DOI:
10.1039/D3SC01048A
(Edge Article)
Chem. Sci., 2023,
14, 8315-8320
Bimetallic tandem catalysis-enabled enantioselective cycloisomerization/carbonyl–ene reaction for construction of 5-oxazoylmethyl α-silyl alcohol†
Received
24th February 2023
, Accepted 6th July 2023
First published on 7th July 2023
Abstract
A bimetallic tandem catalysis-enabled enantioselective cycloisomerization/carbonyl–ene reaction was developed. The reaction proceeded well with a broad range of N-propargylamides and acylsilanes, affording the target chiral 5-oxazoylmethyl α-silyl alcohols in up to 95% yield and 99% ee under mild conditions. Importantly, this facile protocol was available for the late-stage modification of several bioactive molecules. Based on the mechanistic study and control experiments, a possible catalytic cycle and transition state are proposed to elucidate the reaction process and enantioinduction.
Introduction
Organosilicon compounds are widely applied in synthetic chemistry, pharmaceutical chemistry and materials science because of their unique physicochemical properties.1 For instance, the incorporation of silicon into bioactive molecules may increase the lipophilicity and potency in comparison to the parent (Fig. 1).1b,2 The related discovery and evaluation have been demonstrated by Schreiber and others.2
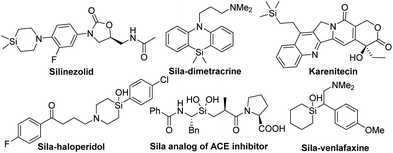 |
| Fig. 1 Selected examples of silicon-containing bioactive molecules and drugs. | |
As a special subset, chiral tertiary α-silyl alcohols serve as important building blocks in versatile transformations for the construction of complex molecules.3 Asymmetric nucleophilic addition to acylsilanes4 containing an sp2 carbon atom binding to both a silicon and an oxygen atom is one of the most direct and efficient accesses to optically active α-silyl alcohols (Scheme 1a),4 and has attracted considerable attention in the past two decades. A series of intriguing studies were realized by several groups, wherein highly active nucleophilic reagents, such as organometallic reagents,5a–e,h enamine,5f enolate,5g or thiol,5i were commonly employed to offset the poor reactivity of the acylsilanes.
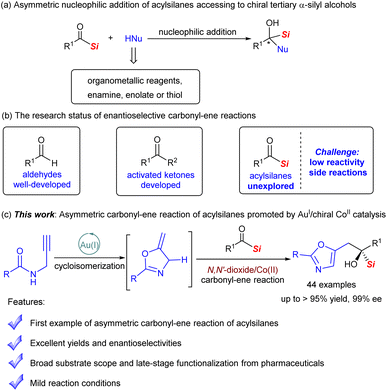 |
| Scheme 1 Catalytic asymmetric reactions of acylsilanes for the construction of chiral α-silyl alcohol and the research status of enantioselective carbonyl–ene reactions. | |
As we know, the catalytic asymmetric carbonyl–ene reaction6 between a carbonyl compound and an alkene bearing an allylic hydrogen is a powerful and stereocontrolled approach to prepare chiral homoallyl alcohols through a six-membered pericyclic process. Along this line, the carbonyl–ene reaction of acylsilanes, a type of unusual carbonyl compound (the enophile), may provide an ideal route to functionalized chiral tertiary α-silyl alcohols. Nevertheless, to the best of our knowledge, although remarkable advances concerning the substance scope of aldehydes and activated ketones have been achieved, few examples of acylsilanes are found, which is highly challenging due to the low reactivity arising from the steric and electron-donating effects of the bulky trisubstituted silyl (Scheme 1b).7 Meanwhile, side reactions may occur, including competing [1,2]-Brook rearrangement3e,4f,8 and possible generation of carbenes4f,p,9 from acylsilanes. On the other hand, in view of the importance of the oxazole skeleton,10 we envisioned that the carbonyl–ene reaction of alkylideneoxazoline with acylsilanes may be favorable by means of the driving aromatization of the former and the assistance of a chiral Lewis acid catalyst lowering the LUMO energy of the latter. Herein, we report the first example of an enantioselective carbonyl–ene reaction of acylsilanes with easily available N-propargylamides in one-pot catalyzed by the bimetallic catalyst system consisting of Au(I) and chiral N,N′-dioxide/Co(II) complex,11,12 producing a chiral 5-oxazoylmethyl α-silyl alcohol framework with high yield and enantioselectivity under mild conditions (Scheme 1c).
Our investigation of the carbonyl–ene reaction began with silyl glyoxylates A1 and N-propargylamide B1 as model substrates to optimize the reaction conditions (Table 1). The reaction exhibited low reactivity in dichloromethane (DCM) at 35 °C and afforded the desired chiral 5-oxazoylmethyl-substituted α-silyl alcohol C1 with 7% yield by using IPrAuCl/AgNTf2 (1
:
1, 5 mol%) as the catalyst (Table 1, entry 1). Next, a multi-metallic catalyst system via the combination of IPrAuCl/AgNTf2 and a chiral Lewis acid catalyst was investigated. The screening of Lewis acids coordinating with an L-proline-derived chiral N,N′-dioxide ligand13L3-PrEt3 showed that Sc(OTf)3 and Fe(OTf)2 gave extremely low yields and ee values (Table 1, entries 2 and 3), but Zn(OTf)2 promoted this reaction resulting in 65% yield with 96% ee (Table 1, entry 4), and the Co(OTf)2/L3-PrEt3 complex provided the best result (Table 1, entry 5, 84% yield with 99% ee). The chiral N,N′-dioxide ligands were then evaluated (Table 1, entries 6–8, see Table S4 in the ESI for details†); a higher yield (88%) and the same ee value were obtained with the use of the L-pipecolic acid-derived L3-PiPr2, which possessed larger steric hindrance at the 2,6-position of the phenyl group of the amide unit (Table 1, entry 8). Comparatively, when other representative chiral ligands, such as chiral BINOL, bis(oxazoline) (tBu-BOX), and pyridine-2,6-bis(oxazoline) (iPr-Pybox) were used instead, only moderate yields with no more than 4% ee were achieved (Table 1, entries 9–11). On decreasing the temperature to 20 °C, a reduced yield (74%) was observed (Table 1, entry 12). When the reaction was performed without IPrAuCl/AgNTf2, the reaction would not happen (Table 1, entry 13), and the yield decreased significantly in the absence of AgNTf2 (Table 1, entry 14), suggesting the important roles of Au(I) and the counterion NTf2−.14 It is worth mentioning that the [1,2]-Brook rearrangement product C1′ (3%) was detected and the yield of C1 decreased significantly in the presence of a small amount of water (Table 1, entry 15). Other reaction parameters, including the solvent, additive and so on were also explored, no better results were obtained (see the ESI for details†).
Table 1 Optimization of the reaction conditionsa
Results and discussion
With the optimized reaction conditions in hand (Table 1, entry 8), the scope of silyl glyoxylate A was investigated with B1 as the ene-reaction precursor. As shown in Table 2, changing the ester group unit from tert-butyl to cyclohexyl and benzyl resulted in an excellent ee value but gradually reduced yields (entries 1–3, C2, 81% yield; C3, 57% yield). The substituents located at the aryl group of the silyl unit had little effect on the reactivity and enantioselectivity, and the desired nearly optically pure products C5–C10 were obtained in 77–88% yields (entries 5–10) except for C4 bearing a methyl group at the 2-position, which might be attributed to the increased steric hindrance (entry 4, 71% yield and 88% ee). The 2-naphthyl substituted silyl glyoxylate also worked well, delivering C11 in 82% yield with 99% ee (entry 11). Nevertheless, the enantioselectivity decreased dramatically for A12 containing a Ph2MeSi group, giving the corresponding product C12 in 73% yield with 29% ee (entry 12). By contrast, the alkyl silyl (TBS, TIPS, TES) substituted substrates exhibited poor reactivities and no reactions occurred even at a higher temperature (80 °C, see Fig. S1 in the ESI for details†).
Table 2 Substrate scope of silyl glyoxylatesa
Subsequently, various N-propargylamides were evaluated (Scheme 2). Regardless of the electronic effect and steric effect of the substituents on the aryl ring, the N-propargylamides were transformed into the corresponding products in high yields with excellent enantioselectivities (C13–C20, 76–95% yields, 96–99% ee). A gram-scale experiment was also carried out, providing the product C20 in 88% yield (1.06 g) with a maintained ee value, and its absolute configuration was determined to be R by X-ray crystallography analysis.15 The 2-naphthyl, ferrocenyl and heteroaromatic substrates worked as well, delivering the optically pure C21–C25 in 76–90% yields. However, no products were observed when introducing pyridine substituted propargylamides as well as urea, thiourea and guanidine-derived products (see Fig. S2 in the ESI for details†). Extensive examination of a wide range of B revealed that the N-propargylamides bearing primary, secondary and tertiary alkyl groups were also tolerated without the influence of the stereocontrol (C26–C33, 34–84% yields, 88–99% ee). Alkenyl-substituted propargylamide showed high reactivity but with decreased enantioselectivity (C34, 92% yield, 83% ee). To further probe the potential utility of this approach, a variety of N-propargylamide derivatives from drug molecules were employed for late-stage modification, furnishing the chiral modified products (C35–C44) with excellent stereocontrol.
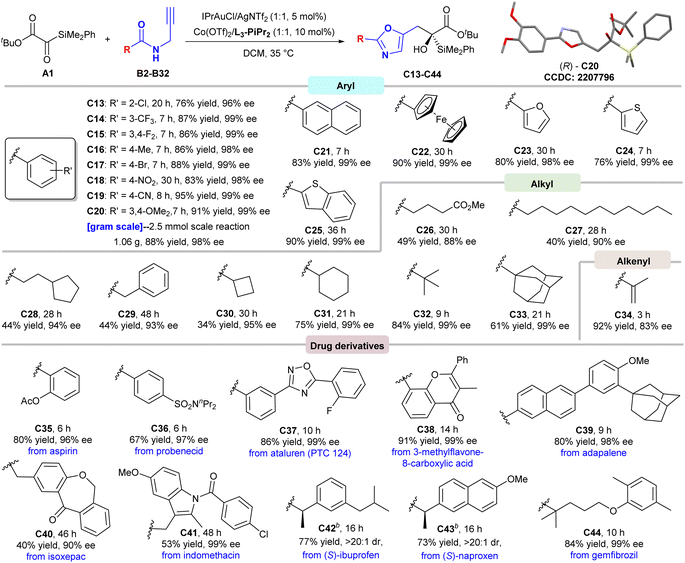 |
| Scheme 2 The substrate scope of N-propargylamides. a Unless otherwise noted, all reactions were performed with IPrAuCl/AgNTf2 (1 : 1, 5 mol%), Co(OTf)2/L3-PiPr2 (1 : 1, 10 mol%), A1 (0.10 mmol), B (0.12 mmol) in DCM (1.0 mL) at 35 °C. Yield of the isolated product. Determined by HPLC analysis on a chiral stationary phase. bEnt-L3-PiPr2 was used instead of L3-PiPr2. | |
Next, some mechanistic studies were conducted to gain an insight into the tandem reaction process. UV-visible absorption spectroscopy indicated a strong interaction between A1 and L3-PiPr2/Co(II) (see Fig. S4 in the ESI for details†). Investigation of the relationship between the ee value of L3-PiPr2 and that of C1 showed a self-evident linear effect, implying that the catalytically active species was likely to be the monomeric complex of Co(OTf)2 and L3-PiPr2 (see Fig. S5 in the ESI for details†). Moreover, the alkylideneoxazoline intermediate D was prepared and tested with the L3-PiPr2/Co(OTf)2 complex as the sole catalyst, the result (92% yield and 99% ee) was similar to that of the one-pot approach (Scheme 3a). Meanwhile, the React IR experiment revealed that intermediate D was formed rapidly within the initial ten minutes and decreased gradually over the reaction, suggesting that the ene-reaction may be involved in the rate-determining step (see Fig. S6 in the ESI for details†). In addition, to make clear the formation of Brook rearrangement product C1′, the conversion experiment of carbonyl–ene product C1 was performed, and it was found that no C1′ was observed with or without addition of H2O (Scheme 3b), indicating that the formation of the carbonyl–ene product and the formation of the Brook rearrangement product were competing processes, and C1′ may be generated through a Prins reaction process (see Fig. S3 in the ESI for details†).7
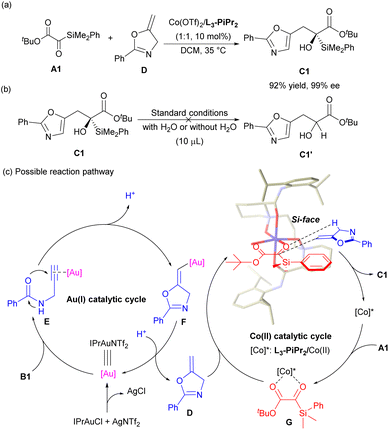 |
| Scheme 3 Control experiments and possible reaction pathway. | |
Based on the above experimental results, the absolute configuration of the product and the X-ray single-crystal structure of N,N′-dioxide/Co(II) complex,16 a possible mechanism involving an Au(I) catalytic cycle and a Co(II) catalytic cycle is proposed (Scheme 3c). Initially, IPrAuNTf2 in situ generated from IPrAuCl and AgNTf2 served as a π-acid to combine with N-propargylamide B1 and formed the intermediate E, which underwent 5-endo-dig cyclization to deliver the (E)-vinylgold intermediate F. The subsequent protodeauration of F would lead to the alkylideneoxazoline D. On the other hand, both the oxygen atoms of the amide and N-oxide portions of the ligand coordinated with the central Co(II) to form the L3-PiPr2/Co(OTf)2 complex in a tetradentate manner, which acted as a chiral Lewis acid catalyst to activate the silyl glyoxylates A1via bidentate coordination with the two oxygen atoms of the carbonyl groups. Mechanistically, the carbonyl–ene reaction considered as a concerted, pericyclic reaction with a six-membered ring transition state occurred. The intermediate D approached from the Si face of A1 because the Re face was shielded by the 2,6-diisopropylphenyl group of the ligand, producing (R)-C1 and regenerating the L3-PiPr2/Co(OTf)2 catalyst.
Conclusions
In summary, we reported the first example of enantioselective carbonyl–ene reaction of acylsilanes with N-propargylamides, broadening the reaction type of acylsilanes and the substrate scope of the carbonyl–ene reaction, providing the chiral 5-oxazoylmethyl α-silyl alcohols with high yields and good to excellent enantioselectivities. Importantly, this facile protocol was available for the late-stage modification of several bioactive molecules. A catalytic cycle and transition state were proposed by the mechanistic studies to elucidate the reaction process and enantioinduction. Further investigations of acylsilanes are currently ongoing in our laboratory.
Data availability
Further details of experimental procedure, 1H, 13C{1H} and 19F {1H} NMR, HPLC spectra, X-ray crystallographic data for C20 are available in the ESI.†
Author contributions
X. P. S. performed experiments and prepared the ESI† and the paper. Y. H. M. participated in structure characterization and discussion. S. Y. L. repeated some experiments. W. D. C. and X. H. L. helped with modifying the paper and ESI.† W. D. C. and X. M. F. conceived and directed the project.
Conflicts of interest
There are no conflicts to declare.
Acknowledgements
The authors acknowledge financial support from the National Natural Science Foundation of China (No. 22071160 and 92256302) and Sichuan Science and Technology Program (no. 2021YJ0562). We are grateful to Dr Yuqiao Zhou (Sichuan University) for the X-ray single crystal diffraction analysis.
Notes and references
-
(a)
K. Miura and A. Hosomi in Main Group Metals in Organic Synthesis, ed. H. Yamamoto and K. Oshima, Wiley-VCH, Weinheim, 2005, p. 409 Search PubMed;
(b) G. K. Min, D. Hernandez and T. Skrydstrup, Acc. Chem. Res., 2013, 46, 457–470 CrossRef CAS PubMed;
(c) T. Komiyama, Y. Minami and T. Hiyama, ACS Catal., 2017, 7, 631–651 CrossRef CAS;
(d)
Organosilicon Chemistry: Novel Approaches and Reactions, ed. T. Hiyama and M. Oestreich, Wiley-VCH, Weinheim, 2019 Search PubMed.
-
(a) G. A. Showell and J. S. Mills, Drug Discovery Today, 2003, 8, 551–556 CrossRef CAS;
(b) A. K. Franz, P. D. Dreyfuss and S. L. Schreiber, J. Am. Chem. Soc., 2007, 129, 1020–1021 CrossRef CAS PubMed;
(c) N. A. Meanwell, J. Med. Chem., 2011, 54, 2529–2591 CrossRef CAS PubMed;
(d) A. K. Franz and S. O. Wilson, J. Med. Chem., 2013, 56, 388–405 CrossRef CAS;
(e) R. Ramesh and D. S. Reddy, J. Med. Chem., 2018, 61, 3779–3798 CrossRef CAS PubMed.
-
(a) A. G. Brook, Acc. Chem. Res., 1974, 7, 77–84 CrossRef CAS;
(b) W. H. Moser, Tetrahedron, 2001, 57, 2065–2084 CrossRef CAS;
(c) G. R. Boyce, S. N. Greszler, J. S. Johnson, X. Linghu, J. T. Malinowski, D. A. Nicewicz, A. D. Satterfield, D. C. Schmitt and K. M. Steward, J. Org. Chem., 2012, 77, 4503–4515 CrossRef CAS;
(d) G. Eppe, D. Didier and I. Marek, Chem. Rev., 2015, 115, 9175–9206 CrossRef CAS PubMed;
(e) N. Lee, C.-H. Tan and D. Leow, Asian J. Org. Chem., 2019, 8, 25–31 CrossRef CAS;
(f) K. Yabushita, A. Yuasa, K. Nagao and H. Ohmiya, J. Am. Chem. Soc., 2019, 141, 113–117 CrossRef CAS;
(g)
L. Gao, W. Yang, Y. Wu and Z. Song, in Organic Reactions, ed. P. A. Evans, et al., Wiley-VCH, Weinheim, 2020, p. 5 Search PubMed.
- For reviews and selected examples on acylsilanes, see:
(a) D. A. Nicewicz and J. S. Johnson, J. Am. Chem. Soc., 2005, 127, 6170–6171 CrossRef CAS PubMed;
(b) N. Arai, K. Suzuki, S. Sugizaki, H. Sorimachi and T. Ohkuma, Angew. Chem., Int. Ed., 2008, 47, 1770–1773 CrossRef CAS PubMed;
(c) J. Matsuo, Y. Hattori and H. Ishibashi, Org. Lett., 2010, 12, 2294–2297 CrossRef CAS;
(d) H.-J. Zhang, D. L. Priebbenow and C. Bolm, Chem. Soc. Rev., 2013, 42, 8540–8571 RSC;
(e) M. Bouzrati-Zerelli, J. Kirschner, C. P. Fik, M. Maier, C. Dietlin, F. Morlet-Savary, J. P. Fouassier, J.-M. Becht, J. E. Klee and J. Lalevée, Macromolecules, 2017, 50, 6911–6923 CrossRef CAS;
(f) D. L. Priebbenow, Adv. Synth. Catal., 2020, 362, 1927–1946 CrossRef CAS;
(g) X. C. Wang, F. P. Liu, Y. J. Li, Z. J. Yan, Q. Qiang and Z.-Q. Rong, ChemCatChem, 2020, 12, 5022–5033 CrossRef CAS;
(h) S. Nakatani, Y. Ito, S. Sakurai, T. Kodama and M. Tobisu, J. Org. Chem., 2020, 85, 7588–7594 CrossRef CAS PubMed;
(i) Y. Ueda, Y. Masuda, T. Iwai, K. Imaeda, H. Takeuchi, K. Ueno, M. Gao, J.-y. Hasegawa and M. Sawamura, J. Am. Chem. Soc., 2022, 144, 2218–2224 CrossRef CAS;
(j) C. H. Tang, W. Wang, G. Y. Luo, C. Y. Song, Z. W. Bao, P. Li, G. F. Hao, Y. R. Chi and Z. C. Jin, Angew. Chem., Int. Ed., 2022, 61, e202206961 CAS;
(k) S. Sakurai, T. Inagaki, T. Kodama, M. Yamanaka and M. Tobisu, J. Am. Chem. Soc., 2022, 144, 1099–1105 CrossRef CAS;
(l) T. Inagaki, S. Sakurai, M. Yamanaka and M. Tobisu, Angew. Chem., Int. Ed., 2022, 61, e202202387 CrossRef CAS PubMed;
(m) T. Yoshida, T. Kodama and M. Tobisu, Asian J. Org. Chem., 2022, 11, e202200610 CrossRef CAS;
(n) H. Fujimoto, S. Yamamura, M. Kusano and M. Tobisu, Org. Lett., 2023, 25, 336–340 CrossRef CAS PubMed;
(o) W. P. Hong, H. N. Lim and I. Shin, Org. Chem. Front., 2023, 10, 819–836 RSC;
(p) G. Zhou, Z. Z. Guo and X. Shen, Angew. Chem., Int. Ed., 2023, e202217189 CAS;
(q) T. Inagaki, T. Ando, S. Sakurai, M. Yamanaka and M. Tobisu, Chem. Sci., 2023, 14, 2706–2712 RSC;
(r) L. Zheng, X. Y. Guo, Y.-C. Li, Y. C. Wu, X.-S. Xue and P. Wang, Angew. Chem., Int. Ed., 2023, 62, e202216373 CAS.
-
(a) R. Unger, F. Weisser, N. Chinkov, A. Stanger, T. Cohen and I. Marek, Org. Lett., 2009, 11, 1853–1856 CrossRef CAS PubMed;
(b) F. Q. Li, S. Zhong, G. Lu and A. S. C. Chan, Adv. Synth. Catal., 2009, 351, 1955–1960 CrossRef CAS;
(c) P. Smirnov, J. Mathew, A. Nijs, E. Katan, M. Karni, C. Bolm, Y. Apeloig and I. Marek, Angew. Chem., Int. Ed., 2013, 52, 13717–13721 CrossRef CAS;
(d) P. Smirnov, E. Katan, J. Mathew, A. Kostenko, M. Karni, A. Nijs, C. Bolm, Y. Apeloig and I. Marek, J. Org. Chem., 2014, 79, 12122–12135 CrossRef CAS;
(e) J. Rong, R. Oost, A. Desmarchelier, A. J. Minnaard and S. R. Harutyunyan, Angew. Chem., Int. Ed., 2015, 54, 3038–3042 CrossRef CAS PubMed;
(f) M. Y. Han, X. Y. Xie, D. Zhou, P. H. Li and L. Wang, Org. Lett., 2017, 19, 2282–2285 CrossRef CAS;
(g) M. Y. Han, W. Y. Luan, P. L. Mai, P. H. Li and L. Wang, J. Org. Chem., 2018, 83, 1518–1524 CrossRef CAS PubMed;
(h) J.-J. Feng and M. Oestreich, Angew. Chem., Int. Ed., 2019, 58, 8211–8215 CrossRef CAS PubMed;
(i) M. M. Guan, S. Y. Wang, Y. Luo, W. D. Cao, X. H. Liu and X. M. Feng, Chem. Sci., 2021, 12, 7498–7503 RSC.
-
(a) K. Mikami and M. Shimizu, Chem. Rev., 1992, 92, 1021–1050 CrossRef CAS;
(b) J. S. Johnson and D. A. Evans, Acc. Chem. Res., 2000, 33, 325–335 CrossRef CAS PubMed;
(c) B. B. Snider, Acc. Chem. Res., 2002, 13, 426–432 CrossRef;
(d) M. L. Clarke and M. B. France, Tetrahedron, 2008, 64, 9003–9031 CrossRef CAS;
(e) X. H. Liu, K. Zheng and X. M. Feng, Synthesis, 2014, 46, 2241–2257 CrossRef CAS;
(f) P. Saha and A. K. Saikia, Org. Biomol. Chem., 2018, 16, 2820–2840 RSC;
(g) A. Bakhtiari and J. Safaei-Ghomi, Synlett, 2019, 30, 1738–1764 CrossRef CAS;
(h) M. Balha, C. Parida and S. Chandra Pan, Asian J. Org. Chem., 2021, 10, 2440–2453 CrossRef CAS.
- M. Y. Han, H. Pan, P. H. Li and L. Wang, J. Org. Chem., 2020, 85, 5825–5837 CrossRef CAS PubMed.
-
(a) A. Kirschning, S. Luiken, A. Migliorini, M. A. Loreto and M. Vogt, Synlett, 2009, 429–432 CrossRef CAS;
(b) Y. Matsuya, K. Wada, D. Minato and K. Sugimoto, Angew. Chem., Int. Ed., 2016, 55, 10079–10082 CrossRef CAS PubMed;
(c) M.-Y. Han, F.-Y. Yang, D. Zhou and Z. G. Xu, Org. Biomol. Chem., 2017, 15, 1418–1425 RSC;
(d) J. F. Collados, P. Ortiz, J. M. Pérez, Y. Y. Xia, M. A. J. Koenis, W. J. Buma, V. P. Nicu and S. R. Harutyunyan, Eur. J. Org. Chem., 2018, 3900–3903 CrossRef CAS;
(e) X. N. Lu, J. Zhang, L. Y. Xu, W. Z. Shen, F. F. Yu, L. Y. Ding and G. F. Zhong, Org. Lett., 2020, 22, 5610–5616 CrossRef CAS;
(f) M. Ratushnyy and A. V. Zhukhovitskiy, J. Am. Chem. Soc., 2021, 143, 17931–17936 CrossRef CAS PubMed;
(g) Y. Zhang, J.-J. Chen and H.-M. Huang, Angew. Chem., Int. Ed., 2022, 61, e202205671 CAS.
-
(a) D. L. Priebbenow, J. Org. Chem., 2019, 84, 11813–11822 CrossRef CAS PubMed;
(b) Q. B. Zhao, Q. H. Geng, Y. L. Li, J. W. Li and Z. X. Liu, Org. Chem. Front., 2023, 10, 1316–1321 RSC.
-
(a) A. V. Gulevich, A. S. Dudnik, N. Chernyak and V. Gevorgyan, Chem. Rev., 2013, 113, 3084–3213 CrossRef CAS PubMed;
(b) Z. Jin, Nat. Prod. Rep., 2013, 30, 869–915 RSC;
(c) H.-Z. Zhang, Z.-L. Zhao and C.-H. Zhou, Eur. J. Med. Chem., 2018, 144, 444–492 CrossRef CAS PubMed;
(d) I. V. Smolyar, A. K. Yudin and V. G. Nenajdenko, Chem. Rev., 2019, 119, 10032–10240 CrossRef CAS PubMed;
(e) N. Aljaar, R. Gujjarappa, M. Al-Refai, M. Shtaiwi and C. C. Malakar, J. Heterocycl. Chem., 2019, 56, 2730–2743 CrossRef CAS;
(f) A. Yoshimura, A. Saito, M. Yusubovc and V. Zhdankin, Synthesis, 2020, 52, 2299–2310 CrossRef CAS.
- For reviews and selected examples on gold-mediated cycloisomerization of N-propargylamides, see:
(a) Y. C. Hu, X. Y. Xin and B. S. Wan, Tetrahedron Lett., 2015, 56, 32–52 CrossRef CAS;
(b) K. Lauder, A. Toscani, N. Scalacci and D. Castagnolo, Chem. Rev., 2017, 117, 14091–14200 CrossRef CAS PubMed;
(c) T. Wang and A. S. K. Hashmi, Chem. Rev., 2021, 121, 8948–8978 CrossRef CAS PubMed;
(d) A. S. K. Hashmi, J. P. Weyrauch, W. Frey and J. W. Bats, Org. Lett., 2004, 6, 4391–4394 CrossRef CAS PubMed;
(e) M. D. Milton, Y. Inada, Y. Nishibayashi and S. Uemura, Chem. Commun., 2004, 2712–2713 RSC;
(f) A. S. K. Hashmi, A. M. Schuster and F. Rominger, Angew. Chem., Int. Ed., 2009, 48, 8247–8249 CrossRef CAS PubMed;
(g) J. P. Weyrauch, A. S. K. Hashmi, A. Schuster, T. Hengst, S. Schetter, A. Littmann, M. Rudolph, M. Hamzic, J. Visus, F. Rominger, W. Frey and J. W. Bats, Chem.–Eur. J., 2010, 16, 956–963 CrossRef CAS PubMed;
(h) O. A. Egorova, H. Seo, Y. Kim, D. Moon, Y. M. Rhee and K. H. Ahn, Angew. Chem., Int. Ed., 2011, 50, 11446–11450 CrossRef CAS PubMed;
(i) A. S. K. Hashmi, M. C. Blanco Jaimes, A. M. Schuster and F. Rominger, J. Org. Chem., 2012, 77, 6394–6408 CrossRef CAS PubMed;
(j) P. Querard, S. A. Girard, N. Uhlig and C.-J. Li, Chem. Sci., 2015, 6, 7332–7335 RSC;
(k) H. H. Peng, N. G. Akhmedov, Y.-F. Liang, N. Jiao and X. D. Shi, J. Am. Chem. Soc., 2015, 137, 8912–8915 CrossRef CAS PubMed;
(l) S. Doherty, J. G. Knight, D. O. Perry, N. A. B. Ward, D. M. Bittner, W. McFarlane, C. Wills and M. R. Probert, Organometallics, 2016, 35, 1265–1278 CrossRef CAS;
(m) V. H. L. Wong, S. V. C. Vummaleti, L. Cavallo, A. J. P. White, S. P. Nolan and K. K. Hii, Chem.–Eur. J., 2016, 22, 13320–13327 CrossRef CAS PubMed;
(n) M. Teci, D. Hueber, P. Pale, L. Toupet, A. Blanc, E. Brenner and D. Matt, Chem.–Eur. J., 2017, 23, 7809–7818 CrossRef CAS PubMed.
-
(a) A. S. K. Hashmi and A. Littmann, Chem.–Asian J., 2012, 7, 1435–1442 CrossRef CAS PubMed;
(b) J. Li, L. L. Lin, B. W. Hu, X. J. Lian, G. Wang, X. H. Liu and X. M. Feng, Angew. Chem., Int. Ed., 2016, 55, 6075–6078 CrossRef CAS PubMed;
(c) B. Wang, Y. Chen, L. Zhou, J. W. Wang and Z. H. Xu, Org. Biomol. Chem., 2016, 14, 826–829 RSC;
(d) H. L. Wei, M. Bao, K. Y. Dong, L. H. Qiu, B. Wu, W. H. Hu and X. F. Xu, Angew. Chem., Int. Ed., 2018, 57, 17200–17204 CrossRef CAS PubMed;
(e) K. S. Nalivela, M. Rudolph, E. S. Baeissa, B. G. Alhogbi, I. A. I. Mkhalid and A. S. K. Hashmi, Adv. Synth. Catal., 2018, 360, 2183–2190 CrossRef CAS;
(f) B. W. Hu, J. Li, W. D. Cao, Q. C. Lin, J. Yang, L. L. Lin, X. H. Liu and X. M. Feng, Adv. Synth. Catal., 2018, 360, 2831–2835 CrossRef CAS;
(g) G. Z. Dong, M. Bao, X. D. Xie, S. K. Jia, W. H. Hu and X. F. Xu, Angew. Chem., Int. Ed., 2021, 60, 1992–1999 CrossRef CAS;
(h) W. D. Cao and X. H. Liu, Chin. J. Org. Chem., 2021, 41, 857–858 CrossRef CAS.
- For selected reviews and examples on N,N'-dioxide ligands, see:
(a) X. H. Liu, L. L. Lin and X. M. Feng, Acc. Chem. Res., 2011, 44, 574–587 CrossRef CAS PubMed;
(b) X. H. Liu, L. L. Lin and X. M. Feng, Org. Chem. Front., 2014, 1, 298–302 RSC;
(c) X. H. Liu, H. F. Zheng, Y. Xia, L. L. Lin and X. M. Feng, Acc. Chem. Res., 2017, 50, 2621–2631 CrossRef CAS PubMed;
(d) X. H. Liu, S. X. Dong, L. L. Lin and X. M. Feng, Chin. J. Chem., 2018, 36, 791–797 CrossRef CAS;
(e) M. Y. Wang and W. Li, Chin. J. Chem., 2021, 39, 969–984 CrossRef CAS;
(f) S. X. Dong, X. H. Liu and X. M. Feng, Acc. Chem. Res., 2022, 55, 415–428 CrossRef CAS;
(g) Y. H. Wen, X. Huang and X. M. Feng, Synlett, 2005, 16, 2445–2448 Search PubMed;
(h) X. Zhang, D. H. Chen, X. H. Liu and X. M. Feng, J. Org. Chem., 2007, 72, 5227–5233 CrossRef CAS PubMed;
(i) K. Zheng, J. Shi, X. H. Liu and X. M. Feng, J. Am. Chem. Soc., 2008, 130, 15770–15771 CrossRef CAS PubMed;
(j) K. Zheng, C. K. Yin, X. H. Liu, L. L. Lin and X. M. Feng, Angew. Chem., Int. Ed., 2011, 50, 2573–2577 CrossRef CAS PubMed;
(k) W. Liu, W. D. Cao, H. P. Hu, L. L. Lin and X. M. Feng, Chem. Commun., 2018, 54, 8901–8904 RSC;
(l) S. L. Ge, W. D. Cao, T. F. Kang, B. W. Hu, H. Zhang, Z. S. Su, X. H. Liu and X. M. Feng, Angew. Chem., Int. Ed., 2019, 58, 4017–4021 CrossRef CAS PubMed;
(m) Q. W. He, D. Zhang, F. C. Zhang, X. H. Liu and X. M. Feng, Org. Lett., 2021, 23, 6961–6966 CrossRef CAS;
(n) L. Z. Hou, Y. Q. Zhou, H. Yu, T. Y. Zhan, W. D. Cao and X. M. Feng, J. Am. Chem. Soc., 2022, 144, 22140–22149 CrossRef CAS PubMed;
(o) X. X. Tang, Z. S. Su, Q. C. Lin, L. L. Lin, S. X. Dong and X. M. Feng, Chin. J. Chem., 2022, 40, 1793–1798 CrossRef CAS;
(p) T. Y. Zhan, L. K. Yang, Q. Y. Chen, R. Weng, X. H. Liu and X. M. Feng, CCS Chem., 2022, 4 DOI:10.31635/ccschem.022.202202405;
(q) Y. H. Mo, Q. Y. Chen, J. Z. Li, D. Ye, Y. Q. Zhou, S. X. Dong, X. H. Liu and X. M. Feng, ACS Catal., 2023, 13, 877–886 CrossRef CAS;
(r) D. Zhang, M. P. Pu, Z. Z. Liu, Y. Q. Zhou, Z. D. Yang, X. H. Liu, Y.-D. Wu and X. M. Feng, J. Am. Chem. Soc., 2023, 145, 4808–4818 CrossRef CAS;
(s) Z. K. Wu, X. Y. Zhang, N. Xu, X. H. Liu and X. M. Feng, ACS Catal., 2023, 13, 815–823 CrossRef CAS;
(t) X. Y. Hu, L. Chen, H. Y. Li, Q. F. Xu, X. H. Liu and X. M. Feng, ACS Catal., 2023, 13, 6675–6682 CrossRef CAS;
(u) J. X. Xu, Y. J. Song, J. Yang, B. Q. Yang, Z. S. Su, L. L. Lin and X. M. Feng, Angew. Chem., Int. Ed., 2023, e202217887 CAS.
- Z. C. Lu, T. T. Li, S. R. Mudshinge, B. Xu and G. B. Hammond, Chem. Rev., 2021, 121, 8452–8477 CrossRef CAS.
- CCDC 2207796 (C20) contains the supplementary crystallographic data for this paper.† These data can be obtained free of charge from The Cambridge Crystallographic Data Centre viahttp://www.ccdc.cam.ac.uk/data_request/cif.
- CCDC 1977471.
Footnote |
† Electronic supplementary information (ESI) available: 1H, 13C{1H} and 19F{1H} NMR, HPLC spectra. X-ray crystallographic data for C20 (CIF). CCDC 2207796. For ESI and crystallographic data in CIF or other electronic format see DOI: https://doi.org/10.1039/d3sc01048a |
|
This journal is © The Royal Society of Chemistry 2023 |
Click here to see how this site uses Cookies. View our privacy policy here.