., 2014, 16, 2888–2891 CrossRef CAS PubMed;
(i) Q. Gao, X. Wu, S. Liu and A. Wu, Org. Lett., 2014, 16, 1732–1735 CrossRef CAS PubMed;
(j) N. Mupparapu, S. Khan, S. Battula, M. Kushwaha, A. P. Gupta, Q. N. Ahmed and R. A. Vishwakarma, Org. Lett., 2014, 16, 1152–1155 CrossRef CAS PubMed.
-
(a) G. Majetich, R. Hicks and S. Reister, J. Org. Chem., 1997, 62, 4321–4326 CrossRef CAS PubMed;
(b) S. Song, X. Li, X. Sun, Y. Yuan and N. Jiao, Green Chem., 2015, 17, 3285–3289 RSC;
(c) S. Song, X. Sun, X. Li, Y. Yuan and N. Jiao, Org. Lett., 2015, 17, 2886–2889 CrossRef CAS PubMed;
(d) M. Karki and J. Magolan, J. Org. Chem., 2015, 80, 3701–3707 CrossRef CAS PubMed.
-
(a) S.-L. Fang, M.-X. Jiang, S. Zhang, Y.-J. Wu and B.-F. Shi, Org. Lett., 2019, 21, 4609–4613 CrossRef CAS PubMed;
(b) J.-Y. Kim, D.-H. Kim, T.-H. Jeon, W.-H. Kim and C.-G. Cho, Org. Lett., 2017, 19, 4688–4691 CrossRef CAS PubMed;
(c) D. Kamakura, H. Todoroki, D. Urabe, K. Hagiwara and M. Inoue, Angew. Chem., Int. Ed., 2020, 59, 479–486 CrossRef CAS PubMed.
- H. Liu, G.-C. He, C.-Y. Zhao, X.-X. Zhang, D.-W. Ji, Y.-C. Hu and Q.-A. Chen, Angew. Chem., Int. Ed., 2021, 60, 24284–24291 CrossRef CAS PubMed.
-
(a) S. Song, X. Li, J. Wei, W. Wang, Y. Zhang, L. Ai, Y. Zhu, X. Shi, X. Zhang and N. Jiao, Nat. Catal., 2020, 3, 107–115 CrossRef CAS;
(b) W. Wang, X. Li, X. Yang, L. Ai, Z. Gong, N. Jiao and S. Song, Nat. Commun., 2021, 12, 3873 CrossRef CAS PubMed;
(c) W. Wang, X. Yang, R. Dai, Z. Yan, J. Wei, X. Dou, X. Qiu, H. Zhang, C. Wang, Y. Liu, S. Song and N. Jiao, J. Am. Chem. Soc., 2022, 144, 13415–13425 CrossRef CAS PubMed.
- D. C. Blakemore, L. Castro, I. Churcher, D. C. Rees, A. W. Thomas, D. M. Wilson and A. Wood, Nat. Chem., 2018, 10, 383–394 CrossRef CAS PubMed.
-
(a) M. N. Feinglos and H. E. Lebovitz, Nature, 1978, 276, 184–185 CrossRef CAS PubMed;
(b) H. E. Lebovitz and M. N. Feinglos, Diabetes Care, 1978, 1, 189–198 CrossRef CAS PubMed.
- J. Cervelló and T. Sastre, Synthesis, 1990, 3, 221–222 CrossRef.
-
(a) K. Mislow, J. T. Melillo, T. Simmons and A. L. Ternay, J. Am. Chem. Soc., 1964, 86, 1452–1453 CrossRef CAS;
(b) M. B. Floyd, M. T. Du, P. F. Fabio, L. A. Jacob and B. D. Johnson, J. Org. Chem., 1985, 50, 5022–5027 CrossRef CAS.
-
(a) M. M. Chau and J. L. Kice, J. Am. Chem. Soc., 1976, 98, 7711–7716 CrossRef CAS;
(b) S. Oae, Y. H. Kim, T. Takata and D. Fukushima, Tetrahedron Lett., 1977, 13, 1995–1998 Search PubMed.
- F. Freeman, Chem. Rev., 1984, 84, 117–135 CrossRef CAS.
|
This journal is © The Royal Society of Chemistry 2023 |
Click here to see how this site uses Cookies. View our privacy policy here.