DOI:
10.1039/D3RA03926A
(Paper)
RSC Adv., 2023,
13, 20467-20476
Eco-friendly synthesis of new olanzapine derivatives and evaluation of their anticancer potential†
Received
12th June 2023
, Accepted 30th June 2023
First published on 10th July 2023
Abstract
New derivatives of the known antipsychotic drug olanzapine have been obtained as potential compounds with anticancer activity in two metabolically different breast cancer cell lines: MCF-7 and triple negative MDA-MB-231. The compounds were obtained under phase transfer catalysis (PTC) in the presence of microwave irradiation (MW) or ultrasound (“)))”), evaluating the effect of solvents such as dimethylformamide, water, or choline chloride/urea (natural deep eutectic solvent, NaDES). In the best option, the compounds were obtained within 2 minutes with a yield of 57–86% in MW. Two of the obtained compounds which have a naphthalimide moiety and a pentyl (7) or hexyl chain (8) show pronounced cytotoxicity. Interestingly, neither olanzapine nor desmethylolanzapine (DOLA), which was one of the substrates for the synthesis reaction, showed any significant activity in the study.
Introduction
Olanzapine is an atypical antipsychotic that is approved by the FDA for use in the treatment of schizophrenia and bipolar disorder, including mixed or manic episodes. In addition, olanzapine may be used with fluoxetine, a selective serotonin reuptake inhibitor (SSRI), in patients who experience depressive episodes associated with bipolar I disorder and in treatment-resistant depression.1 Interestingly, olanzapine can be also used in combination with samidorphan to alleviate olanzapine-induced weight gain in schizophrenia and bipolar I disorder.2,3 Moreover, it can be used in cancer patients as an anti-emetic, but it can also have a beneficial effect on enhancing the effect of chemotherapeutic agents,4,5 as well as in the treatment of insomnia6–9 and as an analgesic agent.10–14 Additionally, it has also been tested in patients with Anorexia Nervosa.15–17 These numerous advantages of olanzapine prompted scientists to search for olanzapine derivatives, which are tested, for example, as selective ligands of 5HT2A/D2 receptors for more effective treatment of schizophrenia18,19 as PDE4B inhibitors. This means the derivatives can have procognitive, neuroprotective, and anti-inflammatory effects.20,21
In recent years, antipsychotic drugs have often been assessed with regard to their anticancer potential.22–32 It should be noted that olanzapine, as an antipsychotic drug, has an antagonistic effect on dopamine D2 receptors and serotonin 5-HT2A receptors. In many studies, it has been observed that D2 receptor antagonists exhibit anticancer properties both in vitro and in animal models to reduce tumor growth, cause apoptosis and induce autophagy.22 The D2 receptor pathway is hypothesized to play a role in promoting cell death due to reduced survivin expression. Some known psychotropic drugs which include olanzapine were previously considered as potential chemotherapeutic agents for the treatment or adjuvant treatment of cancer.34 In recent years, many studies have focused on discovering the link between serotonin and breast cancer. Undoubtedly, there is a connection here, because serotonin antagonists are known to prevent the development of breast cancer, but the full mechanism is still not understood33–42
It is known that such an activity profile, i.e. an antagonist of serotonin and dopamine receptors, is very beneficial in anti-cancer chemotherapeutics. This justifies undertaking research in the context of the use of olanzapine itself as well as its structural analogues for anti-cancer research, especially with regard to breast, lung, and ovarian cancers.
Considering the already known evidence for the anticancer activity of olanzapine43 and the recent results of studies in the context of the assessment of activity to MCF-7 human breast cancer cells,44 we decided to synthesize four new olanzapine derivatives and evaluate their activity to both MCF-7 and triple negative MDA-MB-231.
The methods of obtaining olanzapine by N-methylation of desmethylolanzapine (2-methyl-4-piperazin-1-yl-10H-thieno[2,3-b][1,5]benzodiazepine, DOLA) known so far in the literature are based on syntheses in the presence of sodium hydroxide or hydride, potassium carbonate, triethylamine. This is because an alkaline environment that favors the reaction needs to be created. The most common solvents used are methanol, acetone, dichloromethane, tetrahydrofuran, tert-butanol.45,46 The reaction time is 2–4 hours.
Thanks to previous experience where we successfully obtained olanzapine and its derivatives in conditions in the presence of microwave irradiation in less than one minute or using ultrasound conditions, we decided to use this greener alternative.47 Reactions were carried out under phase transfer catalysis conditions using dimethylformamide as solvent and more ecofrienly options like choline chloride/urea, which is a deeply eutectic solvent. Deep Eutectic Solvents (DES) and Natural Deep Eutectic Solvents (NADES) are eutectic mixtures comprised of one or more hydrogen bond donors and hydrogen bond acceptors (from natural origin in the case of NADES) and have shown great potential as solvents and catalysts for a variety of organic reactions.48–51 The advantages of DES and NADES over conventional organic solvents include low vapor pressure, low production costs, usually high biodegradability and recyclability. Moreover, DES and NADES can be prepared by a 100% atom economy, simply by mixing the components in the proper ratio and heating for a few hours.
Results
Synthesis of olanzapine
We started our research by developing a method of olanzapine synthesis in the presence of microwave irradiation. In this case, instead of the previously used microwave oven, we used a CEM Discovery reactor. In the first reactions, we tested the participation of a phase-transfer catalyst (PTC), i.e. TBAB (tetrabutylammonium bromide), as before. We conducted the experiment under pressure (entries 1, 2, Table 1) and under reflux (entry 3, Table 1). The reaction progress was assessed with TLC. In the next step, we assessed the effects of the addition of DABCO (1,4-diazabicyclo [2.2.2] octane) (entry 4, Table 1), which is often recommended for N-alkylation reactions52,53 and other PTCs (entries 5–9, Table 1), such as BTBAC (benzyltributylammonium chloride), TEAC (tetraethylammonium chloride), TMAB (tetramethylammonium borohydride), TEBA (benzyltriethylammonium chloride), CTAB (hexadecyltrimethylammonium bromide). The reactions proceeded by nucleophilic substitution, which is favored by an alkaline environment. That is why potassium carbonate or in one case DIPEA (N,N-diisopropylethylamine) was used in the process. DMF (dimethylformamide) was used as the solvent in the reactions, but a greener solvent, i.e. choline chloride/urea (NaDES), was also used for comparison (Scheme 1).
Table 1 Conditions for the synthesis of olanzapine derivatives in the reaction of DOLA (1 eq.) : CH3I (1.5 eq.) : K2CO3 or DIPEA (3 eq.) : catalyst (0.01 eq.) in the presence of microwave irradiation (MW) and ultrasound (“)))”)
No. |
Conditions |
Temp.a [°C] |
Pressureb [bar] |
Solvent/base/catalyst |
Reaction time [min] |
Yield [%] |
Highest observed data entered, the maximum temperature was set to 150 °C; reactions in the presence of ultrasound were carried out under reflux, due to the boiling point of CH3I (43 °C). Highest observed data entered, the maximum pressure was set to 10 bar. |
1 |
MW |
147 |
2.3 |
DMF/K2CO3/TBAB |
2 |
57 |
2 |
MW |
150 |
4.7 |
DMF/K2CO3/TBAB |
1.5 |
69 |
3 |
MW |
105 |
Atm |
DMF/K2CO3/TBAB |
1.5 |
48 |
4 |
MW |
150 |
3.2 |
DMF//K2CO3/DABCO |
1 |
71 |
5 |
MW |
150 |
1.9 |
DMF/K2CO3/BTBAC |
1 |
69 |
6 |
MW |
150 |
2.6 |
DMF/K2CO3/TEAC |
1 |
73 |
7 |
MW |
150 |
2.3 |
DMF/K2CO3/TMAB |
1 |
72 |
8 |
MW |
150 |
2.2 |
DMF/K2CO3/TEBA |
1 |
87 |
9 |
MW |
150 |
2.5 |
DMF/K2CO3/CTAB |
1 |
95 |
10 |
MW |
150 |
0.5 |
DMF/K2CO3/- |
1 |
38 |
11 |
MW |
150 |
0.5 |
NaDES/K2CO3/TBAB |
2 |
46 |
12 |
MW |
150 |
0.5 |
NaDES/K2CO3/- |
2 |
37 |
13 |
MW |
150 |
2.5 |
NaDES/Glycerine/K2CO3/TBAB |
2.5 |
53 |
14 |
))) |
45 |
Atm |
DMF/K2CO3/TBAB |
120 |
40 |
15 |
))) |
45 |
Atm |
DMF/K2CO3/DABCO |
120 |
45 |
16 |
))) |
45 |
Atm |
DMF/K2CO3/- |
120 |
15 |
17 |
))) |
45 |
Atm |
NaDES/K2CO3/TBAB |
120 |
25 |
18 |
))) |
45 |
Atm |
NaDES/DIPEA/TBAB |
120 |
61 |
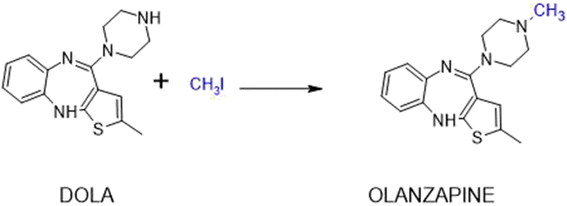 |
| Scheme 1 Synthesis of olanzapine. | |
The experiments carried out proved that olanzapine could be effectively obtained using all tested catalysts (Table 1) and solvents. In the case of reactions carried out in the presence of microwave irradiation and using DMF, the highest yields were obtained for CTAB (Y = 95%) and TEBA (Y = 87%). In other cases, the efficiency was not much lower and amounted to 69–73%. When no catalyst was used (entry 10, Table 1) only 38% yield was observed after 60 seconds. In the case of using NaDES, within 2 minutes, a yield of 46% was obtained with the catalyst and 25% in the reaction without the catalyst. When, apart from NaDES, glycerin was also used as a solvent, the yield of olanzapine was 53%.
We decided to choose TBAB and DABCO to continue research on olanzapine synthesis in ultrasound conditions, because it has several advantages: it is eco-friendly, inexpensive, acts as a non-toxic base, and can be recycled and reused.54,55 In the presence of ultrasonics in DMF, within 2 hours, the highest efficiency was obtained with DABCO, but in the case of TBAB it was also satisfactory (40%). As in the case of microwave irradiation, the lack of the catalyst caused a significant, i.e. more than two-fold decrease in the efficiency of obtaining olanzapine at the same time. In the case of NaDES, in the reaction in the presence of potassium carbonate, the yield was only 25%, but the exchange of the basic medium from solid to liquid increased the yield to 61%.
Synthesis of olanzapine derivatives
In the next stage, a study was carried out to obtain four new naphthalimidoalkyl derivatives of olanzapine (5–8), which differed only in the length of the alkyl chain (Scheme 2). Introduction of naphthalimidoalkyl moiety to DOLA core was aimed to augment the compound with structure bearing nicotinamide part of nicotinamide adenine dinucleotide (NAD+), which serves as an endogenous substrate of poly(ADP-ribose) polymerase (PARP) family of enzymes.56
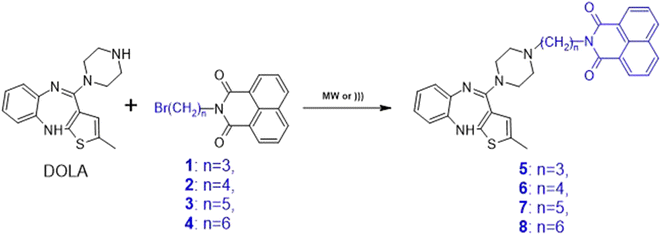 |
| Scheme 2 Synthesis of olanzapine derivatives 5–8. | |
Based on the experience in the synthesis of olanzapine, we decided to carry out the synthesis in the presence of microwave irradiation and ultrasound using similar reaction conditions (Table 2).
Table 2 Conditions for the synthesis of olanzapine derivatives in the reaction of DOLA (1 eq.) : 1–4 (1 eq.) : K2CO3 (3 eq.) : DABCO or TBAB (0.01 eq.) in MW or ultrasound
EntryEE no. |
Comp. |
n |
Conditions |
Temp.a [°C] |
Press.b [bar] |
Solvent/base/catalyst |
Reaction time [min] |
Yield [%] |
Highest observed data entered; the maximum temperature was set to 150 °C; reactions in the presence of ultrasound were carried out under reflux. Highest observed data entered; the maximum pressure was set to 10 bar. |
1 |
5 |
3 |
MW |
150 |
2.8 |
DMF/K2CO3/DABCO |
2 |
86 |
2 |
5 |
3 |
MW |
150 |
0.3 |
NaDES/K2CO3/TBAB |
2 |
30 |
3 |
5 |
3 |
MW |
150 |
4.3 |
NaDES/Glycerine/K2CO3/TBAB |
1.5 |
57 |
4 |
5 |
3 |
))) |
45 |
Atm |
DMF/K2CO3/TBAB |
60 |
16 |
5 |
5 |
3 |
))) |
45 |
Atm |
DMF/K2CO3/TBAB |
120 |
30 |
6 |
5 |
3 |
))) |
45 |
Atm |
H2O/K2CO3/TBAB |
60 |
0 |
7 |
5 |
3 |
))) |
45 |
Atm |
H2O/K2CO3/TBAB |
120 |
6 |
8 |
5 |
3 |
))) |
45 |
Atm |
H2O/K2CO3/DABCO |
60 |
0 |
9 |
5 |
3 |
))) |
65 |
Atm |
H2O/K2CO3/DABCO |
180 |
51 |
10 |
5 |
3 |
))) |
45 |
Atm |
NaDES/K2CO3/TBAB |
120 |
1 |
11 |
5 |
3 |
))) |
45 |
Atm |
NaDES/K2CO3/TBAB |
180 |
30 |
12 |
5 |
3 |
))) |
45 |
Atm |
NaDES/DIPEA/TBAB |
120 |
10 |
13 |
6 |
4 |
MW |
150 |
2.7 |
DMF/K2CO3/DABCO |
2 |
65 |
14 |
6 |
4 |
MW |
150 |
0.5 |
NaDES/K2CO3/TBAB |
2 |
47 |
15 |
6 |
4 |
MW |
150 |
1.5 |
NaDES/Glycerine/K2CO3/TBAB |
3 |
40 |
16 |
6 |
4 |
))) |
45 |
Atm |
H2O/K2CO3/DABCO |
120 |
1 |
17 |
6 |
4 |
))) |
65 |
Atm |
H2O/K2CO3/DABCO |
180 |
0 |
18 |
6 |
4 |
))) |
45 |
Atm |
NaDES/K2CO3/TBAB |
120 |
0 |
19 |
6 |
4 |
))) |
45 |
Atm |
NaDES/DIPEA/TBAB |
120 |
10 |
20 |
7 |
5 |
MW |
150 |
2.3 |
DMF/K2CO3/DABCO |
2 |
57 |
21 |
7 |
5 |
MW |
150 |
0.5 |
NaDES/K2CO3/TBAB |
2 |
36 |
22 |
7 |
5 |
MW |
150 |
4.2 |
NaDES/Glycerine/K2CO3/TBAB |
2 |
13 |
23 |
7 |
5 |
))) |
45 |
Atm |
H2O/K2CO3/DABCO |
120 |
7 |
24 |
7 |
5 |
))) |
65 |
Atm |
H2O/K2CO3/DABCO |
180 |
27 |
25 |
7 |
5 |
))) |
45 |
Atm |
NaDES/K2CO3/TBAB |
120 |
2 |
26 |
7 |
5 |
))) |
45 |
Atm |
NaDES/DIPEA/TBAB |
120 |
0 |
27 |
8 |
6 |
MW |
150 |
2.6 |
DMF/K2CO3/DABCO |
2 |
70 |
28 |
8 |
6 |
MW |
150 |
0.8 |
NaDES/K2CO3/TBAB |
2 |
31 |
29 |
8 |
6 |
MW |
150 |
2.8 |
NaDES/Glycerine/K2CO3/TBAB |
3 |
5 |
30 |
8 |
6 |
))) |
45 |
Atm |
H2O/K2CO3/DABCO |
120 |
0 |
31 |
8 |
6 |
))) |
65 |
Atm |
H2O/K2CO3/DABCO |
180 |
31 |
32 |
8 |
6 |
))) |
45 |
Atm |
NaDES/K2CO3/TBAB |
120 |
2 |
33 |
8 |
6 |
))) |
45 |
Atm |
NaDES/DIPEA/TBAB |
120 |
0 |
For each of the compounds, we performed syntheses in the presence of microwave irradiation using DMF and NaDES. In each case, higher yields (57–86%) were obtained with DMF, but despite the lower yields with NaDES (30–47%) or NaDES/Glycerine (5–53%), it is a very interesting synthesis option due to the non-toxic nature of the solvent. WhenNaDES was used in MW-assisted reactions, it was observed that the yield clearly decreases with the elongation of the linker, so the highest yields are observed for the compound with the propyl chain, and the lowest for the compound with the hexyl linker. In reactions in the presence of ultrasound, the highest yields for derivatives 5, 7 and 8 (Y = 27–51%) were obtained in the reaction with water as a solvent, in the presence of potassium carbonate and TBAB as a catalyst. In the case of derivative 6, product formation was observed only in the NaDES reaction (Y = 10%).
Cytotoxicity
As a result of the synthesis reaction, four new olanzapine derivatives 5–8 were obtained, which were then purified by crystallization from methanol. Next, they were subjected to biological tests to assess their anticancer potential against breast cancer cells, i.e. triple negative MDA-MB-231 and whether they were hormone-dependent (both estrogen and progesterone receptor positive) MCF7. The derivatives showed dose-dependent inhibitory effect against the viability of the cultured breast cancer cells (Fig. 1). The compounds 7 and 8 showed more pronounced cytotoxicity characterized by generally lower half maximal inhibitory concentration (IC50) and higher maximal inhibition compared to other derivatives (Table 3). Interestingly, both parent DOLA and olanzapine itself elicited little to no effect on cell fitness under the conditions of our assay.
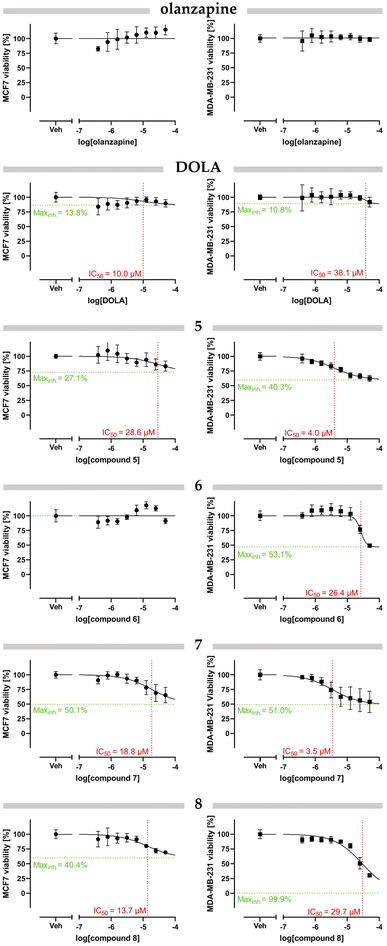 |
| Fig. 1 Dose-response curves for olanzapine, DOLA, and its derivatives (compounds 5–8). The MCF7 (left column) and MDA-MB-231 (right column) cell lines were exposed to the increasing concentrations of our compounds of interest or vehicle (0.1% DMSO). The viability of the cells was measured using MTS assay (see Materials and methods for more details). The obtained values were normalized to vehicle control and three-parameters sigmoidal curve was fitted. Half-maximal inhibitory concentrations (IC50 values, red dotted lines) and levels of maximal inhibition (Maxinh. values, green dotted lines) were calculated. | |
Table 3 Toxicity of olanzapine derivatives towards MCF7 and MDA-MB-231 breast cancer cell lines. Half-maximal inhibitory concentrations (IC50) and maximal inhibition levels were calculated by fitting the normalized experimental values to three-parameter sigmoidal curve equationa
Entry |
Compound |
MCF7 |
MDA-MB-231 |
IC50 |
Maximal inhibition |
IC50 |
Maximal inhibition |
n/a, not determined due to lack of significant inhibition of cell viability. |
1 |
Olanzapine |
n/d |
n/d |
n/d |
n/d |
2 |
DOLA |
10.0 μM |
13.8% |
38.1 μM |
10.8% |
3 |
5 |
28.6 μM |
27.1% |
4.0 μM |
40.3% |
4 |
6 |
n/d |
n/d |
26.4 μM |
53.1% |
5 |
7 |
18.8 μM |
50.1% |
3.5 μM |
51.0% |
6 |
8 |
13.7 μM |
40.4% |
29.7 μM |
99.9% |
In general, MDA-MB-231 cell line was more susceptible to the generated naphthalimidoalkyl derivatives of olanzapine compared to MCF7. As the naphthalimidoalkyl moiety is supposed to affect the activity of PARP enzymes, we decided to investigate the expression of PARP1 gene in cancer cell lines and to cross-compare the expression levels with the CRISPR-Cas9 viability screens using https://depmap.org/portal (Fig. 2). The gathered data indicate that MDA-MB-231 cells express more PARP1 and the MDA-MB-231 cell line is more susceptible to PARP1 knock-down compared to MCF7 cell line. These facts are in line with the cytotoxicity data.
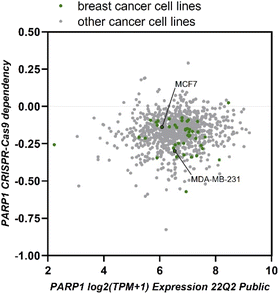 |
| Fig. 2 Dependency of cancer cell lines on PARP1 gene and the expression of PARP1 across cell lines. Data were retrieved from the https://depmap.org/portal. | |
Molecular modeling
To further corroborate the effects of the obtained naphthalimidoalkyl derivatives, compounds 5–8 were evaluated in a molecular modeling procedure using the known crystal structures of three key PARP polymerases, namely TNKS2, TNKS1, and PARP1 (ref. 57–59). In the case of synthesized olanzapine derivatives, the presence of a hydrogen bond with Gly 1032 and a π–π interaction with Tyr 1071 was observed in the complex with TNKS2. The 1,8-naphthalimide moiety was the most important fragment of the structure, directed deep into the binding pocket. Interactions of this type also appear in the reference complex (5S)-5-methyl-5-[4-(4-oxo-3,4-dihydroquinazolin-2-yl)phenyl]imidazolidine-2,4-dione with TNKS2, playing the key role in the activity. No additional stabilization was noted through the interactions in the tricyclic terminal region (Fig. 3A).
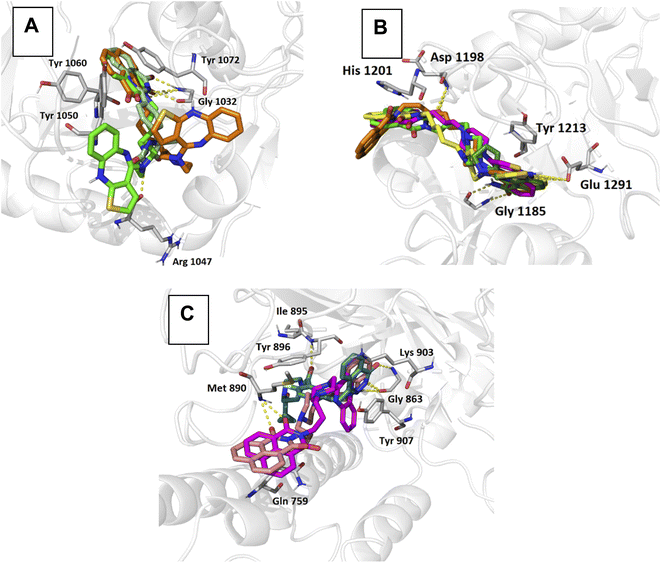 |
| Fig. 3 (A) Binding modes of (5S)-5-methyl-5-[4-(4-oxo-3,4-dihydroquinazolin-2-yl)phenyl]imidazolidine-2,4-dione (pale green), 7 (green), 8 (orange) into TNKS2. The yellow dash-lines indicate the hydrogen bonds. (B) Binding modes of veliparib (dark green), 5 (magenta), 6 (green), 7 (yellow), 8 (orange) into TNKS1. The yellow dash-lines indicate the hydrogen bonds. (C) Binding modes of olaparib (cyan), 5 (magenta), 8 (orange) into PARP1. The yellow dash-lines indicate the hydrogen bonds. | |
In conformations representing the binding mode in complexes with TNKS1, stabilizing π–π interactions with Tyr 1213 and Tyr 1224 residues were observed. In the designed molecules, there was no key interaction with Gly 1185, which may suggest a lack of affinity towards the tested protein target (Fig. 3B).
In complexes with PARP1, a coherent binding mode was obtained as compared to the conformation of the reference compound. In the docked poses of the designed ligands, stabilizing hydrogen bonds with Gly 863 and π-stacking with Tyr907 were observed, which are key interactions for the stability of the complex. Importantly, the long-chain derivatives showed a slightly biased orientation as compared to olaparib in the central region of the binding pocket. However, in the docked poses, additional stabilizing hydrogen-type and π–π interactions were observed in the terminal imide fragment (Fig. 3C).
Discussion
In conclusion, we obtained new alkyl derivatives of olanzapine by reaction under PTC conditions. The compounds were obtained in the presence of microwave irradiation or ultrasound. The main advantage of the 5–8 synthesis method is the possibility to carry out the reaction under green chemistry conditions, i.e. through the use of ecological NaDES solvent and short reaction time. The obtained compounds were tested for inhibition in tests using two metabolically different breast cancer cell lines MCF-7 and triple negative MDA-MB-231. The obtained results, which were additionally justified in the procedure of molecular modeling for three key PARP polymerases (TNKS2, TNKS1, PARP1), prove that olanzapine naphthalimides with a pentyl (7) or hexyl (8) chain have a very interesting profile because they show a clear activity in both tested cell lines. The key limitation of the current study is the lack of biological screening to determine the inhibitory activity of the synthesized compounds against TNKS2, TNKS1, and PARP1. This will be addressed in a separate study and will be reported elsewhere.
Methods
Chemistry. The reactions in the presence of microwave irradiation were carried out in a CEM Discover microwave reactor, while ultrasonic reactions were carried out in the PS-08 ultrasonic bath (80 W, 40 kHz). All reagents and solvents for the synthesis were purchased from Sigma-Aldrich, Merck, POCH, BIOSYNT CARBOSYNTH and used as received. NaDES – choline chloride/urea – was obtained in accordance with the procedure described in 2020.47Sigma-Aldrich silica plates: 200 μm, pore diameter: 60 Å, fluorescence index: 254 nm were used to evaluate the reaction progress with thin-layer chromatography (TLC) using 8
:
2 methanol
:
chloroform as eluent. Purification was carried out by column chromatography using silica gel 0.063–0.200 mm and methylene chloride
:
methanol 8
:
2 as eluent. UPLC-MS analyzes were performed with a Waters Acquity UPLC system – a Waters TQD (ESI-tandem quadrupole) mass spectrometer, PDA detector, Acquity UPLC BEH C18 column, 1.7, 2.1 × 100 mm (Waters Corporation, Milford, MA, USA), isocratically mobile phase: methanol
:
water + formic acid (4
:
6 + 0.1%, v/v). The 1H and 13C NMR spectra of products were recorded on a Bruker Avance 400 MHz spectrometer, using TMS as an internal reference. The detailed description of NMR spectra is provided in the ESI File.†
Cytotoxicity assessment. The cytotoxicity of the newly synthesized compounds was assessed using MTS assay as described before (ref. 60). The cell lines used in this study were human invasive breast ductal carcinoma MCF7 cell line (RRID:CVCL_0031) and human breast adeno-carcinoma cell line MDA-MB-231 (RRID:CVCL_0062). The drug exposure time was 72 h. DMSO (0.1%) was used as vehicle control.
Datamining. Data on gene expression and gene dependency were retrieved from DepMap Project portal available at https://depmap.org/portal/.
Molecular modeling. LigPrep 3.7 was used to prepare the three-dimensional structures. Epic. 2 was used to determine the respective ionization states at pH 7.4. The bond order and the respective ionization states of the amino acids were assigned using the protein preparation wizard. Grids were generated by centering a 35 Å grid field on the appropriate rest using the OPLS_2005 force field. Glide version 7.0 with flexible docking enabled was used for docking.
General procedure for the synthesis of olanzapine in MW. In a test tube equipped with magnetic stirrer bar, N-desmethylolanzapine (DOLA) (90 mg, 0.3 mmol, 1 equiv.), methyl iodide (28 μL, 0.45 mmol, 1.5 equiv.), potassium carbonate (124 mg, 0.9 mmol, 3 equiv.), PTC catalyst (0.03 mmol, 0.1 equiv.), and solvent (2 mL) were mixed under microwave irradiation for 1–2 min. The reaction progress was monitored with TLC. After the reaction, 15 mL of water was added to the mixture and extracted with three portions of methylene chloride. The organic layer was evaporated to dryness, which was then crystallized from methanol or purified on a column chromatography.
General procedure for the synthesis of olanzapine in ultrasound. In a test tube N-desmethylolanzapine (DOLA) (90 mg, 0.3 mmol, 1 equiv.), methyl iodide (28 μL, 0.45 mmol, 1.5 equiv.), potassium carbonate or DIPEA (0.9 mmol, 3 equiv.), PTC catalyst (0.03 mmol, 0.1 equiv.), and solvent (5 mL) placed in an ultrasonic bath and kept at 45–50 °C for 1–3 h. The reaction progress was monitored with TLC. After the reaction, 15 mL of water was added to the mixture and extracted with three portions of methylene chloride. The organic layer was evaporated to dryness, which was then crystallized from methanol or purified on a column chromatography.
General procedure for the synthesis of 5–8 in MW. In a test tube equipped with magnetic stirrer bar, N-desmethylolanzapine (DOLA) (90 mg, 0.3 mmol, 1 equiv.), naphthalimide alkyl derivative 1–4 (0.30 mmol, 1 equiv.), potassium carbonate or DIPEA (0.9 mmol, 3 equiv.), PTC catalyst (0.03 mmol, 0.1 equiv.), and solvent (2 mL) were mixed under microwave irradiation for 1–2 min. The reaction progress was monitored with TLC. After the reaction, 15 mL of water was added to the mixture and extracted with three portions of methylene chloride. The organic layer was evaporated to dryness, which was then crystallized from methanol or purified on a column chromatography.
General procedure for the synthesis of 5–8 in ultrasound. In a test tube N-desmethylolanzapine (DOLA) (90 mg, 0.3 mmol, 1 equiv.), naphthalimide alkyl derivative 1–4 (0.30 mmol, 1 equiv.), potassium carbonate or DIPEA (0.9 mmol, 3 equiv.), PTC catalyst (0.03 mmol, 0.1 equiv.) and solvent (5 mL) placed in an ultrasonic bath and kept at 45–50 °C for 1–3 h. The reaction progress was monitored with TLC. After the reaction, 15 mL of water was added to the mixture and extracted with three portions of methylene chloride. The organic layer was evaporated to dryness, which was then crystallized from methanol or purified on a column chromatography.
Data availability
All data generated or analyzed during this study are included in this published article [and its ESI† File].
Author contributions
A. K. D., W. T., W. B. running experiment, D. K. interpretation of NMR test results, P. Z. molecular modelling research, A. A., A. W., biological research – planning, experiments and interpretation, A. T. A. D. research concept using NaDES, manuscript revision, J. J. supervision, conceptualization, methodology, investigation, visualization, writing original draft.
Conflicts of interest
The authors declare no competing interests.
References
- S. Dodd and M. Berk, Olanzapine/fluoxetine combination for treatment-resistant depression: efficacy and clinical utility, Expert Rev. Neurother., 2008, 8, 1299–1306, DOI:10.1586/14737175.8.9.1299.
- C. Monahan, L. McCoy, J. Powell and J. G. Gums, Olanzapine/Samidorphan: New Drug Approved for Treating Bipolar I Disorder and Schizophrenia, Ann. Pharmacother., 2022, 56, 1049–1057, DOI:10.1177/10600280211070330.
- J. Faden, R. Serdenes and L. Citrome, Olanzapine-samidorphan combination tablets for the treatment of schizophrenia and bipolar I disorder - what is it, and will it be used?, Expert Rev. Neurother., 2022, 22, 365–376, DOI:10.1080/14737175.2022.2060742.
- T. Sanomachi, S. Suzuki, K. Kuramoto, H. Takeda, H. Sakaki, K. Togashi, S. Seino, T. Yoshioka, M. Okada and C. Kitanaka, Olanzapine, an Atypical Antipsychotic, Inhibits Survivin Expression and Sensitizes Cancer Cells to Chemotherapeutic Agents, Anticancer Res., 2017, 37, 6177–6188, DOI:10.21873/anticanres.12067.
- J. Sato, M. Kashiwaba, H. Komatsu, K. Ishida, S. Nihei and K. Kudo, Effect of olanzapine for breast cancer patients resistant to triplet antiemetic therapy with nausea due to anthracycline-containing adjuvant chemotherapy, Jpn. J. Clin. Oncol., 2016, 45, 415–420, DOI:10.1093/jjco/hyw011.
- L. K. Lazowski, B. Townsend, E. R. Hawken, R. Jokic, R. du Toit and R. Milev, Sleep architecture and cognitive changes in olanzapine-treated patients with depression: a double blind randomized placebo controlled trial, BMC Psychiatry, 2014, 14, 1–11, DOI:10.1186/1471-244X-14-202.
- M. Kluge, A. Schacht, H. Himmerich, C. Rummel-Kluge, P. M. Wehmeier, M. Dalal, D. Hinze-Selch, T. Kraus, R. W. Dittmann, T. Pollmächer and A. Schuld, Olanzapine and clozapine differently affect sleep in patients with schizophrenia: results from a double-blind, polysomnographic study and review of the literature, Schizophr. Res., 2014, 152, 255–260, DOI:10.1016/j.schres.2013.11.009.
- J. M. Monti, P. Torterolo and S. R. Pandi Perumal, The effects of second generation antipsychotic drugs on sleep variables in healthy subjects and patients with schizophrenia, Sleep Med. Rev., 2017, 33, 51–57, DOI:10.1016/j.smrv.2016.05.002.
- H. Khazaie, L. Rezaie, F. Darvishi, F. Najafi and K. Avis, Treatment of paradoxical insomnia with atypical antipsychotic drugs. A comparison of olanzapine and risperidone, Neurosciences, 2013, 18, 64–69, DOI:10.1016/j.sleep.2013.11.389.
- K. Torigoe, K. Nakahara, M. Rahmadi, K. Yoshizawa, H. Horiuchi, S. Hirayama, S. Imai, N. Kuzumaki, T. Itoh, A. Yamashita, K. Shakunaga, M. Yamasaki, H. Nagase, M. Matoba, T. Suzuki and M. Narita, Usefulness of olanzapine as an adjunct to opioid treatment and for the treatment of neuropathic pain, Anesthesiol, 2012, 116, 159–169, DOI:10.1097/ALN.0b013e31823c7e56.
- S. Schreiber, V. Getslev, M. M. Backer, R. Weizman and C. G. Pick, The atypical neuroleptics clozapine and olanzapine differ regarding their antinociceptive mechanisms and potency, Pharmacol., Biochem. Behav., 1999, 64, 75–80, DOI:10.1016/S0091-3057(99)00107-0.
- C. L. Gick, G. W. Mirowski, J. S. Kennedy and F. P. Bymaster, Treatment of glossodynia with olanzapine, J. Am. Acad. Dermatol., 2004, 51, 463–465, DOI:10.1016/j.jaad.2004.02.016.
- X. F. Jimenez, T. Sundararajan and E. C. Covington, A systematic review of atypical antipsychotics in chronic pain management: olanzapine demonstrates potential in central sensitization, fibromyalgia, and headache/migraine, Clin. J. Pain, 2018, 34, 585–591, DOI:10.1097/AJP.0000000000000567.
- S. D. Silberstein, M. F. Peres, M. M. Hopkins, A. L. Shechter, W. B. Young and T. D. Rozen, Olanzapine in the treatment of refractory migraine and chronic daily headache, Headache, 2002, 42, 515–518, DOI:10.1046/j.1526-4610.2002.02126.x.
- E. Attia, J. E. Steinglass, B. T. Walsh, Y. Wang, P. Wu, C. Schreyer, J. Wildes, Z. Yilmaz, A. S. Guarda, A. S. Kaplan and M. D. Marcus, Olanzapine Versus Placebo in Adult Outpatients With Anorexia Nervosa: A Randomized Clinical Trial, Am. J. Psychiatry, 2019, 176, 449–456, DOI:10.1176/appi.ajp.2018.18101125.
- R. Han, Q. Bian and H. Chen, Effectiveness of olanzapine in the treatment of anorexia nervosa: A systematic review and meta-analysis, Brain Behav., 2022, 12, e2498, DOI:10.1002/brb3.2498.
- M. P. Davis and G. J. Sanger, The Benefits of Olanzapine in Palliating Symptoms, urr. Treat. Options Oncol., 2020, 22, 5, DOI:10.1007/s11864-020-00804-1.
- S. Jafari, M. E. Bouillon, X. F. Huang, S. G. Pyne and F. Fernandez-Enright, Novel olanzapine analogues presenting a reduced H1 receptor affinity and retained 5HT2A/D2 binding affinity ratio, BMC Pharmacol., 2012, 12, 8, DOI:10.1186/1471-2210-12-8.
- S. Jafari, X.-F. Huang, J. L. Andrews and F. Fernandez-Enright, In Vivo Pharmacological Evaluations of Novel Olanzapine Analogues in Rats: A Potential New Avenue for the Treatment of Schizophrenia, PLoS One, 2013, 8, e80979, DOI:10.1371/journal.pone.0080979.
- D. R. Gorja, S. Mukherjee, C. L. T. Meda, G. S. Deora, K. Lalith Kumar, A. Jain, G. H. Chaudhari, K. S. Chennubhotla, R. K. Banote, P. Kulkarni, K. V. Parsa, K. Mukkanti and M. Pal, Novel N-indolylmethyl substituted olanzapine derivatives: their design, synthesis and evaluation as PDE4B inhibitors, Org. Biomol. Chem., 2013, 11, 2075, 10.1039/c3ob27424a.
- P. Vijaya Babu, D. R. Gorja, C. L. T. Meda, G. S. Deora, S. K. Kolli, K. V. L. Parsa, K. Mukkanti and M. Pal, Synthesis of N-(3-arylprop-2-ynyl)substituted olanzapine derivatives as potential inhibitors of PDE4B, Tetrahedron Lett., 2014, 55, 3176–3180, DOI:10.1016/j.tetlet.2014.04.009.
- J. S. Weissenrieder, J. D. Neighbors, R. B. Mailman and R. J. Hohl, Cancer and he dopamine D2 receptor: a pharmacological perspective, J. Pharmacol. Exp. Ther., 2019, 370, 111–126, DOI:10.1124/jpet.119.256818.
- C. L. B. Kline, M. D. Ralff, A. R. Lulla, J. M. Wagner, P. H. Abbosh, D. T. Dicker, J. E. Allen and W. S. El-Deiry, Role of dopamine receptors in the anticancer activity of ONC201, Neoplasia, 2018, 20, 80–91, DOI:10.1016/j.neo.2017.10.002.
- B. Sonier, M. Arseneault, C. Lavigne, R. J. Ouellette and C. Vaillancourt, The 5-HT2A serotoninergic receptor is expressed in the MCF-7 human breast cancer cell line and reveals a mitogenic effect of serotonin, Biochem. Biophys. Res. Commun., 2006, 343, 1053–1059, DOI:10.1016/j.bbrc.2006.03.080.
- Y. Ballou, A. Rivas, A. Belmont, L. Patel, C. N. Amaya, S. Lipson, T. Khayou, E. B. Dickerson, Z. Nahleh and B. A. Bryan, 5-HT serotonin receptors modulate mitogenic signaling and impact tumor cell viability, Mol. Clin. Oncol., 2018, 9, 243–254, DOI:10.3892/mco.2018.1681.
- S. Suzuki, M. Okada, K. Kuramoto, H. Takeda, H. Sakaki, H. Watarai, T. Sanomachi, S. Seino, T. Yoshioka and C. Kitanaka, Aripiprazole, an antipsychotic and partial dopamine agonist, inhibits cancer stem cells and reverses chemoresistance, Anticancer Res., 2016, 36, 5153–5161, DOI:10.21873/anticanres.11085.
- Y. C. Yin, C. C. Lin, T. T. Chen, J. Y. Chen, H. J. Tsai, C. Y. Wang and S. Y. Chen, Clozapine induces autophagic cell death in non-small cell lung cancer cells, Cell. Physiol. Biochem., 2015, 35, 945–956, DOI:10.1159/000369751.
- E. D. Wiklund, V. S. Catts, S. V. Catts, T. F. Ng, N. J. Whitaker, A. J. Brown and L. H. Lutze-Mann, Cytotoxic effects of antipsychotic drugs implicate cholesterol homeostasis as a novel chemotherapeutic target, Int. J. Cancer, 2010, 126, 28–40, DOI:10.1002/ijc.24813.
- C. T. Yeh, A. T. H. Wu, P. M. Chang, K. Y. Chen, C. N. Yang, S. C. Yang, C. C. Ho, C. C. Chen, Y. L. Kuo, P. Y. Lee, Y. W. Liu, C. C. Yen, M. Hsiao, P. J. Lu, J. M. Lai, L. S. Wang, C. H. Wu, J. F. Chiou, P. C. Yang and C. Y. Huang, Trifluoperazine, an antipsychotic agent, inhibits cancer stem cell growth and overcomes drug resistance of lung cancer, Am. J. Respir. Crit. Care Med., 2012, 186, 1180–1188, DOI:10.1164/rccm.201207-1180OC.
- A. Ranjan, P. Gupta and S. K. Srivastava, Penfluridol: an antipsychotic agent suppresses metastatic tumor growth in triple-negative breast cancer by inhibiting integrin signaling axis, Cancer Res., 2016, 76, 877–890, DOI:10.1158/0008-5472.CAN-15-1233.
- H. W. Cheng, Y. H. Liang, Y. L. Kuo, C. P. Chuu, C. Y. Lin, M. H. Lee, A. T. Wu, C. T. Yeh, E. I. Chen, J. Whang-Peng, C. L. Su and C. Y. Huang, Identification of thioridazine, an antipsychotic drug, as an antiglioblastoma and anticancer stem cell agent using public gene expression data, Cell Death Dis., 2015, 6, e1753, DOI:10.1038/cddis.2015.77.
- J.-K. Lee, D.-H. Nam and J. Lee, Repurposing antipsychotics as glioblastoma therapeutics: potentials and challenges, Oncol. Lett., 2016, 11, 1281–1286, DOI:10.3892/ol.2016.4074.
- T. Sanomachi, S. Suzuki, K. Kuramoto, H. Takeda, H. Sakaki, K. Togashi, S. Seino, T. Yoshioka, M. Okada and C. Kitanaka, Olanzapine, an Atypical Antipsychotic, Inhibits Survivin Expression and Sensitizes Cancer Cells to Chemotherapeutic Agents, Anticancer Res., 2017, 37, 6177–6188, DOI:10.21873/anticanres.12067.
- D. Sarrouilhe and M. Mesnil, Serotonin and human cancer: A critical view, Biochimie, 2019, 161, 46–50, DOI:10.1016/j.biochi.2018.06.016.
- M. A. M. Peters, C. Meijer, R. S. N. Fehrmann, A. M. E. Walenkamp, I. P. Kema, E. G. E. de Vries, H. Hollema and S. F. Oosting, Serotonin and Dopamine Receptor Expression in Solid Tumours Including Rare Cancers, Pathol. Oncol. Res., 2020, 26, 1539–1547, DOI:10.1007/s12253-019-00734-w.
- D. Pereyra and P. Starlinger, Reply to: Intra-platelet serotonin in prognosis of tumorigenesis: Friend or foe?, J. Hepatol., 2018, 68, 1334–1335, DOI:10.1016/j.jhep.2018.03.008.
- B. N. Barmanbay and E. O. Altuntaş, The Role of Serotonin in Breast Cancer, J. Exp. Basic Med. Sci., 2022, 3, 221–226, DOI:10.5606/jebms.2022.1031.
- S. J. Warchal, J. C. Dawson, E. Shepherd, A. F. Munro, R. E. Hughes and A. Makda, et al., High content phenotypic screening identifies serotonin receptor modulators with selective activity upon breast cancer cell cycle and cytokine signaling pathways, Bioorg. Med. Chem., 2020, 28, 115209, DOI:10.1016/j.bmc.2019.115209.
- M. Sola-Penna, L. P. Paixão, J. R. Branco, A. C. Ochioni, J. M. Albanese, D. M. Mundim, D. Baptista-de-Souza, C. P. Figueiredo, W. S. Coelho, M. C. Marcondes and P. Zancan, Serotonin activates glycolysis and mitochondria biogenesis in human breast cancer cells through activation of the Jak1/STAT3/ERK1/2
and adenylate cyclase/PKA, respectively, Br. J. Cancer, 2020, 122, 194–208, DOI:10.1038/s41416-019-0640-1.
- N. Kiliç and O. Erbaş, Antidepressant Drugs, Biological Clocks, and Cancer: Is There a Relation, J. Exp. Basic Med. Sci., 2021, 2, 298–301, DOI:10.5606/jebms.2021.75670.
- B. Boursi, I. Lurie, K. Haynes, R. Mamtani and Y. X. Yang, Chronic therapy with selective serotonin reuptake inhibitors and survival in newly diagnosed cancer patients, Eur. J. Cancer Care, 2018, 27, e12666, DOI:10.1111/ecc.12666.
- M. A. Schneider, L. Heeb, M. M. Beffinger, S. Pantelyushin, M. Linecker, L. Roth, K. Lehmann, U. Ungethüm, S. Kobold, R. Graf, M. van den Broek, J. Vom Berg, A. Gupta and P. A. Clavien, Attenuation of peripheral serotonin inhibits tumor growth and enhances immune checkpoint blockade therapy in murine tumor models, Sci. Transl. Med., 2021, 13, eabc8188, DOI:10.1126/scitranslmed.abc8188.
- T. Sanomachi, S. Suzuki, K. Kuramoto, H. Takeda, H. Sakaki, K. Togashi, S. Seino, T. Yoshioka, M. Okada and C. Kitanaka, Olanzapine, an atypical antipsychotic, inhibits survivin expression and sensitizes cancer cells to chemotherapeutic agents, Anticancer Res., 2017, 37, 6177–6188, DOI:10.21873/anticanres.12067.
- V. A. Varahi, N. Poojith, G. Mohan Krishna, D. Darling Chellatha, M. Vijayalakshmi, P. Krishna Murthy and Y. Sheena, Antiproliferative Effects of Olanzapine against MCF-7 Cells and Its Molecular Interactions with Survivin, Int. J. Nutr., Pharmacol., Neurol. Dis., 2022, 12, 72–78, DOI:10.4103/ijnpnd.IJNPND_82_21.
- H. V. Patel, A. K. Ray, P. B. Patel and M. R. Patel, Process of preparation of olanzapine form I, US Pat.US20040048854A1, 2004 Search PubMed.
- T. Mesar, A. Copar, H. Sturm and J. Ludescher, Synthesis of 2-Methyl-4-(4-Methyl-1-Piperazinly)-10H-Thieno(2,3-B) (1,5) Benzodiazepine and Salts Thereof, US Pat., US20080161557A1, 2008 Search PubMed.
- J. Jaśkowska, P. Kowalski, D. Kułaga and Z. Majka, A new solvent-free method for the synthesis of olanzapine and its derivatives, Przemysl Chem., 2016, 10, 1918–1920, DOI:10.15199/62.2016.10.12.
- A. Tzani, C. Vaitsis, E. Kritsi, M. Smiljkovic, M. Sokovic, P. Zoumpoulakis and A. Detsi, Green synthesis of bis-(β-dicarbonyl)-methane derivatives and biological evaluation as putative anticandidial agents, J. Mol. Struct., 2020, 1216, 128276, DOI:10.1016/j.molstruc.2020.128276.
- M. A. Karadendrou, I. Kostopoulou, V. Kakokefalou, A. Tzani and A. Detsi, L-Proline-Based Natural Deep Eutectic Solvents as Efficient Solvents and Catalysts for the Ultrasound-Assisted Synthesis of Aurones via Knoevenagel Condensation, Catalysts, 2022, 12, 249, DOI:10.3390/catal12030249.
- M. Molnar, M. Lončarić, M. Jakovljević, M. Komar and M. Lončar, Some applications of deep eutectic solvents in alkylation of heterocyclic compounds: A review of the past 10 years, Heterocycl. Commun., 2021, 27, 45–56, DOI:10.1515/hc-2020-0122.
- D. A. Alonso, A. Baeza, R. Chinchilla, G. Guillena, I. M. Pastor and D. J. Ramón, Deep eutectic solvents: the organic reaction medium of the century, Eur. J. Org. Chem., 2016, 4, 612–632, DOI:10.1002/ejoc.201501197.
- W. C. Shieh, M. Lozanov and O. Repic, Accelerated benzylation reaction utilizing dibenzyl carbonate as an alkylating reagent, Tetrahedron Lett., 2003, 44, 6943–6945, DOI:10.1016/S0040-4039(03)01711-8.
- B. Bita, 1,4-Diazabicyclo[2.2.2]octane (DABCO) as a useful catalyst in organic synthesis, Eur. J. Chem., 2010, 1, 54–60, DOI:10.5155/eurjchem.1.1.54-60.2.
- K. J. Dinesh, DABCO as a Base and an Organocatalyst in Organic Synthesis: A Review, Curr. Green Chem., 2020, 7, 146–162, DOI:10.2174/2213346107666191227101538.
- B. K. Banik, B. Banerjee, G. Kaur, S. Saroch and R. Kumar, Tetrabutylammonium Bromide (TBAB) Catalyzed Synthesis of Bioactive Heterocycles, Molecules, 2020, 25, 5918, DOI:10.3390/molecules25245918.
- N. J. Curtin and C. Szabo, Therapeutic applications of PARP inhibitors: Anticancer therapy and beyond, Mol. Aspects Med., 2013, 34, 1217–1256, DOI:10.1016/j.mam.2013.01.006.
- Y. Nkizinkiko, T. Haikarainen and L. Lehtio, Crystal structure of TNKS2 in complex with (5S)-5-methyl-5-[4-(4-oxo-3,4-dihydroquinazolin-2-yl)phenyl]imidazolidine-2,4-dione (PDB ID: 5OWT), 2018, DOI:10.2210/pdb5OWT/pdb.
- K. S. Gajiwala and K. Ryan, Structure of the catalytic domain of tankyrase 1 in complex with veliparib (PDB ID: 7KKQ), 2021, DOI:10.2210/pdb7KKQ/pdb.
- K. S. Gajiwala and K. Ryan, Structure of the catalytic domain of PARP1 in complex with olaparib (PDB ID: 7KK4), 2021, DOI:10.2210/pdb7KK4/pdb.
- P. Zaręba, A. K. Drabczyk, A. Wnorowski, E. Pindelska, G. Latacz and J. Jaśkowska, Eco-friendly methods of synthesis and preliminary biological evaluation of sulfonamide derivatives of cyclic arylguanidines, Ultrason. Sonochem., 2022, 90, 106165, DOI:10.1016/j.ultsonch.2022.106165.
|
This journal is © The Royal Society of Chemistry 2023 |
Click here to see how this site uses Cookies. View our privacy policy here.