DOI:
10.1039/D3RA05246J
(Paper)
RSC Adv., 2023,
13, 26948-26959
Selectfluor-mediated tandem cyclization of enaminones with diselenides toward the synthesis of 3-selenylated chromones†
Received
3rd August 2023
, Accepted 4th September 2023
First published on 8th September 2023
Abstract
A practical and metal-free approach for the regioselective selenation of chromones employing Selectfluor reagent under mild conditions is described. The developed method is suitable for a wide substrate scope and affords 3-selenylated chromones in good to excellent yield with high selectivity. An ionic mechanism is proposed for this transformation. Furthermore, the application of potassium thiocyanate with enaminones for the synthesis of thiocyano chromones in this transformation is also successful.
Introduction
A chromone moiety is the central structure in numerous natural products such as flavonoids, isoflavonoids, as well as other functionalized chromone molecules.1 As privileged heterocyclic scaffolds, chromone and its derivatives, including both naturally occurring and laboratory synthesized ones, have been proved with high application potential in drug discovery.2 Moreover, chromones have also exhibited attractive application in organic synthesis as well as the designation of molecules with useful optical functions.3 In particular, C3-substituted chromones have recently been drawing considerable attention as they exhibit a variety of physiological and biological activities, including anti-inflammatory,4 anti-dyslipidemic,5 antioxidant,6 antimicrobial,7 antitumor,8 anticancer,9 etc. Thus, considerable attention has been devoted to developing novel and efficient protocols for the synthesis of C3-substituted chromone derivatives, and several significant achievements have been reported for the synthesis of 3-substituted chromone derivatives.10
Organoselenium compounds have attracted considerable attention in medicinal chemistry owing to their well-known biological activities, which are mainly attributed to the fact that selenium atoms may serve as an electron donor or a hydrogen bond acceptor in these applications, altering the chemical characteristics of enzyme active sites.11 Moreover, they have gained considerable interest due to their well-known fluorescent properties,12 and wide applications in food chemistry and material science.13 In particular, recent studies revealed that chromones containing selenyl-substituents show unique bioactivities and chemical properties, and are widely adopted in drug design and regulation of biological processes.14 Therefore, many efforts have been devoted to the synthesis of more valuable 3-selenochromone derivatives.15 As the straightforward approach, the direct functionalization on naturally available or prior prepared chromone compounds offers access to 3-substituted chromone derivatives. Through this strategy, a seleno group could be introduced into the chromone framework.16 However, the limited natural sources or tedious preparation of chromone substrates have led to a high demand for alternative synthetic methods using easy and abundant industrial chemicals.
Among the readily available main building blocks, 2-hydroxyphenyl enaminones have been identified as particularly excellent candidates in the synthesis of functionalized chromones by means of tandem alkenyl C–H elaboration and chromone annulation.17 In the past decade, the enaminone-based chromone synthesis has gained splendid success by offering practical accesses to chromones containing different substituents.18 As 3-selenochromones are an important class of compounds, much efforts has been devoted to the assembly of this compound based on the featured chromone annulation of easily available 2-hydroxyphenyl enaminones. In 2016, Blond's group reported AgOTf-catalyzed synthesis of 3-selenochromones through the reaction of 2-hydroxyphenyl enaminones and pre-synthesized electrophilic selenium species (PhSeCl) (Scheme 1a).19 In 2017, Braga's group and Wan's group developed KIO3-mediated synthesis of 3-selenochromones through the reaction of o-hydroxyphenyl enaminones and diaryl diselenides, employing green solvents such as glycerol and ethyl lactate, respectively (Scheme 1b).20 In recent years, photoredox catalysis enabled by visible light has emerged as a fascinating and powerful synthetic protocol to promote a wide range of synthetically useful organic transformations.21 In 2021, a visible-light-promoted synthesis of 3-selenochromones was realized via the selenylation/cyclization of 2-hydroxyphenyl enaminones with diaryl diselenides in the presence of HOAc (Scheme 1c).22 Very recently, Braga's group developed trichloroisocyanuric acid (TCCA)-mediated synthesis of 3-selenochromones from 2-hydroxyphenyl enaminones with diaryl diselenides (Scheme 1d).23 Regardless of their merits, the current strategies suffer from some disadvantages such as transition-metal as a catalyst, limited substrate scope, the inevitability of a strong oxidant, high reaction temperature, or complex reaction conditions. Therefore, more general and mild approaches for the construction of diversely 3-selenochromones are yet highly desirable to satisfy the requirement of discovering more chromone-based functional molecules or lead compounds.
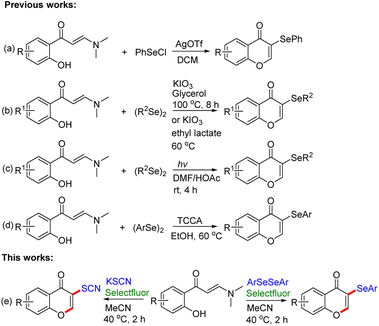 |
| Scheme 1 Different methods to 3-selenochromones from 2-hydroxyphenyl enaminones. (a) AgOTf-catalyzed cyclization; (b) KIO3-mediated cyclization; (c) visible-light-promoted cyclization; (d) TCCA-mediated cyclization; (e) selectfluor-mediated cyclization. | |
Selectfluor is commercially available, exceptionally stable, and useful for a mild oxidant.24 Several functionalized heterocyclic compounds have been synthesized successfully employing Selectfluor as an oxidant.25 Recently, some functionalized heterocycles have been constructed by our group using Selectfluor reagent as an electrophilic reagent or oxidant.26 Expanding the application of Selectfluor for the cross-coupling reaction is still challenging works. To the best of our knowledge, using stable and readily available diaryl diselenides as selenylating reagent, transition-metal free Selectfluor-promoted selenylation/cyclization of 2-hydroxyphenyl enaminones to access 3-arylselenenyl chromones remains yet elusive. As a part of our continuous interest in forming C–Se bond promoted by Selectfluor reagent, herein, we reported a practical and metal-free approach for the regioselective selenation of chromones employing Selectfluor reagent under mild conditions. Furthermore, the application of potassium thiocyanate with enaminones for the synthesis of 3-thiocyano chromones in this transformation is also successful (Scheme 1e). This protocol features a wide substrate scope, good to excellent yields, high selectivity, without the need for toxic metals, ligands, and bases, and could serve as an efficient approach for the construct 3-arylseleno/3-thiocyano chromones under mild conditions.
Results and discussion
Initially, the reaction of enaminone 1a and diphenyl diselenide 2a was screened as a model reaction to identify suitable reaction conditions (Table 1). To our delight, the reaction was performed in CH3CN using tert-butyl hydroperoxide (TBHP, 70% water) as an oxidant at 90 °C for 6 h, and 30% yield of 3-arylseleno chromone 3a was obtained (entry 1, Table 1). Encouraged by this preliminary result, other oxidants including di-tert-butyl peroxide (DTBP), (NH4)2S2O8, K2S2O8, Selectfluor, PhI(OAc)2, and PhI(OCOCF3)2 were screened, the result showed that DTBP was noneffective, however Selectfluor could provide the highest yield (83%, entries 2–9, Table 1). Then, the effect of Selectfluor loading was investigated, and 1.0 equiv. was the best choice to give 83% yield (entries 5, 8 and 9, Table 1). The investigation of various solvents such as DMF, DMSO, THF, CH3OH, DCE, dioxane, and H2O, revealing that CH3CN was the optimal solvent for this reaction and afforded 3a in 83% yield (entries 5 and 10–16). The molar ratio of 1a and 2a was also screened. Decreasing the amount of 2a from 1.0 to 0.5 equiv., the yield of 3a was reduced to 76%, and no obvious change in yield was observed increasing 2a loading for 1.0 to 1.5 equiv. (entries 5, 17 and 18, Table 1). Changing the temperature from 20 °C to 90 °C, 40 °C was the best to provide 85% yield (entries 5, 19–21, Table 1). Moreover, the effect of reaction time was also tested. 2 h was proved be optimal time, and provided 3a in 85% yield (entries 5 and 22–24, Table 1). No desired product 3a was detected in the absence of Selectfluor reagent (entry 25, Table 1), which indicated that Selectfluor was crucial for the reaction to occur. Therefore, the best conditions for the synthesis of 3a were identified as follows: the molar ratio of 1a and 2a is 1
:
1, 1.0 equiv. Selectfluor as the oxidant in CH3CN at 40 °C for 2 h.
Table 1 Optimization of reaction conditionsa
With the optimal reaction conditions identified, the scope and generality of this transformation were firstly evaluated using divergent enaminones (Table 2). Generally, the process is compatible for enaminones with different R groups at the benzene ring containing electron-donating (Me, OMe) and electron-withdrawing (F, Cl, and Br) substituents, which reacted with diphenyl diselenide 2a gave the corresponding products 3aa–3fa in 76–90% yields under the standard conditions. It is noteworthy that an exclusive C-3 site selectivity in this selenylation/cyclization was observed for all the different enaminones. Of particular note was the successful synthesis of the expected 3-arylseleno chromones (3ea, 3fa) bearing chloro and bromo moiety, which provided an opportunity for further functional modification. Furthermore, di-substituted substrates were also suitable for this transformation, delivering the respective products (3ga, 3ha) in good to excellent yields. Notedly, when enaminone at the benzene ring with a strong electron-withdrawing –NO2 group was employed, the selenylation/cyclization reaction could tolerate the reaction conditions to provide 3ia in 75% yield. Gratifyingly, when a fused aromatic substrate (naphthyl) was employed, the reaction could also proceed smoothly to obtain the desired 3ja in excellent yields (83%).
Table 2 Scope of enaminones for the tandem cyclizationa,b
Reaction conditions: enaminone 1 (0.2 mmol), diphenyl diselenide 2a (0.2 mmol, 62.8 mg), Selectfluor agent (0.2 mmol, 70.8 mg) in CH3CN (2.0 mL) at 40 °C for 2.0 h. Isolated yield. |
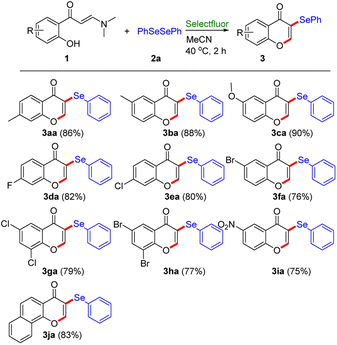 |
Subsequently, we turned our attention to explore the substrate range of diselenides under the standard conditions (Table 3). All of diaryl diselenides 2 with various groups (Me, Et, t-Bu, OMe, F, Cl, Br, I, and OCF3) work well with 2-hydroxyphenyl enaminone 1a, providing the corresponding 3-arylselenenyl enaminone derivatives in good to excellent yields (3a–3p, 55–94%). The diaryl diselenides with electron-donating groups (–Me, –Et, –tBu, –OMe) could provide higher yields than those with electron-withdrawing (–F, –Cl, –Br, –I, –OCF3) substituents. Gratifyingly, when diaryl diselenide with electron-withdrawing group (–OCF3) was employed, this transformation could proceed smoothly to obtain the corresponding product 3p in 73% yield. Moreover, the reaction proceeded smoothly with 1,2-di(naphthalen-2-yl)diselane, furnishing the desired product 3q in 70% yield. Unfortunately, 1,2-di(naphthalen-1-yl)diselane was used as substrate under the standard conditions, this reaction failed to afford the desired product 3t, which suggests that the steric effect had an important effect on this transformation. Besides the generally good results employing conventional diaryl diselenides, a notable point was that heteroaryl diselenide, 1,2-di(thiophen-2-yl)diselane, also exhibited satisfactory tolerance to the synthetic protocol to provide the desired product 3r, albeit in low yield (58%). Interestingly, when aliphatic diselenide, 1,2-dibenzyldiselane, was employed, the reaction could also proceed smoothly to obtain the desired product 3s in excellent yields (84%).
Table 3 Scope of diselenides on the tandem cyclization of enaminonesa,b
Reaction conditions: enaminone 1a (0.2 mmol, 38.2 mg), diaryl diselenide 2 (0.2 mmol), Selectfluor agent (0.2 mmol, 70.8 mg) in CH3CN (2.0 mL) at 40 °C for 2.0 h. Isolated yield. |
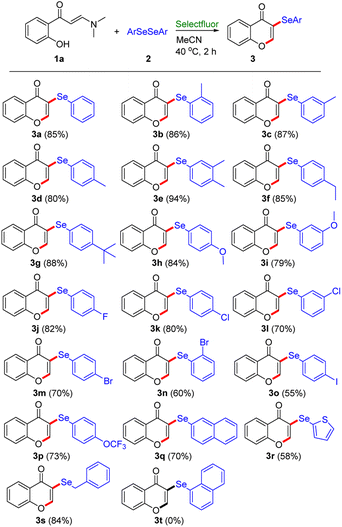 |
Organic thiocyanates as key skeletal structures are moieties possessing enriched biological and pharmaceutical activities in both synthesized and naturally occurring molecules.27 Moreover, the enriched reactivity of the thiocyano group also endows organic thiocyanates with widespread application as building blocks in organic synthesis.28 However, the synthesis of thiocyanochromenones is rare.29 In order to testify the utility and robustness of this protocol, Selectfluor-promoted synthesis of 3-thiocyanochromenone derivatives was explored (Table 4). We were glad to find that our protocol was applied well in the synthesis of 3-thiocyanochromenone derivatives using KSCN as a thiocyano source under the standard conditions. In general, all of enaminones 1 at the benzene ring with either electron-donating groups (Me, OMe) or electron-withdrawing groups (F, Cl, and Br) reacted smoothly to provide the corresponding products 5a–5h in good yields (76–86%). Unfortunately, enaminone with strong electron-withdrawing group (–NO2) failed to provide the desired product (5i), potentially due to the low reactivity of this intermediate, thus producing difficultly the cyclizing product. Gratifyingly, disubstituted enaminones also actively participated in this transformation to dispense the desired products 5j and 5k in 82% and 76% yield, respectively.
Table 4 Synthesis of 3-thiocyanochromonesa,b
Reaction conditions: enaminones 1 (0.2 mmol), potassium thiocyanate 4 (0.2 mmol, 19.4 mg), Selectfluor agent (0.2 mmol, 70.8 mg) in CH3CN (2.0 mL) at 40 °C for 2.0 h. Isolated yield. |
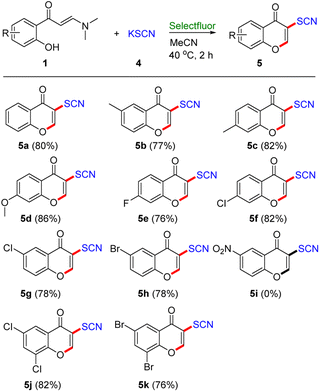 |
Additionally, to verify the utility of this protocol, gram–scale reactions were conducted using 2-hydroxyphenyl enaminone 1a (5 mmol, 0.955 g) with diphenyl diselenide 2a under the standard conditions. As expected, the corresponding desired products 3a and 5a were obtained in 80% and 76% yield, respectively (Scheme 2a), which provides promising application in preparative synthesis. Then, the synthetic transformation of 5a was carried out to explore the thiocyano group with widespread application as a building block in organic synthesis (Scheme 2b).
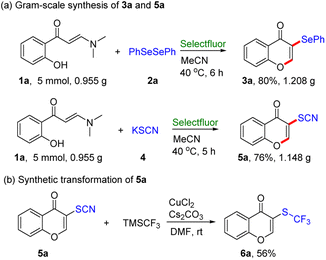 |
| Scheme 2 Gram-scale synthesis of 3a/5a, and synthetic transformation of 5a. | |
To investigate the possible process of the reaction, several control experiments were designed as outlined in Scheme 3. Initially, the entry directly employing chromone 6 and diphenyl diselenide 2a with standard reaction conditions was found to be incapable of yielding target product 3a, and 92% of the substrate 6 was recovered (Scheme 3a), which showed that the annulation to chromone was not the initial step in the reaction. Subsequently, radical trapping experiments of enaminone 1a and diphenyl diselenide 2a were examined in the presence of 2,2,6,6-tetramethyl-1-piperidinyloxyl (TEMPO, 3.0 equiv.) or 2,6-di-tert-butyl-4-methylphenol (BHT, 3.0 equiv.) under the standard conditions, and the desired product 3a was provided in 70% and 73% yield, respectively (Scheme 3b). These results indicate that the reaction might not involve radical intermediates, which was in agreement with the case in previous work.26a Furthermore, when phenyl hypochloroselenoite was added to the reaction system instead of diphenyl diselenide 2a under the standard conditions, the product 3a was obtained with 82% yield (Scheme 3c), demonstrating that this transformation may proceed via an ion pathway, instead of a radical pathway.
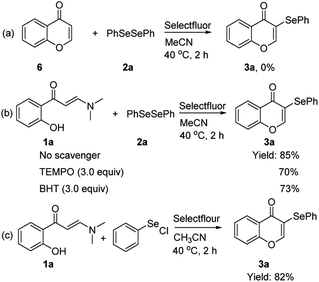 |
| Scheme 3 Control experiments. (a) Direct selenylation of chromone; (b) radical trapping experiments; (c) phenyl hypochloroselenoite as selenylation reagent. | |
On the basis of these results and previous reports,19–23 a plausible mechanism for this transformation was proposed (Scheme 4). Initially, the reaction involves the oxidation of diphenyl diselenide by Selectfluor reagent to form the electrophilic species I and II.26b,d Then, these species attack C–C double bond of 2-hydroxyphenyl enaminone 1a to form species III. Subsequently, the species III would afford the cyclic intermediate IV through the intramolecular nucleophilic attack of the carbon atom of the C
N moiety. The intermediate V is made by the proton transfer. Finally, the elimination of dimethylamine from the intermediate V would furnish the expected product 3a. For the synthetic mechanism of 3-thiocyanochromenone derivatives, the thiocyano cation could be formed with KSCN using Selectfluor reagent as an oxidant. 3-Thiocyanochromenone derivatives could be provided by the similar mechanism.
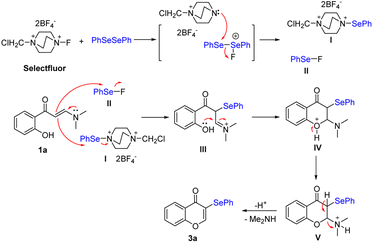 |
| Scheme 4 Proposed reaction mechanism. | |
Conclusions
In conclusion, an intriguing Selectfluor-promoted, metal-free, and efficient synthetic approach to access 3-selenylated chromones have been developed from enaminones and easily available diaryl diselenides. This approach exhibits a broad substrate scope, simple procedure, mild reaction condition, good to excellent yields and high selectivity. Furthermore, the application of potassium thiocyanate with enaminones for the synthesis of 3-thiocyanochromenone derivatives is also successful.
Experimental
General information
All chemicals were commercially available and used as received without further. Column chromatography was performed using 300–400 mesh silica. Nuclear magnetic resonance spectra were recorded on Bruker Avance 400 MHz spectrometer. Chemical shifts for 1H NMR spectra are recorded in parts per million from tetramethylsilane. Data were reported as follows: chemical shift, multiplicity (s = singlet, d = doublet, t = triplet, m = multiplet and br = broad), coupling constant in Hz and integration. Chemical shifts for 13C NMR spectra were recorded in parts per million from tetramethylsilane. Chemical shifts for 19F NMR spectra were recorded in parts per million with fluorobenzene as external standard. High resolution mass spectra (HR MS) were obtained on Thermo Scientific LTQ Orbitrap XL instrument using the ESI technique. IR spectra were recorded on WQF-510 Fourier transform infrared spectrophotometer. Melting points were measured on an XT4A microscopic apparatus uncorrected.
General experimental procedure for the synthesis of 3-arylselenenyl chromones (3)
Enaminones 1 (0.2 mmol), diaryl diselenides 2 (0.2 mmol), Selectfluor agent (0.2 mmol, 70.8 mg), and acetonitrile (2.0 mL) were added to a 10 mL reaction tube. The mixture was stirred at 40 °C for 2 h. After completion of the reaction, the solvent was distilled under vacuum. Then, the resulting mixture was dissolved with ethyl acetate (20 mL), washed with saturated sodium chloride solution (10 mL × 2). The organic phase was dried over anhydrous Na2SO4 and concentrated under vacuum. The residue was purified by silica gel column chromatography to give 3-arylseleno chromones 3 using ethyl acetate/petroleum ether as eluant.
7-Methyl-3-(phenylselanyl)-4H-chromen-4-one (3aa). Light yellow crystal, mp 107–108 °C (lit.16b 99–100 °C); IR (KBr) ν (cm−1): 2921, 1644, 1621, 1476, 1435, 1344, 1298, 1064; 1H NMR (400 MHz, CDCl3) δ: 8.10 (d, JH–H = 8.0 Hz, 1H), 7.85 (s, 1H), 7.59–7.57 (m, 2H), 7.29–7.26 (m, 3H), 7.22–7.20 (m, 2H), 2.46 (s, 3H); 13C NMR (100 MHz, CDCl3) δ: 175.0 (C
O), 156.5, 155.6 (CH), 145.3, 133.7 (CH), 129.5 (CH), 128.3, 128.0 (CH), 127.1 (CH), 126.1 (CH), 120.9, 117.7 (CH), 117.6, 21.8 (CH3); HR MS (ESI) m/z: calcd for C16H13O2Se [M + H]+ 317.0075, found 317.0069.
6-Methyl-3-(phenylselanyl)-4H-chromen-4-one (3ba). Colorless crystal, mp 96–97 °C (lit.16b 99–100 °C); IR (KBr) ν (cm−1): 1640, 1616, 1598, 1477, 1436, 1308, 1227, 1067; 1H NMR (400 MHz, CDCl3) δ: 8.02 (d, JH–H = 1.0 Hz, 1H), 7.90 (s, 1H), 7.60–7.57 (m, 2H), 7.47 (dd, JH–H = 8.5 Hz, JH–H = 2.0 Hz, 1H), 7.33 (d, JH–H = 8.6 Hz, 1H), 7.29–7.28 (m, 3H), 2.44 (s, 3H); 13C NMR (100 MHz, CDCl3) δ: 175.3 (C
O), 155.9 (CH), 154.6 (CH), 135.6, 135.1 (CH), 133.6 (CH), 129.5 (CH), 128.4, 128.0 (CH), 125.6 (CH), 122.8, 117.8 (CH), 117.4, 20.9 (CH3); HR MS (ESI) m/z: calcd for C16H13O2Se [M + H]+ 317.0075, found 317.0068.
6-Methoxy-3-(phenylselanyl)-4H-chromen-4-one (3ca). Colorless crystal, mp 99–100 °C (lit.20a 99–101 °C); IR (KBr) ν (cm−1): 2932, 1630, 1615, 1546, 1487, 1264; 1H NMR (400 MHz, CDCl3) δ: 7.92 (s, 1H), 7.60–7.58 (m, 3H), 7.37 (d, JH–H = 9.2 Hz, 1H), 7.30–7.24 (m, 4H), 3.88 (s, 3H); 13C NMR (100 MHz, CDCl3) δ: 175.0 (C
O), 157.2, 155.8 (CH), 151.2, 133.6 (CH), 129.5 (CH), 128.4, 128.0 (CH), 124.0 (CH), 123.8, 119.5 (CH), 116.8, 105.2 (CH), 55.9 (CH3); HR MS (ESI) m/z: calcd for C16H13O3Se [M + H]+ 333.0024, found 333.0019.
7-Fluoro-3-(phenylselanyl)-4H-chromen-4-one (3da). Colorless crystal, mp 115–116 °C (lit.16b 120–121 °C); IR (KBr) ν (cm−1): 1605, 1474, 1431, 1349, 1251, 1092; 1H NMR (400 MHz, CDCl3) δ: 8.26–8.22 (m, 1H), 7.79 (s, 1H), 7.61–7.59 (m, 2H), 7.32–7.30 (m, 3H), 7.16–7.11 (m, 2H); 13C NMR (100 MHz, CDCl3) δ: 174.3 (C
O), 165.6 (d, JF–C = 254.2 Hz), 157.3 (d, JF–C = 13.2 Hz), 155.3 (CH), 134.1 (CH), 129.6 (CH), 129.0 (CH), 128.9 (CH), 128.3 (CH), 127.6, 119.9 (d, JF–C = 2.3 Hz), 118.4, 114.4 (d, JF–C = 22.7 Hz, CH), 104.7 (d, JF–C = 25.2 Hz, CH); 19F NMR (376 MHz, CDCl3) δ: −102.1. HR MS (ESI) m/z: calcd for C15H10FO2Se [M + H]+ 320.9825, found 320.9818.
7-Chloro-3-(phenylselanyl)-4H-chromen-4-one (3ea). Light yellow crystal, mp 111–112 °C (lit.16b 112–113 °C); IR (KBr) ν (cm−1): 1651, 1602, 1576, 1477, 1339, 1282, 1021; 1H NMR (400 MHz, CDCl3) δ: 8.13 (d, JH–H = 8.6 Hz, 1H), 7.76 (s, 1H), 7.60–7.58 (m, 2H), 7.40 (d, JH–H = 1.8 Hz, 1H), 7.35–7.27 (m, 4H); 13C NMR (100 MHz, CDCl3) δ: 174.3 (C
O), 156.3, 155.0 (CH), 139.8, 134.2 (CH), 129.6 (CH), 128.4 (CH), 127.6 (CH), 127.5, 126.4 (CH), 121.4, 118.6, 118.0 (CH); HR MS (ESI) m/z: calcd for C15H10ClO2Se [M + H]+ 336.9529, found 336.9519.
6-Bromo-3-(phenylselanyl)-4H-chromen-4-one (3fa). Colorless crystal, mp 90–91 °C (lit.16b 90–91 °C); IR (KBr) ν (cm−1): 1645, 1601, 1540, 1422, 1348, 1297, 1058; 1H NMR (400 MHz, CDCl3) δ: 8.34 (d, JH–H = 2.4 Hz, 1H), 7.83 (s, 1H), 7.73 (dd, JH–H = 8.9 Hz, JH–H = 2.4 Hz, 1H), 7.61–7.58 (m, 1H), 7.33–7.30 (m, 4H); 13C NMR (100 MHz, CDCl3) δ: 173.9 (C
O), 155.4 (CH), 155.0, 136.8 (CH), 134.1 (CH), 129.6 (CH), 128.8 (CH), 128.4 (CH), 127.5, 124.2, 120.0 (CH), 118.9, 118.3; HR MS (ESI) m/z: calcd for C15H10BrO2Se [M + H]+ 380.9024, found 380.9010.
6,8-Dichloro-3-(phenylselanyl)-4H-chromen-4-one (3ga). Light yellow crystal, mp 114–115 °C (lit.20a 113–115 °C); IR (KBr) ν (cm−1): 1644, 1591, 1447, 1356, 1296, 1197; 1H NMR (400 MHz, CDCl3) δ: 8.08 (d, JH–H = 2.5 Hz, 1H), 7.76 (s, 1H), 7.68 (d, JH–H = 2.5 Hz, 1H), 7.64–7.61 (m, 2H), 7.36–7.30 (m, 3H); 13C NMR (100 MHz, CDCl3) δ: 173.5 (C
O), 154.2 (CH), 150.6, 134.8 (CH), 133.9 (CH), 131.1, 129.8 (CH), 128.8 (CH), 126.6, 124.4, 124.3 (CH), 119.3; HR MS (ESI) m/z: calcd for C15H9Cl2O2Se [M + H]+ 370.9139, found 370.9141.
6,8-Dibromo-3-(phenylselanyl)-4H-chromen-4-one (3ha). Orange yellow crystal, mp 115–116 °C (lit.20a 118–119 °C); IR (KBr) ν (cm−1): 1656, 1540, 1449, 1345, 1288, 1069; 1H NMR (400 MHz, CDCl3) δ: 8.29 (d, JH–H = 2.4 Hz, 1H), 8.00 (d, JH–H = 2.4 Hz, 1H), 7.77 (s, 1H), 7.64–7.62 (m, 2H), 7.37–7.31 (m, 3H); 13C NMR (100 MHz, CDCl3) δ: 173.5 (C
O), 154.2 (CH), 152.0, 139.5 (CH), 134.8 (CH), 129.8 (CH), 128.8 (CH), 128.2 (CH), 126.6, 124.7, 119.3, 118.7, 112.8; HR MS (ESI) m/z: calcd for C15H9Br2O2Se [M + H]+ 458.8129, found 458.8134.
6-Nitro-3-(phenylselanyl)-4H-chromen-4-one (3ia). Yellow crystal, mp 108–109 °C (lit.16b 115–116 °C); IR (KBr) ν (cm−1): 1637, 1574, 1437, 1344, 1206, 1089; 1H NMR (400 MHz, CDCl3) δ: 9.08 (d, JH–H = 2.7 Hz, 1H), 8.48 (dd, JH–H = 9.1 Hz, JH–H = 2.4 Hz, 1H), 7.76 (s, 1H), 7.65–7.63 (m, 2H), 7.59 (d, JH–H = 9.2 Hz, 1H), 7.39–7.32 (m, 3H); 13C NMR (100 MHz, CDCl3) δ: 173.9 (C
O), 159.0, 154.4 (CH), 144.9, 134.9 (CH), 129.8 (CH), 128.9 (CH), 128.0 (CH), 126.4, 123.0 (CH), 122.7, 120.0, 119.9 (CH); HR MS (ESI) m/z: calcd for C15H10NO4Se [M + H]+ 347.9770, found 347.9773.
3-(Phenylselanyl)-4H-benzo[h]chromen-4-one (3ja). Light yellow crystal, mp 110–112 °C (lit.16b 105–106 °C); IR (KBr) ν (cm−1): 1618, 1559, 1464, 1301, 1260, 1155; 1H NMR (400 MHz, CDCl3) δ: 8.35 (d, JH–H = 8.2 Hz, 1H), 8.13 (d, JH–H = 8.8 Hz, 1H), 7.90–7.87 (m, 2H), 7.72 (d, JH–H = 8.7 Hz, 1H), 7.69–7.59 (m, 4H), 7.34–7.31 (m, 3H); 13C NMR (100 MHz, CDCl3) δ: 175.0 (C
O), 153.8 (CH), 135.7, 134.4 (CH), 129.7 (CH), 129.4 (CH), 128.4 (CH), 128.1 (CH), 127.5, 127.2 (CH), 125.6 (CH), 123.8, 122.1 (CH), 121.0 (CH), 120.2, 119.1; HR MS (ESI) m/z: calcd for C19H13O2Se [M + H]+ 353.0075, found 353.0069.
3-(Phenylselanyl)-4H-chromen-4-one (3a). Light yellow crystal, mp 63–64 °C (lit.22 yellow liquid); IR (KBr) ν (cm−1): 1626, 1610, 1461, 1342, 1110; 1H NMR (400 MHz, CDCl3) δ: 8.24–8.21 (m, 1H), 7.89 (s, 1H), 7.68–7.63 (m, 1H), 7.60–7.58 (m, 2H), 7.43–7.38 (m, 2H), 7.31–7.27 (m, 3H); 13C NMR (100 MHz, CDCl3) δ: 175.2 (C
O), 156.3, 155.8 (CH), 133.9 (CH), 133.8 (CH), 129.5 (CH), 128.1 (CH), 126.3 (CH), 125.5 (CH), 123.1, 118.0 (CH), 117.8; HR MS (ESI) m/z: calcd for C15H11O2Se [M + H]+ 302.9919, found 302.9911.
3-(o-Tolylselanyl)-4H-chromen-4-one (3b). Light yellow crystal, mp 95–96 °C (lit.22 118–119 °C); IR (KBr) ν (cm−1): 2920, 1643, 1610, 1343, 1251, 1073; 1H NMR (400 MHz, CDCl3) δ: 8.25 (dd, JH–H = 8.5 Hz, JH–H = 1.8 Hz, 1H), 7.69–7.65 (m, 2H), 7.48 (dd, JH–H = 7.5 Hz, JH–H = 0.8 Hz, 1H), 7.44–7.41 (m, 2H), 7.25–7.23 (m, 2H), 7.21–7.08 (m, 1H), 2.50 (s, 3H); 13C NMR (100 MHz, CDCl3) δ: 175.3 (C
O), 156.4, 154.7 (CH), 140.6, 134.4 (CH), 133.8 (CH), 130.5 (CH), 128.5 (CH), 128.4, 127.0 (CH), 126.3 (CH), 125.5 (CH), 122.9, 118.0 (CH), 117.3, 22.3 (CH3); HR MS (ESI) m/z: calcd for C16H13O2Se [M + H]+ 317.0075, found 317.0070.
3-(m-Tolylselanyl)-4H-chromen-4-one (3c). Light yellow crystal, mp 73–74 °C; IR (KBr) ν (cm−1): 2915, 1651, 1551, 1455, 1359, 1334, 1306, 1069; 1H NMR (400 MHz, CDCl3) δ: 8.23 (dd, JH–H = 8.4 Hz, JH–H = 1.7 Hz, 1H), 7.85 (s, 1H), 7.68–7.63 (m, 1H), 7.43–7.38 (m, 4H), 7.18 (t, JH–H = 7.6 Hz, 1H), 7.11 (d, JH–H = 7.6 Hz, 1H), 2.31 (s, 3H); 13C NMR (100 MHz, CDCl3) δ: 175.2 (C
O), 156.3, 155.4 (CH), 139.4, 134.5 (CH), 133.7 (CH), 131.0 (CH), 129.3 (CH), 129.0 (CH), 127.7, 126.3 (CH), 125.5, 123.1, 118.0 (CH), 21.3 (CH3); HR MS (ESI) m/z: calcd for C16H13O2Se [M + H]+ 317.0075, found 317.0070.
3-(p-Tolylselanyl)-4H-chromen-4-one (3d). Light yellow crystal, mp 85–86 °C (lit.22 83–84 °C); IR (KBr) ν (cm−1): 2918, 1641, 1606, 1550, 1486, 1360, 1341, 1326, 1207; 1H NMR (400 MHz, CDCl3) δ: 8.23 (dd, JH–H = 8.5 Hz, JH–H = 1.9 Hz, 1H), 7.77 (s, 1H), 7.67–7.63 (m, 1H), 7.53 (d, JH–H = 8.1 Hz, 2H), 7.42–7.38 (m, 2H), 7.12 (d, JH–H = 7.8 Hz, 2H), 2.33 (s, 3H); 13C NMR (100 MHz, CDCl3) δ: 175.2 (C
O), 156.3, 154.8 (CH), 138.5, 134.6 (CH), 133.7 (CH), 130.4 (CH), 126.2 (CH), 125.4 (CH), 123.8, 123.0, 118.6, 118.0 (CH), 21.2 (CH3); HR MS (ESI) m/z: calcd for C16H13O2Se [M + H]+ 317.0075, found 317.0070.
3-((3,4-Dimethylphenyl)selanyl)-4H-chromen-4-one (3e). Light yellow crystal, mp 110–111 °C; IR (KBr) ν (cm−1): 2917, 1620, 1606, 1554, 1450, 1312, 1107; 1H NMR (400 MHz, CDCl3) δ: 8.23(dd, JH–H = 8.0 Hz, JH–H = 1.6 Hz, 1H), 7.74 (s, 1H), 7.67–7.63 (m, 1H), 7.42–7.35 (m, 4H), 7.07 (d, JH–H = 7.8 Hz, 1H),2.24 (s, 3H), 2.23 (s, 3H); 13C NMR (100 MHz, CDCl3) δ: 175.2 (C
O), 156.3, 154.5 (CH), 138.2, 137.2, 135.7 (CH), 133.7 (CH), 132.2 (CH), 130.8 (CH), 126.3 (CH), 125.4 (CH), 123.9, 123.0, 118.7, 118.0 (CH), 19.6 (CH3), 19.5 (CH3); HR MS (ESI) m/z: calcd for C17H15O2Se [M + H]+ 331.0232, found 331.0226.
3-((4-Ethylphenyl)selanyl)-4H-chromen-4-one (3f). Light yellow crystal, mp 98–99 °C (lit.30 yellow oil liquid); IR (KBr) ν (cm−1): 2963, 1636, 1610, 1462, 1342, 1309, 1109, 1057; 1H NMR (400 MHz, CDCl3) δ: 8.24 (dd, JH–H = 8.4 Hz, JH–H = 1.8 Hz, 1H), 7.79 (s, 1H), 7.68–7.64 (m, 1H), 7.55 (d, JH–H = 8.2 Hz, 2H), 7.43–7.39 (m, 2H), 7.15 (d, JH–H = 8.2 Hz, 2H), 2.63 (q, JH–H = 7.6 Hz, 2H), 1.22 (t, JH–H = 7.6 Hz, 3H); 13C NMR (100 MHz, CDCl3) δ: 175.4 (C
O), 156.3 (CH), 154.9 (CH), 144.8, 134.6 (CH), 133.7 (CH), 129.2 (CH), 126.3 (CH), 125.4 (CH), 124.1, 123.0, 118.5, 118.0 (CH), 28.5 (CH2), 15.4 (CH3); HR MS (ESI) m/z: calcd for C17H15O2Se [M + H]+ 331.0232, found 331.0227.
3-((4-(tert-Butyl)phenyl)selanyl)-4H-chromen-4-one (3g). Light yellow crystal, mp 110–111 °C; IR (KBr) ν (cm−1): 2959, 2922, 1642, 1607, 1555, 1459, 1342, 1111; 1H NMR (400 MHz, CDCl3) δ: 8.24 (dd, JH–H = 8.4 Hz, JH–H = 1.5 Hz, 1H), 7.82 (s, 1H), 7.65 (td, JH–H = 7.8 Hz, JH–H = 1.7 Hz, 1H), 7.55 (d, JH–H = 8.4 Hz, 2H), 7.42–7.39 (m, 2H), 7.33 (d, JH–H = 8.5 Hz, 2H), 1.30 (s, 9H); 13C NMR (100 MHz, CDCl3) δ: 175.3 (C
O), 156.3, 155.0 (CH), 151.6, 134.2 (CH), 133.7 (CH), 126.7 (CH), 126.3 (CH), 125.4 (CH), 124.1, 123.0, 118.3, 118.0 (CH), 34.6, 31.2 (CH3); HR MS (ESI) m/z: calcd for C19H19O2Se [M + H]+ 359.0545, found 259.0538.
3-((4-Methoxyphenyl)selanyl)-4H-chromen-4-one (3h). Light yellow crystal, mp 125–126 °C (lit.22 131–132 °C); IR (KBr) ν (cm−1): 2959, 1643, 1581, 1552, 1462, 1245, 1022; 1H NMR (400 MHz, CDCl3) δ: 8.22 (dd, JH–H = 8.3 Hz, JH–H = 1.6 Hz, 1H), 7.66–7.59 (m, 4H), 7.41–7.37 (m, 2H), 6.86 (d, JH–H = 8.8 Hz, 2H), 3.80 (s, 3H); 13C NMR (100 MHz, CDCl3) δ: 175.3 (C
O), 160.2, 156.3, 153.8 (CH), 137.1 (CH), 133.6 (CH), 126.1 (CH), 125.3 (CH), 122.8, 119.3, 118.0 (CH), 117.0, 115.3 (CH), 55.3 (CH3); HR MS (ESI) m/z: calcd for C16H13O3Se [M + H]+ 333.0024, found 333.0018.
3-((3-Methoxyphenyl)selanyl)-4H-chromen-4-one (3i). Light yellow crystal, mp 89–90 °C (lit.16b 88–89 °C); IR (KBr) ν (cm−1): 2962, 1648, 1586, 1551, 1454, 1288, 1038; 1H NMR (400 MHz, CDCl3) δ: 8.23 (dd, JH–H = 7.9 Hz, JH–H = 1.4 Hz, 1H), 7.91 (s, 1H), 7.68–7.64 (m, 1H), 7.43–7.39 (m, 2H), 7.20 (t, JH–H = 7.8 Hz, 1H), 7.18–7.14 (m, 2H), 6.84–6.81 (m, 1H), 3.77 (s, 3H); 13C NMR (100 MHz, CDCl3) δ: 175.1 (C
O), 160.0, 156.3, 155.8 (CH), 133.8 (CH), 130.3 (CH), 129.0, 126.3 (CH), 125.8 (CH), 125.5 (CH), 123.1, 118.9 (CH), 118.0 (CH), 117.6, 113.9 (CH), 55.3 (CH3); HR MS (ESI) m/z: calcd for C16H13O3Se [M + H]+ 333.0024, found 333.0018.
3-((4-Fluorophenyl)selanyl)-4H-chromen-4-one (3j). Light yellow crystal, mp 93–94 °C (lit.16b 94–95 °C); IR (KBr) ν (cm−1): 1643, 1361, 1221, 1064; 1H NMR (400 MHz, CDCl3) δ: 8.23 (dd, JH–H = 8.3 Hz, JH–H = 1.5 Hz, 1H), 7.90 (s, 1H), 7.69–7.65 (m, 1H), 7.64–7.60 (m, 2H), 7.44–7.40 (m, 2H), 7.00 (t, JH–H = 8.7 Hz, 2H); 13C NMR (100 MHz, CDCl3) δ: 175.2 (C
O), 162.9 (d, JF–C = 247.0 Hz), 156.3, 155.5 (CH), 136.4 (d, JF–C = 7.9 Hz), 133.9, 126.3, 125.6, 123.1, 122.6, 122.5, 118.0, 116.7 (d, JF–C = 21.3 Hz); 19F NMR (376 MHz, CDCl3) δ: −112.9. HR MS (ESI) m/z: calcd for C15H10FO2Se [M + H]+ 320.9825, found 320.9818.
3-((4-Chlorophenyl)selanyl)-4H-chromen-4-one (3k). Light yellow crystal, mp 133–134 °C (lit.22 133–134 °C); IR (KBr) ν (cm−1): 1628, 1551, 1460, 1308, 1249, 1075; 1H NMR (400 MHz, CDCl3) δ: 8.23 (dd, JH–H = 8.0 Hz, JH–H = 1.4 Hz, 1H), 8.01 (s, 1H), 7.71–7.66 (m, 1H), 7.52 (d, JH–H = 8.5 Hz, 2H), 7.46–7.41 (m, 2H), 7.26–7.24 (m, 2H); 13C NMR (100 MHz, CDCl3) δ: 175.0 (C
O), 156.4 (CH), 156.3, 134.8 (CH), 134.3, 133.9 (CH), 129.6 (CH), 126.6, 126.4 (CH), 125.7 (CH), 123.2, 118.1 (CH), 117.2; HR MS (ESI) m/z: calcd for C15H10ClO2Se [M + H]+ 336.9529, found 336.9519.
3-((3-Chlorophenyl)selanyl)-4H-chromen-4-one (3l). Light yellow crystal, mp 89–90 °C (lit.16b 90–91 °C); IR (KBr) ν (cm−1): 1652, 1605, 1564, 1552, 1405, 1360, 1308, 1101; 1H NMR (400 MHz, CDCl3) δ: 8.24 (dd, JH–H = 8.0 Hz, JH–H = 1.4 Hz, 1H), 8.09 (s, 1H), 7.72–7.67 (m, 1H), 7.53 (t, JH–H = 1.6 Hz, 1H), 7.47–7.42 (m, 3H), 7.25–7.18 (m, 2H); 13C NMR (100 MHz, CDCl3) δ: 175.0 (C
O), 157.1 (CH), 156.4, 134.9, 134.0 (CH), 132.4 (CH), 130.9 (CH), 130.4 (CH), 128.0 (CH), 126.4 (CH), 125.8 (CH), 123.3, 118.1 (CH), 116.6; HR MS (ESI) m/z: calcd for C15H10ClO2Se [M + H]+ 336.9529, found 336.9521.
3-((4-Bromophenyl)selanyl)-4H-chromen-4-one (3m). Light yellow crystal, mp 124–125 °C (lit.16b 129–130 °C); IR (KBr) ν (cm−1): 1627, 1608, 1549, 1379, 1329, 1100; 1H NMR (400 MHz, CDCl3) δ: 8.23 (dd, JH–H = 8.0 Hz, JH–H = 1.6 Hz, 1H), 8.03 (s, 1H), 7.71–7.66 (m, 1H), 7.46–7.38 (m, 6H); 13C NMR (100 MHz, CDCl3) δ: 175.0 (C
O), 156.6 (CH), 156.3, 134.9 (CH), 134.0 (CH), 132.5 (CH), 127.4, 126.4 (CH), 125.7 (CH), 123.2, 122.4, 118.1 (CH), 117.0; HR MS (ESI) m/z: calcd for C15H10BrO2Se [M + H]+ 380.9024, found 380.9014.
3-((2-Bromophenyl)selanyl)-4H-chromen-4-one (3n). Yellow crystal, mp 130–131 °C; IR (KBr) ν (cm−1): 1637, 1610, 1549, 1436, 1313, 1167; 1H NMR (400 MHz, CDCl3) δ: 8.23 (dd, JH–H = 8.0 Hz, JH–H = 1.6 Hz, 1H), 8.05 (s, 1H), 7.71–7.67 (m, 1H), 7.59 (d, JH–H = 8.4 Hz, 2H), 7.46–7.41 (m, 2H), 7.30 (d, JH–H = 8.4 Hz, 2H); 13C NMR (100 MHz, CDCl3) δ: 175.0 (C
O), 159.3 (CH), 156.5, 134.1 (CH), 133.0, 132.9 (CH), 131.1 (CH), 128.1 (CH), 128.0 (CH), 126.6 (CH), 125.9 (CH), 124.3, 123.6, 118.2 (CH), 115.2; HR MS (ESI) m/z: calcd for C15H10BrO2Se [M + H]+ 380.9024, found 380.9013.
3-((4-Iodophenyl)selanyl)-4H-chromen-4-one (3o). Light yellow crystal, mp 121–122 °C; IR (KBr) ν (cm−1): 1629, 1548, 1458, 1309, 1063; 1H NMR (400 MHz, CDCl3) δ: 8.23 (dd, JH–H = 7.9 Hz, JH–H = 1.6 Hz, 1H), 8.05 (s, 1H), 7.71–7.67 (m, 1H), 7.59 (d, JH–H = 8.3 Hz, 2H), 7.46–7.42 (m, 2H), 7.30 (d, JH–H = 8.4 Hz, 2H); 13C NMR (100 MHz, CDCl3) δ: 175.0 (C
O), 156.8, 156.4, 138.4, 134.9, 134.0, 128.5, 126.4, 125.7, 123.3, 118.1, 116.9, 93.8; HR MS (ESI) m/z: calcd for C15H10IO2Se [M + H]+ 428.8885, found 428.8874.
3-((4-(Trifluoromethoxy)phenyl)selanyl)-4H-chromen-4-one (3p). Light yellow crystal, mp 90–91 °C; IR (KBr) ν (cm−1): 1640, 1611, 1554, 1461, 1112, 1062; 1H NMR (400 MHz, CDCl3) δ: 8.24 (dd, JH–H = 7.8 Hz, JH–H = 1.6 Hz, 1H), 8.07 (s, 1H), 7.71–7.67 (m, 1H), 7.61 (d, JH–H = 8.8 Hz, 2H), 7.46–7.42 (m, 2H), 7.13 (dd, JH–H = 8.7 Hz, JH–H = 0.7 Hz, 2H); 13C NMR (100 MHz, CDCl3) δ: 175.1 (C
O), 156.9 (CH), 156.4, 149.1 (q, JF–C = 1.5 Hz), 134.8 (CH), 134.0 (CH), 126.9, 126.4 (CH), 125.8 (CH), 123.3, 121.9 (CH), 118.1 (CH), 116.9; 19F NMR (376 MHz, CDCl3) δ: −57.8. HR MS (ESI) m/z: calcd for C16H10F3O3Se [M + H]+ 386.9742, found 386.9734.
3-(Naphthalen-2-ylselanyl)-4H-chromen-4-one (3q). Light yellow crystal, mp 77–78 °C (lit.22 white liquid); IR (KBr) ν (cm−1): 1627, 1604, 1554, 1499, 1253, 1108; 1H NMR (400 MHz, CDCl3) δ: 8.40 (d, JH–H = 7.8 Hz, 1H), 8.25 (dd, JH–H = 8.0 Hz, JH–H = 1.6 Hz, 1H), 7.96 (dd, JH–H = 7.1 Hz, JH–H = 1.1 Hz, 1H), 7.90 (d, JH–H = 8.2 Hz, 1H), 7.86 (dd, JH–H = 7.0 Hz, JH–H = 1.6 Hz, 1H), 7.65–7.61 (m, 1H), 7.57–7.50 (m, 2H), 7.44–7.38 (m, 2H), 7.34 (d, JH–H = 8.5 Hz, 1H), 7.32 (s, 1H); 13C NMR (100 MHz, CDCl3) δ: 175.4 (C
O), 156.2, 153.3 (CH), 135.4 (CH), 134.3, 134.2, 133.7 (CH), 130.2 (CH), 128.7 (CH), 127.7 (CH), 127.4 (CH), 126.6 (CH), 126.1 (CH), 125.9, 125.4 (CH), 122.7, 118.3, 118.0 (CH); HR MS (ESI) m/z: calcd for C19H13O2Se [M + H]+ 353.0075, found 353.0069.
3-(Thiophen-2-ylselanyl)-4H-chromen-4-one (3r). Light yellow crystal, mp 119–120 °C (lit.16b 120–121 °C); IR (KBr) ν (cm−1): 1629, 1609, 1548, 1458, 1329, 1201, 1063; 1H NMR (400 MHz, CDCl3) δ: 8.22 (dd, JH–H = 8.3 Hz, JH–H = 1.7 Hz, 1H), 7.69–7.64 (m, 1H), 7.59 (s, 1H), 7.49 (d, JH–H = 8.4 Hz, 1H), 7.43–7.40 (m, 3H), 7.07–7.05 (m, 1H); 13C NMR (100 MHz, CDCl3) δ: 175.1 (C
O), 156.3, 153.3 (CH), 137.9 (CH), 133.8 (CH), 132.7 (CH), 128.6 (CH), 126.1 (CH), 125.5 (CH), 122.7, 120.0, 119.9, 118.0 (CH); HR MS (ESI) m/z: calcd for C13H9O2SSe [M + H]+ 308.9483, found 308.9477.
3-(Benzylselanyl)-4H-chromen-4-one (3s). Light yellow crystal, mp 109–110 °C (lit.16b 94–95 °C); IR (KBr) ν (cm−1): 1610, 1556, 1463, 1378, 1073; 1H NMR (400 MHz, CDCl3) δ: 8.27 (dd, JH–H = 8.0 Hz, JH–H = 1.6 Hz, 1H), 7.87 (s, 1H), 7.69–7.64 (m, 1H), 7.45–7.39 (m, 2H), 7.23–7.13 (m, 5H), 4.10 (s, 2H); 13C NMR (100 MHz, CDCl3) δ: 175.8 (C
O), 157.5 (CH), 156.3, 138.5, 133.7 (CH), 128.9 (CH), 128.4 (CH), 126.8 (CH), 126.3 (CH), 125.6 (CH), 123.3, 118.1 (CH), 114.2, 29.7 (CH2); HR MS (ESI) m/z: calcd for C16H13O2Se [M + H]+ 317.0075, found 317.0070.
General experimental procedure for the synthesis of 3-thiocyano chromones (5)
Enaminones 1 (0.2 mmol), potassium thiocyanate 4 (0.2 mmol, 19.4 mg), Selectfluor agent (0.2 mmol, 70.8 mg), and acetonitrile (2.0 mL) were added to a 10 mL reaction tube. The mixture was stirred at 40 °C for 2 h. After completion of the reaction, the solvent was distilled under vacuum. Then, the resulting mixture was dissolved with ethyl acetate (20 mL), washed with saturated sodium chloride solution (10 mL × 2). The organic phase was dried over anhydrous Na2SO4 and concentrated under vacuum. The residue was purified by silica gel column chromatography to give 3-thiocyano chromones 5 using ethyl acetate/petroleum ether as eluant.
3-Thiocyanato-4H-chromen-4-one (5a). Light yellow crystal, mp 139–140 °C (lit.29d 149–150 °C); IR (KBr) ν (cm−1): 1651, 1550, 1457, 1363, 1204, 1086; 1H NMR (400 MHz, CDCl3) δ: 8.34 (s, 1H), 8.25 (dd, JH–H = 8.0 Hz, JH–H = 1.5 Hz, 1H), 7.80–7.76 (m, 1H), 7.56–7.50 (m, 2H); 13C NMR (100 MHz, CDCl3) δ: 173.0 (C
O), 156.3, 155.4 (CH), 135.0 (CH), 126.6 (CH), 126.1 (CH), 122.6, 118.4 (CH), 112.5, 108.9; HR MS (ESI) m/z: calcd for C10H6NO2S [M + H]+ 204.0114, found 204.0109.
6-Methyl-3-thiocyanato-4H-chromen-4-one (5b). Light yellow crystal, mp 124–125 °C (lit.29e 134–135 °C); IR (KBr) ν (cm−1): 3070, 1648, 1559, 1479, 1331, 1147, 1125; 1H NMR (400 MHz, CDCl3) δ: 8.31 (s, 1H), 8.02 (d, JH–H = 1.1 Hz, 1H), 7.58 (dd, JH–H = 8.6 Hz, JH–H = 2.0 Hz, 1H), 7.44 (d, JH–H = 8.6 Hz, 1H), 2.48 (s, 3H); 13C NMR (100 MHz, CDCl3) δ: 173.0 (C
O), 155.3 (CH), 154.6, 136.9, 136.2 (CH), 125.3 (CH), 122.3, 118.1 (CH), 112.2, 109.0, 21.0 (CH3); HR MS (ESI) m/z: calcd for C11H8NO2S [M + H]+ 218.0270, found 218.0266.
7-Methyl-3-thiocyanato-4H-chromen-4-one (5c). Colorless crystal, mp 135–136 °C; IR (KBr) ν (cm−1): 2922, 1608, 1436, 1245, 1211, 1199, 1092; 1H NMR (400 MHz, CDCl3) δ: 8.18 (d, JH–H = 8.9 Hz, 1H), 8.07 (d, JF–H = 3.2 Hz, 1H), 7.02 (dd, JH–H = 8.9 Hz, JH–H = 2.2 Hz, 1H), 6.86 (d, JF–H = 2.2 Hz, 1H), 3.91 (s, 3H); 13C NMR (100 MHz, CDCl3) δ: 164.4 (C
O), 157.7, 150.6, 148.1, 142.5, 142.1, 127.3, 127.2, 114.9, 100.3, 55.9 (CH3); HR MS (ESI) m/z: calcd for C11H8NO2S [M + H]+ 218.0270, found 218.0267.
7-Methoxy-3-thiocyanato-4H-chromen-4-one (5d). Light yellow crystal, mp 157–158 °C (lit.29e 159–160 °C); IR (KBr) ν (cm−1): 2923, 2162, 1729, 1614, 1433, 1375, 1276, 1241, 1081; 1H NMR (400 MHz, CDCl3) δ: 8.23 (s, 1H), 8.13 (d, JH–H = 8.9 Hz, 1H), 7.05 (dd, JH–H = 8.9 Hz, JH–H = 2.4 Hz, 1H), 6.89 (d, JF–H = 2.4 Hz, 1H), 3.93 (s, 3H); 13C NMR (100 MHz, CDCl3) δ: 172.2 (C
O), 165.0, 158.2, 154.5 (CH), 127.4 (CH), 116.3, 115.9 (CH), 112.7, 109.1, 100.5 (CH), 56.0 (CH3); HR MS (ESI) m/z: calcd for C11H8NO3S [M + H]+ 234.0219, found 234.0212.
7-Fluoro-3-thiocyanato-4H-chromen-4-one (5e)29c. Colorless crystal, mp 120–121 °C; IR (KBr) ν (cm−1): 1638, 1439, 1375, 1234, 1141; 1H NMR (400 MHz, CDCl3) δ: 8.31 (s, 1H), 8.30–8.26 (m, 1H), 7.28–7.22 (m, 2H); 13C NMR (100 MHz, CDCl3) δ: 172.0 (C
O), 166.2 (d, JF–C = 256.7 Hz), 157.3 (d, JF–C = 13.2 Hz), 155.1 (CH), 128.8 (d, JF–C = 10.8 Hz, CH), 119.5 (d, JF–C = 2.3 Hz), 115.6 (d, JF–C = 22.7 Hz, CH), 113.2, 108.6, 105.2 (d, JF–C = 25.6 Hz, CH); 19F NMR (376 MHz, CDCl3) δ: −99.5; HR MS (ESI) m/z: calcd for C10H5FNO2S [M + H]+ 222.0020, found 222.0014.
7-Chloro-3-thiocyanato-4H-chromen-4-one (5f). Colorless crystal, mp 165–166 °C (lit.29f 176–178 °C); IR (KBr) ν (cm−1): 2158, 1634, 1607, 1423, 1347, 1163, 1066; 1H NMR (400 MHz, CDCl3) δ: 8.30 (s, 1H), 8.19 (d, JH–H = 8.6 Hz, 1H), 7.52 (d, JH–H = 1.8 Hz, 1H), 7.48 (d, JH–H = 8.6 Hz, JH–H = 1.8 Hz, 1H); 13C NMR (100 MHz, CDCl3) δ: 172.2 (C
O), 156.3 (CH), 155.0, 141.3 (CH), 127.5, 127.4 (CH), 121.1, 118.5 (CH), 113.3, 108.5; HR MS (ESI) m/z: calcd for C10H5ClNO2S [M + H]+ 237.9724, found 237.9720.
6-Chloro-3-thiocyanato-4H-chromen-4-one (5g). Colorless crystal, mp 137–138 °C (lit.29d 136–137 °C); IR (KBr) ν (cm−1): 2160, 1647, 1605, 1556, 1462, 1301, 1093, 836; 1H NMR (400 MHz, CDCl3) δ: 8.33 (s, 1H), 8.20 (d, JH–H = 2.5 Hz, 1H), 7.72 (d, JH–H = 9.0 Hz, JH–H = 2.5 Hz, 1H), 7.52 (d, JH–H = 9.0 Hz, 1H); 13C NMR (100 MHz, CDCl3) δ: 171.9 (C
O), 155.3 (CH), 154.6, 135.2 (CH), 132.7, 125.4 (CH), 123.5, 120.1 (CH), 112.9, 108.5; HR MS (ESI) m/z: calcd for C10H5ClNO2S [M + H]+ 237.9724, found 237.9720.
6-Bromo-3-thiocyanato-4H-chromen-4-one (5h). Light yellow crystal, mp 180–181 °C (lit.29f 189–191 °C); IR (KBr) ν (cm−1): 2919, 1648, 1458, 1377, 1299, 1019; 1H NMR (400 MHz, DMSO-d6) δ: 9.06 (s, 1H), 8.20 (d, JH–H = 2.4 Hz, 1H), 8.07 (dd, JH–H = 9.0 Hz, JH–H = 2.4 Hz, 1H), 7.76 (d, JH–H = 9.0 Hz, 1H); 13C NMR (100 MHz, DMSO-d6) δ: 171.9 (C
O), 161.5, 155.3, 138.3, 127.8, 124.6, 121.9, 119.5, 110.9, 110.8; HR MS (ESI) m/z: calcd for C10H5BrNO2S [M + H]+ 281.9219, found 281.9215.
6,8-Dichloro-3-thiocyanato-4H-chromen-4-one (5j). Colorless crystal, mp 123–124 °C; IR (KBr) ν (cm−1): 2166, 1630, 1555, 1444, 1309, 1108, 876; 1H NMR (400 MHz, CDCl3) δ: 8.39 (s, 1H), 8.31 (d, JH–H = 2.5 Hz, 1H), 7.82 (d, JH–H = 2.5 Hz, 1H); 13C NMR (100 MHz, CDCl3) δ: 171.4 (C
O), 154.7 (CH), 150.7, 135.1 (CH), 132.5, 124.9, 124.2, 124.1 (CH), 113.8, 108.0; HR MS (ESI) m/z: calcd for C10H4Cl2NO2S [M + H]+ 271.9334, found 271.9330.
6,8-Dibromo-3-thiocyanato-4H-chromen-4-one (5k). Colorless crystal, mp 139–140 °C; IR (KBr) ν (cm−1): 2163, 1630, 1589, 1541, 1449, 1431, 1305, 1092, 775; 1H NMR (400 MHz, CDCl3) δ: 8.41 (s, 1H), 8.31 (d, JH–H = 2.2 Hz, 1H), 8.13 (d, JH–H = 2.2 Hz, 1H); 13C NMR (100 MHz, CDCl3) δ: 171.3 (C
O), 154.9 (CH), 152.0, 140.8 (CH), 127.9 (CH), 124.4, 120.1, 113.6, 113.2, 108.0; HR MS (ESI) m/z: calcd for C10H4Br2NO2S [M + H]+ 359.8324, found 359.8317.
Synthesis of 3-((trifluoromethyl)thio)-4H-chromen-4-one (6a). A mixture of 3-thiocyanato-4H-chromen-4-one 5a (0.3 mmol, 60.9 mg), trimethyl(trifluoromethyl)silane (TMSCF3, 0.45 mmol, 63.9 mg), CuCl2 (0.06 mmol, 8.0 mg), Cs2CO3 (0.3 mmol, 97.8 mg) and DMF (5.0 mL) was added to a 25 mL reaction tube. The mixture was stirred at room temperature. After completion of the reaction, the solvent was distilled under vacuum. Then, the resulting mixture was dissolved with ethyl acetate (20 mL), washed with saturated sodium chloride solution (10 mL × 2). The organic phase was dried over anhydrous Na2SO4 and concentrated under vacuum. The residue was purified by silica gel column chromatography to give 3-((trifluoromethyl)thio)-4H-chromen-4-one 6a using ethyl acetate/petroleum ether as eluant.Colorless crystal, mp 120–121 °C (lit.31 122–123 °C); 1H NMR (400 MHz, CDCl3) δ: 8.39 (s, 1H), 8.29 (dd, JH–H = 7.8 Hz, JH–H = 1.3 Hz, 1H), 7.77–7.73 (m, 1H), 7.52–7.48 (m, 2H); 13C NMR (100 MHz, CDCl3) δ: 174.3 (C
O), 162.3, 156.2, 134.6, 138.9 (q, JF–C = 306.2 Hz), 126.7, 126.5, 123.9, 118.2, 111.3; 19F NMR (376 MHz, CDCl3) δ: −42.8; HR MS (ESI) m/z: calcd for C10H6F3O2S [M + H]+ 247.0035, found 247.0036.
Conflicts of interest
There are no conflicts to declare.
Acknowledgements
This work was supported by the Fundamental Research Funds for the Henan Provincial Colleges and Universities in Henan University of Technology (No. 2017RCJH08).
Notes and references
-
(a) Y. S. Jin, Recent advances in natural antifungal flavonoids and their derivatives, Bioorg. Med. Chem. Lett., 2019, 29, 126589 CrossRef CAS PubMed;
(b) M. P. Beller and U. Koert, Synthetic studies on chromone natural products: the preussochromones, Synthesis, 2022, 54, 2778–2786 CrossRef CAS;
(c) K. S. Masters and S. Bräse, Xanthones from fungi, lichens, and bacteria: the natural products and their synthesis, Chem. Rev., 2012, 112, 3717–3776 CrossRef CAS PubMed;
(d) S. K. Sharma, S. Kumar, K. Chand, A. Kathuria, A. Gupta and R. Jain, An update on natural occurrence and biological activity of chromones, Curr. Med. Chem., 2011, 18, 3825–3852 CrossRef CAS PubMed.
-
(a) T. Y. Wang, Q. Li and K. S. Bi, Bioactive flavonoids in medicinal plants: structure, activity and biological fate, Asian J. Pharm. Sci., 2018, 13, 12–23 CrossRef PubMed;
(b) R. S. Keri, S. Budagumpi, R. K. Pai and R. G. Balakrishna, Chromones as a privileged scaffold in drug discovery: a review, Eur. J. Med. Chem., 2014, 78, 340–374 CrossRef CAS PubMed;
(c) A. Gaspar, M. J. Matos, J. Garrido, E. Uriarte and F. Borges, Chromone: a valid scaffold in medicinal chemistry, Chem. Rev., 2014, 114, 4960–4992 CrossRef CAS PubMed;
(d) T. E. Ali, M. A. Assiri, A. A. Shati, M. Y. Alfaifi and S. E. I. Elbehairi, One-pot three-component synthesis of a series of 2-amino-4-(4-oxo-4H-chromen-3-yl)-5-(2,2,2-trifluoroacetyl)-6-(trifluoromethyl)-4H-pyrans and 2-amino-4-(4-oxo-4H-chromen-3-yl)-5-(thiophene-2-carbonyl)-6-(trifluoromethyl)-4H-pyrans as promising anticancer agents, Russ. J. Org. Chem., 2022, 58, 584–591 CrossRef CAS.
-
(a) M. Zhang, Y. Gong, Y. Zhou, Y. Zhou and X. L. Liu, Recent advances of chromone-based reactants in the catalytic asymmetric domino annulation reaction, Org. Chem. Front., 2021, 8, 3968–3989 RSC;
(b) S. W. Ng, L. H. Chung, C. F. Yeung, H. S. Lo, H. L. Shek, T. S. Kang, C. H. Leung, D. L. Ma and C. Y. Wong, Metalated chromene and chromone complexes: pH switchable metal–carbon bonding interaction, photo-triggerable chromone delivery application, and antioxidative activity, Chem. – Eur. J., 2018, 24, 1779–1783 CrossRef CAS PubMed;
(c) N. Yadav, R. Kumar, A. K. Singh, S. Mohiyuddin and P. Gopinath, Systematic approach of chromone skeleton for detecting Mg2+, ion: applications for sustainable cytotoxicity and cell imaging possibilities, Spectrochim. Acta, Part A, 2020, 235, 118290 CrossRef CAS PubMed;
(d) L. Fan, J. C. Qin, T. R. Li, B. D. Wang and Z. Y. Yang, A novel rhodamine chromone-based “Off-On” chemosensor for the differential detection of Al(III) and Zn(II) in aqueous solutions, Sens. Actuators, B, 2014, 203, 550–556 CrossRef CAS.
-
(a) H. H. Lee, J. S. Shin, W. S. Lee, B. Ryu, D. S. Jang and K. T. Lee, Biflorin, isolated from the flower buds of Syzygium aromaticum L., suppresses LPS-induced inflammatory mediators via STAT1 inactivation in macrophages and protects mice from endotoxin shock, J. Nat. Prod., 2016, 79, 711–720 CrossRef CAS PubMed;
(b) C. F. M. Silva, D. C. G. A. Pinto and A. M. S. Silva, Chromones: a promising ring system for new anti-inflammatory drugs, ChemMedChem, 2016, 11, 2252–2260 CrossRef CAS PubMed;
(c) Y. Chen, Y. Xu, H. Zhang, J. Yin, X. Fan, D. Liu, H. Fu and B. Wan, Emodin alleviates jejunum injury in rats with sepsis by inhibiting inflammation response, Biomed. Pharmacother., 2016, 84, 1001–1007 CrossRef CAS PubMed.
- Q. Sun and G. Chou, Isoflavonoids from Crotalaria albida inhibit adipocyte differentiation and lipid accumulation in 3T3-L1 cells via suppression of PPAR-γ pathway, PLoS One, 2015, 10, e0135893 CrossRef PubMed.
-
(a) S. Martens and A. Mithöfer, Flavones and flavone synthases, Phytochemistry, 2005, 66, 2399–2407 CrossRef CAS PubMed;
(b) S. Tong, C. Chu, Y. Wei, L. Wang, X. Gao, X. Xu and J. Yu, Preparation and effects of 2,3-dehydrosilymarin, a promising and potent antioxidant and free radical scavenger, J. Pharm. Pharmacol., 2011, 63, 238–244 CrossRef CAS PubMed.
- S. Lin, J. J. Koh, T. T. Aung, W. L. W. Sin, L. Wang, R. Lakshminarayanan, L. Zhou, H. Tan, D. Cao, R. W. Beuerman, L. Ren and S. Liu, Semisynthetic flavone-derived antimicrobials with therapeutic potential against methicillin-resistant Staphylococcus aureus (MRSA), J. Med. Chem., 2017, 60, 6152–6165 CrossRef CAS PubMed.
- S. Gobbi, A. Rampa, A. Bisi, F. Belluti, L. Piazzi, P. Valenti, A. Caputo, A. Zampiron and M. Carrara, Synthesis and biological evaluation of 3-alkoxy analogues of flavone-8-acetic acid, J. Med. Chem., 2003, 46, 3662–3669 CrossRef CAS PubMed.
-
(a) G. H. Yan, X. F. Li, B. C. Ge, X. D. Shi, Y. F. Chen, X. M. Yang, J. P. Xu, S. W. Liu, P. L. Zhao, Z. Z. Zhou, C. Q. Zhou and W. H. Chen, Synthesis and anticancer activities of 3-arylflavone-8-acetic acid derivatives, Eur. J. Med. Chem., 2015, 90, 251–257 CrossRef CAS PubMed;
(b) S. Mei, H. Ma and X. Chen, Anticancer and anti-inflammatory properties of mangiferin: a review of its molecular mechanisms, Food Chem. Toxicol., 2021, 149, 111997–112010 CrossRef CAS PubMed.
- For selected examples, see:
(a) T. Guo, L. Bi, M. Zhang, C. J. Zhu, L. B. Yuan and Y. H. Zhao, Access to sulfur-containing bisheterocycles through base-promoted consecutive tandem cyclization/sulfenylation with elemental sulfur, J. Org. Chem., 2022, 87, 16907–16912 CrossRef CAS PubMed;
(b) T. Luo, J. P. Wan and Y. Y. Liu, Toward C2-nitrogenated chromones by copper-catalyzed β-C(sp2)–H N-heteroarylation of enaminones, Org. Chem. Front., 2020, 7, 1107–1112 RSC;
(c) P. N. Bagle, M. V. Mane, S. P. Sancheti, A. B. Gade, S. R. Shaikh, M. H. Baik and N. T. Patil, Gold(I)-catalyzed hydroxy group assisted C(sp2)–H alkylation of enaminones with diazo compounds to access 3-alkyl chromones, Org. Lett., 2019, 21, 335–339 CrossRef CAS PubMed;
(d) H. Xiang, Q. Zhao, Z. Tang, J. Xiao, P. Xia, C. Wang, C. Yang, X. Chen and H. Yang, Visible-light-driven, Radical-triggered tandem cyclization of o-hydroxyaryl enaminones: Facile access to 3-CF2/CF3-containing chromones, Org. Lett., 2017, 19, 146–149 CrossRef CAS PubMed;
(e) S. Mkrtchyan and V. O. Iaroshenko, Visible-light-mediated arylation of ortho-hydroxyarylenaminones: Direct access to isoflavones, Chem. Commun., 2020, 56, 2606–2609 RSC;
(f) T. Luo, H. Wu, L. H. Liao, J. P. Wan and Y. Liu, Synthesis of 3,3-dihalogenated 2-aminochromanones via tandem dihalogenation and cyclization of o-hydroxyarylenaminones with NXS (X = Cl or Br), J. Org. Chem., 2021, 86, 15785–15791 CrossRef CAS PubMed;
(g) L. Fu, Z. Xu, J. P. Wan and Y. Liu, The domino chromone annulation and a transient halogenation-mediated C–H alkenylation toward 3-vinyl chromones, Org. Lett., 2020, 22, 9518–9523 CrossRef CAS PubMed;
(h) Y. Guo, Y. Xiang, L. Wei and J. P. Wan, Thermoinduced free-radical C–H acyloxylation of tertiary enaminones: Catalyst-free synthesis of acyloxyl chromones and enaminones, Org. Lett., 2018, 20, 3971–3974 CrossRef CAS PubMed.
-
(a) Z. Chen, H. Lai, L. Hou and T. Chen, Rational design and action mechanisms of chemically innovative organoselenium in cancer therapy, Chem. Commun., 2020, 56, 179–196 RSC;
(b) Q. Miao, J. Xu, A. Lin, X. Wu, L. Wu and W. Xie, Recent advances for the synthesis of selenium-containing small molecules as potent antitumor agents, Curr. Med. Chem., 2018, 25, 2009–2033 CrossRef CAS PubMed;
(c) L. Kursvietiene, A. Mongirdiene, J. Bernatoniene, J. Sulinskiene and I. Staneviciene, selenium anticancer properties and impact on cellular redox status, Antiox, 2020, 9, 80–90 CrossRef CAS PubMed.
-
(a) E. E. Frieben, S. Amin and A. K. Sharma, Development of isoselenocyanate compounds' syntheses and biological applications, J. Med. Chem., 2019, 62, 5261–5275 CrossRef CAS PubMed;
(b) M. Fourmigue and A. Dhaka, Chalcogen bonding in crystalline diselenides and selenocyanates: From molecules of pharmaceutical interest to conducting materials, Coord. Chem. Rev., 2020, 403, 213084–213100 CrossRef.
-
(a) J. Vahter, K. Viht, A. Uri, G. B. Manoharan and E. Enkvist, Thiazole-and selenazole-comprising high-affinity inhibitors possess bright microsecond-scale photoluminescence in complex with protein kinase CK2, Bioorg. Med. Chem., 2018, 26, 5062–5068 CrossRef CAS PubMed;
(b) M. Tian, Y. Yang, F. W. Avila, T. Fish, H. Yuan, M. Hui, T. W. Thannhauser, L. Li, M. Tian and S. Pan, Effects of selenium supplementation on glucosinolate biosynthesis in broccoli, J. Agric. Food Chem., 2018, 66, 8036–8044 CrossRef CAS PubMed;
(c) A. Csonka, A. Kincses, M. Nove, Z. Vadas, G. Spengler, A. Csonka, C. Sanmartin, C. Sanmartin and E. Dominguez-Alvarez, Selenoesters and selenoanhydrides as novel agents against resistant breast cancer, Anticancer Res., 2019, 39, 3777–3783 CrossRef CAS PubMed.
-
(a) X. Meng, P. Zhong, Y. Wang, H. Wang, H. Tang and Y. Pan, Electrochemical difunctionalization of olefines: Access to selenomethyl-substituted cyclic rthers or lactones, Adv. Synth. Catal., 2020, 362, 506–511 CrossRef CAS;
(b) S. F. Fonseca, N. B. Padilha, S. Thurow, J. A. Roehrs, L. Savegnago, M. N. de Souza, M. G. Fronza, T. Collares, J. Buss, F. K. Seixas, D. A. Eder and J. Lenardão, Ultrasound-promoted copper-catalyzed synthesis of bis-arylselanyl chrysin derivatives with boosted antioxidant and anticancer activities, Ultrason. Sonochem., 2017, 39, 827–836 CrossRef CAS PubMed.
-
(a) C. Feng, J. Zhu, Q. Tang and A. Zhou, Synthesis of ArSe-substituted flavone derivatives using Se powder, Chin. J. Org. Chem., 2019, 39, 1187–1192 CrossRef CAS;
(b) P. Xu, Z. Zhong, H. Huang and A. Zhou, Selenation of 2-hydroxyphenyl enaminones with Se powder to generate ArSe-subsituted chromone derivatives, ChemistrySelect, 2022, 7, e202202854 CrossRef CAS.
-
(a) J. Zhu, B. Xu, J. Yu, Y. Ren, J. Wang, P. Xie, C. U. Pittman and A. Zhou, Copper-catalyzed generation of flavone selenide and thioether derivatives using KSeCN and KSCN via C–H functionalization, Org. Biomol. Chem., 2018, 16, 5999–6005 RSC;
(b) C. Ding, Y. Yu, Q. Yu, Z. Xie, Y. Zhou, J. Zhou, G. Liang and Z. Song, NIS/TBHP induced regioselective selenation of (hetero)arenes via direct C–H functionalization, ChemCatChem, 2018, 10, 5397–5401 CrossRef CAS.
-
(a) L. Fu and J. P. Wan, C3-functionalized chromones synthesis by tandem C–H elaboration and chromone annulation of enaminones, Asian J. Org. Chem., 2019, 8, 767–776 CrossRef CAS;
(b) X. Y. Chen, X. Zhang and J. P. Wan, Recent advances in transition metal-free annulation toward heterocycle diversity based on the C–N bond cleavage of enaminone platform, Org. Biomol. Chem., 2022, 20, 2356–2369 RSC.
- For selected examples, see:
(a) T. Zhang, W. Yao, J. P. Wan and Y. Liu, Transition-metal-free C(sp2)-H dithiocarbamation and chromone annulation cascade for 3-dithiocarbamyl chromone synthesis, Adv. Synth. Catal., 2021, 363, 4811–4816 CrossRef CAS;
(b) G. S. Sorabad and M. R. Maddani, Metal-free, facile synthesis of sulfenylated chromones and indoles promoted by an aqueous HBr-DMSO system, Asian J. Org. Chem., 2019, 8, 1336–1343 CrossRef CAS;
(c) H. Xiang and C. Yang, A facile and general approach to 3-((trifluoromethyl)thio)-4H-chromen-4-one, Org. Lett., 2014, 16, 5686–5689 CrossRef CAS;
(d) J. P. Wan, Z. Tu and Y. Wang, Transient and recyclable halogenation coupling (TRHC) for isoflavonoid synthesis with site-selective arylation, Chem. - Eur. J., 2019, 25, 6907–6910 CrossRef CAS PubMed;
(e) Y. Lin, J. P. Wan and Y. Liu, Cascade in Situ iodination, chromone annulation, and cyanation for site-selective synthesis of 2-cyanochromones, J. Org. Chem., 2023, 88, 4017–4023 CrossRef CAS PubMed.
- J. Joussot, A. Schoenfelder, L. Larquetoux, M. Nicolas, J. Suffert and G. Blond, Synthesis of 3-substituted chromones and quinolones from enaminones, Synthesis, 2016, 48, 3364–3372 CrossRef CAS.
-
(a) J. Rafique, S. Saba, A. R. Schneider, M. S. Franco, S. M. Silva and A. L. Braga, Metal- and solvent-free approach to access 3-Se/S-chromones from the cyclization of enaminones in the presence of dichalcogenides catalyzed by KIO3, ACS Omega, 2017, 2, 2280–2290 CrossRef CAS PubMed;
(b) S. Zhong, Y. Liu, X. Cao and J. P. Wan, KIO3-catalyzed domino C(sp2)–H bond sulfenylation and C–N bond oxygenation of enaminones toward the synthesis of 3-sulfenylated chromones, ChemCatChem, 2017, 9, 465–468 CrossRef CAS.
- Selective examples:
(a) N. A. Romero and D. A. Nicewicz, Organic photoredox catalysis, Chem. Rev., 2016, 116, 10075–10166 CrossRef CAS PubMed;
(b) K. L. Skubi, T. R. Blum and T. P. Yoon, Dual catalysis strategies in photochemical synthesis, Chem. Rev., 2016, 116, 10035–10074 CrossRef CAS PubMed;
(c) D. A. Nicewicz and D. W. C. MacMillan, Merging photoredox catalysis with organocatalysis: the direct asymmetric alkylation of aldehydes, Science, 2008, 322, 77–80 CrossRef CAS PubMed;
(d) J. Xuan and W. J. Xiao, Visible-light photoredox catalysis, Angew. Chem., Int. Ed., 2012, 51, 6828–6838 CrossRef CAS PubMed;
(e) T. P. Yoon, M. A. Ischay and J. Du, Visible light photocatalysis as a greener approach to photochemical synthesis, Nat. Chem., 2010, 2, 527–532 CrossRef CAS PubMed;
(f) L. Shi and W. Xia, Photoredox functionalization of C–H bonds adjacent to a nitrogen atom, Chem. Soc. Rev., 2012, 41, 7687–7697 RSC.
- H. Y. Liu, J. R. Zhang, G. B. Huang, Y. H. Zhou, Y. Y. Chen and Y. L. Xu, Visible light-promoted selenylation/cyclization of enaminones toward the formation of 3-selanyl-4H-chromen-4-ones, Adv. Synth. Catal., 2021, 363, 1656–1661 CrossRef CAS.
- C. V. Doerner, J. S. S. Neto, C. R. Cabreira, S. Saba, L. P. Sandjo, J. Rafique, A. L. Braga and F. F. de Assis, Synthesis of 3-selanyl-isoflavones from 2-hydroxyphenyl enaminones using trichloroisocyanuric acid (TCCA): a sustainable approach, New J. Chem., 2023, 47, 5598–5602 RSC.
-
(a) Y. L. Ban, L. You, K. W. Feng, F. C. Ma, X. L. Jin and Q. Liu, Meyer-Schuster-Type rearrangement of propargylic alcohols into α-selenoenals and-enones with diselenides, J. Org. Chem., 2021, 86, 5274–5283 CrossRef CAS PubMed;
(b) L. Y. Xie, J. Qu, S. Peng, K. J. Liu, Z. Wang, M. H. Ding, Y. Wang, Z. Cao and W. M. He, Selectfluor-mediated regioselective nucleophilic functionalization of N-heterocycles under metal- and base-free conditions, Green Chem., 2018, 20, 760–764 RSC;
(c) P. Xu, S. Guo, L. Wang and P. Tang, Silver-catalyzed oxidative activation of benzylic C–H bonds for the synthesis of difluoromethylated arenes, Angew. Chem., Int. Ed., 2014, 53, 5955–5958 CrossRef CAS PubMed;
(d) J. L. Li, E. Lin, X. L. Han, Q. Li and H. Wang, Synthesis of α-fluorinated imides via direct fluorohydroxylation of ynamides, Org. Lett., 2019, 21, 4255–4258 CrossRef CAS PubMed;
(e) P. Liu, Y. Gao, W. Gu, Z. Shen and P. Sun, Regioselective fluorination of imidazo[1,2-a]pyridines with selectfluor in aqueous condition, J. Org. Chem., 2015, 80, 11559–11565 CrossRef CAS.
- Selective examples:
(a) L. Niu, J. Liu, X. A. Liang, S. Wang and A. Lei, Visible light-induced direct α C–H functionalization of alcohols, Nat. Commun., 2019, 10, 467 CrossRef;
(b) H. Fei, Z. Xu, H. Wu, L. Zhu, H. B. Jalani, G. Li, Y. Fu and H. Lu, Stereospecific electrophilic fluorocyclization of α,β-unsaturated amides with Selectfluor, Org. Lett., 2020, 22, 2651–2656 CrossRef CAS PubMed;
(c) J. D. Galloway, D. N. Mai and R. D. Baxter, Silver-catalyzed Minisci reactions using selectfluor as a mild oxidant, Org. Lett., 2017, 19, 5772–5775 CrossRef CAS PubMed;
(d) Y. Kong, X. Sun and J. Weng, Selectfluor as “fluorine-free” functional reagent applied to organic synthesis under transition metal-free conditions, Chin. J. Org. Chem., 2020, 40, 2641–2657 CrossRef CAS;
(e) X. Wang, Q. Wang, Y. Xue, K. Sun, L. Wu and B. Zhang, An organoselenium-catalyzed N1- and N2-selective aza-Wacker reaction of alkenes with benzotriazoles, Chem. Commun., 2020, 56, 4436–4439 RSC.
-
(a) J. W. Yuan, G. C. Huang, L. L. Wang, X. Y. Wang, L. R. Yang, S. R. Zhang, P. Mao, Y. M. Xiao and L. B. Qu, Chalcogenative spirocyclization of N-aryl propiolamides with diselenides/disulfides promoted by Selectfluor, Z. Naturforsch., 2022, 77b, 75–85 CrossRef;
(b) J. W. Yuan, Q. Chen, W. T. Wu, J. J. Zhao, L. R. Yang, Y. M. Xiao, P. Mao and L. B. Qu, Selectfluor-mediated construction of 3-arylselenenyl and 3, 4-bisarylselenenyl spiro[4.5]trienones via cascade annulation of N-phenylpropiolamides with diselenides, New J. Chem., 2022, 46, 9451–9460 RSC;
(c) W. P. Mai, J. W. Yuan, J. L. Zhu, Q. Q. Li, L. R. Yang, Y. M. Xiao, P. Mao and L. B. Qu, Selectfluor-mediated direct C–H phosphonation of quinoxalin-2(1H)-ones under base and transition-metal free conditions, ChemistrySelect, 2019, 4, 11066–11070 CrossRef CAS;
(d) J. Yuan, F. Zeng, W. Mai, L. Yang, Y. Xiao, P. Mao and D. Wei, Fluorination-triggered tandem cyclization of styrene-type carboxylic acids to access 3-aryl isocoumarin derivatives under microwave irradiation, Org. Biomol. Chem., 2019, 17, 5038–5046 RSC;
(e) J. W. Yuan, Y. Zhang, G. C. Huang, M. Y. Ma, T. Y. Yang, L. R. Yang, S. R. Zhang, P. Mao and L. B. Qu, Site-specific C–H chalcogenation of quinoxalin-2(1H)-ones enabled by Selectfluor reagent, Org. Chem. Front., 2021, 8, 6937–6949 RSC.
-
(a) R. J. Capon, C. Skene, E. H. T. Liu, E. Lacey, J. H. Gill, K. Heiland and T. Friedel, The isolation and synthesis of novel nematocidal dithiocyanates from an australian marine sponge, Oceanapia sp., J. Org. Chem., 2001, 66, 7765–7769 CrossRef CAS PubMed;
(b) S. Dutta, H. Abe, S. Aoyagi, C. Kibayashi and K. S. Gates, DNA damage by fasicularin, J. Am. Chem. Soc., 2005, 127, 15004–15005 CrossRef CAS PubMed;
(c) L. J. Dean and M. R. Prinsep, The chemistry and chemical ecology of nudibranchs, Nat. Prod. Rep., 2017, 34, 1359–1390 RSC.
-
(a) L. Zhen, K. Yuan, X. Li, C. Zhang, J. Yang, H. Fan and L. Jiang, Cascade reaction of propargyl amines with AgSCF3, as well as one-pot reaction of propargyl amines, AgSCF3, and di-tert-butyl peroxide: access to allenyl thiocyanates and allenyl trifluoromethylthioethers, Org. Lett., 2018, 20, 3109–3113 CrossRef CAS PubMed;
(b) W. L. Lei, T. Wang, K. W. Feng, L. Z. Wu and Q. Liu, Visible-light-driven synthesis of 4-alkyl/aryl-2-aminothiazoles promoted by in situ generated copper photocatalyst, ACS Catal., 2017, 7, 7941–7945 CrossRef CAS;
(c) B. Bayarmagnai, C. Matheis, K. Jouvin and L. J. Goossen, Synthesis of difluoromethyl thioethers from difluoromethyl trimethylsilane and organothiocyanates generated in situ, Angew. Chem., Int. Ed., 2015, 54, 5753–5756 CrossRef CAS PubMed.
-
(a) Y. Gao, Y. Liu and J. P. Wan, Visible light-induced thiocyanation of enaminone C–H bond to access polyfunctionalized alkenes and thiocyano chromones, J. Org. Chem., 2019, 84, 2243–2251 CrossRef CAS PubMed;
(b) Z. Yang, L. Hu, T. Cao, L. An, L. Li, T. Yang and C. Zhou, PIDA-mediated α-C–H functionalization of enaminones: the synthesis of thiocyano enaminones and chromones in water, New J. Chem., 2019, 43, 16441–16444 RSC;
(c) X. Z. Zhang, D. L. Ge, S. Y. Chen and X. Q. Yu, A catalyst-free approach to 3-thiocyanato-4 H-chromen-4-ones, RSC Adv., 2016, 6, 66320–66323 RSC;
(d) J. A. Xiao, X. L. Cheng, R. F. Meng, X. S. Qin, H. Peng, J. W. Ren, Z. Z. Xie, J. G. Cui and Y. M. Huang, Straightforward synthesis of 3-selenocyanato-substituted chromones through electrophilic selenocyanation of enaminones under grinding conditions, Synthesis, 2021, 53, 954–960 CrossRef CAS;
(e) Y. Gao, Y. Liu and J. P. Wan, Visible light-induced thiocyanation of enaminone C–H bond to access polyfunctionalized alkenes and thiocyano chromones, J. Org. Chem., 2019, 84, 2243–2251 CrossRef CAS PubMed;
(f) Z. Yang, Y. Wang, L. Hu, J. Yu, A. Li, L. Li, T. Yang and C. Zhou, Electrochemically induced thiocyanation of enaminones: synthesis of functionalized alkenes and chromones, Synthesis, 2020, 52, 711–718 CrossRef CAS.
- M. Jakubczyk, S. Mkrtchyan, I. D. Madura, P. H. Marek and V. O. Iaroshenko, Copper-catalyzed direct C-H arylselenation of 4-nitro-pyrazoles and other heterocycles with selenium powder and aryl iodides. Access to unsymmetrical heteroaryl selenides, RSC Adv., 2019, 9, 25368–25376 RSC.
- H. Xiang and C. Yang, A facile and general approach to 3-((trifluoromethyl)thio)-4H-chromen-4-one, Org. Lett., 2014, 16, 5686–5689 CrossRef CAS PubMed.
|
This journal is © The Royal Society of Chemistry 2023 |