DOI:
10.1039/D3RA05171D
(Paper)
RSC Adv., 2023,
13, 30420-30428
Facile synthesis and anion binding studies of fluorescein/benzo-12-crown-4 ether based bis-dipyrromethane (DPM) receptors†
Received
31st July 2023
, Accepted 10th October 2023
First published on 16th October 2023
Abstract
Two novel fluorescein as well as benzo-12-crown-4 ether functionalized dipyrromethane receptors (DPM3 and DPM4) have successfully been synthesized. The anion (used as their TBA salts) binding studies of thus prepared DPM3 and DPM4 receptors were evaluated by the UV-visible spectrophotometric titrations. Binding affinities as well as the stoichiometry were determined through the UV-visible titrations data with the involvement of the BindFit (v0.5) package available online at https://supramolecular.org. Moreover, binding events were validated by means of the comparison of the partial 1H-NMR spectrum of the simple host molecule with that of the host–guest complex, and the 1
:
1 stoichiometry were further confirmed by the Job's method of continuous variation. From the results, we observed the binding constant (Ka) values of DPM3/DPM4 with various tested anions in the range of 516.07 M−1 to 63789.81 M−1, depending upon the nature/shape/size of the anions. Moreover, the anion–π interactions were confirmed by the partial 1H-NMR spectral data, and further supported by the literature reported systems. The authors hope that such types of valued receptors will be benefitted in future for the recognizing/binding of a variety of biologically important anions.
Introduction
In the past decade, supramolecular chemistry has displayed a promising role by producing a myriad of sensitive as well as selective artificial receptors for recognizing/capturing of various neutral guest molecules or ionic entities (cations, anions and/or ion-pairs), which has enormously revolutionized the host–guest chemistry.1–5 Notably, scientists worldwide are developing the anion coordination chemistry by presenting a variety of synthetic receptors for the recognition, sensing, and/or extraction of the diverse biologically/environmentally significant polystructural anionic species.6,7 Although, anions are considered indispensable components for numerous biological systems, as many physiological processes are directly or indirectly controlled in the presence of anions of specific importance.8,9 However, decrease or increase in the concentrations of the anions from the human body or in the environment causes several diseases including Bartter's syndrome, Pendred's syndrome, cystic fibrosis, dent's disease, osteoporosis, and pollutants linked with the anionic entities are contamination of drinking water, carcinogenesis and eutrophication of rivers etc.10 In this regard, to date, though, a plethora of anionic receptors have fruitfully been developed, exhibiting selectively high affinity towards sensing or recognition of the anions by fabricating changes in the spectroscopic signals.11–19 But, we believe that, there is still a pressing necessity to develop simple-to-make yet highly sensitive and selective anion receptors.20–30
Gratifyingly, preorganization leads to the selective as well as effective binding between the specific receptor and analyte(s). Moreover, it also upturns the rigidity, which usually hinders the entree of analyte towards the binding site(s). This can produce undesirable interruption in the measurement of equilibrium, and also at the same time retard the kinetic measurements, hence the receptor rigidity and flexibility must be kept in a proper balance, while designing the receptor(s) of particular interest. Noticeably, a central requirement for a chemosensors functioning is the reversible binding of receptor to analyte – allowing the concentration of the analyte to be measured at the equilibrium through analytically useful signals. Therefore, while scheming the supramolecular receptors, certain requirements must be kept in mind: (1) choice of chromophore/fluorophore; (2) analyte binding site(s); (3) immobilized methods and optical signaling mechanism; (4) preorganization characteristics; (5) lastly, the composed flexibility as well as the rigidity.28,31,32
Undoubtedly, the design and construction of anion receptors is highly challenging because of: (1) diverse geometries/shapes of the anions; (2) their large size-to-charge ratio; (3) and the higher hydration/solvation energies of the anions as compared to the cationic receptors. Generally, a combination of non-covalent/supramolecular interactions participates in the anion recognition chemistry, including coulombic interactions, halogen bonding, hydrogen bonding, CH–anion, anion–π interactions, π–π stacking, and other important non-covalent forces, as well.33–46 However, most frequent moieties consisting of the active hydrogen bonding sites for instance urea, thiourea, phenol, amide, pyrrole etc., have prominently been used for the design and synthesis of the anion receptors.47–52 Remarkably, as can be inspected from the Fig. 1, by utilizing the hydrogen bonding sites of pyrrolic systems, Sessler's research group has reported that receptors 8 & 12, displayed high affinity towards the biologically important dihydrogenphosphate and pyrophosphate anions (used as their tetrabutylammonium salts) in chloroform solvent.53–55 On the other hand, the same research group has also revealed the selective binding of tetrabutylammonium dihydrogen phosphate over other tested anionic salts with the pyrene-functionalized tetrakis-(1H-pyrrole-2-carbaldehyde) (9) in CHCl3.56 Similarly, Bao and co-workers have reported two novel tweezer-like receptors, namely, the 2,2′-bis(2-cyano-2-phenylvinyl)-5,5′-dimethyl dipyrromethene (10) and 2,2′-bis[2-cyano-2-(4-nitrophenyl)vinyl]-5,5′-dimethyl dipyrromethane (11), displaying strong and selective binding towards dihydrogenphosphate and fluoride anions (Fig. 1).57 In a separate report, Zuo and teammates have described a selective optical and electrochemical sensing of the fluoride anion with the usage of tetrathiafulvalene (TTF) based boron-dipyrromethene receptor through 1H-NMR titration, which was further supported by virtue of the Density Functional Theory (DFT) calculations.58 On the other occasion, Sessler's team has also achieved worthy outcomes with bipyrrole and dipyrromethane appended amido-imine hybrid macrocycles, toward oxoanions in acetonitrile.59 On the other hand, Ustynyuk's group has reported impactful results of anion binding studies using polypyrrolic 2,5-diamidothiophene Schiff base with 1.2
:
1 hydrogen sulfate anions versus nitrate selectivity in flexible dipyrromethane, whereas rigid congener displayed 7.4
:
1 selectivity towards hydrogen sulfate.60
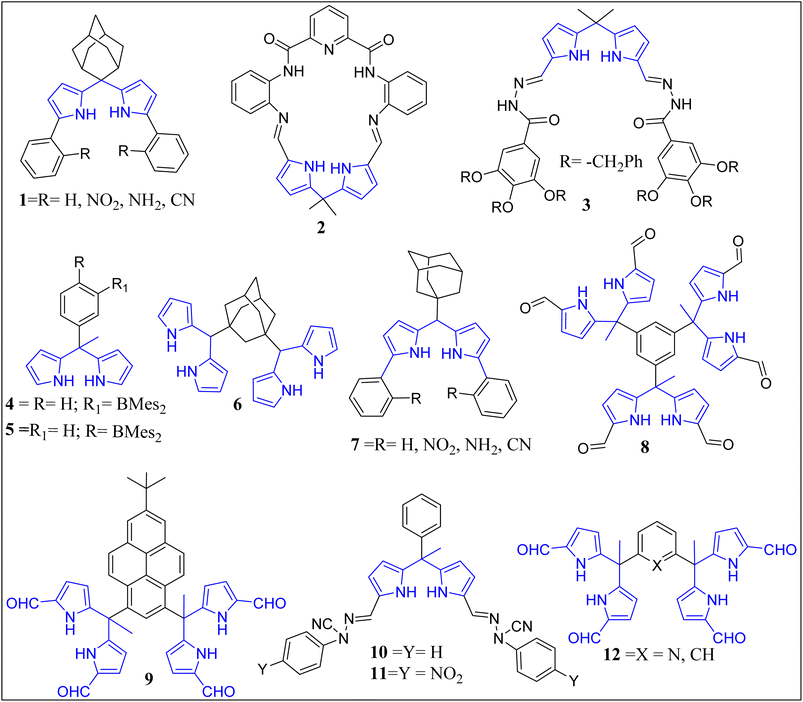 |
| Fig. 1 A list of already reported DPMs by different research groups for anion binding. | |
Fluorescein and its congeners have a potential future as fluorescent probes for diverse analytes as well as bio-labeling reagents. Additionally, exhibiting outstanding fluorescence quantum yields and other photophysical characteristics, such as the capacity to emit and absorb light at extended wavelengths and have great light stability.61–65 On the other hand, crown ethers, cyclic compounds containing various ether groups, displaying strong binding towards cations by forming complexes. Crown ethers exhibiting extinction coefficients and are mostly used for phase transfer catalysis, synthesis of ion/ion pair receptors, biological/pharmacological applications, and mechanically interlocked supramolecular systems.66–69 Therefore, bearing the importance of fluorescein, crown ethers and dipyrromethenes (DPMs) containing receptors in mind, in addition to further extend our work on the DPM-based systems,70 herewith we envision to construct two novel dipyrromethane receptors (DPM3 and DPM4), to investigate their anion binding affinities. We are in the opinion that these newly developed receptors, may open several hidden opportunities for the young researchers, to further advanced the arena of molecular recognition/sensing in many years to come.
Results and discussion
Synthesis and characterization
Towards the construction of our targeted dipyrromethane receptors (DPM3 and DPM4), we embarked with the preparation of DPM1 through the condensation reaction of easily available pyrrole (13) and p-hydroxyacetophenone (14) under a low melting deep eutectic mixture, using our earlier reported protocol (Scheme 1).70 Later, the DPM1 was converted into the long-tailed ether based DPM2 in good yield (69%) by refluxing it with 1,2-dibromoethane (15) in the presence of K2CO3/MeCN for overnight. Next, DPM2 was treated with the parent fluorescein (16) using K2CO3 in refluxing acetonitrile to furnish the desired DPM3.
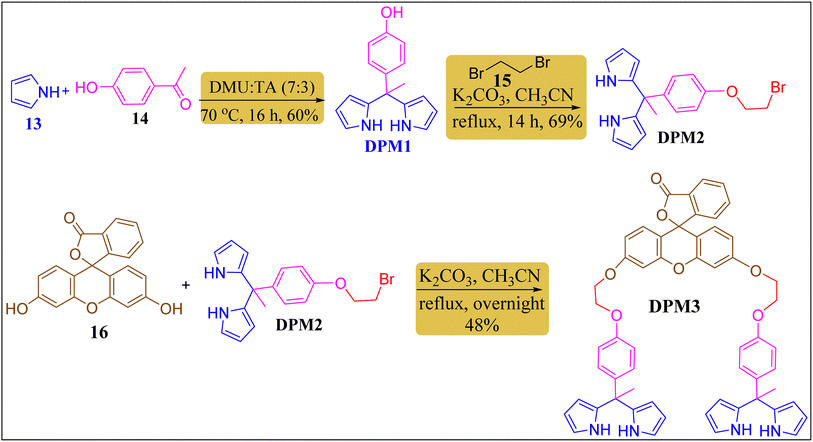 |
| Scheme 1 Synthesis of the fluorescein-based bis-dipyrromethane DPM3. | |
On the other hand, synthesis of the DPM4 was achieved from the commercially available tri-ethyleneglycol (17), p-toluenesolfunyl chloride (18), and the catechol (20). In this regard, first we prepared the ditosylated compound (19) by reacting 17 with 18 using KOH in dichloromethane (DCM), which was then treated with catechol (20) in the presence of NaOH to yield the benzo-12-crown-4 ether (21) in low yield (Scheme 2). Furthermore, the reaction of benzo-12-crown-4 (21) with paraformaldehyde and HBr in DCM afforded the 4,5-dibromomethyl benzo-12-crown-4 (22) in 55% yield. Next, the compound 22 was treated with the DPM1 to provide the anticipated DPM4 in respectable yield (Scheme 2). Thus, prepared DPM receptors were characterized and identified by means of NMR spectroscopy and mass spectrometry (see ESI†).
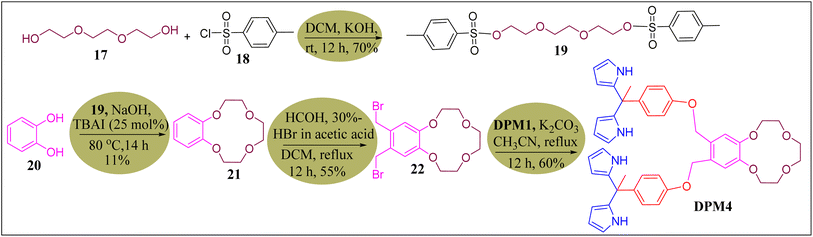 |
| Scheme 2 Synthesis of the benzo fused 12-crown-4 based bis-DPM4 from catechol and 19. | |
Anion binding studies through UV-vis titrations
After successful synthesis and characterization of the targeted DPM3/DPM4 (5.0 × 10−6 mol L−1), next we performed the UV-vis titration experiments using them with a variety of anions for example, HSO4−, NO3−, SCN−, AcO−, H2PO4−, F−, Cl−, Br−, and I−, used in the form of their tetrabutylammonium salts (1.5 × 10−4 mol L−1) in CH3CN solvent. Both the newly synthesized bis-dipyrromethanes i.e., DPM3 and DPM4, displayed maximum spectral change in complex (anion + receptor) form (increase in absorbance by increasing the concentration of guest), and different binding affinities with the test anions. Towards the first stage of the experiment, UV-vis spectra of the free DPM3 and DPM4 (5.0 × 10−6 M) in MeCN solution was recorded, then the guest molecule TBAF (1.5 × 10−4 M) was added in portionwise (5 μL and 10 μL) till the saturation established. From the inspection of the Fig. 2a, it can clearly be seen that the spectra of DPM3 and DPM4 showed the bands with maximum wavelength at ca. 275 nm, and 277 nm respectively. Noticeably, upon successive addition of 5 μL of TBAF to DPM3, the decrease in the absorbance values were observed at 275 nm. Whereas, upon the successive addition 10 μL TBAF to DPM4, the increase in the absorbance values was observed at 275 nm, which continues till the saturation point is obtained (Fig. 2a and 3a). Data obtained were fitted in the Bindfit v0.5 software at 275 nm and 258 nm, respectively for the DPM3 and DPM4 with the fluoride anion, the 1
:
1 stoichiometry were observed for both the receptors with the binding constant values of K1:1 14923.78 M−1 and 63789.81 M−1, respectively (Fig. 2 and 3). Moreover, the linear acetate anion also showed good results with both the receptors, stoichiometry (1
:
1) and binding constants K1:1 = 6018.41 M−1 for DPM3@AcO− and K1:1 = 12935.35 M−1 for DPM4@AcO− (<5% error). The stoichiometry and binding constant values of the bis-dipyrromethanes with various other tested anions were calculated in similar manner, using Nelder–Mead fit method from online supramolecular Bindfit v0.5 software (see ESI†). From the calculations of the binding constants (K1:1, K1:2, and K2:1), we observed that the in all the cases, the 1
:
1 stoichiometry was obtained with satisfied value of K1:1 within error <10, whereas for the data fitting to 1
:
2, and 2
:
1 stoichiometry, negative or unsatisfied values with more errors were observed (Table 1). Noticeably, similar results were obtained by Pakkirisamy and co-workers with various dipyrromethane towards different anions using UV-vis, fluorescence, NMR spectroscopy besides the DFT studies.71 On the other hand, Ganguly's and Majerski's research groups, independently, have reported similar studies using different dipyrromethane receptors with varied anions (Fig. 4 and 5).72,73
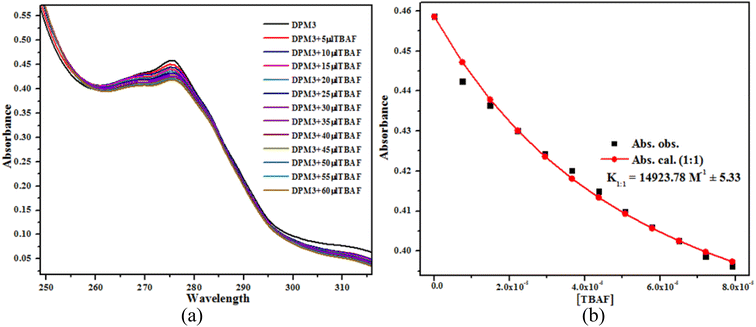 |
| Fig. 2 The UV-vis titration of DPM3 with TBAF in CH3CN (a), and binding isotherm fitting of thus obtained data using online Bindfit v0.5 program (b). | |
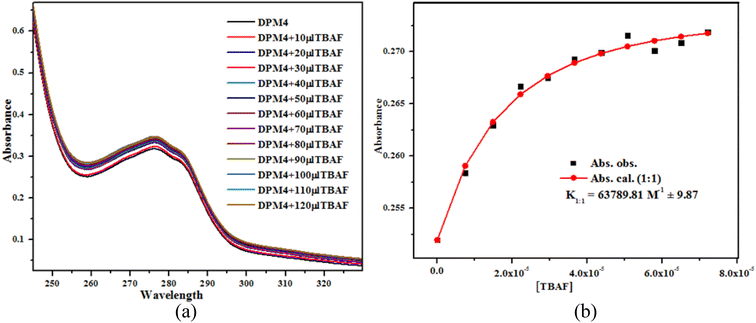 |
| Fig. 3 The UV-vis titration of DPM4 with TBAF in CH3CN (a), and binding isotherm fitting of thus obtained data using online Bindfit v0.5 program (b). | |
Table 1 Binding constant values of DPM3 and DPM4 receptors with various test anionsa
S. no |
Anion |
DPM3 (Ka, M−1) |
DPM4 (Ka, M−1) |
Ka values, calculated using the Bindfit software through 1 : 1 binding model. The errors are in parenthesis with <10%, [DPM3 and DPM4] = 5.0 × 10−6 M, [anions TBA salts] = 1.5 × 10−4 M at T = 32 °C ± 2 °C. ND = not detectable. |
1 |
F− |
14923.78 (±5.33) |
63789.81 (±9.87) |
2 |
Cl− |
12616.17 (±1.63) |
12670.68 (±4.78) |
3 |
Br− |
5527.42 (±2.70) |
8644.5 (±9.96) |
4 |
I− |
ND |
ND |
5 |
NO3− |
1900.37 (±0.38) |
3640.14 (±1.77) |
6 |
SCN− |
NA |
516.07 (±1.38) |
7 |
AcO− |
6018.41 (±3.95) |
12935.35 (±4.09) |
8 |
H2PO4− |
ND |
1838.17 (±1.98) |
9 |
HSO4− |
2825.47 (±1.17) |
2121.77 (±0.50) |
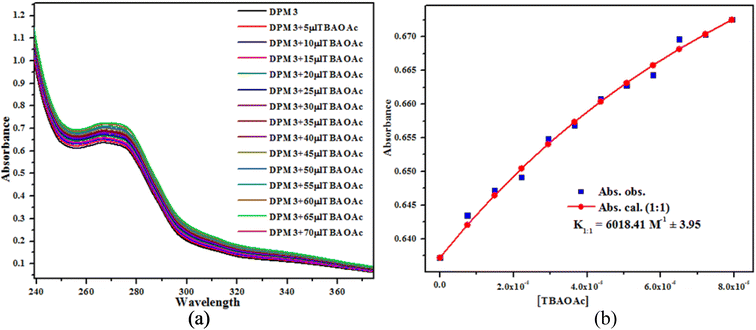 |
| Fig. 4 The UV-vis titration of DPM3 with TBAOAc in CH3CN (a), and binding isotherm fitting of thus obtained data using online Bindfit v0.5 program (b). | |
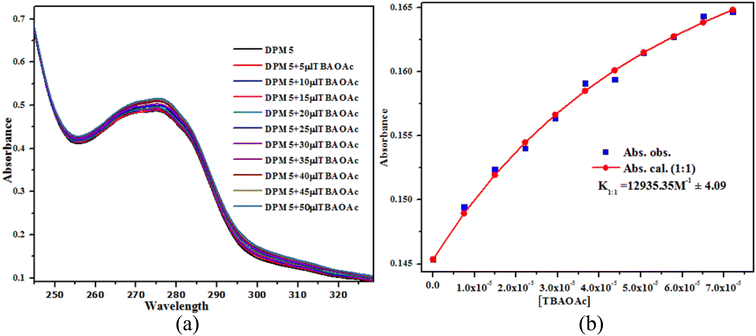 |
| Fig. 5 UV-vis titration of receptor DPM4 with TBAOAc in CH3CN (a), and binding isotherm fitting of UV-vis titration data by using Bindfit v0.5 program (b). | |
1H-NMR interpretation and the Job's method of continuous variation
To further support the results of UV-vis spectroscopy, obtained from the online supramolecular Bindfit program, partial 1H-NMR spectra of the DPM3 and DPM4 were compared with that of the complexed ones (DPM3/DPM4@TBAF). From the inspection of the Fig. 6, it could be clearly noticed that a peak at 10.37 ppm, attributed to the pyrrolic NH-protons, and the peaks in the range of 8.20–6.55 ppm relates to the aromatic protons, whereas the signals appeared ca. at 5.89 ppm and 5.58 ppm belongs to the beta-pyrrolic protons. From the spectrum of the complexed one (DPM3@F−), it could be seen that the pyrrolic N–H protons shifts downfield from 10.37 ppm to 10.87 ppm with Δδ = 0.50 ppm, whereas the aromatic ring protons as well as the beta-pyrrolic protons moved towards upfield. Therefore, it could be pointed out that the DPM3 binds with fluoride anion through both NH-anion as well as anion–π interactions (Fig. 6). On the other front, it could be inspected from the Fig. 7 that in the case of the DPM4, pyrrolic N–H protons largely shift towards the low field (downfield) i.e., from 7.80 ppm to the 10.83 ppm (Δδ = 3.03 ppm) (Fig. 6). Herein, it was observed that the overall binding constants results were obtained from combination of four N–H-anion hydrogen bonding as well as through anion–π interactions (Fig. 7).74–77
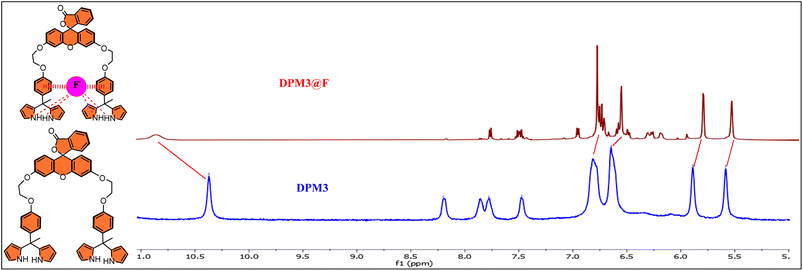 |
| Fig. 6 Comparative 1H-NMR spectra of DPM3 receptor and complex form (DPM3@F−) recorded in the recorded in DMSO-d6 solvent. | |
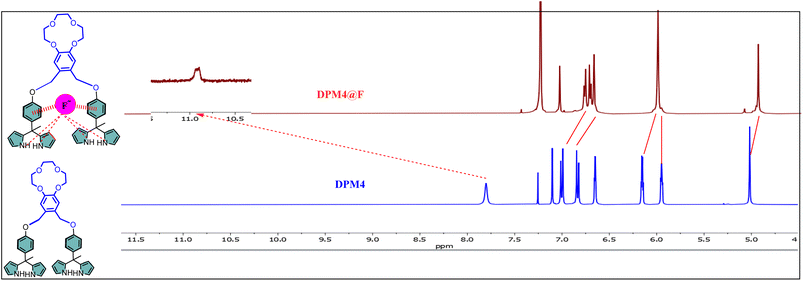 |
| Fig. 7 1H-NMR spectra of DPM4 & complex (DPM4@F−) recorded in CDCl3 solvent. | |
The Job's method of continuous variation was performed to authenticate the stoichiometric results acquired from the online Bindfit v0.5 software for DPM3@anions and DPM4@anions. In this method, the titration was performed between the host molecule (DPM3 & DPM4) and guest molecule (fluoride & acetate) in acetonitrile solution at ambient temperature. However, the concentrations of both the host and guest molecules were held constant (5.0 × 10−6 M), whereas their mole fractions were varied. Furthermore, the graph was plotted between change in the absorption at λmax and the mole fraction of DPM3/DPM4 vs. the mole fraction of TABF-DPM3 or TABF-DPM4. From the inspection of the Fig. 8, it was confirmed that the complex concentration of DPM3@F− and DPM4@F− appeared maximum at value 0.5, thereby confirming the 1
:
1 host–guest complex formation. Along similar lines, for DPM3@CH3COO− as well as DPM4@CH3COO−, we noticed the 1
:
1 stoichiometry (see ESI†). From the results of various techniques such as UV-vis titrations, Bindfit v0.5 program, 1H-NMR analysis, and Job's plot, 1
:
1 stoichiometry is confirmed besides the N–H⋯anions (H-bonding) and anion–π interactions (Fig. 9).
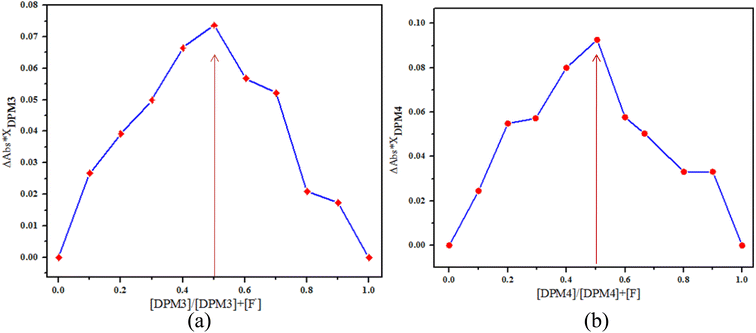 |
| Fig. 8 Job's plot of DPM3 & DPM4 with TBAF in acetonitrile at ambient temperature. | |
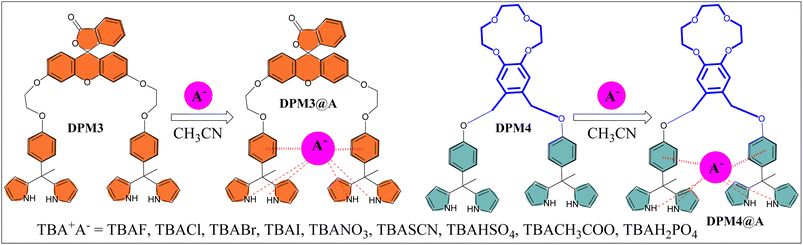 |
| Fig. 9 The probable complex structures of the novel bis-dipyrromethane receptors DPM3 and DPM4 with different anions in 1 : 1 stoichiometric ratio. | |
Conclusions and future prospects
Two novel fluorescein and benzo-12-crown-4 ether based dipyrromethane receptors DPM3 and DPM4 were successfully synthesized, and evaluated for anion-binding assay through UV-vis spectrophotometric titrations. The binding constants of both the DPM3 and DPM4 with different anions were determined in acetonitrile using online supramolecular Bindfit v0.5 program, displaying 1
:
1 stoichiometry for all the examined anions, were further valuated by Job's plots. The comparative study of 1H-NMR spectra revealed that the anions are captured through both hydrogen bonding and anion–π interactions. The ion-pair studies of these DPMs are underway, and will be published in due course. Moreover, benzo-12-crown-4 ether in addition to other higher analogous crown ethers based strapped calix[4]pyrroles (C4Ps) are also under investigations, and will be published shortly. Hopefully, these outcomes will guide to the researchers to develop even more interesting crown ether-based systems for varied applications in future studies.
Conflicts of interest
There are no conflicts to declare.
Acknowledgements
Dr Rashid Ali thanks DST-SERB New Delhi for the financial support (project file no. ECR/2017/000821). Shafieq Ahmad Wagay thanks to University Grants Commission, New Delhi, and Government of India for providing doctoral fellowship. The authors are also thankful to Department of Chemistry, Jamia Millia Islamia, New Delhi for providing the necessary research facilities.
References
- I. V. Kolesnichenko and E. V. Anslyn, Chem. Soc. Rev., 2017, 46, 2385–2390 RSC.
- D.-X. Wang and M.-X. Wang, Acc. Chem. Res., 2020, 53, 1364–1380 CrossRef CAS PubMed.
- X. Ma and Y. Zhao, Chem. Rev., 2015, 115, 7794–7839 CrossRef CAS PubMed.
- A. D. O'Donnell, S. Salimi, L. R. Hart, T. S. Babra, B. W. Greenland and W. Hayes, React. Funct. Polym., 2022, 172, 105209 CrossRef.
- U. Lüning, Angew. Chem., Int. Ed., 2013, 52, 4724 CrossRef.
- P. D. Beer and P. A. Gale, Angew. Chem., Int. Ed., 2001, 40, 486–516 CrossRef CAS PubMed.
- S. Kubik, Chem. Soc. Rev., 2010, 39, 3648 RSC.
- J. R. Knowles, Annu. Rev. Biochem., 1980, 49, 877–919 CrossRef CAS PubMed.
- D. Markovich, Physiol. Rev., 2001, 81, 1499–1533 CrossRef CAS PubMed.
- J. Han, L. Kiss, H. Mei, A. M. Remete, M. Ponikvar-Svet, D. M. Sedgwick, R. Roman, S. Fustero, H. Moriwaki and V. A. Soloshonok, Chem. Rev., 2021, 121, 4678–4742 CrossRef CAS PubMed.
- K. Bowman-James, Acc. Chem. Res., 2005, 38, 671–678 CrossRef CAS PubMed.
- Anion Coordination Chemistry, ed. K. Bowman James, A. Bianchi and E. García España, Wiley, 2011 Search PubMed.
- S. O. Kang, M. A. Hossain and K. Bowman-James, Coord. Chem. Rev., 2006, 250, 3038–3052 CrossRef CAS.
- B. a Moyer, D. A. Bostick, C. J. Fowler, H.-A. Kang, A. Ruas, L. H. Delmau, T. J. Haverlock, J. M. Llinares, A. Hossain, S. O. Kang, K. Bowman-James, J. A. Shriver, M. Marquez and J. L. Sessler, Supramolecular Chemistry of Selective Anion Recognition for Anions of Environmental Relevance, 2005 Search PubMed.
- E. García-España, P. Díaz, J. M. Llinares and A. Bianchi, Coord. Chem. Rev., 2006, 250, 2952–2986 CrossRef.
- T. Clifford, A. Danby, J. M. Llinares, S. Mason, N. W. Alcock, D. Powell, J. A. Aguilar, E. García-España and K. Bowman-James, Inorg. Chem., 2001, 40, 4710–4720 CrossRef CAS PubMed.
- A. Bencini, A. Bianchi, E. Garcia-Espana, M. Giusti, S. Mangani, M. Micheloni, P. Orioli and P. Paoletti, Inorg. Chem., 1987, 26, 3902–3907 CrossRef CAS.
- A. Schaly, R. Belda, E. García-España and S. Kubik, Org. Lett., 2013, 15, 6238–6241 CrossRef CAS PubMed.
- L. Rodríguez, J. C. Lima, A. J. Parola, F. Pina, R. Meitz, R. Aucejo, E. Garcia-España, J. M. Llinares, C. Soriano and J. Alarcón, Inorg. Chem., 2008, 47, 6173–6183 CrossRef PubMed.
- P. A. Gale, E. N. W. Howe and X. Wu, Chem, 2016, 1, 351–422 CAS.
- P. A. Gale and C. Caltagirone, Chem. Soc. Rev., 2015, 44, 4212–4227 RSC.
- S. A. Wagay, M. Alam and R. Ali, J. Mol. Struct., 2023, 135982 CrossRef CAS.
- N. Busschaert, C. Caltagirone, W. Van Rossom and P. A. Gale, Chem. Rev., 2015, 115, 8038–8155 CrossRef CAS PubMed.
- N. Kaur, G. Kaur, U. A. Fegade, A. Singh, S. K. Sahoo, A. S. Kuwar and N. Singh, TrAC, Trends Anal. Chem., 2017, 95, 86–109 CrossRef CAS.
- A. Pal, M. Karmakar, S. R. Bhatta and A. Thakur, Coord. Chem. Rev., 2021, 448, 214167 CrossRef CAS.
- A. Borissov, I. Marques, J. Y. C. Lim, V. Félix, M. D. Smith and P. D. Beer, J. Am. Chem. Soc., 2019, 141, 4119–4129 CrossRef CAS PubMed.
- M. J. Langton, C. J. Serpell and P. D. Beer, Angew. Chem., Int. Ed., 2016, 55, 1974–1987 CrossRef CAS PubMed.
- X. Wu, A. M. Gilchrist and P. A. Gale, Chem, 2020, 6, 1296–1309 CAS.
- G. M. Romano, M. Savastano, C. Bazzicalupi, R. Chelli, V. Lippolis and A. Bencini, Dalton Trans., 2023, 52, 6457–6472 RSC.
- Anion Recognition in Supramolecular Chemistry, ed. P. A. Gale and W. Dehaen, Springer Berlin Heidelberg, Berlin, Heidelberg, 2010, vol. 24 Search PubMed.
- T. W. Bell and N. M. Hext, Chem. Soc. Rev., 2004, 33, 589–598 CAS.
- Y. Wang, Y. Wang, S. Sahu, A. A. Gallo and X.-D. Zhou, Polymers, 2023, 15, 352 CrossRef CAS PubMed.
- I. A. Rather, S. A. Wagay and R. Ali, Coord. Chem. Rev., 2020, 415, 213327 CrossRef CAS.
- I. A. Rather, S. A. Wagay, M. S. Hasnain and R. Ali, RSC Adv., 2019, 9, 38309–38344 RSC.
- M. Savastano, C. García-Gallarín, M. D. López de la Torre, C. Bazzicalupi, A. Bianchi and M. Melguizo, Coord. Chem. Rev., 2019, 397, 112–137 CrossRef CAS.
- M. Savastano, C. Bazzicalupi, C. García-Gallarín, C. Giorgi, M. D. López de la Torre, F. Pichierri, A. Bianchi and M. Melguizo, Dalton Trans., 2018, 47, 3329–3338 RSC.
- M. Savastano, C. Bazzicalupi, C. Giorgi, P. Gratteri and A. Bianchi, Molecules, 2017, 22, 816 CrossRef PubMed.
- C. Bazzicalupi, A. Bianchi, C. Giorgi, P. Gratteri, P. Mariani and B. Valtancoli, Dalton Trans., 2013, 42, 12130 RSC.
- S. A. Wagay, L. Khan and R. Ali, Chem.–Asian J., 2023, 18, e202201080 CrossRef CAS PubMed.
- I. A. Rather, S. A. Khan, R. Ali, T. A. Khan and R. Ali, J. Mol. Struct., 2022, 367, 120406 CAS.
- S. A. Wagay, I. A. Rather and R. Ali, Mater. Today: Proc., 2021, 36, 657–678 CrossRef CAS.
- J. Černý and P. Hobza, Phys. Chem. Chem. Phys., 2007, 9, 5291 RSC.
- E. R. Johnson, S. Keinan, P. Mori-Sánchez, J. Contreras-García, A. J. Cohen and W. Yang, J. Am. Chem. Soc., 2010, 132, 6498–6506 CrossRef CAS PubMed.
- A. S. Novikov, Polymers, 2023, 15, 1139 CrossRef CAS PubMed.
- C. Puzzarini, L. Spada, S. Alessandrini and V. Barone, J. Phys.: Condens.Matter, 2020, 32, 343002 CrossRef CAS PubMed.
- M. Savastano, C. Cappanni, C. Bazzicalupi, C. Lofrumento and A. Bianchi, Crystals, 2023, 13, 823 CrossRef CAS.
- E. Mulugeta, Q. He, D. Sareen, S.-J. Hong, J. H. Oh, V. M. Lynch, J. L. Sessler, S. K. Kim and C.-H. Lee, Chem, 2017, 3, 1008–1020 CAS.
- J. Yoo, M.-S. Kim, S.-J. Hong, J. L. Sessler and C.-H. Lee, J. Org. Chem., 2009, 74, 1065–1069 CrossRef CAS PubMed.
- I. A. Rather, F. A. Sofi, M. A. Bhat and R. Ali, ACS Omega, 2022, 7, 15082–15089 CrossRef CAS PubMed.
- I. A. Rather, R. Ali and A. Ali, Org. Chem. Front., 2022, 9, 6416–6440 RSC.
- I. A. Rather, S. A. Khan, R. Ali and T. A. Khan, J. Mol. Liq., 2022, 367, 120406 CrossRef CAS.
- A. Kim, R. Ali, S. H. Park, Y.-H. Kim and J. S. Park, Chem. Commun., 2016, 52, 11139–11142 RSC.
- M. K. Deliomeroglu, V. M. Lynch and J. L. Sessler, Chem. Sci., 2016, 7, 3843–3850 RSC.
- M. K. Deliomeroglu, V. M. Lynch and J. L. Sessler, Chem. Commun., 2014, 50, 11863–11866 RSC.
- P. A. Gale, J. L. Sessler, V. Král and V. Lynch, J. Am. Chem. Soc., 1996, 118, 5140–5141 CrossRef CAS.
- C. Guo, S. Sun, Q. He, V. M. Lynch and J. L. Sessler, Org. Lett., 2018, 20, 5414–5417 CrossRef CAS PubMed.
- Y. Zhou and X. Bao, Spectrochim. Acta, Part A, 2019, 210, 1–8 CrossRef CAS PubMed.
- R. Yi, X.-L. Liu, Z.-H. Tang, C. Huang, B.-X. Zhu and C. Zhu, Chem. Pap., 2021, 75, 4405–4411 CrossRef CAS.
- E. A. Katayev, N. V. Boev, V. N. Khrustalev, Y. A. Ustynyuk, I. G. Tananaev and J. L. Sessler, J. Org. Chem., 2007, 72, 2886–2896 CrossRef CAS PubMed.
- J. L. Sessler, V. Roznyatovskiy, G. D. Pantos, N. E. Borisova, M. D. Reshetova, V. M. Lynch, V. N. Khrustalev and Y. A. Ustynyuk, Org. Lett., 2005, 7, 5277–5280 CrossRef CAS PubMed.
- X. Zhang, Y. Shiraishi and T. Hirai, Tetrahedron Lett., 2007, 48, 8803–8806 CrossRef CAS.
- Z.-H. Fu, X. Han, Y. Shao, J. Fang, Z.-H. Zhang, Y.-W. Wang and Y. Peng, Anal. Chem., 2017, 89, 1937–1944 CrossRef CAS PubMed.
- D. Y. Lee, N. Singh, M. J. Kim and D. O. Jang, Org. Lett., 2011, 13, 3024–3027 CrossRef CAS PubMed.
- I. Hussain, N. B. Singh, A. Singh, H. Singh and S. C. Singh, Biotechnol. Lett., 2016, 38, 545–560 CrossRef CAS PubMed.
- H. J. Jung, N. Singh, D. Y. Lee and D. O. Jang, Tetrahedron Lett., 2010, 51, 3962–3965 CrossRef CAS.
- F. Ullah, T. A. Khan, J. Iltaf, S. Anwar, M. F. A. Khan, M. R. Khan, S. Ullah, M. Fayyaz ur Rehman, M. Mustaqeem, K. Kotwica-Mojzych and M. Mojzych, Appl. Sci., 2022, 12, 1102 CrossRef CAS.
- G. W. Gokel, W. M. Leevy and M. E. Weber, Chem. Rev., 2004, 104, 2723–2750 CrossRef CAS PubMed.
- V. Rüdiger, H.-J. Schneider, V. P. Solov’ev, V. P. Kazachenko and O. A. Raevsky, Eur. J. Org Chem., 1999, 1999, 1847–1856 CrossRef.
- A. A. Saddik, M. Mohammed and H.-C. Lin, J. Mater. Chem. B, 2020, 8, 9961–9970 RSC.
- I. A. Rather and R. Ali, Green Chem., 2021, 23, 5849–5855 RSC.
- P. C. A. Swamy, R. N. Priyanka and T. Pakkirisamy, Dalton Trans., 2014, 43, 4067 RSC.
- M. Alešković, N. Basarić, K. Mlinarić-Majerski, K. Molčanov, B. Kojić-Prodić, M. K. Kesharwani and B. Ganguly, Tetrahedron, 2010, 66, 1689–1698 CrossRef.
- M. Alešković, N. Basarić, I. Halasz, X. Liang, W. Qin and K. Mlinarić-Majerski, Tetrahedron, 2013, 69, 1725–1734 CrossRef.
- I.-W. Park, J. Yoo, B. Kim, S. Adhikari, S. K. Kim, Y. Yeon, C. J. E. Haynes, J. L. Sutton, C. C. Tong, V. M. Lynch, J. L. Sessler, P. A. Gale and C.-H. Lee, Chem.–Eur. J., 2012, 18, 2514–2523 CrossRef CAS PubMed.
- G. Gil-Ramírez, E. C. Escudero-Adán, J. Benet-Buchholz and P. Ballester, Angew. Chem., Int. Ed., 2008, 47, 4114–4118 CrossRef PubMed.
- S. K. Kim, H. G. Lee, G. V-Zfflciga, V. M. Lynch, C. Kim and J. L. Sessler, Chem.–Eur. J., 2014, 20, 11750–11759 CrossRef CAS PubMed.
- J. Yoo, I. Park, T. Y. Kim and C. H. Lee, Bull. Korean Chem. Soc., 2010, 31(3), 630–634 CrossRef CAS.
Footnote |
† Electronic supplementary information (ESI) available: The original 1H-NMR, 13C-NMR, HRMS of all the synthesized compounds are available in ESI. The UV-vis titration of receptor with anions in CH3CN. Snapshot capture of Bindfit plots for receptors and anion titration, displaying 1 : 1, 1 : 2 and 2 : 1 stoichiometry utilizing Nelder–Mead fit are available in ESI. See DOI: https://doi.org/10.1039/d3ra05171d |
|
This journal is © The Royal Society of Chemistry 2023 |
Click here to see how this site uses Cookies. View our privacy policy here.