DOI:
10.1039/D3SC00986F
(Edge Article)
Chem. Sci., 2023,
14, 5431-5437
Nitrogen-interrupted halo-Prins/halo-Nazarov fragment coupling cascade for the synthesis of indolines†
Received
22nd February 2023
, Accepted 20th April 2023
First published on 24th April 2023
Abstract
The nitrogen-interrupted Nazarov cyclization can be a powerful method for the stereocontrolled synthesis of sp3-rich N-heterocycles. However, due to the incompatibility between the basicity of nitrogen and the acidic reaction conditions, examples of this type of Nazarov cyclization are scarce. Herein, we report a one-pot nitrogen-interrupted halo-Prins/halo-Nazarov coupling cascade that joins two simple building blocks, an enyne and a carbonyl partner, to furnish functionalized cyclopenta[b]indolines with up to four contiguous stereocenters. For the first time, we provide a general method for the alkynyl halo-Prins reaction of ketones, thus enabling the formation of quaternary stereocenters. Additionally, we describe the outcomes of secondary alcohol enyne couplings, which exhibit helical chirality transfer. Furthermore, we investigate the impact of aniline enyne substituents on the reaction and evaluate the tolerance of different functional groups. Finally, we discuss the reaction mechanism and demonstrate various transformations of the prepared indoline scaffolds, highlighting their applicability in drug discovery campaigns.
Introduction
Nitrogen heterocycles are highly valuable motifs in pharmaceutical chemistry, found in more than 75% of U.S. FDA-approved drugs.1 Among them, simple indoles and pyrrolidines are two of the ten most frequently observed nitrogen-containing heterocyclic scaffolds.2 Their more complex relatives, cyclopentannulated indoles and indolines, are part of Nature's large bioactive indole alkaloid family.3 The cyclopenta[b]indoline scaffold is particularly attractive for drug discovery campaigns due to its molecular three-dimensionality, which has been associated with the reduction of off-target effects leading to toxicity.4
Interrupted Nazarov cyclization strategies are powerful methods for generating cyclopentanoid structures, providing rapid access to highly functionalized, stereochemically rich, three-dimensional scaffolds.5 Stereospecific electrocyclization produces a cyclopentenyl cation that can be diastereoselectively trapped by various carbon and oxygen nucleophiles.5 However, very few examples of nitrogen atom-interrupted Nazarov cyclization have been reported (Scheme 1A),6 and only once been leveraged to build cyclopenta[b]indoles (Scheme 1B).6g In the context of our recent development of cascade cyclization sequences (halo-Prins coupling/interrupted halo-Nazarov cyclization),7 we sought to design a system that could engage nitrogen in the interruption step. Herein, we describe an expedient strategy for the assembly of cyclopentannulated indolines from simple precursors 1 and 2, featuring intramolecular capture of the intermediate halo-cyclopentenyl cation with an aniline moiety (Scheme 1C).
 |
| Scheme 1 Interrupted Nazarov cyclizations: application to the synthesis of N-heterocycles. | |
Nitrogen substitution
The investigation of the nitrogen-interrupted halo-Prins/halo-Nazarov cascade commenced with the reaction of the N-tosyl aniline enyne 1a and benzaldehyde (2a). When these building blocks are exposed to triflic acid (TfOH) and tetrabutylammonium iodide (TBAI) in dichloromethane (DCM),7 the halo-Prins coupling occurs smoothly. Subsequent addition of HFIP initiates the halo-Nazarov cascade and delivers the desired indoline 3a in high yield (88%; Table 1, entry 1). This one-pot process extends well to the N-mesyl analog 1b, producing the corresponding 3b in 86% yield (entry 2). Unfortunately, it is not possible to remove these N-sulfonyl groups cleanly (see ESI† for details). In search of a more labile aniline protecting group, we tested carbamate analog 1c, which cleanly transforms into 3c in 84% yield (entry 3). However, the carbamate resists undergoing clean deprotection when one atmosphere of hydrogen is used under typical hydrogenolysis conditions. 2-(Trimethylsilyl)ethanesulfonyl group (SES) is designed for simple removal using fluoride reagents.8 Indeed, when the coupling cascade is carried out using enyne 1d, capture with aniline is realized, delivering the desired indoline 4a after TBAF-mediated deprotection (50% yield overall, entry 4). Even better, the use of N-nosyl (2-nitrobenzenesulfonyl) enyne 1e allows execution of three reactions in one-pot (halo-Prins coupling, halo-Nazarov cyclization, deprotection9), affording the N-unsubstituted indoline 4a in 81% yield (entry 5).
Table 1 Scope (enyne 1 aniline N-substitution)a

|
Entry |
Aniline 1 |
Indoline 3 (yieldb) |
Indoline 4a (yieldc) |
|
One-pot reaction conditions: 1 (0.2 mmol), 2a (1.2 equiv.), TBAI (1.2 equiv.), TfOH (1.25 equiv.), DCM, −40 °C to rt; then HFIP, 0 °C to rt; then deprotection (see ESI for details).
Isolated yield across two steps.
Isolated yield across three steps.
Removal of these N-groups was unsuccessful (see ESI for details).
One-pot halo-Prins/halo-Nazarov/deprotection.
|
1 |
1a (R = Ts) |
3a (88%) |
—d |
|
2 |
1b (R = Ms) |
3b (86%) |
—d |
3 |
1c (R = Cbz) |
3c (84%) |
—d |
4 |
1d (R = SES) |
3d (71%) |
50% |
5
|
1e
(R = Ns)
|
3e
(74%)
|
81%
|
The unprotected enyne 1f cannot be converted into the desired indoline 4a using the optimized conditions (Scheme 2). Instead, the halo-Prins product 3′f is obtained, as an undesired imine adduct. No reaction is observed upon the subjection of 3′f to the halo-Nazarov reaction conditions (in one-pot or in sequence).
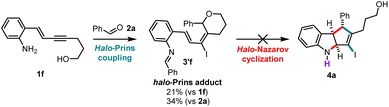 |
| Scheme 2 The behavior of the unprotected enyne 1f. | |
Aldehyde scope
Once we identified the optimal enyne 1e, our goal was to demonstrate efficient synthesis of indoline scaffolds with structural features of value (Scheme 3).10 Thus, we focused primarily on aldehydes containing halogens (that enable further cross-coupling strategies for functionalization), medicinally relevant fragments (heterocycles), and different alkyl groups (that increase three-dimensionality). Indolines 4b–4d, bearing arenes with functional handles, form smoothly in 66–76% yield. Heteroaromatic aldehydes containing a thiophene (2e) or a benzofuran (2f) core also afford the indolines 4e and 4f in good yields (73% and 56% yield, respectively). Unfortunately, the reaction of 1e with pyridine-4-carbaldehyde is not successful, using either 1.2 or 2.4 equiv. of TfOH. Neither the desired product 4g nor any of the expected intermediates were observed.
 |
| Scheme 3 Exploration of scope (aldehyde reactants 2).a aYields correspond to isolated yields of pure products across the three-step sequence (one-pot unless otherwise stated). bNo product was observed. cDimethoxymethane and 1,3,5-trioxane were used as formaldehyde surrogates. d1,1-Dimethoxyethane was used as an acetaldehyde surrogate. eThe sequence was not done in one-pot (see ESI† for details). | |
Next, we examined aliphatic aldehydes. Due to difficulties in handling neat formaldehyde and acetaldehyde, acetals were used as surrogates. Unfortunately, formaldehyde, in the form of dimethoxymethane or 1,3,5-trioxane,11a is not a suitable carbonyl partner, and cannot be incorporated into the scaffolds. On the other hand, 1,1-dimethoxyethane11b as the acetaldehyde surrogate reacts well, producing 4i in a moderate 31% yield. We were pleased to observe that isobutyraldehyde performs just as well as aromatic aldehydes, successfully furnishing 4j (69%). It was satisfying to observe that (E)-4-hexenal undergoes efficient reaction to yield 4k (62%). There is no evidence of degradation of the alkene functionality under the reaction conditions. Surprisingly, much bulkier cyclohexanecarbaldehyde performs better than the smaller aliphatic aldehydes, smoothly affording 4l in 78% yield. Heteroatom-containing cyclic aldehydes do not react as well as other aldehydes; they convert to the desired products 4m and 4n in diminished yields (29% and 15% respectively).
Ketone optimization and scope
The engagement of ketones in the alkynyl Prins coupling is challenging, with limited reports in the literature.12 Subjection of the ketone 5a to the one-pot halo-Prins/halo-Nazarov conditions used for aldehydes leads to the formation of a complex mixture that is difficult to purify. However, a telescoped, two-step sequence greatly simplifies the subsequent isolation of the halo-Nazarov product 6a. Using this method, we investigated the reactivity of ketone 5a, along with its dimethyl ketal and methyl enol ether analogs (Table 2). The reactivity of the ketone and ketal is almost identical, and in each case, a higher yield of 6a is observed with a larger excess of the carbonyl partner (entries 1–4). Methyl enol ether reacts faster, the reactions are cleaner, and the yield of 6a is strikingly higher (entry 6) compared to the reactions in which the ketone or ketal are used. Furthermore, when reacted with secondary alcohol enynes, methyl enol ethers are the only ketone equivalents that produce halo-Prins and halo-Nazarov products in significant yields.13 The use of methyl enol ethers is a broadly effective, general method for engaging ketones in the alkynyl halo-Prins coupling/cyclization.
Table 2 Optimization of the halo-Prins/halo-Nazarov coupling cascade for the formation of ketone-derived indolinesa
With the optimized conditions in hand, we briefly explored the scope of the ketone-derived cyclopenta[b]indolines (Scheme 4). The enol ether of an unsymmetrical cyclic ketone (2-methylcyclohexanone, 5b) reacts well, producing an inseparable mixture of diastereomers (major diastereomer is tentatively assigned as 6b). Enol ethers of larger-sized cyclic ketones can also be engaged, as shown by the facile formation of 6c. Enol ethers of acyclic ketones do not behave as well as the cyclic ones and lower yields of the desired products are observed. Both acetone and acetophenone methyl enol ethers give the desired indoline scaffolds 6d and 6e, respectively; the latter as an inseparable mixture of diastereomers. Interestingly, the major diastereomer of 6e is the result of a conrotatory cyclization of a pentadienyl cation in which the phenyl group is located “in” and the methyl group “out”.14
 |
| Scheme 4 Exploration of scope (ketones masked as methyl enol ethers 5).a aYields correspond to isolated yields of pure products across two steps. Diastereomeric ratios (dr) were determined from 1H NMR. bStereochemistry of the major diastereomer is tentatively assigned. cX-Ray structure of the major diastereomer of 6e (recrystallized from the mixture).15 | |
Aniline substitution
To explore the effect of the aniline enyne substitution on the halo-Prins/halo-Nazarov reactivity, enynes 1g–1i were prepared and subjected to the one-pot reaction conditions (Scheme 5). The methoxy-substituted enyne 1g requires careful control of the reaction conditions, as it reacts erratically to give 7a in a diminished 40% yield.16 Conversely, the trifluoromethyl-substituted enyne 1h and the chloro-substituted enyne 1i produce the desired 7b and 7c smoothly in 82% yield. Chloroindoline 7c is of particular value as it introduces a new functional handle for further transformations.
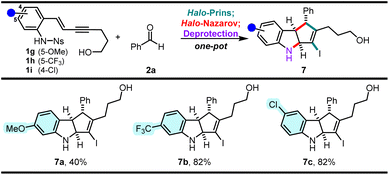 |
| Scheme 5 Effect of the aniline (ring) substitution on the halo-Prins/halo-Nazarov reaction cascade.a aYields correspond to the isolated yields of pure products across three steps in one-pot. | |
Secondary alcohols
It was previously discovered that the halo-Prins/halo-Nazarov coupling cascades proceed via a conrotatory cyclization of helically chiral pentadienyl cations.7c Furthermore, helical preorganization of the pentadienyl cation, dictated by the stereochemical elements present in the enyne, can transfer the chirality to the halo-Nazarov product, leading to partial or complete diastereoselectivity in the cationic cascade.17
To investigate this phenomenon in our system, we prepared the enyne secondary18 alcohol 1j and coupled it with three stereochemically and electronically different carbonyl partners (Scheme 6). Benzaldehyde 2a reacts smoothly and affords the desired product 8a in 81% yield, in a 4.0
:
1 dr. On the other hand, isobutyraldehyde 2j reacts more sluggishly than 2a, giving 8b in 53% yield, with a similar 4.1
:
1 dr. Lastly, cyclohexanone methyl enol ether 5a couples with 1j, albeit with a slower rate compared to the coupling with 1e, and affords 8c in 64% yield, in a 2.7
:
1 dr. This is the first example of the chirality transfer observed in the formation of ketone-derived halo-Nazarov products.
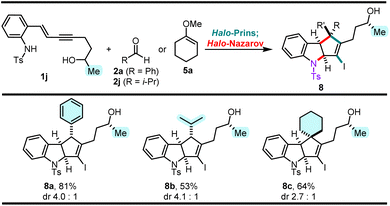 |
| Scheme 6 Effect of a secondary (alcohol) enyne on the diastereoselectivity of the halo-Prins/halo-Nazarov coupling cascade.a aYields correspond to isolated yields of pure products across two steps. Diastereomeric ratios were determined by normal-phase HPLC separation. Structures of major diastereomers are tentatively assigned according to ref. 7c. | |
Functional group compatibility
A functional group robustness screen, developed by Glorius et al., is a convenient method for the rapid assessment of the compatibility of functional groups with the reactions of interest.19 In this method, the reaction is performed in the presence of one equivalent of an additive containing the functional group of interest, and the outcome of the reaction is analyzed using an appropriate analytical method. This approach effectively “decouples” the functional group from the alteration of steric and electronic properties of the substrates, which can often lead to erroneous conclusions.
Using this method, we analyzed the effect of six common functional groups on the halo-Prins/halo-Nazarov coupling cascade that forms the indoline 4Nb (Table 3). The effect of additives on the reaction was monitored using TLC analysis, and the yield of 4Nb was quantified using quantitative 1H NMR (qNMR). In the absence of any additives, the reaction proceeds smoothly and forms the desired product in 91% yield (entry 1). The reaction is unaffected by methyl phenylacetate A1, and the desired product is obtained in 90% yield (entry 2). No evidence of transesterification is observed in the qNMR. The presence of dibenzyl ether A2 and benzyl cyanide A3 has a slight deleterious effect on the reaction, and the indoline is obtained in 81–83% yield (entries 2 and 3). On the other hand, phenylacetylene A4 undergoes partial conversion leading to the unselective formation of a mixture of products (entry 5). However, the formation of the desired indoline 4Nb is still observed, albeit with a diminished 58% yield. A comparable result is obtained with the t-butyldiphenylsilyl ether of 4-pentyn-1-ol (A5), leading to the formation of the product in 54% yield (entry 6). In this case, however, desilylation is also observed.
Table 3 Effect of additives containing various functional groups on the halo-Prins/halo-Nazarov cascade
Product transformations
Lastly, we explored various transformations that further diversify prepared scaffolds, using 4b/4Nb and 4k/4Nk as model substrates (Scheme 7).20N-Alkylation of the indoline 4b can be achieved using reductive amination conditions, giving N-methyl indoline 9a in 42% yield. Efforts to achieve alcohol oxidation using in situ prepared RuO4 produce aldehyde 9b from N-nosyl 4Nb in 29% yield, whereas insoluble material is obtained from unprotected indoline 4b. Suzuki–Miyaura cross-coupling showed complete chemoselectivity for the vinylic iodide over aryl chloride, effortlessly furnishing 9c and 9d in >90% yields. The pendant olefin of 4k readily engages in olefin cross metathesis, affording the terpene-like product 9e in 77% yield. In contrast, ozonolysis of unprotected indoline 4k produced insoluble material. However, this is not the case with the N-nosyl 4Nk, which gives the diol 9f readily after a reductive workup. The nosyl group was cleanly removed to give 9g, and the reduced yield can be attributed to the challenges associated with the isolation of the significantly more polar dihydroxy indoline. Finally, a lithium–halogen exchange procedure leads to smooth deiodination to form the vinylic iodide 9h.
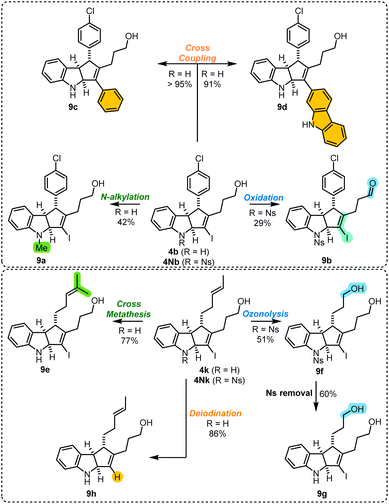 |
| Scheme 7 Transformations of the cyclopenta[b]indoline scaffolds.a aReaction conditions: N-alkylation (9a): 4b, HCHO (aq.), AcOH, NaCNBH3, rt. Oxidation (9b): 4Nb, RuCl3 hydrate, NaIO4, MeCN/H2O, 0 °C to rt. Cross coupling (9c): 4b, PhB(OH)2, Pd(PPh3)4, Na2CO3 (aq.), toluene, EtOH, 80 °C. Cross coupling (9d): 4b, (9H-carbazol-2-yl)boronic acid pinacol ester, Pd(PPh3)4, K2CO3, 1,4-dioxane, water, 100 °C. Cross metathesis (9e): 4k, 2-methyl-2-butene, HG-II catalyst, toluene, 60 °C. Ozonolysis (9f): 4Nk, pyridine, DCM, O3/O2 gas mixture, −78 °C; then MeOH, NaBH4, 0 °C. Ns removal (9g): 9f, HSCH2CO2H, K2CO3, MeOH, 0 °C to rt. Deiodination (9h): 4k, THF, t-BuLi, −78 to −40 °C. Yields refer to isolated yields of pure products. | |
Mechanism
The proposed mechanism for the halo-Prins/halo-Nazarov coupling cascade is depicted in Scheme 8.21 Upon Brønsted acid-mediated condensation of aniline-containing enyne 1 with the aldehyde 2 or enol ether 5, oxycarbenium intermediate 10 is formed. This intermediate undergoes cyclization to afford the halo-Prins product 11. This product may be isolated or the cascade can be continued by the addition of HFIP. This results in the ionization of the labile C–O bond and formation of the 3-halo pentadienyl cation 12, which transforms via a 4π conrotatory electrocyclization (stage 1) into the halo-cyclopentenyl cation 13. Finally, this cation is captured by the nitrogen atom (stage 2) to afford the halo-Nazarov product 14. In case when 1 is a secondary alcohol, its stereogenic center controls the helical conformation of the non-planar U-shaped pentadienyl cation 12. The observed diastereoselectivity of the products is a result of the competing rates of electrocyclization and helical epimerization.
 |
| Scheme 8 Proposed mechanism for the halo-Prins/halo-Nazarov coupling cascade. | |
DFT calculations were performed to gain further insight into the energetics of the halo-Nazarov cyclization and the results are depicted in Fig. 1. The N-mesyl analog of 4i was chosen for this case study to minimize the computational time.
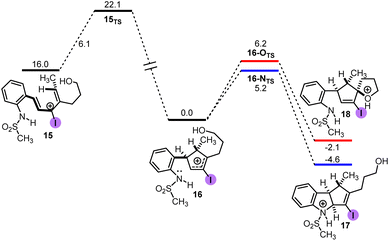 |
| Fig. 1 Computational study (M06-2x/Def2-TZVP, SMD = DCM, free energies in kcal mol−1) of the nitrogen-interrupted halo-Nazarov cyclization. | |
The results reveal several key observations: the 4π electrocyclization is rapid
and irreversible
thus it is the diastereo determining step; the barriers for the heteroatom trappings (either nitrogen or oxygen) are low in both directions, implying that these processes are fast and reversible; the nitrogen trapping is kinetically and thermodynamically preferred over the oxygen trapping (ΔΔG‡ = 1.0 and ΔΔG = 2.5, both in favor of the nitrogen trapping).
Conclusions
In conclusion, we have developed an efficient method for the synthesis of stereochemically complex cyclopenta[b]indolines from two simple precursors. Using a nitrogen-interrupted halo-Prins/halo-Nazarov coupling cascade strategy, four new bonds and two new rings, along with up to four contiguous stereogenic centers are forged. Synthesized products contain up to four distinct functionalities, including an aniline, alcohol, and vinyl iodide, which were demonstrated to perform well under a variety of transformations. The methodology possesses broad functional group tolerance, although some reactive and Lewis basic groups give products in diminished yields. Additionally, computational studies were used to provide further insight into the energetics of the halo-Nazarov cyclization and to explain the selectivity in the intramolecular capture of the halo-cyclopentenyl cation with the nitrogen atom.
Data availability
All data associated with this publication are provided in the ESI.†
Author contributions
AM synthesized and characterized most of the compounds, carried out qNMR and HPLC studies, completed all DFT calculations and contributed to manuscript writing. CH synthesized and characterized the rest of the compounds. AJF conceived and supervised the project and contributed to manuscript writing.
Conflicts of interest
There are no conflicts to declare.
Acknowledgements
We thank the NSF (CHE-1900050 and CHE-2154854) for funding this study. We thank Dr W. W. Brennessel (Dept. of Chemistry, University of Rochester) for running X-ray crystallography analyses and the NSF (CHE-1725028) for funding our X-ray diffractometer. We also thank Kevin Welle, the University of Rochester Mass Spectrometry Resource Laboratory, and are thankful for the NIH instrument grant (S10OD021486). We also thank Jackson J. Hernandez, Patrycia K. Zybura, and Dasha Rodina (Dept. of Chemistry, University of Rochester) for helpful discussions.
Notes and references
- N. Kerru, L. Gummidi, S. Maddila, K. K. Gangu and S. B. Jonnalagadda, Molecules, 2020, 25, 1909 CrossRef CAS PubMed.
- E. Vitaku, D. T. Smith and J. T. Njardarson, J. Med. Chem., 2014, 57, 10257 CrossRef CAS PubMed.
- T. Vivekanand, B. Satpathi, S. K. Bankar and S. S. V. S. V. Ramasastry, RSC Adv., 2018, 8, 18576 RSC and references therein.
- F. Lovering, MedChemComm, 2013, 4, 515 RSC.
- Two extensive reviews on the interrupted Nazarov cyclizations have been published
(a) A. V. Yadykov and V. Z. Shirinian, Adv. Synth. Catal., 2020, 362, 702 CrossRef CAS;
(b) T. N. Grant, C. J. Rieder and F. G. West, Chem. Commun., 2009, 5676 RSC . Additionally, an account describing advances in this field by our group has been published, see;
(c) A. J. Frontier and J. J. Hernandez, Acc. Chem. Res., 2020, 53, 1822 CrossRef CAS PubMed.
- To the extent of our knowledge, this is the list of all nitrogen-interrupted Nazarov cyclizations reported so far
(a) F. Dhoro and M. A. Tius, J. Am. Chem. Soc., 2005, 127, 12472 CrossRef CAS PubMed;
(b) A. Rostami, Y. Wang, A. M. Arif, R. McDonald and F. G. West, Org. Lett., 2007, 9, 703 CrossRef CAS PubMed;
(c) F. Dhoro, T. E. Kristensen, V. Stockmann, G. P. A. Yap and M. A. Tius, J. Am. Chem. Soc., 2007, 129, 7256 CrossRef CAS PubMed;
(d) D. Song, A. Rostami and F. G. West, J. Am. Chem. Soc., 2007, 129, 12019 CrossRef CAS PubMed;
(e) O. Scadeng, M. J. Ferguson and F. G. West, Org. Lett., 2011, 13, 114 CrossRef CAS PubMed;
(f) R. William, W. L. Leng, S. Wang and X.-W. Liu, Chem. Sci., 2016, 7, 1100 RSC;
(g) Z. Wang, X. Xu, Z. Gu, W. Feng, H. Qian, Z. Li, X. Sun and O. Kwon, Chem. Commun., 2016, 52, 2811 RSC.
-
(a) G. Alachouzos and A. J. Frontier, Angew. Chem., Int. Ed., 2017, 56, 15030 CrossRef CAS PubMed;
(b) G. Alachouzos and A. J. Frontier, J. Am. Chem. Soc., 2019, 141, 118 CrossRef CAS PubMed;
(c) G. Alachouzos, C. Holt and A. J. Frontier, Org. Lett., 2020, 22, 4010 CrossRef CAS PubMed.
- P. Ribière, V. Declerck, J. Martinez and F. Lamaty, Chem. Rev., 2006, 106, 2249 CrossRef PubMed.
- S. P. Miller, Y.-L. Zhong, Z. Liu, M. Simeone, N. Yasuda, J. Limanto, Z. Chen, J. Lynch and V. Capodanno, Org. Lett., 2014, 16, 174 CrossRef CAS PubMed.
- Reactivity of aldehydes with different electronics in the halo-Prins/halo-Nazarov reactions has already been evaluated, e.g. compounds 4j–4l in C. Holt, G. Alachouzos and A. J. Frontier, J. Am. Chem. Soc., 2019, 141, 5461 CrossRef CAS PubMed.
-
(a)
A. B. Concepcion, H. Yamamoto, R. K. Boeckman Jr, K. F. Biegasiewicz and D. J. Tusch, in Encycl. Reag. Org. Synth., John Wiley & Sons, Ltd, 2018 Search PubMed;
(b)
M. J. Taschner, in Encycl. Reag. Org. Synth., John Wiley & Sons, Ltd, 2001 Search PubMed.
- S. Abdul-Rashed, C. Holt and A. J. Frontier, Synthesis, 2020, 52, 1991 CrossRef CAS and references therein.
- In an independent project, we have discovered that methyl enol ethers of 4-aryl-2-butanones (4-aryl-2-methoxy-1-butenes) are the only ketone equivalents that undergo the alkynyl halo-Prins coupling with secondary (enyne) alcohols in an efficient manner (≥60% yields). Ketones
were found to be unreactive and dimethyl ketals gave the desired halo-Prins adducts in low yields (≈30% yields).
- The terminology is defined in ref. 7b (Scheme 6). This outcome is unusual, as the corresponding A-values for the phenyl and methyl group (3.0 vs. 1.7, respectively) imply that the bulkier group (phenyl) would prefer to be positioned “out“, which would produce the opposite epimer (epi-6e) as the major diastereomer.
- While an X-ray structure of a single diastereomer from a mixture cannot be used as a rationale for the structure determination of the major diastereomer, 2D-NOESY can, and this data is presented in the ESI (see pages S110–S111†).
- If the temperature of the cold bath used for the reaction is not well maintained (i.e. if the halo-Prins reaction is let to warm up above 0 °C), a complex mixture of side products forms, further diminishing the yield of the desired 7a. This is not the case with other enynes, which are well-behaved and do not require precise control of the reaction conditions.
- Additionally, as shown in ref. 7c, this strategy can be used for the synthesis of enantiopure products (without a need for chiral separation). Starting with an enantiopure secondary alcohol enyne, the obtained halo-Nazarov product mixture can be separated and the stereocenter originating from the secondary alcohol can be removed (via oxidative or reductive methods). Such obtained products would be enantiomers, with one enantiomer in excess compared to the other.
- Tertiary alcohols were deemed to be outside of the scope of the manuscript, as the ionization of the halo-Prins intermediate is expected to produce side-products originating from the competitive ionization that forms a tertiary carbocation.
- K. D. Collins and F. Glorius, Nat. Chem., 2013, 5, 597 CrossRef CAS PubMed.
- Procedures for the transformations were obtained from the following publications:
(a) D. Ma, G. Tang and A. P. Kozikowski, Org. Lett., 2002, 4, 2377 CrossRef CAS PubMed . (Eschweiler–Clarke reaction);
(b) L. Shi, K. Meyer and M. F. Greaney, Angew. Chem., Int. Ed., 2010, 49, 9250 CrossRef CAS PubMed . (Oxidation);
(c) K. F. Johnson, E. A. Schneider, B. P. Schumacher, A. Ellern, J. D. Scanlon and L. M. Stanley, Chem.–Eur. J., 2016, 22, 15619 CrossRef CAS PubMed . (Suzuki–Miyaura reaction for the preparation of 8c);
(d) N. Mace, A. R. Thornton and S. B. Blakey, Angew. Chem., Int. Ed., 2013, 52, 5836 CrossRef CAS . (Suzuki–Miyaura reaction for the preparation of 8d);
(e) A. Sytniczuk, A. Kajetanowicz and K. Grela, Catal. Sci. Technol., 2017, 7, 1284 RSC . (Cross metathesis with 2-methyl-2-butene);
(f) R. Willand-Charnley, T. J. Fisher, B. M. Johnson and P. H. Dussault, Org. Lett., 2012, 14, 2242 CrossRef CAS PubMed . (Ozonolysis);
(g) S. Xu, K. Hirano and M. Miura, Org. Lett., 2020, 22, 9059 CrossRef CAS PubMed . (Halogen–lithium exchange).
- This mechanism is analogous to the previously reported mechanisms for the halo-Prins/halo-Nazarov cascades, see ref. 7.
|
This journal is © The Royal Society of Chemistry 2023 |
Click here to see how this site uses Cookies. View our privacy policy here.