DOI:
10.1039/D3RA04996E
(Paper)
RSC Adv., 2023,
13, 27456-27460
Multicomponent reaction (MCR) for constructing bis-spirocyclohexane skeletons using β-nitrostyrene derived MBH acetates, 1,3-indanedione and aldehydes via [1 + 1 + 1 + 3] annulation†
Received
24th July 2023
, Accepted 4th September 2023
First published on 13th September 2023
Abstract
An AB2C type four-component quadruple cascade reaction has been established between nitroallylic MBH acetate, 1,3-indanedione and aldehyde for generating bis-spirocyclohexanes. This reaction progressed in an unusual [1 + 1 + 1 + 3] annulation manner via a Knoevenagel/Michael/Michael/Michael sequence, resulting in the generation of three/four chiral centres, and two all-carbon quaternary centres through the formation of 3 new C–C bonds.
Introduction
The development of novel synthetic strategies for constructing new chiral spirocyclic compounds with varied medicinal potentials is a great challenge in organic and pharmaceutical chemistry.1 In this regard, Multicomponent Reactions (MCRs)2 are preferred for the synthesis of chiral spirocyclic compounds3 by virtue of their advantages, i.e., one-pot synthesis, avoiding isolation of intermediates and atom economy.
In recent times, the β-nitrostyrene derived Morita–Baylis–Hillman (MBH)4 adducts have been explored extensively towards the construction of various MCRs.5 The β-nitrostyrene derived MBH adducts6–8 are one of the essential classes of molecules in medicinal chemistry, due to their utility as building blocks for constructing complex and biologically sound molecules.9
Among the various β-nitrostyrene derived MBH adducts, nitroallylic MBH acetates have been explored extensively to give various spirocycles,10 heterocycles,11 carbocycles,12 bicyclic skeletons,13 and arenes.14 But to date there are no available reports for the construction of bis-spirocyclohexanes utilizing nitrostyrene derived MBH acetate with 1,3-indanedione15 or arylidene indanedione.16,17
In 2015, He et al. successfully utilized acrylate derived MBH adducts along with 2-arylidene-1,3-indanediones to demonstrate a [2 + 2 + 2] annulation protocol for producing substituted bis-spirocyclohexanes (eqn (a), Scheme 1).18
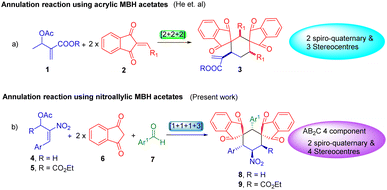 |
| Scheme 1 Construction of substituted bis-spirocyclohexanes using MBH acetates. | |
Hereby, we propose an MCR strategy to explore the utility of nitroallylic MBH acetate for generating fully substituted bis-spirocyclohexanes in a unique [1 + 1 + 1 + 3] annulation fashion (eqn (b), Scheme 1).
In continuation of our perpetual study on heterocycles and spirocycles,19 we have demonstrated an AB2C type four-component reaction20 between 1,3-indanedione, aryl aldehydes, and β-nitrostyrene derived MBH acetates via [1 + 1 + 1 + 3] cyclization strategy (Scheme 2).
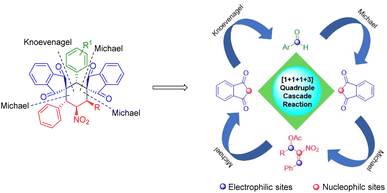 |
| Scheme 2 AB2C type four component quadruple cascade reaction. | |
Results and discussion
Optimization studies of quadruple cascade reactions were carried out and the obtained results were tabulated (Table 1). The reaction initially carried out with potassium carbonate (1.5 equiv.) in acetonitrile at ambient temperature, gave the desired compound 8a in 24% yield (entry 1, Table 1). Increasing the amount of indanedione to 2 equiv. gave a marginal increase in the yield i.e., 25% for the product formation 8a (entry 2, Table 1). The use of chlorinated solvents, i.e., chloroform, didn't alter the yield even after 24 h (entry 3, Table 1). The yield for product 8a improved to 35% with the use of potassium carbonate in 3 equiv. amounts (entry 4, Table 1). There is no improvement was observed with the change in reaction time as well as temperature (entries 5 and 6, Table 1). The use of methanol as solvent decreased the diastereomeric excess and yield due to the poor solubility of in situ-formed KC product (entry 7, Table 1). Utilisation of organic bases such as DABCO, urea and thiourea couldn't improve the yield for the product formation 8a (entries 8–15, Table 1). We also examined the effect of other inorganic bases such as Cs2CO3 but, there is no appreciable improvement was observed for the formation of desired product 8a (entries 16 and 17, Table 1). It was observed that, there is no improvement in the yield with the higher concentrations of aldehyde (entries 18–20, Table 1). As the base concentration increases, the yield of 8a gets decreases due to the high amount of side products formation (entries 21 and 22 Table 1). The use of mixture of solvents did not improve the yield and delivered the compound 8a in 25% of yield (entry 23, Table 1). This is due to the less solubility of in situ formed KC side product for further cascade process.
Table 1 Optimization studies for quadruple cascade reactiona
The lower yield for 8a is due to the formation of side product 11 (i.e., double Michael reaction of MBH acetate 4a with 1,3-indanedione 6) and 10a (Knoevenagel condensation between aldehyde 7a and 1,3-indanedione 6). It was also observed that, recovery of aldehyde for the most of the reactions was predominant. In conclusion of the optimization studies, the use of potassium carbonate (3 equiv.) in acetonitrile solvent gave the maximum yield. The final product 8a obtained with highly diastereoselectivity was fully characterized by 1H NMR, 13C NMR, DEPT-135 and the structure was further confirmed by single crystal X-ray analysis.21
The studies for the formation of bis-spirocyclohexane were further explored into substrate scope study with various MBH acetates 4/5 and aldehydes 7a–i possessing electron withdrawing and donating substituents (Table 2). This reaction when employed with p-nitrobenzaldehyde resulted in 34% yield for product 8b. When the reaction was carried out with p-cyanobenzaldehyde, the corresponding bis-spirocyclohexane 8c was obtained in a 39% yield. Compound 8d was obtained in 31% of yield with the use of unsubstituted benzaldehyde. m-bromobenzaldehyde on reacting with indanedione 6 and MBH aetate 4 retained high diastereoselectivity for product 8e in 24% of yield. It was observed that the substitution of an electron-withdrawing group i.e., m-cyano, gave 8f with a yield of 31%. The ortho-nitro substituted benzaldehyde delivered the corresponding bis-spirocyclohexane 8g in 35% yield.
Table 2 Substrate scope for construction of bis-spiroyclohexanesa,b,c
Compound 4/5 (0.5 mmol), 6 (1.0 mmol), aldehyde 7 (0.5 mmol) and K2CO3 (1.5 mmol) were dissolved in 5 mL of ACN and stirred at 30 °C. Isolated yields. dr was determined by 1H NMR. |
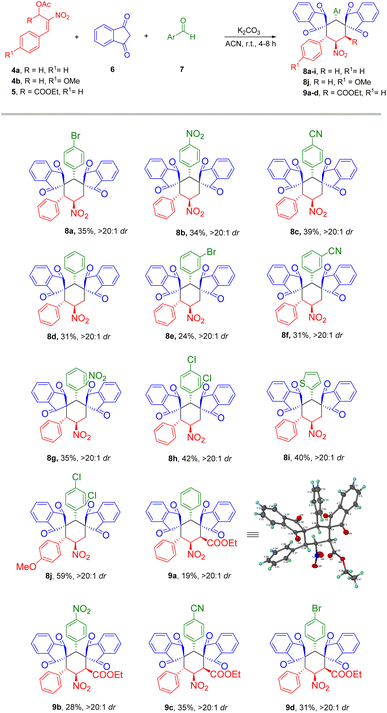 |
A good yield of 42% for the formation of compound 8h was observed when o,p-dichlorobenzaldehyde was employed in the reaction. Heteroaromatic aldehydes also tolerated the reaction conditions towards the formation of bis-spirocyclohexanes. The quadruple cascade reaction with 2-thiophenyl aldehyde produced compound 8i in a good yield of 40%. An impressive yield of 59% was observed for compound 8j, by the reaction of p-methoxy MBH acetate 4b, 1,3-indanedione and o,p-dichloro benzaldehyde. We further explored the utility of secondary nitroallylic MBH acetate 5 to develop fully substituted bis-spirocyclohexanes 9a–d. The reaction involving unsubstituted benzaldehyde, gave the desired compound 9a in 19% yield. The substitution of electron-withdrawing groups like –NO2, –CN at the para position of the benzaldehyde resulted in compounds 9b and 9c in 28% and 35%, respectively. Whereas, the p-bromo benzaldehyde delivered the compound 9d in 31% of the yield. Attempts using aliphatic aldehydes led to trace amounts of corresponding bis-spirocyclohexanes. This may be presumably due to poor reactivity of 2-alkylidene indanediones.
We further examined the scalability of our protocol by gram scale preparation of bis-spirocyclohexane 8a (Scheme 3). To our delight the reaction retained with high diastereoselectivity for product 8a formation.
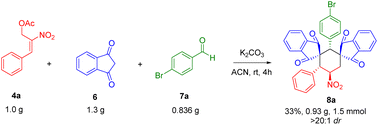 |
| Scheme 3 Gram scale synthesis of bis-spirocyclohexane 8a. | |
A possible mechanistic pathway for the formation of bis-spirocyclohexane products 8/9 is explained in Scheme 4. Base assisted MBH acetate 4a on reaction with 1,3-indanedione via SN2 manner generates intermediate A as a Michael adduct. Intermediate A undergoes another Michael addition with in situ generated KC product arylideneindanedione 10a to form intermediate B which further undergoes 6-endo-trig cyclization via Michael addition to produce 8a. The overall reaction follows a Knoevenagel/Michael/Michael/Michael quadruple cascade reaction sequence via [1 + 1 + 1 + 3] annulation. This plausible mechanism is further supported by the isolation of side product 11. The formation of side product 11 is due to a parallel reaction of intermediate A with MBH acetate 4a in a Michael addition manner via double SN2 fashion.
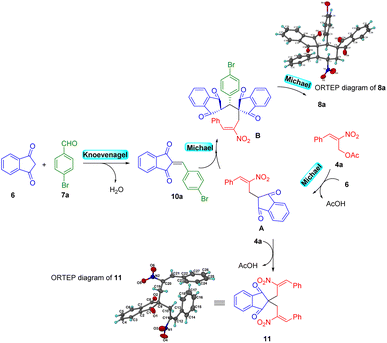 |
| Scheme 4 Plausible reaction mechanism for the quadruple cascade sequence. | |
Conclusions
A multicomponent reaction was demonstrated for developing fully substituted bis-spirocyclohexanes derived from β-nitrostyrene derived MBH acetates. This AB2C type four-component cascade protocol between nitroallylic MBH acetate, 1,3-indanedione, and aldehyde resulted in synthesis of bis-spirocyclohexanes in high diastereoselectivity.
Conflicts of interest
There are no conflicts of interest.
Acknowledgements
SA greatly acknowledges DST-SERB for providing financial support under TARE (TAR/2022/000207). GVB thanks CSIR-New Delhi for Senior Research Fellowship (09/1253(0003)/2020-EMR-I). We are thankful to VFSTR for providing Central Instrumentation facilities at CoExAMMPC.
Notes and references
-
(a) W. Francke and W. Kitching, Curr. Org. Chem., 2001, 5, 233 CrossRef CAS;
(b) J. Huang and A. J. Frontier, J. Am. Chem. Soc., 2007, 129, 8060 CrossRef CAS PubMed;
(c) X. Companyo, A. Zea, A. N. R. Alba, A. Mazzanti, A. Moyano and R. Rios, Chem. Commun., 2010, 46, 6953 RSC;
(d) R. Rios, Chem. Soc. Rev., 2012, 41, 1060 RSC.
-
(a) E. Ruijter, R. Scheffelaar and R. V. A. Orru, Angew. Chem., Int. Ed., 2011, 50, 6234 CrossRef CAS PubMed;
(b) A. Domling and I. Ugi, Angew. Chem., Int. Ed., 2000, 39, 3168 CrossRef CAS;
(c) J. Zhu and H. Bienayme, Multicomponent Reactions, Wiley-VCH, Weinheim, Germany, 2005 CrossRef;
(d) L. F. Tietze, Chem. Rev., 1996, 96, 115 CrossRef CAS PubMed;
(e) K. C. Nicolaou, D. J. Edmonds and P. G. Bulger, Angew. Chem., Int. Ed., 2006, 45, 7134 CrossRef CAS PubMed;
(f) L. F. Tietze, G. Brasche and K. M. Gericke, Domino Reactions in Organic Synthesis, Wiley-VCH, Weinheim, Germany, 2006 CrossRef;
(g) S. F. Basha, T. N. Prasad, V. B. Gudise, V. S. Kumar, N. Mulakayala and S. Anwar, Synth. Commun., 2019, 49, 3181 CrossRef.
-
(a) Y. Yang, X. Wang, X. Ye, B. Wang, X. Bao and H. Wang, Org. Biomol. Chem., 2021, 19, 4610 RSC;
(b) A. J. Boddy and J. A. Bull, Org. Chem. Front., 2021, 8, 1026 RSC;
(c) P.-W. Xu, J.-S. Yu, C. Chen, Z.-Y. Cao, F. Zhou and J. Zhou, ACS Catal., 2019, 9, 1820 CrossRef CAS;
(d) Y.-S. Cheng, S. Anwar and K. Chen, Mini-Rev. Org. Chem., 2018, 15, 364 CrossRef CAS.
-
(a) K.-i. Morita, Z. Suzuki and H. Hirose, Bull. Chem. Soc. Jpn., 1968, 41, 2815 CrossRef CAS;
(b) A. B. Baylis and M. E. D. Hillman, German Patent DE 2155113, 1972.
- W.-Y. Huang, S. Anwar and K. Chen, Chem. Rec., 2016, 17, 363 CrossRef PubMed.
- For 2° nitroallylic amines refer
(a) R. Gurubrahamam, Y. M. Chen, W.-Y. Huang, Y.-T. Chan, H.-K. Chang, M.-K. Tsai and K. Chen, Org. Lett., 2016, 18, 3046 CrossRef CAS PubMed;
(b) Y. Wang, S. Zhu and D. Ma, Org. Lett., 2011, 13, 1602 CrossRef CAS PubMed.
- For 1° nitroallylic alcohols refer
(a) P. S. Akula, Y.-J. Wang, B.-C. Hong, G.-H. Lee and S.-Y. Chien, Org. Lett., 2021, 23, 4688 CrossRef CAS PubMed;
(b) P. Suresh, S. Thamotharan and S. S. Ganesan, ChemistrySelect, 2021, 6, 2036 CrossRef CAS;
(c) R. Parella, S. Jakkampudi, H. Arman and J. C.-G. Zhao, Adv. Synth. Catal., 2019, 361, 208 CrossRef CAS PubMed;
(d) S. Biswas, A. Dagar, A. Srivastava and S. Samanta, Eur. J. Org Chem., 2015, 4493 CrossRef CAS;
(e) D. C. Cruz, R. Mose, C. V. Gomez, S. V. Torbensen, M. S. Larsen and K. A. Jorgensen, Chem.–Eur. J., 2014, 20, 11331 CrossRef PubMed;
(f) B.-C. Hong, D.-J. Lan, N. S. Dange, G.-H. Lee and J.-H. Liao, Eur. J. Org Chem., 2013, 2472 CrossRef CAS.
- For 2
nitroallylic alcohols refer
(a) R. J. Reddy, M. Waheed, T. Karthik and A. Shankar, New J. Chem., 2018, 42, 980 RSC;
(b) R. Gurubrahamam, Y.-S. Cheng and K. Chen, Org. Lett., 2015, 17, 430 CrossRef CAS PubMed;
(c) B. Han, X. Xie, W. Huang, X. Li, L. Yang and C. Peng, Adv. Synth. Catal., 2014, 356, 3676 CrossRef CAS. -
(a) T. Kumar, D. Verma, R. F. S. Barreto, W. O. Valenca, E. N. da Silva Junior and I. N. N. Namboothiri, Org. Biomol. Chem., 2015, 13, 1996 RSC;
(b) K. R. Eeda, R. Chandran, A. M. Sajith, K. V. Dileep, C. Sadasivan and S. Anwar, RSC Adv., 2016, 6, 77431 RSC;
(c) T. V. Baiju, R. G. Almeida, S. T. Sivanandan, C. A. de Simone, L. M. Brito, B. C. Cavalcanti, C. Pessoa, I. N. N. Namboothiri and E. N. da Silva Junior, Eur. J. Med. Chem., 2018, 151, 686 CrossRef CAS PubMed.
-
(a) A. Pareek, S. T. Sivanandan, S. Bhagat and I. N. N. Namboothiri, Tetrahedron, 2022, 108, 132650 CrossRef CAS;
(b) Y.-Y. Ai, D.-A. Li, G. Li, H.-P. Li, X.-H. He, X.-J. Fu, Y.-T. Wang, G. Zhan and B. Han, Adv. Synth. Catal., 2021, 363, 3283 CrossRef CAS;
(c) J.-Y. Liu, J. Zhao, J.-L. Zhang and P.-F. Xu, Org. Lett., 2017, 19, 1846 CrossRef CAS PubMed;
(d) R. Chen, X. Fan, J. Gong and Z. He, Asian J. Org. Chem., 2014, 3, 877 CrossRef CAS.
-
(a) S. T. Sivanandan and I. N. N. Namboothiri, J. Org. Chem., 2021, 86, 8465 CrossRef CAS PubMed;
(b) J. Liu, Q. Li, Z.-M. Cao, Y. Jin, J. Lin and S.-J. Yan, J. Org. Chem., 2019, 84, 1797 CrossRef CAS PubMed;
(c) J.-Q. Zhang, J.-J. Liu, C.-L. Gu, D. Wang and L. Liu, Eur. J. Org Chem., 2014, 5885 CrossRef CAS;
(d) S. Anwar, W.-Y. Huang, C.-H. Chen, Y.-S. Cheng and K. Chen, Chem.–Eur. J., 2013, 19, 4344 CrossRef CAS PubMed;
(e) D. R. Magar, Y.-J. Ke and K. Chen, Asian J. Org. Chem., 2013, 2, 330 CrossRef CAS;
(f) W.-Y. Huang, Y.-C. Chen and K. Chen, Chem.–Asian J., 2012, 7, 688 CrossRef CAS PubMed.
-
(a) W. Xiao, X. Yin, Z. Zhou, W. Du and Y.-C. Chen, Org. Lett., 2016, 18, 116 CrossRef CAS PubMed;
(b) T. Shu, Q. Ni, X. Song, K. Zhao, T. Wu, R. Puttreddy, K. Rissanen and D. Enders, Chem. Commun., 2016, 52, 2609 RSC;
(c) L. F. Yeh, S. Anwar and K. Chen, Tetrahedron, 2012, 68, 7317 CrossRef CAS.
- C.-L. Cao, Y.-Y. Zhou, J. Zhou, X.-L. Sun, Y. Tang, Y.-X. Li, G.-Y. Li and J. Sun, Chem.–Eur. J., 2009, 15, 11384 CrossRef CAS PubMed.
-
(a) Y.-L. Ji, X.-H. He, G. Li, Y.-Y. Ai, H.-P. Li, C. Peng and B. Han, Org. Chem. Front., 2020, 7, 563 RSC;
(b) L. Satham and I. N. N. Namboothiri, J. Org. Chem., 2018, 83, 9471 CrossRef CAS PubMed;
(c) D. Majee, S. Biswas, S. M. Mobin and S. Samanta, J. Org. Chem., 2016, 81, 4378 CrossRef CAS PubMed.
-
(a) S. Das, Tetrahedron, 2022, 122, 132954 CrossRef CAS;
(b) M. Wang, L. Yin, L. Cheng, Y. Yang and Y. Li, J. Org. Chem., 2021, 86, 12956 CrossRef CAS PubMed;
(c) Y. Mu, Q. Yao, L. Yin, S. Fu, M. Wang, Y. Yuan, L. Kong and Y. Li, J. Org. Chem., 2021, 86, 6755 CrossRef CAS PubMed;
(d) Z. Chen, F. Yu, R. Liu, S. Yang, J. Liu, B. Chen, S. Nagaraju, M. Zeng, C. Ding and X. Fang, Org. Lett., 2020, 22, 2381 CrossRef CAS PubMed;
(e) S. Das, RSC Adv., 2020, 10, 18875 RSC;
(f) S. Asadi and G. M. Ziarani, Mol. Diversity, 2016, 20, 111 CrossRef CAS PubMed.
-
(a) P. C. Settipalli and S. Anwar, Chem. Commun., 2022, 58, 10400 RSC;
(b) S. Anwar, L.-T. Lin, V. Srinivasadesikan, V. B. Gudise and K. Chen, RSC Adv., 2021, 11, 38648 RSC;
(c) V. B. Gudise, P. C. Settipalli, Y. P. Reddy and S. Anwar, ChemistrySelect, 2021, 6, 13589 CrossRef CAS;
(d) Y. P. Reddy, P. C. Settipalli, V. B. Gudise and S. Anwar, ChemistrySelect, 2021, 6, 4456 CrossRef CAS;
(e) V. B. Gudise, P. C. Settipalli, E. K. Reddy and S. Anwar, Eur. J. Org Chem., 2019, 2234 CrossRef CAS;
(f) Q.-Z. Xi, Z.-J. Gan, E.-Q. Li and Z. Duan, Eur. J. Org Chem., 2018, 4917 CrossRef CAS.
- For the synthesis of 1,3-indanedione derived bis-spirocycles refer
(a) W.-J. Yang, H.-L. Fang, J. Sun and C.-G. Yan, ACS Omega, 2019, 4, 13553 CrossRef CAS PubMed;
(b) Y.-Y. Zhang, R. Gurubrahamam and K. Chen, Adv. Synth. Catal., 2015, 357, 2457 CrossRef CAS;
(c) H.-H. Kuan, C.-H. Chien and K. Chen, Org. Lett., 2013, 15, 2880 CrossRef CAS PubMed;
(d) B. Dai, L. Song, P. Wang, H. Yi, W. Cao, G. Jin, S. Zhu and M. Shao, Synlett, 2009, 1842 CAS.
- R. Chen, S. Xu, X. Fan, H. Li, Y. Tang and Z. He, Org. Biomol. Chem., 2015, 13, 398 RSC.
-
(a) Y. P. Reddy, V. Srinivasadesikan, R. Balamurugan, M. C. Lin and S. Anwar, RSC Adv., 2023, 13, 5796 RSC;
(b) Y. P. Reddy and S. Anwar, RSC Adv., 2022, 12, 34634 RSC;
(c) V. S. Kumar, V. B. Gudise, P. C. Settipalli, E. K. Reddy, S. F. Basha, Y. P. Reddy, V. Srinivasadesikan, S.-L. Lee and S. Anwar, ChemistrySelect, 2020, 5, 3080 CrossRef CAS;
(d) P. C. Settipalli, Y. P. Reddy, V. B. Gudise and S. Anwar, ChemistrySelect, 2021, 6, 47 CrossRef CAS;
(e) V. S. Kumar, V. B. Gudise, E. K. Reddy and S. Anwar, J. Heterocycl. Chem., 2019, 56, 2753 CrossRef CAS;
(f) T. N. Prasad, K. R. Eeda, V. B. Gudise, S. F. Basha and S. Anwar, Synth. Commun., 2019, 49, 1277 CrossRef.
- D. Tejedor and F. G. Tellado, Chem. Soc. Rev., 2007, 36, 484 RSC.
- CCDC 2149813 for 8a compound contains the crystallographic data for this paper. See ESI† for complete XRD data of 9a (CCDC 2149825) and 11 (CCDC 2175054).
|
This journal is © The Royal Society of Chemistry 2023 |
Click here to see how this site uses Cookies. View our privacy policy here.