DOI:
10.1039/D3RA04182D
(Paper)
RSC Adv., 2023,
13, 22122-22147
Design, synthesis, in silico studies, and biological evaluation of novel pyrimidine-5-carbonitrile derivatives as potential anti-proliferative agents, VEGFR-2 inhibitors and apoptotic inducers†
Received
21st June 2023
, Accepted 11th July 2023
First published on 24th July 2023
Abstract
A novel series of pyrimidine-5-carbonitrile derivatives bearing benzylidene and hydrazone moieties with different linkers (spacers) were designed and synthesized as possible inhibitors of the vascular endothelial growth factor receptor-2 (VEGFR-2). The newly synthesized compounds were evaluated in vitro for their cytotoxic activities against two human cancer cell lines namely colon cancer (HCT-116) and breast cancer (MCF-7) using sorafenib as a standard anticancer drug. Compounds 9d, 11e, 12b, and 12d showed higher cytotoxic activities than sorafenib with IC50 values ranging from 1.14 to 10.33 μM. In particular, compound 11e exhibited excellent activities against HCT-116 and MCF-7 with IC50 values of 1.14 and 1.54 μM, respectively. Moreover, compound 11e exhibited about 47.32-fold cytotoxic activity against normal human fibroblast (WI-38) cells, lower than the cytotoxicity against the cancer cells. Compounds 11e and 12b were the most potent VEGFR-2 inhibitors with IC50 values of 0.61 and 0.53 μM, respectively, compared to sorafenib. Bedsides, compound 11e arrested the HCT-116 cell growth at S and sub-G1 phases, induced a significant increase in the apoptotic cells, and caused remarkable decrease in the levels of TNF-α, IL-6, and caspase-3. Finally, the binding patterns of the target derivatives were investigated through the docking study against the proposed molecular target (VEGFR-2, PDB ID 1YWN). The results of molecular docking studies showed similar binding modes to sorafenib against VEGFR-2. In addition, molecular dynamic simulations revealed the stability of compound 11e in the active site for 100 ns.
1. Introduction
Cancer, a disease characterized by uncontrollable cell growth, is still one of the most serious diseases to threaten human life.1 Although there are wide advances dealing with its biochemistry and trying to understand its progression, the complete cure of cancer has not been achieved yet.2–4 The increased incidence of cancer cases returns primarily to the resistance of the cancer cells to the known medications, the reason that opens the door to research to either find new targets or to construct new chemical scaffolds to act on the previously identified targets.
Vascular Endothelial Growth Factor (VEGF) has been nominated as one of the most prevalent tumor angiogenesis mediators. VEGF initiates endothelial cell activation and proliferation.5 However, it promotes vascular permeability in solid tumors.6 These processes are all mediated via stimulation of a specific type of tyrosine kinase receptors, VEGFR-2 receptor.7 However, VEGF/VEGFR-2 pathway contributes to the progress of numerous cancer types including breast, colon, gastric and lung via migration, metastasis and stimulation of angiogenesis.8 Therefor hindering the VEGF/VEGFR-2 pathway appears crucial in stopping angiogenesis.9 Accordingly, VEGFR-2 gained an increasing importance in the anti-angiogenic therapy against cancer. Unfortunately, treatment with the currently FDA approved VEGFR-2 inhibitors faced several drawbacks represented in their serious adverse reactions and development of a secondary resistance.10 There drawbacks motivated the medicinal chemists to continue searching for new small molecules aiming to overcome the adverse effects and minimize resistance.11–14
Observing the structure–activity relationship of the FDA approved VEGFR-2 inhibitors revealed that they almost shared four common features namely, (a) a heterocyclic head with minimum one nitrogen atom to occupy the hinge region of the active site, (b) a spacer to occupy gatekeeper region, (c) a hydrogen bonding moiety or a pharmacophore that makes essential H-bonds with the DFG amino acids, and (d) a hydrophobic tail that fills the receptor's allosteric site15–20 (Fig. 1A). However, Fig. 1B illustrated the role of sorafenib in inhibiting the VEGFR-2-mediated angiogenesis effect.
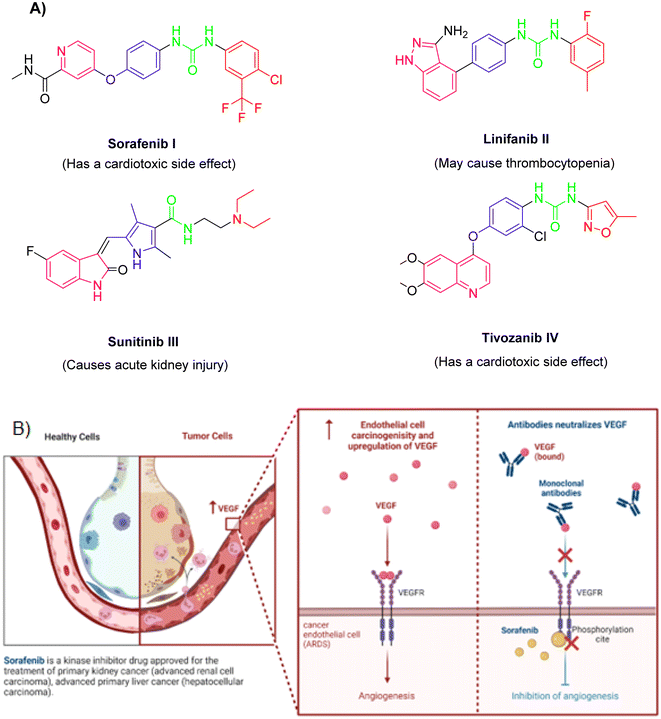 |
| Fig. 1 (A) Some reported VEGFR-2 inhibitors and their necessary four parts. (B) Function of VEGFR-2 and effects of sorafenib. | |
In light of the abovementioned facts, we were encouraged to build a new scaffold constructed from the four key reported VEGFR-2 inhibitors features. The rationale of our molecular design depended basically on the bioisosteric modification of the well-defined VEGFR-2 inhibitors at their four main parts. However, the 6-aryl-5-cyano-thiouracil moiety was used in the current as a heterocyclic head. The choice of such a moiety was suitable as it contains a pyrimidine core that can fill the large size space of the ATP binding region. Besides, the pyrimidine's nitrogen atoms may serve as H-bond acceptors that may potentiate binding of the designed compounds with the VEGFR-2 receptor. Supporting with the structure of sorafenib I, the potent VEGFR-2 inhibitor, we have designed the spacer part of the target members to share the same length of that of sorafenib. The spacer part, hence, consisted of a four-carbon bridge attached to an NH group which are directly linked to heterocyclic head. The length of the designed linker was then convenient to occupy the gate keeper region in VEGFR-2 pocket (Fig. 2).
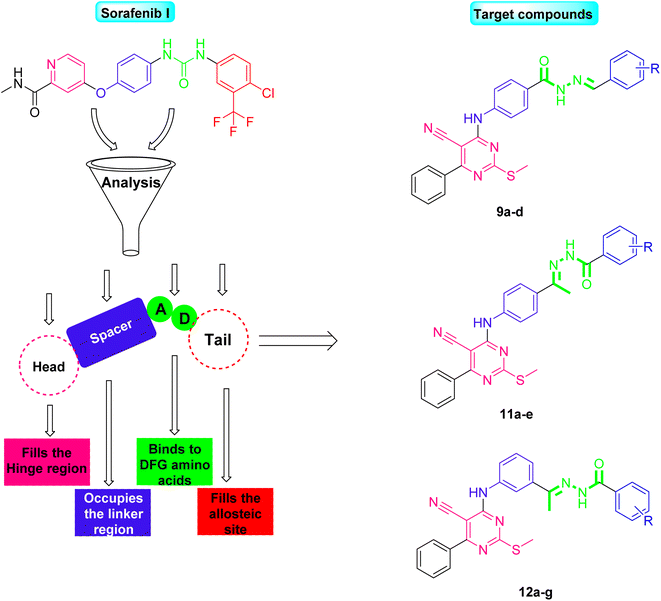 |
| Fig. 2 Rationale of molecular design for synthesis of novel VEGFR-2 inhibitors. | |
Two different hydrophilic moieties were selected in the current study to play the role of the compounds' pharmacophore part. The choice of the moieties was based on their ability to act either as H-bond donor or acceptor. Thus, they could bind to the two essential receptor's amino acids, Glu883 and Asp1044. The final position to be modified was the terminal hydrophobic tail. Herein, we fixed the sorafenib phenyl hydrophobic tail with a change in its substitutions as we selected a wide variety of substitutions that enabled us to study the SAR of the designed compounds against VEGFR-2 tyrosine kinase receptor (Fig. 2).
2. Results and discussion
2.1. Chemistry
Chemical reactions for the synthesis of the target derivatives were depicted in Schemes 1–3. First, the starting material 2-mercapto-6-oxo-4-phenyl-1,6-dihydropyrimidine-5-carbonitril 1 was obtained in a good yield by the cyclocondensation of benzaldehyde with ethyl cyanoacetate and thiourea in alkaline media according to the reported method.21–23 The derivative 1 was subjected to the alkylation with an equivalent amount of methyl iodide in 10% alcoholic KOH to afforded 2-(methylthio)-6-oxo-4-phenyl-1,6-dihydropyrimidine-5-carbonitrile 2. Thus, refluxing of compound 2 with phosphorus oxychloride afforded the corresponding the key intermediate 3. The latter 3 was refluxed in n-butanol with the commercially available aromatic amines namely, 4-aminobenzoic acid, 4-aminoacetophenone, and 3-aminoacetophenone to yield the corresponding target intermediates 4, 5, and 6, respectively (Scheme 1).
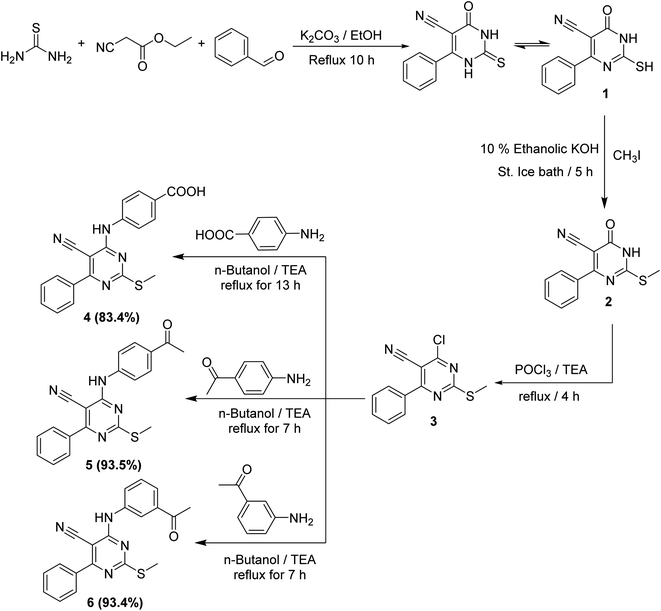 |
| Scheme 1 General procedure for synthesis of intermediate compounds 4, 5, and 6. | |
The 1H NMR analyses of such intermediates (4, 5, and 6) showed three exchangeable signals at δ 10.12, 10.15, and 10.10 ppm corresponding to the NH protons, respectively. Besides, the 1H NMR spectra of 5 and 6 exhibited characteristic singlet signal for COCH3 group resonating at rang of δ 2.53–2.58 ppm.
In order to accomplish the target final compounds, the intermediate 4 was firstly activated by refluxing with thionyl chloride according to the reported method16 to achieve the acid chloride derivative 7 which was then utilized as freshly prepared without further purification to react with hydrazine hydrate in absolute ethanol to afford acid hydrazide analog 8. Such reaction was performed using an ice bath to avoid desulfurization which may take place through the attack hydrazine hydrate on the SCH3 group. The evidences for the formation of the acid hydrazide was verified by spectral data. The IR spectrum displayed strong bands at 3296, 3202 and 1658 cm−1 corresponding to NH, NH2 and C
O groups, respectively. 1H NMR spectrum revealed appearance of a broad singlet signal at δ 4.72 ppm that refers to NH2 protons and another two singlet signals of two NH groups at δ 9.97 and 9.74 ppm. Then, the hydrazide 8 was allowed to react with appropriate aldehydes at refluxing ethanol temperature in the presence of a catalytic amount of glacial acetic acid to obtain the respective imine compounds or Schiff's bases 9a–d (Scheme 2).
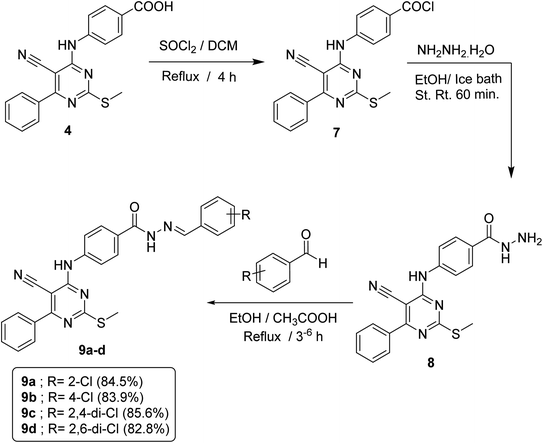 |
| Scheme 2 General procedure for synthesis of target compounds 9a–d. | |
IR spectra of these compounds showed the disappearance of NH2 absorption band of the hydrazide compound 8. On the other hand, 1H NMR spectra of such compounds displayed a down-field singlet signal at a range of δ 8.84–8.00 ppm that refers to the new benzylidene proton, in addition to an increase in the aromatic protons by five, four or three protons according to the aldehyde used.
Condensation of the keton derivatives 5 and 6 with the appropriate acid hydrazides 10a–g (obtained according to the reported procedures)16,23,24 at heating and refluxing temperature in absolute ethanol containing catalytic amount of glacial acetic acid afforded the respective hydrazono compounds 11a–e and 12a–g, respectively (Scheme 3).
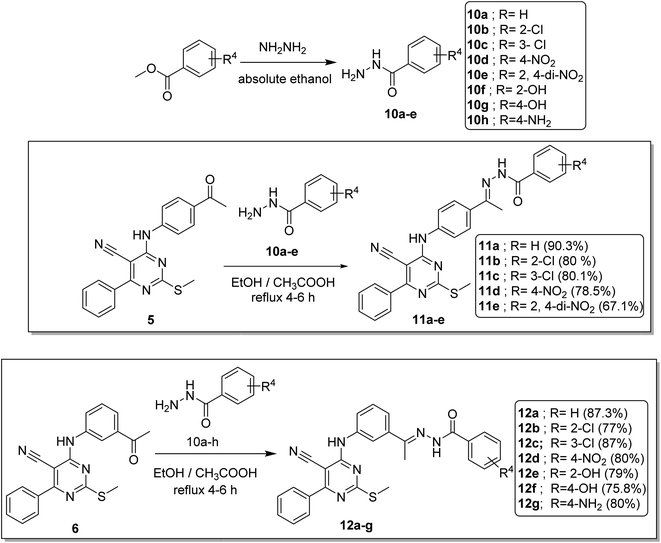 |
| Scheme 3 General procedure for synthesis of target compounds 11a–e and 12a–g. | |
IR spectra of 11a–e and 12a–g series confirmed presence of the carbonyl absorption band at a range of 1666–1637 cm−1 and NH bands at a range of 3341–3196 cm−1. On the other hand, the 1H NMR spectra showed up-field singlet signals of the aliphatic SCH3 and CH3 protons at δ range of 2.51–2.42 ppm and 2.44–2.20 ppm as well as the presence of NH protons at a δ range of 11.13–9.90 ppm. An important point was noticed regarding a couple of compounds, 11b and 12b, as the 1H NMR analyses revealed that they existed as E/Z isomers. This conclusion was reached through the investigation of signaling patterns of two methyl groups and two NH groups. For compound 11b, the two isomers appeared as about 60%
:
40%. While, for compound 12b, the two isomers appeared as about 65%
:
35%. Complete details for signaling ratio of each signal group were illustrated in ESI (Table S-1 and 1H NMR charts).† Moreover, the 13C NMR spectra of such series showed signals of the two methyl carbons at δ range of 19.20–13.77 ppm.
2.2. Biological evaluation
2.2.1. In vitro growth inhibitory activity against HCT-116 and MCF-7 cell lines. The target derivatives were assessed for their in vitro anti-proliferative activities of against two overexpressed VEGFR-2 cells; colon cancer (HCT-116), and breast cancer (MCF-7) cell lines via MTT assay method.25–27 Results of the in vitro evaluation against HCT-116 and MCF-7 cell lines revealed that these compounds possessed high inhibitory activity towards the two cell lines with IC50 ranges of 1.14–32.16 μM. Four out of the thirteen target compounds (9d, 11e, 12b, 12d) showed higher cytotoxic activity than the standard sorafenib (IC50 = 8.96 and 11.83 μM) with IC50 values ranging from 1.14 to 10.33 μM against HCT-116 and MCF-7, respectively. In particular, compound 11e exhibited excellent activities against HCT-116 and MCF-7 with IC50 values of 1.14 and 1.54 μM, respectively. Moreover, four compounds such as 9b, 11b, 11c, 11d, and 12c showed remarkable activities against two cell lines with IC50 values ranging from 9.25 to 15.26 μM. These results were found to be a little lower than the standard drug. On the other hand, several compounds showed moderate to weak anti-proliferative activities against the tested cell lines.
2.2.2. In vitro cytotoxicity against normal human lung cells (WI-38). In order to assess the selectivity of the target compounds against cancer cells over normal ones, the cytotoxicity of the most active compound 11e was estimated in vitro against normal human lung cells (WI-38). The obtained results revealed that, compounds 11e exhibited cytotoxic IC50 value of 63.41 ± 0.015 μM against normal human lung cells. These results indicated that its cytotoxicity against normal cells is about 47.32-fold lower than the cytotoxicity against the cancer cells (Table 1).
Table 1 In vitro anti-proliferative activities of the synthesized compounds against HCT-116 and MCF-7 cell lines their VEGFR-2 inhibitory activities on HCT-116 cell line and cytotoxicity for compound 11e against normal human lung cells (WI-38)
Comp. no. |
In vitro cytotoxicity IC50a (μM) |
VEGFR-2a (μM) |
HCT 116 |
MCF-7 |
WI-38 |
Data are presented as mean ± S. D of the IC50 values from two different experiments. NT: not tested. |
9a |
30.15 ± 0.25 |
28.66 ± 0.2 |
NTb |
NTb |
9b |
12.34 ± 0.09 |
15.26 ± 0.12 |
NTb |
NTb |
9c |
20.62 ± 0.15 |
26.75 ± 0.24 |
NTb |
NTb |
9d |
7.14 ± 0.025 |
9.85 ± 0.035 |
NTb |
2.41 ± 0.16 |
11a |
23.58 ± 0.18 |
32.16 ± 0.28 |
NTb |
NTb |
11b |
10.7 ± 0.05 |
13.48 ± 0.1 |
NTb |
1.55 ± 0.25 |
11c |
10.45 ± 0.05 |
10.92 ± 0.05 |
NTb |
1.38 ± 0.03 |
11d |
13.78 ± 0.11 |
14.31 ± 0.15 |
NTb |
2.32 ± 0.21 |
11e |
1.14 ± 0.01 |
1.54 ± 0.01 |
63.41 ± 0.015 |
0.61 ± 0.01 |
12a |
17.98 ± 0.15 |
25.18 ± 0.19 |
NTb |
NTb |
12b |
8.65 ± 0.05 |
9.77 ± 0.06 |
NTb |
0.53 ± 0.07 |
12c |
9.25 ± 0.07 |
10.17 ± 0.05 |
NTb |
0.74 ± 0.15 |
12d |
7.15 ± 0.06 |
10.33 ± 0.05 |
NTb |
1.61 ± 0.18 |
12e |
19.44 ± 0.14 |
30.14 ± 0.26 |
NTb |
NTb |
12f |
21.36 ± 0.15 |
26.11 ± 0.18 |
NTb |
NTb |
12g |
16.39 ± 0.12 |
21.72 ± 0.15 |
NTb |
NTb |
Sorafenib |
8.96 ± 0.05 |
11.83 ± 0.07 |
NTb |
0.19 ± 0.15 |
2.2.3. In vitro VEGFR-2 kinase inhibitory assay. The most active derivatives (9d, 11b, 11c, 11d, 11e, 12b, 12c, and 12d) that exhibited promising anti-proliferative activities were subjected to further investigation for their ability to inhibit VEGFR-2 using sorafenib as a reference VEGFR-2 inhibitor.28,29 Kinase assay protocol was utilized in this test using Human VEGFR-2 TK ELISA kit. The results of VEGFR-2 inhibitory activity revealed that the compounds showed inhibitory activity with IC50 values ranging from 0.53 to 2.41 μM. Compounds 11c, 11e, 12b, and 12c were the most potent VEGFR-2 inhibitors with IC50 values of 1.38 ± 0.03, 0.61 ± 0.01, 0.53 ± 0.07, and 0.74 ± 0.15 μM, respectively, compared with that of sorafenib (IC50 = 0.19 ± 0.15 μM). On the other hand, compounds 9d, 11b, 11d, and 12d showed promising activity towards VEGFR-2 with IC50 values of 2.41 ± 0.16, 1.55 ± 0.25, 2.32 ± 0.21, 1.61 ± 0.18 μM, respectively.
2.2.4. Structure–activity relationships (SAR). As outlined in the rationale molecular design, we aimed at studying the SAR of newly synthesized pyrimidine derivatives as potential VEGFR-2 TK inhibitors. Observing the results of different biological tests (in vitro anti-proliferative activity, measurement of VEGFR-2 TK activity), we could deduce valuable data about the structure–activity relationships.• Comparing the cytotoxicity of compounds containing m-disubstituted linkers (12d, 12b, 12a, and 12g) with their corresponding compounds of the p-disubstituted linkers (11d, 11b, and 11a), respectively, indicated that, the m-disubstituted derivatives are more active than the p-disubstituted ones (Fig. 3).
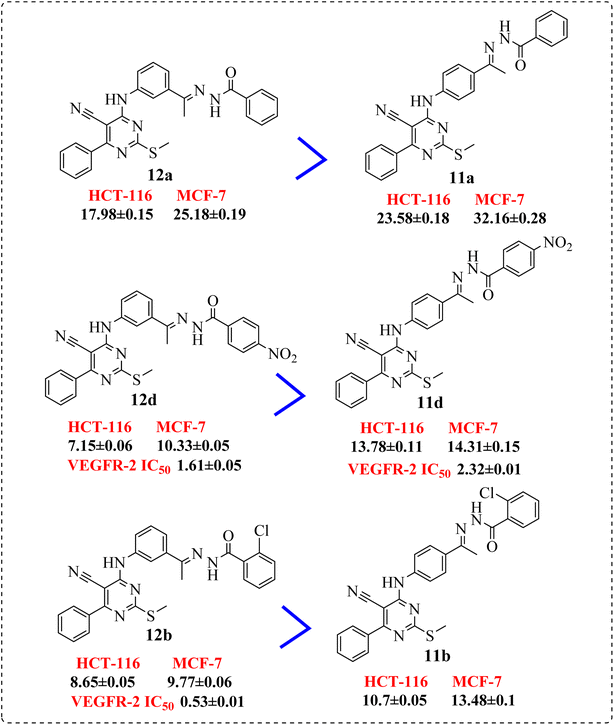 |
| Fig. 3 SAR studies of 12a, 12d, and 12b compared to 11a, 11d, and 11b, respectively. | |
With regard to the benzylidene derivatives (containing hydrazone moiety) 9a–d:
• The p-electron withdrawing substitution derivatives (9b) were more active than these with o-electron withdrawing ones (9a) (Fig. 4).
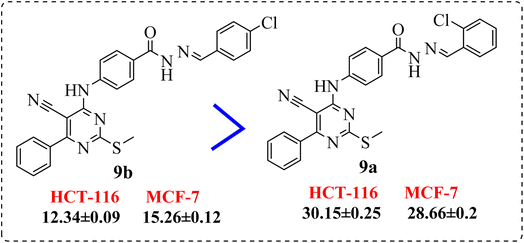 |
| Fig. 4 SAR studies of 9b compared to 9a. | |
• Introduction of a chlorine atom at p-position exhibited preferable activity than o-position. Meanwhile, di-chloro substitution at 2,6-positions (9d) showed an activity stronger than the p-substituted one, but 2,4-dichloro derivative (9c), elicited lower activity than both (Fig. 5).
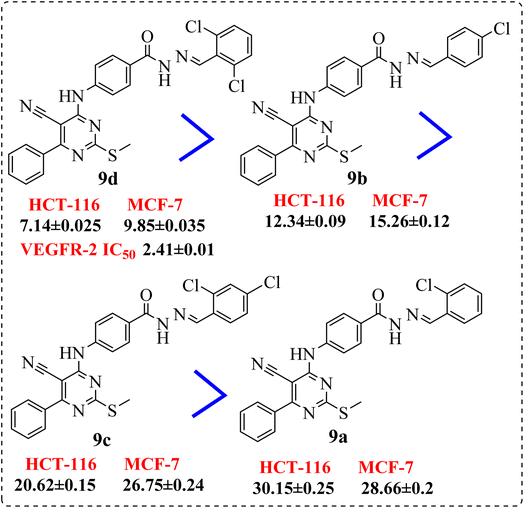 |
| Fig. 5 SAR studies of 9d and 9c compared to 9b and 9a, respectively. | |
• Compound 11e containing di-nitro substitution at 2,4-positions showed the highest cytotoxic activity on the both tested cell lines, moreover, shifting the nitro group from o-position showed a sever decreasing in cytotoxicity and VEGFR-2 TK inhibitory activity (Fig. 6).
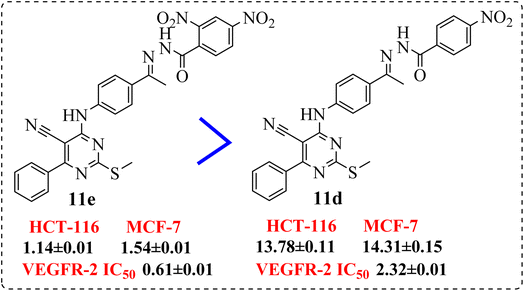 |
| Fig. 6 SAR studies of 11e compared to 11d. | |
• Shifting the chloro group from m-position (11c) to o-position (11b) showed notable decrease in the cytotoxic activity (Fig. 7).
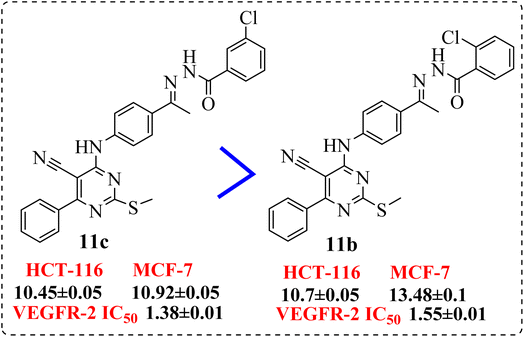 |
| Fig. 7 SAR studies of 11c compared to 11b. | |
Regarding to the derivatives 12a–g containing m-disubstituted linkers, observation of the cytotoxic activity revealed that:
• Compounds 12b and 12d, containing terminal hydrophobic tail substituted with electron withdrawing groups with their corresponding derivatives with electron donating ones 12e and 12g, indicated the derivatives of electron withdrawing groups were more active than those donating one (Fig. 8).
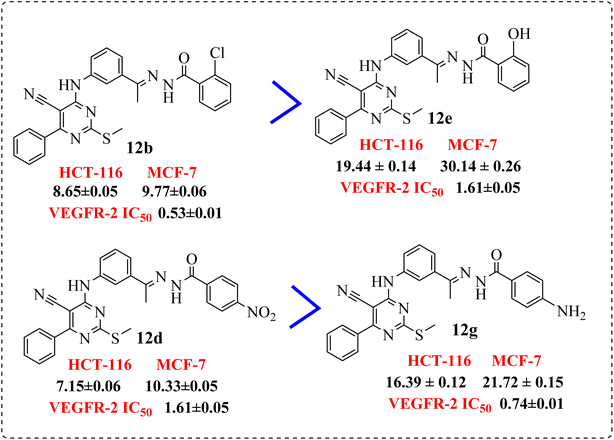 |
| Fig. 8 SAR studies of 12b and 12d compared to 12e and 12g, respectively. | |
• Introduction of electron withdrawing group at o-position and m-position exhibited preferable activity than p-position (Fig. 9).
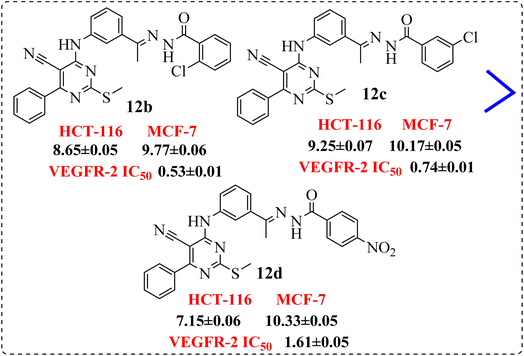 |
| Fig. 9 SAR studies of 12b and 12c compared to 12d. | |
2.2.5. Effect on cell cycle progression. To investigate the effect of the most promising compound 11e on the various phases of the cell cycle in HCT-116 cells, flow cytometric analysis of cell cycle was performed using Epics XL-MCL™ Flow Cytometer22,30 according to flow cytometric analysis technique.31 The results obtained after exposure of HCT-116 cells to compound 11e (as presented in Table 2, Fig. 10 and 11) indicated that the percentage of HCT-116 cells decreased at the G0/G1 and G2/M phases. For G0/G1 phase, it decreased from 54.18% in control cells to 41.36%. At the G2/M phase, it decreased from 32.33% in the untreated cells to 22.43% in treated cells. On the contrary, the percentage of HCT-116 cells increased at Sub-G1 and S phases. For Sub-G1 phase, it increased from 2.70% in control cells to 21.36% (7.91-fold) in treated cells. For S phase, it increased from 13.49% in control cells to 36.21% (2.68-fold). These findings indicated that compound 11e mainly arrested the cell cycle at S phase and induced apoptosis at Sub-G1 phase. Such findings were coordinated with the recorded results which established that the VEGFR-2 TK inhibitors can arrest the cell growth at S and Sub-G1 phases.32
Table 2 Effect of compound 11e on cell cycle progression in HCT-116 cells
Sample |
Cell cycle distributiona (%) |
% Sub-G1 |
% G0/G1 |
% S |
% G2/M |
Values are given as mean of twice independent experiments. |
Cont./HCT-116 |
2.70 |
54.18 |
13.49 |
32.33 |
Compound 11e/HCT-116 |
21.36 |
41.36 |
36.21 |
22.43 |
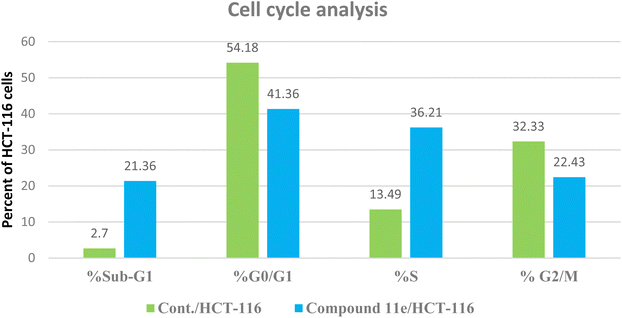 |
| Fig. 10 The representative histograms show the cell cycle distribution of control (HCT-116), and cells treated with compound 11e. | |
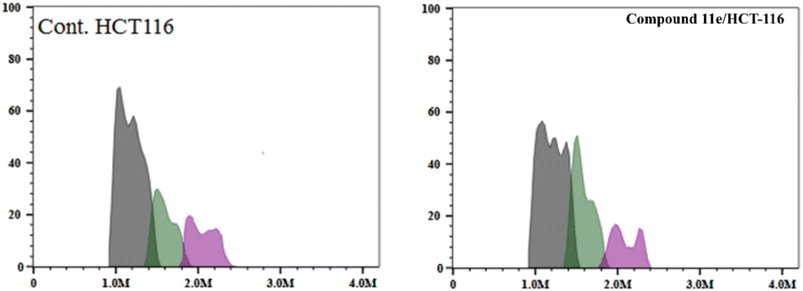 |
| Fig. 11 Flow cytometric analysis of cell cycle phases post the compound 11e treatment. | |
2.2.6. Induction of apoptosis. Flow cytometry cell apoptosis analysis was used to investigate the apoptotic effect of the most active candidate on the treated HCT-116 cells. Apoptotic effect of compound 11e on the treated HCT-116 cells was analyzed with the aid of annexin V-FITC-apoptosis detection kit (PN IM3546) using Epics XL-MCL™ Flow Cytometer.30,33 The results of apoptosis induction (as represented in Table 3, Fig. 12 and 13) indicated that exposure of HCT-116 cells to compound 11e resulted in a significant increase in the percentage of apoptotic cells in the early stage from 0.85% (untreated control cells) to 5.47%. Furthermore, in the late phase, there was an increase in the apoptotic cells to attain 15.70% in comparison to 1.31% in control HCT-116 cells.
Table 3 Effect of compound 11e on stages of the cell death process in HCT-116 cells
Sample |
Viablea (left bottom) |
Apoptosisa |
Necrosisa (left top) |
Early (right top) |
Late (right bottom) |
Values are given as mean of twice independent experiments. |
Cont./HCT-116 |
97.30 |
0.85 |
1.31 |
0.54 |
Comp. 11e/HCT-116 |
78.64 |
5.47 |
15.70 |
0.19 |
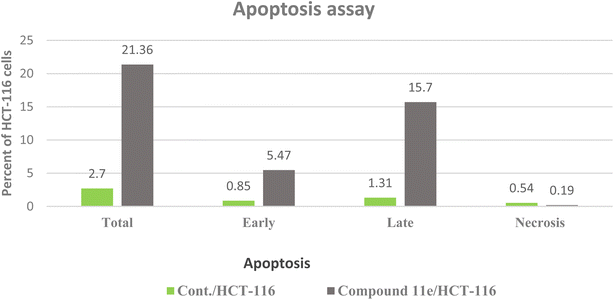 |
| Fig. 12 The representative flow cytometric charts for control (HCT-116) and the cells treated with compound 11e. | |
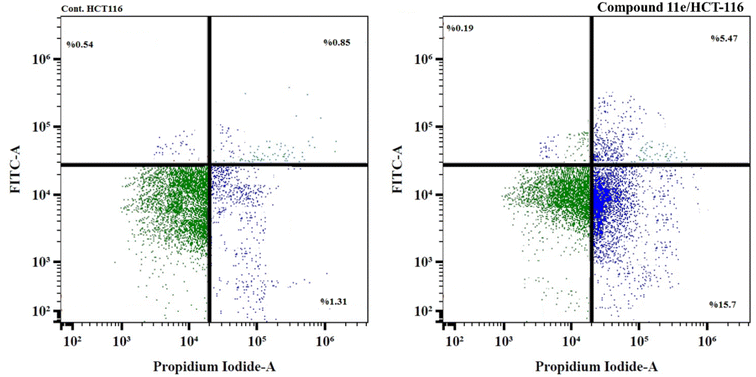 |
| Fig. 13 Flow cytometric analysis of apoptosis in HCT-116 cells exposed to compound 11e. For each dot plot chart, the quadrant regions represent the cells in each sub-population; lower left quadrant (viable cells), lower right quadrant (late apoptosis), upper right quadrant (early apoptosis), and upper left quadrant (necrosis). | |
2.2.7. In vitro immunomodulatory assay of TNF-α and IL-6. The selected compound 11e, was further evaluated for its effects on the expression levels of TNF-α and IL-6 in HCT-116 cell line. Dexamethasone was used as positive controls as included in literature.8 The effects of compound 11e on the expression of TNF-α and IL-6 were determined using qRTPCR technique.34 The quantity of immunomodulatory proteins (TNF-α and IL-6) in control and compound 11e (at the IC50 concentration)-treated HCT-116 cells was assessed by qRTPCR (reference).In these experiments, we calculated the changes in the expression levels of such proteins that resulted from the treatment of HCT-116 cells for 24 h with the selected molecule at their IC50. The data outlined in (Table 4) showed the significance of compound 11e as potent inhibitor for the pro-inflammatory factors TNF-α and IL-6, which played main role in immunomodulatory activity (Fig. 14). Compound 11e caused remarkable decrease in the levels of TNF-α and IL-6, with inhibition percent 70.22 pg per mL % for TNF-α & 81.39, 79.52 and 84.46 pg per mL % for IL-6, respectively.
Table 4 Immunomodulatory assay of the compound 11e against the pro-inflammatory factors TNF-α and IL-6
Sample |
Pro-inflammatory factors inhibition (pg per mL %) |
TNF-α |
IL-6 |
Dexamethasone |
82.47 |
93.15 |
Compound 11e/HCT-116 |
70.22 |
84.46 |
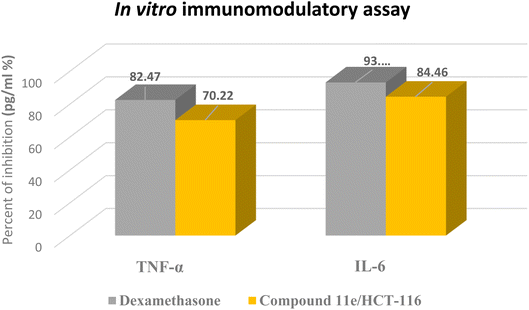 |
| Fig. 14 The representative in vitro immunomodulatory assay charts for dexamethasone as positive control and the tested compound 11e. | |
2.2.8. In vitro assessment of caspase-3 expression. The effect of the selected candidate 11e on the expression level of caspase-3 in HCT-116 cells was evaluated in comparison to HCT-116 cells treated with DMSO represented as a negative control. As can be seen from (Table 5), the tested molecule was comparable to control in its effects on caspase-3 levels. Compound 11e showed a notable increase in caspase-3 concentration (304.67 pg mL−1) by 5.4 folds, compared to the negative control (see Fig. 15).
Table 5 Caspase-3 expression analysis of the tested compound 11e as apoptotic inducer
Sample |
Apoptosis markers assay (pg mL−1) |
Caspase-3 |
Fold |
Cont./HCT-116 |
56.48 |
1 |
Compound 11e/HCT-116 |
304.67 |
5.4 |
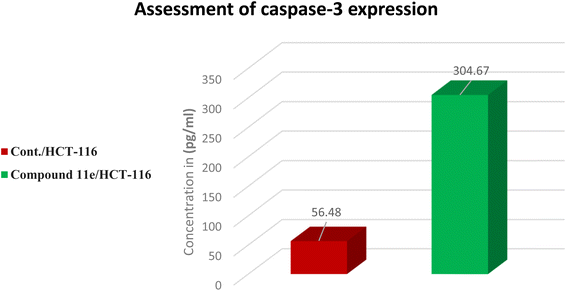 |
| Fig. 15 The representative in vitro caspase-3 expression chart for the tested compound 11e. | |
2.3. In silico studies
2.3.1. Docking study. Docking study was performed to get insight into how the designed compounds could bind to the ATP binding site of VEGFR-2 TK and inhibit its downstream signaling pathways. The results of these studies could help us to understand the binding modes of the target compounds with the crucial amino acids in the ATP binding site of VEGFR-2 TK (Table 6). In these studies, the free energies, and binding modes of the designed molecules against the VEGFR-2 TK active site were determined. Molecular Operating Environment (MOE 19.0102) software was used for the docking analysis and Biovia discovery studio 2016 was used for the visualization. Validation of the docking protocol was performed through the re-docking of the co-crystalized ligand inside the active site of VEGFR-2 TK. The resulted RMSD between the re-docked and the co-crystallized ligand was 0.49 Å which indicated the validity of the docking protocol (Fig. 16).
Table 6 The calculated ΔG (binding free energies) of the synthesized compounds and reference drug against VEGFR-2 TK (ΔG in kcal mol−1)
Comp. |
ΔG [kcal mol−1] |
Comp. |
ΔG [kcal mol−1] |
9a |
−8.70 |
12a |
−8.95 |
9b |
−8.23 |
12b |
−9.17 |
9c |
−8.95 |
12c |
−8.99 |
9d |
−8.85 |
12d |
−9.01 |
11a |
−9.32 |
12e |
−9.34 |
11b |
−8.82 |
12f |
−8.60 |
11c |
−9.06 |
12g |
−8.90 |
11d |
−9.30 |
Sorafenib |
−8.70 |
11e |
−9.36 |
|
|
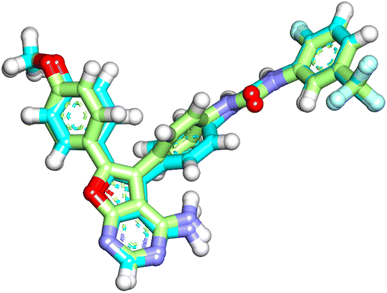 |
| Fig. 16 Superimposition of the native ligand (turquoise) and the re-docked one (green) in the VEGFR-2 TK active site (RMSD = 0.49 Å). | |
The native ligand was exhibited an affinity score of −10.90 kcal mol−1 with RMSD value equal 0.49 Å (Fig. 17). It showed many important interactions with the residues at the active site of VEGFR-2 TK. The urea side chain possessed both hydrogen bond acceptor and donor, which bound with two crucial residues (Glu883 and Asp1044) of the receptor, where the NH motifs of the urea moiety usually formed two hydrogen bonds with the carboxylate of Glu883 with distance of 2.01 and 1.94 Å. Whereas the C
O motif formed another hydrogen bond with the backbone NH of with Asp1044 with a distance of 2.05 Å at DFG region. The heterocyclic moiety showed two extra hydrogen bonds with Glu915 and Cys917. Besides, the phenyl group (spacer) interacted with the hydrophobic site (Val914, Ala864, Cys1043 and Val846) in the linker region by pi interactions. These results were found to be identical to the reported data.35
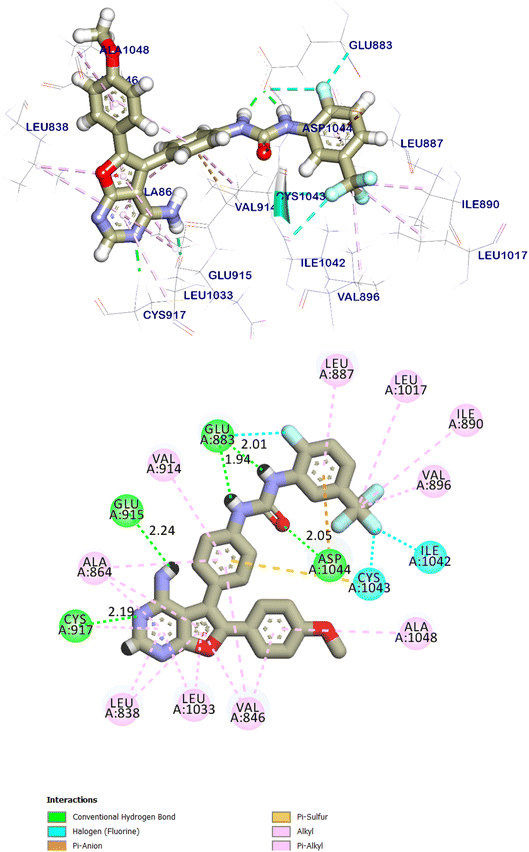 |
| Fig. 17 The predicted binding mode for the co-crystalized ligand with the active site of VEGFR-2 TK. | |
Sorafenib interactions with the amino acids of the pocket have been studied and displayed in 2D and 3D style in (Fig. 18). The proposed binding mode of sorafenib, revealed an affinity value of −8.70 kcal mol−1. It demonstrated the important interactions with the residues at the active site of VEGFR-2 TK. The urea moiety possessed both hydrogen bond acceptor and donor that bound with two crucial residues (Glu883 and Asp1044) of the receptor, where the NH motifs of the urea moiety formed two hydrogen bonds with the carboxylate of Glu883 with distances of 2.02 and 1.98 Å. Whereas the C
O motif formed another hydrogen bond with the backbone NH of with Asp1044 with a distance of 2.03 Å at DFG region. Besides, the phenyl group (spacer) interacted with the hydrophobic site (Cys917, Cys1043 and Phe916) in the linker region. Moreover, the distal hydrophobic moiety attached to the urea linker occupied the hydrophobic pocket formed by Cys1043, Val896, Asp1044, Ile886, Ile890, Ile1042, and Leu887. Furthermore, the N-methylpicolinamide moiety occupied the hinge region, it was formed one hydrogen bond with Cys917 with a distance of 2.79 Å and pi interactions with Ala864, Phe916, Leu838, Leu1033 and Cys917 (Fig. 18). The urea linker played an important role in the binding affinity towards VEGFR-2 TK, which was responsible for the higher binding affinity of sorafenib. These results were found to be identical to the reported data.36
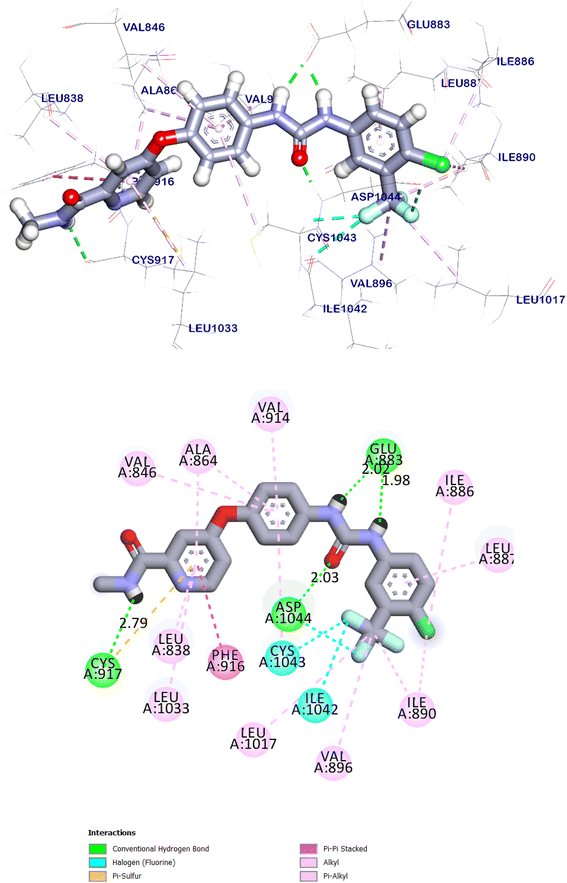 |
| Fig. 18 The predicted binding mode for sorafenib with the active site of VEGFR-2 TK. | |
The docking score for compound 9d was −8.85 kcal mol−1. Through its pharmacophoric group, it formed two hydrogen bonds with Glu883 and Asp1044. The spacer moiety (4-aminophenyl ethylidene) interacted hydrophobically with Val914, and Cys1043. Many hydrophobic interactions in the hinge region occurred between the 5-cyano-2-(methylthio)-6-phenyl-pyrimidin moiety and different amino acid residues including Leu838, Val846, Gly1046, Phe843, and Ala1048. Furthermore, in the allosteric pocket, the 2,6-dichloro phenyl group formed numerous hydrophobic interactions with Val896, Val897, Ile890, Ile886, Phe1045 and Leu887. It also had a pi–anion interaction with Asp1044 (Fig. 19).
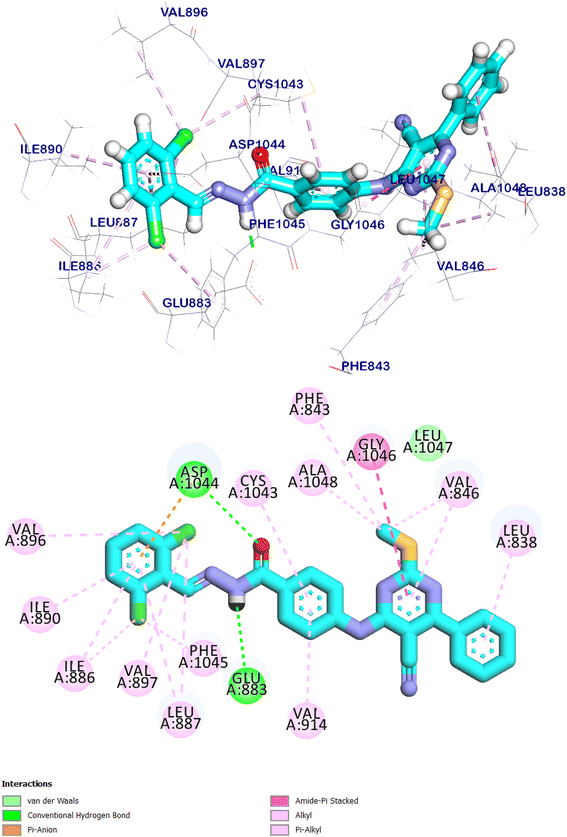 |
| Fig. 19 The predicted binding mode of compound 9d with the active site of VEGFR-2 TK. | |
The docking findings of compound 11e revealed that it can interact with the essential amino acids in the VEGFR-2 TK active site. As displayed in (Fig. 20), it was showed docking energy of −9.36 kcal mol−1. Both 4-amino phenyl and 5-cyano-2-(methylthio)-6-phenylpyrimidin moieties were oriented towards the gate keeper area and the hinge region, respectively, which bound with Ala1048 and Arg1049 by two hydrogen bonds with distances 2.75 and 2.80 Å, additionally, it was interacted with Val846, Ala864, Lys866, Val914, Cys1043, Ala1048 and Arg1049 by seven hydrophobic interactions. Also, compound 11e incorporated in two hydrogen bonds with the key amino acids; Glu883 (2.18 Å) and Asp1044 (2.52 Å) in the DFG motif. Additionally, the of the later moieties' orientation allowed the hydrophobic substituents in the docked compound to fit inside the hydrophobic allosteric pocket. Such a binding pattern of compound 11e encouraged us to study its MD simulation.
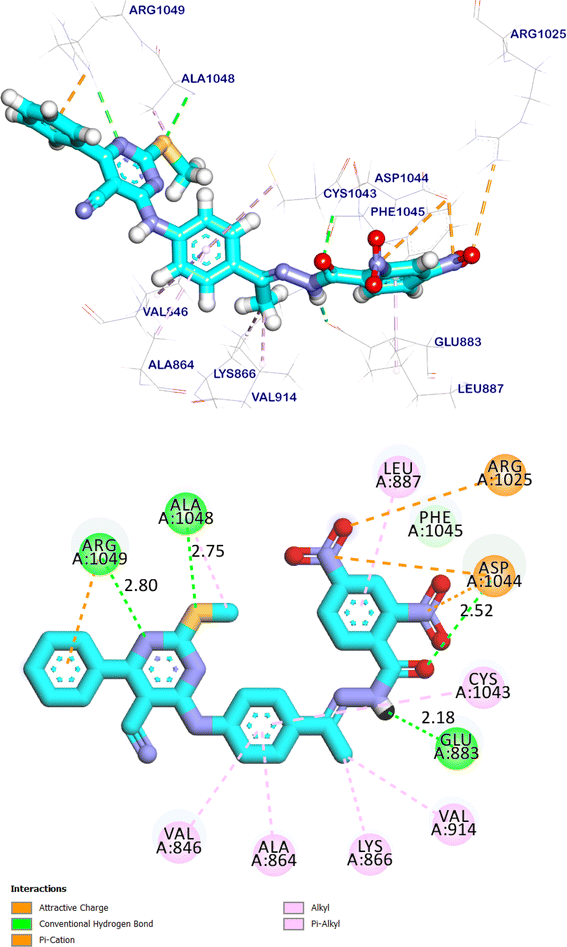 |
| Fig. 20 The predicted binding mode of compound 11e with the active site of VEGFR-2 TK. | |
Compound 12a showed docking energy of −8.95 kcal mol−1. The 5-cyano-2-(methylthio)-6-phenylpyrimidin moiety formed seven hydrophobic interactions in the hinge region with Arg1049, Ala1048, Leu1047, Leu1033, and Cys1043. Additionally, it was formed two hydrogen bonds with Arg1049 and Arg1030. The 4-amino phenyl (linker) moiety formed an extra hydrogen bond with Gly1046 in addition to two hydrophobic interactions with Val846, and Ala864. The pharmacophore moiety occupied the DFG region forming two hydrogen bonds with Glu883 Asp1044. The terminal hydrophobic moiety occupied the allosteric pocket forming four hydrophobic interactions with Leu887, Lys866, Asp1044, and Val917 (Fig. 21).
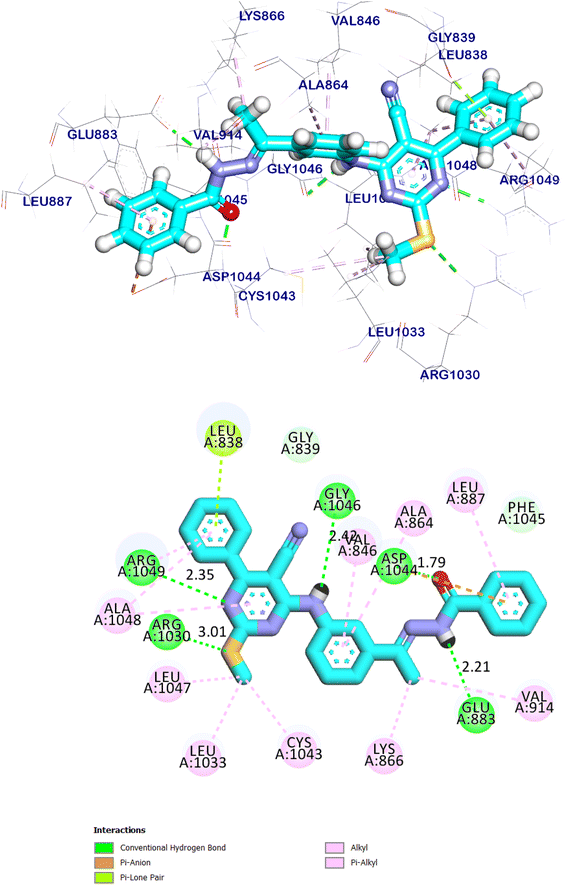 |
| Fig. 21 The predicted binding mode of compound 12a with the active site of VEGFR-2 TK. | |
The net result of computational studies of the designed molecules indicates that most of the synthesized compounds showed significant VEGFR-2 TK inhibition affinities.
Comparing to the binding modes of the reference drugs, we found a lot of compounds have excellent binding modes with ΔG values higher than or at least similar to that of the reference drugs (sorafenib), against VEGFR-2 TK with ΔG values ranging from −8.23 to −9.36 compared with sorafenib ΔG value −8.70. Other compounds showed binding affinities lower than that of the reference drugs. The results may give guidance for further structural modifications.
The p-di-substituted and m-di-substituted benzohydrazide derivatives 11a–e and 12a–g exhibited a higher ability to form hydrogen bonds interaction than other groups, which explains their higher cytotoxic activity. Specifically, compounds with benzohydrazide side chains, such as 11e, 11b, 11d, 11c, 12b, 12d, and 12c, showed high affinity toward VEGFR-2 TK with (ΔG −9.36, −8.82, −9.30, −9.06, −9.17, −9.01, and −8.99), respectively, and these results were consistent with the cytotoxicity MTT assay. Furthermore, the series with a benzylidene side chain exhibited a remarkable affinity score against VEGFR-2 TK, such as compounds 9c which had affinity score (−8.95 kcal mol−1) higher than sorafenib (−8.70 kcal mol−1).
2.3.2. Molecular dynamic (MD) simulations. The dynamic movements of atoms and conformational variations of backbone atoms of the protein–ligand complex were calculated by RMSD to detect their stability upon apo and ligand bonded state. It was observed that the protein, ligand, and the complex exhibited a lower RMSD values with no major fluctuations throughout the simulation, indicating their greater stability. The flexibility of each residue was calculated in terms of RMSF to get better insight on the region of proteins that are being fluctuated during the simulation. It can be understood that the binding of ligand makes the protein flexible in 1025–1075 residue areas. The compactness of the complex was represented by the radius of gyration (Rg). The lower degree of fluctuation throughout the simulation period indicates the greater compactness of a system. The Rg of the complex was found to be similar compared to the starting period. Interaction between protein–ligand complexes and solvents was measured by solvent accessible surface area (SASA) over the simulation period. So, SASA of the complex was calculated to analyze the extent of the conformational changes occurred during the interaction. Interestingly, the protein featured neither reduction nor expansion of the surface area showing relatively similar SASA value than the starting period. Hydrogen bonding between a protein–ligand complex is essential to stabilize the structure. It was observed that the highest number of conformations of the protein formed up to two hydrogen bonds with the ligand (Fig. 22).
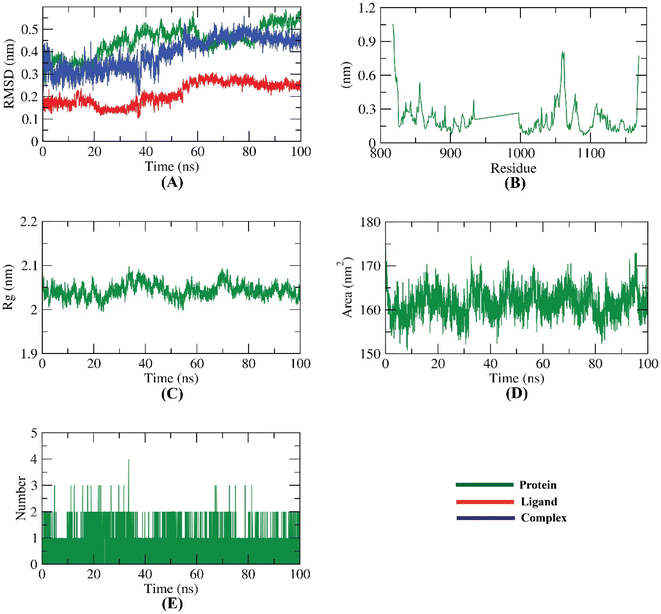 |
| Fig. 22 MD simulations; (A) RMSD, (B) RMSF (C) Rg (D) SASA, and (E) hydrogen bonding for compound 11e-VEGFR-2 TK complex over the MD run (100 ns). | |
2.3.3. Molecular mechanics Poisson–Boltzmann surface area (MM-PBSA). We calculated the binding free energy of the last 20 ns of MD production run of the protein–ligand complex with an interval of 100 ps from MD trajectories using MM/PBSA method. We also utilized the MmPbSaStat.py script that calculated the average free binding energy and its standard deviation/error from the output files that were obtained from g_mmpbsa. The ligand showed binding free energy of −139 kJ mol−1 with the protein. Further, we identified the contribution of each residue of the protein in terms of binding free energy to the interaction with the ligand. By decomposing the total binding free energy of the system into per residue contribution energy, the contribution of each residue was calculated. This gave us an insight into the ‘crucial’ residues that contributes favorably to the binding of this molecule to the protein. It was found that Leu838, Val846, Ala864, Ile886, Leu887, Val897, Val914 and Leu1033 residues of the protein contributed higher than −5 kJ mol−1 binding energy and thereby are hotspot residues in binding with the ligand (Fig. 23).
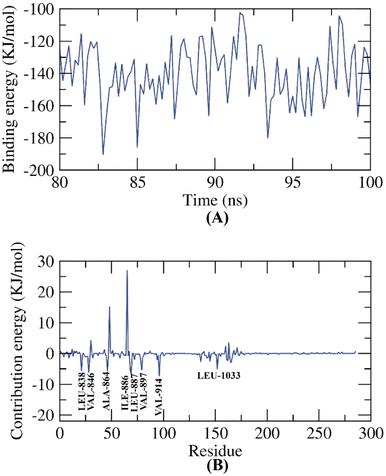 |
| Fig. 23 MM-PBSA outputs of the compound 11e-VEGFR-2 TK complex. | |
3. Conclusion
A new sixteen pyrimidine-5-carbonitrile derivatives were designed and synthesized as VEGFR-2 TK inhibitors. The synthesized compounds were evaluated in vitro for their anti-proliferative activities against colon cancer (HCT-116), and breast cancer (MCF-7) cell lines. Compounds 9b, 9d, 11b, 11c, 11e, 12b, 12c, and 12d exhibited the highest activities towards both cell lines. In particular, compound 11e exhibited the best cytotoxic activity against HCT-116 and MCF-7 with IC50 values of 1.14 ± 0.01 and 1.54 ± 0.01 μM, respectively, compared to sorafenib as a reference drug with IC50 values of 8.96 ± 0.05 & 11.83 ± 0.07 μM, respectively. In addition, the most active cytotoxic agents 11e was further assessed for their inhibitory action on VEGFR-2 TK activity to confirm the mechanism responsible for their induced cytotoxic activities. The in vitro analysis of VEGFR-2 TK inhibition revealed that, compounds 11e and 12b were the most potent VEGFR-2 TK inhibitors with an IC50 values of 0.61 ± 0.01 and 0.53 ± 0.07 μM, respectively, in comparing with that of sorafenib (IC50 = 0.19 ± 0.15 μM). Moreover, compound 11e arrested the cell growth in HCT-116 cells at S and sub-G1 phase and induced a significant increase in the apoptotic cells. Finally, the binding patterns of the target derivatives were investigated through the docking study against the proposed molecular target (VEGFR-2 TK, PDB ID 1YWN). The results of molecular docking studies showed similar binding modes and interactions to sorafenib against VEGFR-2 TK active site.
4. Experimental
4.1. Chemistry
Compounds, 4-oxo-6-phenyl-2-thioxo-1,2,3,4-tetrahydropyrimidine-5-carbonitrile 1,21,22 2-(methylthio)-6-oxo-4-phenyl-1,6-dihydro-pyrimidine-5-carbonitrile 2,37 4-chloro-2-(methylthio)-6-phenylpyrimidine-5-carbonitrile 3,37 substitutedbenzohydrazide 10a–e24,38–40 were prepared according to the reported procedures.
4.1.1. General procedure for the synthesis of compounds 4, 5 & 6. To a solution of compound 3 (2.61 g, 0.01 mol) in n-butanol (15 mL) containing few drops of TEA, the appropriates amines namely p-amino benzoic acid, p-amino acetophenone, and m-amino acetophenone (0.01 mol) were added. The mixture was heated under reflux for sufficient time. After completion of reaction (monitored using TLC), the reaction mixture was concentrated, cooled, and poured onto ice water (50 mL). Then, the solution was acidified with dil. HCl with continuous stirring for 15 min. The formed precipitate was filtered, washed with water several times and dried. The cropped powder was crystallized from ethanol to afford the target compounds 4, 5, & 6, respectively.
4.1.1.1. 4-[(5-Cyano-2-(methylthio)-6-phenylpyrimidin-4-yl)amino]benzoic acid 4. Off-white powder (yield 83.4%, 3.1 g); mp = 273–274 °C; IR (KBr) ν cm−1: 3451 (OH), 3319 (NH), 2975 (CH aromatic), 2928 (CH aliphatic), 2210 (C
N), 1696 (C
O), 1614 (C
N); 1H NMR (400 MHz, DMSO-d6) δ ppm: 12.85 (s, 1H, OH), 10.12 (s, 1H, NH), 7.96 (d, J = 8.5 Hz, 2H, Ar–H, H-3 & H-5 of –C6H4), 7.90 (d, J = 6.5 Hz, 2H, Ar–H, H-2 & H-6 of –C6H5), 7.79 (d, J = 8.5 Hz, 2H, Ar–H, H-2 & H-6 of –C6H4), 7.65–7.57 (m, 3H, Ar–H, H-3, H-4 & H-5 of –C6H5), 2.51 (s, 3H, SCH3); 13C NMR (101 MHz, DMSO-d6) δ ppm: 174.72, 168.71, 167.38, 160.39, 142.53, 136.24, 131.78, 130.24 (2C), 129.25 (2C), 129.06 (2C), 126.81, 122.93 (2C), 116.40, 85.84, 14.30; molecular formula C19H14N4O2S (362.41).
4.1.1.2. 4-[(4-Acetylphenyl)amino]-2-(methylthio)-6-phenylpyrimidine-5-carbonitrile 5. Beige fluffy powder (yield 93.5%, 3.36 g); mp = 236–235 °C; 1H NMR (400 MHz, DMSO-d6) δ ppm: 10.15 (s, 1H, NH), 7.99 (d, J = 8.5 Hz, 2H, Ar–H, H-3 & H-5 of –C6H4), 7.90 (d, J = 6.2 Hz, 2H, Ar–H, H-2 & H-6 of –C6H5), 7.83 (d, J = 8.4 Hz, 2H, Ar–H, H-2 & H-6 of –C6H4), 7.66–7.58 (m, 3H, Ar–H, H-3, H-4 & H-5 of –C6H5), 2.58 (s, 3H, COCH3), 2.52 (s, 3H, SCH3); 13C NMR (101 MHz, DMSO-d6) δ ppm: 197.21, 174.73, 168.73, 160.34, 142.88, 136.23, 133.11, 131.80, 129.30 (2C), 129.26 (2C), 129.07 (2C), 122.73 (2C), 116.40, 85.99, 27.05, 14.34; molecular formula C20H16N4OS (360.44).
4.1.1.3. 4-[(3-Acetylphenyl)amino]-2-(methylthio)-6-phenylpyrimidine-5-carbonitrile 6. Faint brown fluffy powder (yield 93.4%, 3.36 g); mp = 226–225 °C; 1H NMR (500 MHz, DMSO-d6) δ ppm: 10.10 (s, 1H, NH), 7.93 (d, J = 8.8 Hz, 2H, Ar–H, H-2 & H-6 of –C6H5), 7.85 (s, 1H, Ar–H, H-2 of –C6H4), 7.83 (d, J = 2.3 Hz, 1H, Ar–H, H-4 of –C6H4), 7.76–7.73 (m, 2H, Ar–H, H-5 & H-6 of –C6H4), 7.57–7.53 (m, 3H, Ar–H, H-3, H-4 & H-5 of –C6H5), 2.53 (s, 3H, COCH3), 2.45 (s, 3H, SCH3); 13C NMR (126 MHz, DMSO-d6) δ ppm: 197.26, 174.64, 168.73, 160.40, 142.76, 136.38, 132.96, 131.80, 129.36 (2C), 129.30 (2C), 129.12 (2C), 122.83 (2C), 116.65, 86.16, 27.11, 14.37; molecular formula C20H16N4OS (360.44).
4.1.2. 4-[(5-Cyano-2-(methylthio)-6-phenylpyrimidin-4-yl)amino]benzoyl chloride 7. To a suspension of compound 4 (3.62 g, 0.01 mol) in dichloromethane (20 mL), thionyl chloride (15 mL) was added and the mixture was heated gently under reflux for 2–3 h. Then, the reaction mixture was filtrated while hot, and the excess of thionyl chloride was removed under vacuum. After cooling, the formed yellow crystals were washed with diethyl ether several times and dried to afford the intermediate compound 7.
4.1.3. 4-[(5-Cyano-2-(methylthio)-6-phenylpyrimidin-4-yl)amino]benzohydrazide 8. Hydrazine hydrate 70% (0.5 mL, 0.5 g, 0.1 mol) was added to a stirred mixture of compound 7 (3.80 g, 0.01 mol) and absolute ethanol (50 mL) in ice bath. The reaction mixture was further stirred for 30–60 min. After completion of reaction, the formed precipitate was filtered. The cropped powder was crystallized from ethanol to afford the target compounds 8.White crystals (yield 90%, 3.4 g); mp = 294–295 °C; IR (KBr) ν cm−1: 3296 (NH), 3202 (NH2), 3035 (CH aromatic), 2922 (CH aliphatic), 2213 (C
N), 1658 (C
O), 1602 (C
N); 1H NMR (500 MHz, DMSO-d6) δ ppm: 9.97 (s, 1H, CONH), 9.74 (s, 1H, NH), 7.84 (d, J = 6.6 Hz, 2H, Ar–H, H-3 & H-5 of –C6H4), 7.79 (d, J = 8.7 Hz, 2H, Ar–H, H-2 & H-6 of –C6H5), 7.67 (d, J = 8.8 Hz, 2H, Ar–H, H-2 & H-6 of –C6H4), 7.58–7.53 (m, 3H, Ar–H, H-3, H-4 & H-5 of –C6H5), 4.72 (s, 2H, NH2), 2.44 (s, 3H, SCH3); 13C NMR (126 MHz, DMSO-d6) δ ppm: 174.71, 168.71, 165.98, 160.46, 140.92, 136.35, 131.81, 129.62, 129.29 (2C), 129.12 (2C), 127.81 (2C), 123.17 (2C), 116.51, 85.61, 14.32; molecular formula C19H16N6OS (376.44).
4.1.4. General procedure for synthesis of compounds 9a–d. Equimolar amounts of compound 8 (0.25 g, 0.0067 mol) and the appropriate aldehydes namely, 2-chlorobenzaldehyde, 4-chlorobenzaldehyde, 2,4-dichlorobenzaldehyde, and 2,6-di-chlorobenzaldehyde (0.001 mol) were refluxed in absolute ethanol (25 mL) with catalytic amount of glacial acetic acid for 3–6 h. The reaction was followed up by TLC. After completion of reaction, the mixture was cooled. The precipitate formed was filtered, dried and recrystallized from ethanol to give the corresponding compounds 9a–d, respectively.
4.1.4.1. N-(2-Chlorobenzylidene)-4-[(5-cyano-2-(methylthio)-6-phenyl pyrimidin-4-yl)-amino]benzohydrazide 9a. White fluffy powder (yield 84.5%, 0.27 g); mp = 300–301 °C; IR (KBr) ν cm−1: 3276, 3236 (NH), 3062 (CH aromatic), 2931 (CH aliphatic), 2222 (C
N), 1649 (C
O), 1599 (C
N); 1H NMR (500 MHz, DMSO-d6) δ ppm: 12.05 (s, 1H, CONH), 10.08 (s, 1H, NH), 8.84 (s, 1H, N
CH), 8.00 (d, J = 5.6 Hz, 1H, Ar–H, H-6 of 2-ClC6H4), 7.93 (d, J = 8.4 Hz, 2H, Ar–H, H-3 & H-5 of –C6H4), 7.85 (d, J = 7.5 Hz, 2H, Ar–H, H-2 & H-6 of –C6H5), 7.79 (d, J = 8.2 Hz, 2H, Ar–H, H-2 & H-6 of –C6H4), 7.60–7.53 (m, 3H, Ar–H, H-3, H-4 & H-5 of –C6H5), 7.50 (d, J = 7.5 Hz, 1H, Ar–H, H-3 of 2-Cl–C6H4), 7.43–7.39 (m, 2H, Ar–H, H-4 & H-5 of 2-Cl–C6H4), 2.48 (s, 3H, SCH3); 13C NMR (126 MHz, DMSO-d6) δ ppm: 174.78, 168.76, 163.05, 160.43, 143.89, 141.79, 136.32, 133.71, 132.17, 132.02, 131.85, 130.50, 129.32 (3C), 129.14 (3C), 128.64, 128.20, 127.39, 123.06 (2C), 116.50, 85.80, 14.40; molecular formula C26H19ClN6OS (498.99).
4.1.4.2. N-(4-Chlorobenzylidene)-4-[(5-cyano-2-(methylthio)-6-phen-ylpyrimidin-4-yl)-amino]benzohydrazide 9b. Off-white fluffy powder (yield 83.9%, 0.275 g); mp = 309–310 °C; HPLC purity 99.53%; IR (KBr) ν cm−1: 3300, 3227 (NH), 3062 (CH aromatic), 2924 (CH aliphatic), 2214 (C
N), 1650 (C
O), 1607 (C
N); 1H NMR (500 MHz, DMSO-d6) δ ppm: 11.89 (s, 1H, CONH), 10.07 (s, 1H, NH), 8.41 (s, 1H, N
CH), 7.91 (d, J = 8.4 Hz, 2H, Ar–H, H-3 & H-5 of –C6H4), 7.85 (d, 2H, J = 6.8 Hz, Ar–H, H-2 & H-6 of –C6H5), 7.77 (d, J = 8.4 Hz, 2H, Ar–H, H-2 & H-6 of –C6H4), 7.72 (d, J = 8.3 Hz, 2H, Ar–H, H-2 & H-6 of 4-ClC6H4), 7.57–7.49 (m, 3H, Ar–H, H-3, H-4 & H-5 of –C6H5), 7.50 (d, J = 8.1 Hz, 2H, Ar–H, H-3 & H-5 of 4-ClC6H4), 2.48 (s, 3H, SCH3); 13C NMR (126 MHz, DMSO-d6) δ ppm: 174.77, 168.76, 163.06, 160.44, 146.65, 141.70, 136.33, 134.99, 133.88, 131.85, 129.51 (2C), 129.31 (3C), 129.25, 129.14 (3C), 128.59 (2C), 123.09 (2C), 116.52, 85.79, 14.39; MS (m/z): 497.99 (M+, 31.63%), 500.61 (M2+, 28.55%), 462.04 (100%, base peak); molecular formula C26H19ClN6OS (498.99).
4.1.4.3. 4-[(5-Cyano-2-(methylthio)-6-phenylpyrimidin-4-yl)amino]-N-(2,4-dichloro-benzylidene)benzohydrazide 9c. Off-white powder (yield 85.6%, 0.30 g); mp = 290–291 °C; HPLC purity 97.46%; IR (KBr) ν cm−1: 3303, 3201 (NH), 3043 (CH aromatic), 2925 (CH aliphatic), 2215 (C
N), 1650 (C
O), 1612 (C
N); 1H NMR (500 MHz, DMSO-d6) δ ppm: 12.09 (s, 1H, CONH), 10.07 (s, 1H, NH), 8.78 (s, 1H, N
CH), 7.99 (d, J = 8.5 Hz, 1H, Ar–H, H-6 of –C6H3), 7.93 (d, J = 8.5 Hz, 2H, Ar–H, H-3 & H-5 of –C6H4), 7.85 (d, J = 7.4 Hz, 2H, Ar–H, H-2 & H-6 of –C6H5), 7.79 (d, J = 8.4 Hz, 2H, Ar–H, H-2 & H-6 of –C6H4), 7.70 (s, 1H, Ar–H, H-3 of –C6H3), 7.60–7.49 (m, 3H, Ar–H, H-3, H-4 & H-5 of –C6H5), 7.50 (d, J = 8.6 Hz, 1H, Ar–H, H-5 of –C6H3), 2.48 (s, 3H, SCH3); 13C NMR (126 MHz, DMSO-d6) δ ppm: 174.78, 168.77, 163.05, 160.43, 142.81, 141.89, 136.32, 135.57, 134.37, 131.86, 131.31, 129.96, 129.32 (3C), 129.14 (3C), 129.03, 128.66, 128.58, 123.07 (2C), 116.51, 85.82, 14.40; molecular formula C26H18Cl2N6OS (533.43).
4.1.4.4. 4-[(5-Cyano-2-(methylthio)-6-phenylpyrimidin-4-yl)amino]-N-(2,6-dichloro-benzylidene)benzohydrazide 9d. White fluffy powder (yield 82.8%, 0.29 g); mp = 298–299 °C; IR (KBr) ν cm−1: 3311, 3202 (NH), 3062 (CH aromatic), 2926 (CH aliphatic), 2218 (C
N), 1645 (C
O), 1610 (C
N); 1H NMR (500 MHz, DMSO-d6) δ ppm: 12.11 (s, 1H, CONH), 10.07 (s, 1H, NH), 8.62 (s, 1H, N
CH), 7.93 (d, J = 8.5 Hz, 2H, Ar–H, H-3 & H-5 of –C6H4), 7.85 (d, J = 7.2 Hz, 2H, Ar–H, H-2 & H-6 of –C6H5), 7.78 (d, J = 8.5 Hz, 2H, Ar–H, H-2 & H-6 of –C6H4), 7.59–7.54 (m, 5H, Ar–H, H-3 & H-5 of –C6H3 and H-3, H4 & H5 of –C6H5), 7.42 (t, J = 8.1 Hz, 1H, Ar–H, H-4 of –C6H3), 2.50 (s, 3H, SCH3); 13C NMR (126 MHz, DMSO-d6) δ ppm: 177.55, 171.12, 165.64, 160.46, 147.49, 136.34, 131.85, 129.67 (4C), 129.31 (5C), 129.14 (4C), 123.09, 115.53, 113.17, 112.36, 81.83, 14.38; molecular formula C26H18C12N6OS (533.43).
4.1.5. General procedure for synthesis of target compounds 11a–e. To a solution of compound 5 (0.25 g, 0.0069 mol) in absolute ethanol (30 mL), the appropriate benzohydrazide derivatives 10a–e namely, benzohydrazide, 2-chlorobenzohydrazide, 4-chlorobenzohydrazide, 4-nitrobenzohydrazide, and 2,4-dinitrobenzohydrazide (0.001 mol) were added. The reaction mixture was refluxed with vigorous stirring till completion of the reaction (4–6 h). After completion of the reaction, the reaction mixture was cooled to room temperature and poured onto ice water (100 mL). The crude product formed was filtered, washed with water, and crystallized from ethanol to afford the corresponding derivatives 11a–e.
4.1.5.1. N-(1-(4-[(5-Cyano-2-(methylthio)-6-phenylpyrimidin-4-yl)amino]phenyl)ethylidene)benzohydrazide 11a. Off-white powder (yield 90.3%, 0.30 g); mp = 251–252 °C; IR (KBr) ν cm−1: 3294, 3229 (NH), 3055 (CH aromatic), 2921 (CH aliphatic), 2216 (C
N), 1650 (C
O), 1612 (C
N); 1H NMR (500 MHz, DMSO-d6) δ ppm: 10.74 (s, 1H, CONH), 9.97 (s, 1H, NH), 7.85–7.82 (m, 4H, Ar–H, H-2 & H-6 of –C6H5 and H-2 & H-6 of –COC6H5), 7.69 (d, J = 8.1 Hz, 2H, Ar–H, H-2 & H-6 of –C6H4), 7.61–7.51 (m, 6H, Ar–H, H-3 & H-5 of –C6H4, H-3 & H-5 of –C6H5 and H-3 & H-5 of –COC6H5), 7.50–7.47 (m, 2H, Ar–H, H-4 of –COC6H5 and H-4 of –C6H5), 2.46 (s, 3H, SCH3), 2.34 (s, 3H, CH3); 13C NMR (126 MHz, DMSO-d6) δ ppm: 174.71, 168.69, 160.40, 155.46, 139.55, 136.40, 134.65, 131.79, 129.29 (4C), 129.12 (4C), 128.91, 128.43, 127.10, 123.41 (2C), 122.81, 116.58, 111.45, 85.45, 14.94, 14.37; molecular formula C27H22N6OS (478.57).
4.1.5.2. 2-Chloro-N-(1-(4-[(5-cyano-2-(methylthio)-6-phenylpyrimidin-4-yl)amino]phenyl)-ethylidene)benzohydrazide 11b. Off-white fluffy powder (yield 80%, 0.28 g); mp = 232–233 °C; HPLC purity 99.01%; IR (KBr) ν cm−1: 3300, 3224 (NH), 3057 (CH aromatic), 2924 (CH aliphatic), 2214 (C
N), 1658 (C
O), 1612 (C
N); 1H NMR (500 MHz, DMSO-d6) δ ppm: 11.09 (d, J = 125.9 Hz, 1H, CONH), 9.90 (d, J = 67.3 Hz, 1H, NH), 7.85–7.81 (m, 3H, Ar–H, H-2 & H-6 of –C6H5 and H-6 of 2-ClC6H4), 7.69 (d, J = 8.4 Hz, 1H, Ar–H, H-5 of 2-ClC6H4), 7.58–7.51 (m, 4H, Ar–H, H-2, H-3, H-5 & H-6 of –C6H4), 7.50–7.45 (m, 2H, Ar–H, H-3 & H-5 of –C6H5), 7.43–7.35 (m, 3H, Ar–H, H-4 of –C6H4 and H-3 & H-4 of 2-ClC6H4), 2.42 (d, J = 32.2 Hz, 3H, SCH3), 2.26 (d, J = 16.6 Hz, 3H, CH3); 13C NMR (126 MHz, DMSO-d6) δ ppm: 174.73–174.61, 170.13, 168.69–168.62, 163.53, 160.40–160.33, 154.52, 148.58, 139.59, 139.03, 137.29, 136.38, 136.31, 134.69, 134.50, 131.77, 131.67, 131.01, 130.81, 130.24, 130.11, 129.35, 129.28, 129.10 (2C), 127.68, 127.38, 127.12, 126.40, 123.37, 116.54, 85.46, 85.37, 14.96, 14.39, 14.2, 14.15; molecular formula C27H21ClN6OS (513.02).
4.1.5.3. 3-Chloro-N-(1-(4-[(5-cyano-2-(methylthio)-6-phenylpyramid-in-4-yl)amino]-phenyl)ethylidene)benzohydrazide 11c. Yellow powder (yield 80.1%, 0.285 g); mp = 262–263 °C; HPLC purity 96.25%; IR (KBr) ν cm−1: 3306, 3194 (NH), 3060 (CH aromatic), 2926 (CH aliphatic), 2222 (C
N), 1666 (C
O), 1620 (C
N); 1H NMR (400 MHz, DMSO-d6) δ ppm: 10.88 (s, 1H, CONH), 9.98 (s, 1H, NH), 7.96–7.86 (m, 5H, Ar–H, H-2 & H-6 of –C6H5 and H-2, H-4 & H-6 of 3-ClC6H4), 7.74 (d, J = 9.1 Hz, 2H, Ar–H, H-2 & H-6 of –C6H4), 7.67 (d, J = 9.9 Hz, 2H, Ar–H, H-3 & H-5 of –C6H4), 7.63–7.57 (m, 4H, Ar–H, H-3, H-4 & H-5 of –C6H5 and H-5 of 3-ClC6H4), 2.51 (s, 3H, SCH3), 2.40 (s, 3H, –CH3); 13C NMR (101 MHz, DMSO-d6) δ ppm: 174.66, 168.61, 163.03, 160.36, 136.35, 134.48, 131.71, 131.07, 129.21 (4C), 129.04 (4C), 128.08, 127.12, 125.52, 123.31 (3C), 116.46, 85.45, 56.51, 19.02, 14.30; MS (m/z): 513.59 (M+, 14.25%), 515.73 (M+ + 2, 10.12%), 172.61 (100%, base peak); molecular formula C27H21ClN6OS (513.02).
4.1.5.4. N-(1-(4-[(5-Cyano-2-(methylthio)-6-phenylpyrimidin-4-yl)amino]phenyl)-ethylidene)-4-nitrobenzohydrazide 11d. Yellow fluffy powder (yield 78.5%, 0.285 g); mp = 268–269 °C; HPLC purity 93.41%; IR (KBr) ν cm−1: 3307, 3210 (NH), 3007 (CH aromatic), 2924 (CH aliphatic), 2213 (C
N), 1657 (C
O), 1611 (C
N), 1528, 1343 (NO2); 1H NMR (500 MHz, DMSO-d6) δ ppm: 11.06 (s, 1H, CONH), 9.98 (s, 1H, NH), 8.32 (d, J = 8.4 Hz, 2H, Ar–H, H-3 & H-5 of 4-NO2C6H4), 8.09 (d, J = 8.7 Hz, 2H, Ar–H, H-2 & H-6 of 4-NO2C6H4), 7.87 (d, J = 8.5 Hz, 2H, Ar–H, H-2 & H-6 of –C6H4), 7.84 (d, J = 7.1 Hz, 2H, Ar–H, H-2 & H-6 of –C6H5), 7.70 (d, J = 8.2 Hz, 2H, Ar–H, H-3 & H-5 of –C6H4), 7.58–7.54 (m, 3H, Ar–H, H-3, H-4 & H-5 of –C6H5), 2.46 (s, 3H, SCH3), 2.36 (s, 3H, CH3); 13C NMR (126 MHz, DMSO-d6) δ ppm: 174.72, 168.68, 162.95, 160.39, 156.81, 149.62, 140.43, 139.82, 136.38, 134.33, 131.79, 131.13, 129.99, 129.36, 129.29 (2C), 129.11 (2C), 127.23, 126.73, 124.02, 123.37, 122.80, 116.56, 85.49, 15.19, 14.38; molecular formula C27H21N7O3S (523.57).
4.1.5.5. N-(1-(4-[(5-Cyano-2-(methylthio)-6-phenylpyrimidin-4-yl)amino]phenyl)-ethylidene)-2,4-dinitrobenzohydrazide 11e. Reddish-orange fine powder (yield 67.1%, 0.265 g); mp = 298–299 °C; IR (KBr) ν cm−1: 3297, 3204 (NH), 3113 (CH aromatic), 2925 (CH aliphatic), 2209 (C
N), 1614 (C
N), 1550, 1362 (NO2); 1H NMR (500 MHz, DMSO-d6) δ ppm: 11.11 (s, 1H, CONH), 10.01 (s, 1H, NH), 8.87 (d, J = 3.1 Hz, 1H, Ar–H, H-3 of –C6H3), 8.38 (dd, J = 9.6, 2.5 Hz, 1H, Ar–H, H-5 of –C6H3), 8.11 (d, J = 9.6 Hz, 1H, Ar–H, H-6 of –C6H3), 7.96 (d, J = 8.8 Hz, 2H, Ar–H, H-2 & H-6 of –C6H4), 7.85 (d, J = 6.9 Hz, 2H, Ar–H, H-2 & H-6 of –C6H5), 7.74 (d, J = 8.7 Hz, 2H, Ar–H, H-3 & H-5 of –C6H4), 7.60–7.54 (m, 3H, Ar–H, H-3, H-4 & H-5 of –C6H5), 2.48 (s, 3H, SCH3), 2.44 (s, 3H, CH3); 13C NMR (126 MHz, DMSO-d6) δ ppm: 174.74, 168.71, 166.67, 160.35, 146.91, 144.96, 142.13, 140.07, 136.38, 133.43, 131.53, 130.72, 130.16, 129.31 (2C), 129.14 (2C), 127.28 (2C), 124.09, 123.40 (2C), 117.17, 116.13, 86.39, 14.41, 13.77; molecular formula C27H20N8O5S (568.57).
4.1.6. General procedure for synthesis of target compounds 12a–g. To a solution of compound 6 (0.25 g, 0.0069 mol) in absolute ethanol (30 mL), the appropriate benzohydrazide derivatives 10a-h namely, benzohydrazide, 2-chlorobenzohydrazide, 3-chlorobenzohydrazide, 4-nitrobenzohydrazide, 2-hydroxybenzohydrazide, 4-hydroxybenzo- hydrazide, and 4-aminobenzohydrazide (0.001 mol) were added. The mixture was heated at refluxing temperature for 4–6 h. After completion of the reaction, the reaction mixture was cooled to room temperature and poured onto ice water (100 mL). The crude product formed was filtered, washed with water, and crystallized from ethanol to afford the corresponding derivatives 12a–g, respectively.
4.1.6.1. N-(1-(3-[(5-Cyano-2-(methylthio)-6-phenylpyrimidin-4-yl)amino]phenyl)-ethylidene)benzohydrazide 12a. Beige fluffy powder (yield 87.3%, 0.29 g); mp = 242–225 °C; HPLC purity 99.38%; IR (KBr) ν cm−1: 3341, 3254 (NH), 3056 (CH aromatic), 2926 (CH aliphatic), 2206 (C
N), 1649 (C
O), 1615 (C
N); 1H NMR (400 MHz, DMSO-d6) δ ppm: 10.80 (s, 1H, CONH), 9.98 (s, 1H, NH), 7.92–7.87 (m, 4H, Ar–H, H-2, H-6 of –C6H5 and Ar–H, H-2 & H-6 of –COC6H5), 7.70–7.67 (m, 2H, Ar–H, H-4 of –C6H4 and H-4 –COC6H5), 7.62–7.59 (m, 5H, Ar–H, H-3, H-4, H-5 of –C6H5 and H-3 & H-5 of –COC6H4), 7.54 (d, J = 7.3 Hz, 2H, Ar–H, H-2 & H-5 of –C6H4), 7.47 (d, J = 8.00 Hz, 1H, Ar–H, H-6 of –C6H4), 2.47 (s, 3H, SCH3), 2.40 (s, 3H, CH3); 13C NMR (101 MHz, DMSO-d6) δ ppm: 174.63, 168.58, 161.83, 160.58, 155.46, 138.85, 138.33, 136.40, 134.55, 132.02, 131.65, 129.19 (3C), 129.03 (3C), 128.83 (2C), 128.42, 125.10, 123.44, 122.10, 116.49, 85.10, 15.03, 14.22; molecular formula C27H22N6OS (478.57).
4.1.6.2. 2-Chloro-N-(1-(3-[(5-cyano-2-(methylthio)-6-phenyl pyri-midin-4-yl)amino]-phenyl)ethylidene)benzohydrazide 12b. White fluffy powder (yield 77%, 0.27 g); mp = 221–222 °C; IR (KBr) ν cm−1: 3396 (NH), 3147, 3026 (CH aromatic), 2993 (CH aliphatic), 2202 (C
N), 1664 (C
O), 1613 (C
N); 1H NMR (500 MHz, DMSO-d6) δ ppm: 11.13 (d, J = 136.2 Hz, 1H, CONH), 9.96 (d, J = 72.0 Hz, 1H, NH), 8.17 (d, J = 34.5 Hz, 1H, Ar–H, H-2 of –C6H4), 7.84 (d, J = 7.2 Hz, 2H, Ar–H, H-2 & H-6 of –C6H5), 7.69–7.64 (m, 1H, Ar–H, H-6 of –C6H4), 7.60 (dd, J = 15.0, 7.2 Hz, 1H, Ar–H, H-6 of –C6H4), 7.56–7.47 (m, J = 12.8, 5.9 Hz, 3H, Ar–H, H-3 of 3-ClC6H4 and Ar–H, H-3 & H-5 of –C6H5), 7.46–7.37 (m, 3H, Ar–H, H-4 of –C6H4, 4 H-4 of 3-ClC6H4 & Ar–H, H-4 of –C6H5), 7.35–7.27 (m, 1H, Ar–H, H-5 of –C6H4), 7.25–7.15 (m, 1H, Ar–H, H-5 of 3-ClC6H4), 2.45 (s, 3H, SCH3), 2.27 (s, 3H, CH3); 13C NMR (126 MHz, DMSO-d6) δ ppm: 174.70, 170.21, 168.66, 163.60, 160.61, 154.48, 148.32, 138.76, 138.59, 137.14, 136.44, 131.73, 130.99, 130.19, 129.27, 129.10, 128.90, 127.69, 125.22, 24.86, 123.58, 122.53, 116.58, 85.16, 84.92, 15.38, 14.30; molecular formula C27H21ClN6OS (513.02).
4.1.6.3. 3-Chloro-N-(1-(3-[(5-cyano-2-(methylthio)-6-phenylpyramidin-4-yl)amino]phenyl)-ethylidene)benzohydrazide 12c. Beige crystals (yield 87%, 0.31 g); mp = 230–231 °C; HPLC purity 100%; IR (KBr) ν cm−1: 3308, 3178 (NH), 3067 (CH aromatic), 2923 (CH aliphatic), 2216 (C
N), 1669 (C
O), 1628 (C
N); 1H NMR (400 MHz, DMSO-d6) δ ppm: 10.91 (s, 1H, CONH), 10.00 (s, 1H, NH), 8.18 (s, 1H, Ar–H, H-2 of 3-ClC6H4), 7.96 (s, 1H, Ar–H, H-6 of 3-ClC6H4), 7.90–7.88 (m, 3H, Ar–H, H-4 of –C6H4 and H-2 & H-6 of –C6H5), 7.63–7.53 (m, 6H, Ar–H, H-2 of –C6H4, H-4, H-5 of 3-ClC6H4 and Ar–H, H-3, H-4 & H-5 of –C6H5), 7.57–7.53 (m, 1H, Ar–H, H-5 of –C6H4), 7.46 (d, J = 4.00 Hz, 1H, Ar–H, H-6 of –C6H4), 2.47 (s, 3H, SCH3), 2.41 (s, 3H, CH3); 13C NMR (101 MHz, DMSO-d6) δ ppm: 174.62, 168.56, 162.95, 160.60, 150.58, 138.71, 138.37, 137.74, 136.42, 136.35, 133.22, 131.65, 131.07, 129.17 (2C), 129.02 (2C), 128.86, 128.47, 126.99, 125.04, 123.39, 122.13, 116.47, 85.11, 27.23, 14.20; molecular formula C27H21ClN6OS (513.02).
4.1.6.4. N-(1-(3-[(5-cyano-2-(methylthio)-6-phenylpyrimidin-4-yl)amino]phenyl)-ethylidene)-4-nitrobenzohydrazide 12d. Off-white crystals (yield 80%, 0.29 g); mp = 265–266 °C; IR (KBr) ν cm−1: 3305, 3196 (NH), 3076 (CH aromatic), 2927 (CH aliphatic), 2213 (C
N), 1670 (C
O), 1597 (C
N), 1543, 1345 (NO2); 1H NMR (400 MHz, DMSO-d6) δ ppm: 11.10 (s, 1H, CONH), 9.98 (s, 1H, NH), 8.36 (d, J = 8.0 Hz, 2H, Ar–H, H-3 & H-5 of 4-NO2C6H4), 8.16 (d, 2H, Ar–H, H-2 & H-6 of 4-NO2C6H4), 8.06 (s, 1H, Ar–H, H-2 of –C6H4), 7.89 (d, 2H, J = 8.0 Hz, Ar–H, H-2 & H-6 of –C6H5), 7.71–7.68 (m, 1H, Ar–H, H-5 of –C6H4), 7.61–7.59 (m, 4H, Ar–H, H-3, H-4 & H-5 of –C6H5 and H-4 of –C6H4), 7.47 (d, 1H, Ar–H, H-6 of –C6H4), 2.51 (s, 3H, SCH3), 2.20 (s, 3H, CH3); 13C NMR (101 MHz, DMSO-d6) δ ppm: 174.61, 168.58, 164.39, 163.07, 160.56, 138.15, 137.19, 136.39, 131.65, 129.95 (3C), 129.19 (2C), 129.05 (2C), 129.01, 128.92, 125.36, 124.02, 123.93, 123.70, 122.14, 116.50, 85.11, 15.04, 14.21; molecular formula C27H21N7O3S (523.57).
4.1.6.5. N-(1-(3-[(5-Cyano-2-(methylthio)-6-phenylpyrimidin-4-yl)amino]phenyl)-ethylidene)-2-hydroxybenzohydrazide 12e. Beige crystals (yield 79%, 0.27 g); mp = 204–205 °C; IR (KBr) ν cm−1: 3392 (OH), 3297 (NH), 3060 (CH aromatic), 2925 (CH aliphatic), 2213 (C
N), 1649 (C
O), 1602 (C
N); 1H NMR (500 MHz, DMSO-d6) δ ppm: 11.39 (s, 1H, OH), 9.97 (s, 1H, CONH), 8.14 (s, 1H, NH), 7.96 (d, J = 5.00 Hz, 1H, Ar–H, H-6 of 2-OHC6H4), 7.84 (d, J = 7.2 Hz, 2H, Ar–H, H-2 & H-6 of –C6H5), 7.66–7.61 (m, 2H, Ar–H, H-5 of –C6H4 & H-4 of 2-OHC6H4), 7.60–7.51 (m, 4H, Ar–H, H-3, H-4 & H-5 of –C6H5 and H-4 of –C6H4), 7.43–7.37 (m, 2H, Ar–H, H-2 & H-6 of –C6H4), 6.99 (d, J = 8.2 Hz, 1H, Ar–H, H-3 of 2-OHC6H4), 6.94 (t, J = 7.6 Hz, 1H, Ar–H, H-5 of 2-OHC6H4), 2.43 (s, 3H, SCH3), 2.32 (s, 3H, CH3); 13C NMR (126 MHz, DMSO-d6) δ ppm: 174.71, 168.65, 162.55, 160.62, 157.21, 152.12, 138.69, 138.39, 136.45, 133.94, 131.73, 131.21, 129.27 (2C), 129.10 (2C), 128.93, 125.11, 123.56, 122.04, 120.13, 118.44, 117.47, 116.58, 85.13, 14.42, 14.30; molecular formula C27H22N6O2S (494.57).
4.1.6.6. N-(1-(3-[(5-Cyano-2-(methylthio)-6-phenylpyrimidin-4-yl)amino]phenyl)-ethylidene)-4-hydroxybenzohydrazide 12f. Beige fine powder (yield 75.8%, 0.26 g); mp = 231–232 °C; HPLC purity 94.88%; IR (KBr) ν cm−1: 3316 (OH), 3270, 3196 (NH), 3011 (CH aromatic), 2806 (CH aliphatic), 2204 (C
N), 1659 (C
O), 1614 (C
N); 1H NMR (400 MHz, DMSO-d6) δ ppm: 10.53 (s, 1H, CONH), 9.99 (s, 1H, OH), 9.49 (s, 1H, NH), 7.89 (d, J = 7.5 Hz, 2H, Ar–H, H-2 & H-6 of –C6H5), 7.81 (d, J = 8.3 Hz, 1H, Ar–H, H-4 of –C6H4), 7.71–7.67 (m, 3H, Ar–H, H-2 & H-6 of 4-OHC6H4 and H-2 of –C6H4), 7.64–7.59 (m, 3H, Ar–H, H-3, H-4 & H-5 of –C6H5), 7.45 (t, J = 8.0 Hz, 1H, Ar–H, H-5 of –C6H4), 6.87 (d, J = 8.4 Hz, 1H, Ar–H, H-6 of –C6H4), 6.79 (d, J = 8.2 Hz, 2H, Ar–H, H-3 & H-5 of 4-OHC6H4), 2.47 (s, 3H, SCH3), 2.38 (s, 3H, CH3); 13C NMR (101 MHz, DMSO-d6) δ ppm: 174.61, 168.57, 166.40, 161.01, 160.57, 160.47, 139.00, 136.42, 131.64, 129.28 (3C), 129.19 (2C), 129.02 (2C), 128.80, 124.90, 124.44, 123.34, 122.03, 115.29 (3C), 85.10, 14.80, 14.21; MS (m/z): 494.37 (M+, 54.40%), 419.31 (100%, base peak); molecular formula C27H22N6O2S (494.57).
4.1.6.7. 4-Amino-N-(1-(3-[(5-cyano-2-(methylthio)-6-phenylpyrimidin-4-yl)amino]phenyl)-ethylidene)benzohydrazide 12g. White fluffy powder (yield 80%, 0.27 g); mp = 227–228 °C; IR (KBr) ν cm−1: 3478, 3363, 3293 (2NH, NH2), 3071 (CH aromatic), 2924, (CH aliphatic), 2215 (C
N), 1637 (C
O), 1606 (C
N); 1H NMR (400 MHz, DMSO-d6) δ ppm: 10.29 (s, 1H, CONH), 9.97 (s, 1H, NH), 8.12 (s, 1H, Ar–H, H-4 of –C6H4), 7.91 (d, J = 8.00 Hz, 2H, Ar–H, H-2 & H-6 of –C6H5), 7.68 (d, J = 8.4 Hz, 2H, Ar–H, H-2 & H-6 of 4-NH2C6H4), 7.65 (d, J = 3.9 Hz, 1H, Ar–H, H-6 of –C6H4), 7.64–7.56 (m, 4H, Ar–H, H-2 of –C6H4, and H-3, H-4 & H-5 of –C6H5), 7.45 (t, J = 7.9 Hz, 1H, Ar–H, H-5 of –C6H4), 6.61 (d, J = 8.3 Hz, 2H, Ar–H, H-3 & H-5 of 4-NH2C6H4), 5.75 (s, 2H, NH2), 2.47 (s, 3H, SCH3), 2.37 (s, 3H, CH3); 13C NMR (101 MHz, DMSO-d6) δ ppm: 174.61, 168.57, 164.59, 160.57, 152.66, 139.18, 138.35, 136.43, 131.63, 130.31, 129.19 (3C), 129.02 (3C), 128.76, 124.79, 123.26, 121.97, 120.53, 116.52, 113.00 (2C), 85.09, 14.60, 14.22; molecular formula C27H23N7OS (493.59).
4.2. Biological evaluation
4.2.1. In vitro antiproliferative activities. The in vitro antiproliferative activeness of all the synthesized derivatives had been evaluated towards HCT-116 and MCF-7 cell lines, using the MTT assay protocol25–27 as prescribed in as shown in ESI.† Sorafenib was utilized as the reference drug.The cell lines were obtained from the Holding company for biological products and vaccines (VACSERA), Cairo, Egypt.
4.2.2. In vitro cytotoxicity against normal human lung cells. Cytotoxic activity of the most active anti-proliferative compound 11e was estimated against normal human lung cells (WI-38) using the MTT assay protocol41 as shown in ESI.†
4.2.3. In vitro VEGFR-2 kinase inhibitory assay. The VEGFR-2 inhibitory activity for the most active cytotoxic compounds (9d, 11b, 11c, 11d, 11e, 12b, 12c, and 12d) were assessed in vitro using the Human VEGFR-2 TK ELISA kit as shown in ESI.†
4.2.4. In vitro DNA-flow cytometric (cell cycle) analysis. To investigate the effect of compound 11e on the various phases of the cell cycle, flow cytometric analysis of cell cycle was performed using Epics XL-MCL™ Flow Cytometer22,41 as shown in ESI.†
4.2.5. Annexin V-FITC apoptosis assay. Apoptotic effect of compound 11e on the treated cells was analyzed with the aid of Annexin V-FITC-apoptosis detection kit (PN IM3546) using Epics XL-MCL™ Flow Cytometer33,41 as prescribed in ESI.†
4.2.6. In vitro immunomodulatory assay of TNF-α and IL-6. Cell culture supernatants as well as cell lysate were prepared from HepG2 cancer cell line utilized for immunoassay using different kits.24,42,43 The protocols for cell culture supernatants and cell lysate preparation were written in details in ESI.†
4.2.7. Assessment of caspase-3 expression. The levels of caspase-3, cell culture supernatants was estimated by ELISA technique using commercially available matched paired antibodies (R&D Systems Inc., Minneapolis, MN) according to reported procedure44 and described in details in ESI.†
4.3. In silico studies
4.3.1. Docking studies. Docking studies towards VEGFR-2 TK (PDB ID 1YWN, resolution: 1.71 Å) were performed by MOE2019 software as shown in ESI.†
4.3.2. MD simulations. MD simulation studies were directed by the CHARMM-GUI web server and GROMACS 2021 as an MD engine as shown in ESI.†45,46
4.3.3. MM-GBSA. MM-GBSA and PLIP analyses were performed by the Gmx_MMPBSA package as shown in ESI.†47
Conflicts of interest
This work was funded by the authors and there is no any conflict of interest.
Acknowledgements
This paper is based upon work supported by Science, Technology & Innovation Funding Authority (STIFA) under grant number 44434.
References
- V. Ganapathy, M. Thangaraju and P. D. Prasad, Nutrient transporters in cancer: relevance to Warburg hypothesis and beyond, Pharmacol. Therapeut., 2009, 121(1), 29–40 CrossRef CAS PubMed
. - H. Varmus, The new era in cancer research, Science, 2006, 312(5777), 1162–1165 CrossRef CAS PubMed
. - Z. Wang, X.-H. Shi, J. Wang, T. Zhou, Y.-Z. Xu and T.-T. Huang, et al., Synthesis, structure–activity relationships and preliminary antitumor evaluation of benzothiazole-2-thiol derivatives as novel apoptosis inducers, Bioorg. Med. Chem. Lett., 2011, 21(4), 1097–1101 CrossRef CAS PubMed
. - R. L. Siegel, K. D. Miller and A. Jemal, Cancer statistics, Ca-Cancer J. Clin., 2018, 68(1), 7–30 CrossRef PubMed
. - N. M. Saleh, A. A. H. Abdel-Rahman, A. M. Omar, M. M. Khalifa and K. El-Adl, Pyridine-derived VEGFR-2 inhibitors: rational design, synthesis, anticancer evaluations, in silico ADMET profile, and molecular docking, Arch. Pharm., 2021, 354(8), 2100085 CrossRef CAS PubMed
. - R. G. Yousef, W. M. Eldehna, A. Elwan, A. S. Abdelaziz, A. B. Mehany and I. M. Gobaara, et al., Design, Synthesis, In Silico and In Vitro Studies of New Immunomodulatory Anticancer Nicotinamide Derivatives Targeting VEGFR-2, Molecules, 2022, 27(13), 4079 CrossRef CAS PubMed
. - F. Ran, W. Li, Y. Qin, T. Yu, Z. Liu and M. Zhou, et al., Inhibition of vascular smooth muscle and cancer cell proliferation by new VEGFR inhibitors and their immunomodulator effect: design, synthesis, and biological evaluation, Oxid. Med. Cell. Longev., 2021, 2021, 1–21 Search PubMed
. - R. G. Yousef, A. Ibrahim, M. M. Khalifa, W. M. Eldehna, I. M. Gobaara and A. B. Mehany, et al., Discovery of new nicotinamides as apoptotic VEGFR-2 inhibitors: virtual screening, synthesis, anti-proliferative, immunomodulatory, ADMET, toxicity, and molecular dynamic simulation studies, J. Enzym. Inhib. Med. Chem., 2022, 37(1), 1389–1403 CrossRef CAS PubMed
. - M. S. Taghour, H. A. Mahdy, M. H. Gomaa, A. Aglan, M. G. Eldeib and A. Elwan, et al., Benzoxazole derivatives as new VEGFR-2 inhibitors and apoptosis inducers: design, synthesis, in silico studies, and antiproliferative evaluation, J. Enzym. Inhib. Med. Chem., 2022, 37(1), 2063–2077 CrossRef CAS
. - E. B. Elkaeed, R. G. Yousef, M. M. Khalifa, A. Ibrahim, A. B. Mehany and I. M. Gobaara, et al., Discovery of New VEGFR-2 Inhibitors: Design, Synthesis, Anti-Proliferative Evaluation, Docking, and MD Simulation Studies, Molecules, 2022, 27(19), 6203 CrossRef CAS PubMed
. - M. S. Taghour, H. Elkady, W. M. Eldehna, N. M. El-Deeb, A. M. Kenawy and E. B. Elkaeed, et al., Design and synthesis of thiazolidine-2, 4-diones hybrids with 1, 2-dihydroquinolones and 2-oxindoles as potential VEGFR-2 inhibitors: in vitro anticancer evaluation and in silico studies, J. Enzyme Inhib. Med. Chem., 2022, 37(1), 1903–1917 CrossRef CAS PubMed
. - M. M. Alanazi, H. Elkady, N. A. Alsaif, A. J. Obaidullah, H. M. Alkahtani and M. M. Alanazi, et al., New quinoxaline-based VEGFR-2 inhibitors: design, synthesis, and antiproliferative evaluation with in silico docking, ADMET, toxicity, and DFT studies, RSC Adv., 2021, 11(48), 30315–30328 RSC
. - A. R. Kotb, D. A. Bakhotmah, A. E. Abdallah, H. Elkady, M. S. Taghour and I. H. Eissa, et al., Design, synthesis, and biological evaluation of novel bioactive thalidomide analogs as anticancer immunomodulatory agents, RSC Adv., 2022, 12(52), 33525–33539 RSC
. - I. H. Eissa, M. A. Dahab, M. K. Ibrahim, N. A. Alsaif, A. Alanazi and S. I. Eissa, et al., Design and discovery of new antiproliferative 1, 2, 4-triazin-3 (2H)-ones as tubulin polymerization inhibitors targeting colchicine binding site, Bioorg. Chem., 2021, 112, 104965 CrossRef CAS PubMed
. - M. A. Abdelgawad, K. El-Adl, S. S. El-Hddad, M. M. Elhady, N. M. Saleh and M. M. Khalifa, et al., Design, molecular docking, synthesis, anticancer
and anti-hyperglycemic assessments of thiazolidine-2, 4-diones bearing sulfonylthiourea moieties as potent VEGFR-2 inhibitors and PPARγ agonists, Pharmaceuticals, 2022, 15(2), 226 CrossRef CAS PubMed
. - I. H. Eissa, R. El-Haggar, M. A. Dahab, M. F. Ahmed, H. A. Mahdy and R. I. Alsantali, et al., Design, synthesis, molecular modeling and biological evaluation of novel benzoxazole-benzamide conjugates via a 2-thioacetamido linker as potential anti-proliferative agents, VEGFR-2 inhibitors and apoptotic inducers, J. Enzyme Inhib. Med. Chem., 2022, 37(1), 1587–1599 CrossRef CAS PubMed
. - M. A. Dahab, H. A. Mahdy, H. Elkady, M. S. Taghour, A. Elwan and M. A. Elkady, et al., Semi-synthesized anticancer theobromine derivatives targeting VEGFR-2: in silico and in vitro evaluations, J. Biomol. Struct. Dyn., 2023, 1–20 CrossRef PubMed
. - N. A. Alsaif, H. A. Mahdy, M. M. Alanazi, A. J. Obaidullah, H. M. Alkahtani and A. M. Al-Hossaini, et al., Targeting VEGFR-2 by new quinoxaline derivatives: design, synthesis, antiproliferative assay, apoptosis induction, and in silico studies, Arch. Pharm., 2022, 355(2), 2100359 CrossRef CAS
. - N. A. Alsaif, A. Elwan, M. M. Alanazi, A. J. Obaidullah, W. A. Alanazi and A. F. Alasmari, et al., Design, synthesis and molecular docking of new [1, 2, 4] triazolo [4, 3-a] quinoxaline derivatives as anticancer agents targeting VEGFR-2 kinase, Mol. Diversity, 2022, 26(4), 1915–1932 CrossRef CAS
. - H. A. Mahdy, M. K. Ibrahim, A. M. Metwaly, A. Belal, A. B. Mehany and K. M. El-Gamal, et al., Design, synthesis, molecular modeling, in vivo studies and anticancer evaluation of quinazolin-4 (3H)-one derivatives as potential VEGFR-2 inhibitors and apoptosis inducers, Bioorg. Chem., 2020, 94, 103422 CrossRef CAS PubMed
. - S. Kambe, A one step synthesis of 4-oxo-2-thioxopyrimidine derivatives by the ternary condensation of ethyl cyanoacetate, aldehydes, and thiourea, Synthesis, 1979, 4, 287–289 CrossRef
. - A. A. Nasser, I. H. Eissa, M. R. Oun, M. A. El-Zahabi, M. S. Taghour and A. Belal, et al., Discovery of new pyrimidine-5-carbonitrile derivatives as anticancer agents targeting EGFR WT and EGFR T790M, Org. Biomol. Chem., 2020, 18(38), 7608–7634 RSC
. - I. A. Osman, R. R. Ayyad and H. A. Mahdy, New pyrimidine-5-carbonitrile derivatives as EGFR inhibitors with anticancer and apoptotic activities: design, molecular modeling and synthesis, New J. Chem., 2022, 46(24), 11812–11827 RSC
. - M. A. El-Zahabi, H. Sakr, K. El-Adl, M. Zayed, A. S. Abdelraheem and S. I. Eissa, et al., Design, synthesis, and biological evaluation of new challenging thalidomide analogs as potential anticancer immunomodulatory agents, Bioorg. Chem., 2020, 104, 104218 CrossRef CAS PubMed
. - T. Mosmann, Rapid colorimetric assay for cellular growth and survival: application to proliferation and cytotoxicity assays, J. Immunol. Methods, 1983, 65(1–2), 55–63 CrossRef CAS PubMed
. - F. Denizot and R. Lang, Rapid colorimetric assay for cell growth and survival: modifications to the tetrazolium dye procedure giving improved sensitivity and reliability, J. Immunol. Methods, 1986, 89(2), 271–277 CrossRef CAS PubMed
. - M. Thabrew, R. D. Hughes and I. G. McFarlane, Screening of hepatoprotective plant components using a HepG2 cell cytotoxicity assay, J. Pharm. Pharmacol., 1997, 49(11), 1132–1135 CrossRef CAS
. - T. Aiebchun, P. Mahalapbutr, A. Auepattanapong, O. Khaikate, S. Seetaha and L. Tabtimmai, et al., Identification of vinyl sulfone derivatives as egfr tyrosine kinase inhibitor: in vitro and in silico studies, Molecules, 2021, 26(8), 2211 CrossRef CAS
. - E. M. Mohi El-Deen, M. M. Anwar, A. A. A. El-Gwaad, E. A. Karam, M. K. El-Ashrey and R. R. Kassab, Novel Pyridothienopyrimidine Derivatives: Design, Synthesis and Biological Evaluation as Antimicrobial and Anticancer Agents, Molecules, 2022, 27(3), 803 CrossRef CAS PubMed
. - N. A. Alsaif, A. Elwan, M. M. Alanazi, A. J. Obaidullah, W. A. Alanazi and A. F. Alasmari, et al., Design, synthesis and molecular docking of new [1, 2, 4] triazolo [4, 3-a] quinoxaline derivatives as anticancer agents targeting VEGFR-2 kinase, Mol. Diversity, 2021, 1–18 Search PubMed
. - H. Van De Waterbeemd and E. Gifford, ADMET in silico modelling: towards prediction paradise?, Nat. Rev. Drug Discov., 2003, 2(3), 192–204 CrossRef CAS PubMed
. - A. Elwan, A. E. Abdallah, H. A. Mahdy, M. A. Dahab, M. S. Taghour and E. B. Elkaeed, et al., Modified benzoxazole-based VEGFR-2 inhibitors and apoptosis inducers: design, synthesis, and anti-proliferative evaluation, Molecules, 2022, 27(15), 5047 CrossRef CAS PubMed
. - T. Al-Warhi, M. F. Abo-Ashour, H. Almahli, O. J. Alotaibi, M. M. Al-Sanea and G. H. Al-Ansary, et al., Novel [(N-alkyl-3-indolylmethylene) hydrazono] oxindoles arrest cell cycle and induce cell apoptosis by inhibiting CDK2 and Bcl-2: synthesis, biological evaluation and in silico studies, J. Enzym. Inhib. Med. Chem., 2020, 35(1), 1300–1309 CrossRef CAS
. - A. E. Abdallah, I. H. Eissa, A. B. Mehany, H. Sakr, A. Atwa and K. El-Adl, et al., Immunomodulatory quinazoline-based thalidomide analogs: design, synthesis, apoptosis and anticancer evaluations, J. Mol. Struct., 2023, 1281, 135164 CrossRef CAS
. - Y. Miyazaki, S. Matsunaga, J. Tang, Y. Maeda, M. Nakano and R. J. Philippe, et al., Novel 4-amino-furo [2, 3-d] pyrimidines as Tie-2 and VEGFR2 dual inhibitors, Bioorg. Med. Chem. Lett., 2005, 15(9), 2203–2207 CrossRef CAS PubMed
. - M. Hagras, M. A. Saleh, R. R. Ezz Eldin, A. A. Abuelkhir, E. G. Khidr and A. A. El-Husseiny, et al., 1, 3, 4-Oxadiazole-naphthalene hybrids as potential VEGFR-2 inhibitors: design, synthesis, antiproliferative activity, apoptotic effect, and in silico studies, J. Enzym. Inhib. Med. Chem., 2022, 37(1), 386–402 CrossRef CAS
. - A. M. Farghaly, O. M. AboulWafa, Y. A. Elshaier, W. A. Badawi, H. H. Haridy and H. A. Mubarak, Design, synthesis, and antihypertensive activity of new pyrimidine derivatives endowing new pharmacophores, Med. Chem. Res., 2019, 28, 360–379 CrossRef CAS
. - N. A. Alsaif, M. A. Dahab, M. M. Alanazi, A. J. Obaidullah, A. A. Al-Mehizia and M. M. Alanazi, et al., New quinoxaline derivatives as VEGFR-2 inhibitors with anticancer and apoptotic activity: Design, molecular modeling, and synthesis, Bioorg. Chem., 2021, 110, 104807 CrossRef CAS
. - I. H. Eissa, A.-G. A. El-Helby, H. A. Mahdy, M. M. Khalifa, H. A. Elnagar and A. B. Mehany, et al., Discovery of new quinazolin-4 (3H)-ones as VEGFR-2 inhibitors: Design, synthesis, and anti-proliferative evaluation, Bioorg. Chem., 2020, 105, 104380 CrossRef CAS PubMed
. - M. M. Alanazi, H. A. Mahdy, N. A. Alsaif, A. J. Obaidullah, H. M. Alkahtani and A. A. Al-Mehizia, et al., New bis ([1, 2, 4] triazolo)[4, 3-a: 3′, 4′-c] quinoxaline derivatives as VEGFR-2 inhibitors and apoptosis inducers: Design, synthesis, in silico studies, and anticancer evaluation, Bioorg. Chem., 2021, 112, 104949 CrossRef CAS PubMed
. - N. A. Alsaif, M. S. Taghour, M. M. Alanazi, A. J. Obaidullah, W. A. Alanazi and A. Alasmari, et al., Identification of new [1, 2, 4] triazolo [4, 3-a] quinoxalines as potent VEGFR-2 tyrosine kinase inhibitors: Design, synthesis, anticancer evaluation, and in silico studies, Bioorg. Med. Chem., 2021, 46, 116384 CrossRef CAS PubMed
. - R. Talaat, W. El-Sayed, H. Agwa, A. Gamal-Eldeen, S. Moawia and M. Zahran, Novel thalidomide analogs: Anti-angiogenic and apoptotic effects on Hep-G2 and MCF-7 cancer cell lines, Biomed. Aging Pathol., 2014, 4(3), 179–189 CrossRef CAS
. - T. Inoue, K. Kibata, M. Suzuki, S. Nakamura, R. Motoda and K. Orita, Identification of a vascular endothelial growth factor (VEGF) antagonist, sFlt-1, from a human hematopoietic cell line NALM-16, FEBS Lett., 2000, 469(1), 14–18 CrossRef CAS PubMed
. - R. M. Talaat, Soluble angiogenesis factors in sera of Egyptian patients with hepatitis C virus infection: correlation with disease severity, Viral Immunol., 2010, 23(2), 151–157 CrossRef CAS
. - E. B. Elkaeed, I. H. Eissa, H. Elkady, A. Abdelalim, A. M. Alqaisi and A. A. Alsfouk, et al., A Multistage In Silico Study of Natural Potential Inhibitors Targeting SARS-CoV-2 Main Protease, Int. J. Mol. Sci., 2022, 23(15), 8407 CrossRef CAS
. - E. B. Elkaeed, F. S. Youssef, I. H. Eissa, H. Elkady, A. A. Alsfouk and M. L. Ashour, et al., Multi-Step In Silico Discovery of Natural Drugs against COVID-19 Targeting Main Protease, Int. J. Mol. Sci., 2022, 23(13), 6912 CrossRef CAS PubMed
. - E. B. Elkaeed, R. G. Yousef, H. Elkady, I. M. M. Gobaara, B. A. Alsfouk and D. Z. Husein, et al., Design, Synthesis, Docking, DFT, MD Simulation Studies of a New Nicotinamide-Based Derivative: In Vitro Anticancer and VEGFR-2 Inhibitory Effects, Molecules, 2022, 27(14), 4606 CrossRef CAS PubMed
.
|
This journal is © The Royal Society of Chemistry 2023 |
Click here to see how this site uses Cookies. View our privacy policy here.