DOI:
10.1039/D3RA03651K
(Paper)
RSC Adv., 2023,
13, 21231-21235
Microwave-accelerated cross-dehydrogenative-coupling (CDC) of N-(quinolin-8-yl)amides with acetone/acetonitrile under metal-free conditions†
Received
31st May 2023
, Accepted 7th July 2023
First published on 13th July 2023
Abstract
A highly selective remote C(sp3)–H acetonation of N-(quinolin-8-yl)amide scaffolds at the C5-position under microwave irradiation has been developed. In the absence of a transition-metal-catalyst, benzoyl peroxide (BPO)-promoted cross-dehydrogenation coupling (CDC) of N-(quinolin-8-yl)amides with acetone/acetonitrile occurred smoothly to generate the corresponding 5-acetonated/acetonitriled N-(quinolin-8-yl)amides in good yields. The transformation is operationally simple, rapid, easily scaled-up to the gram scale, and shows a broad substrate scope.
Quinolines play an important role as nitrogen-containing heterocyclic compounds in natural products, pharmaceuticals and pesticides (Fig. 1).1 As a particular member of the quinoline compounds, N-(quinolin-8-yl)benzamide has an irreplaceable role in the C–H bond activation reaction system as a classical bidentate directing group or as a ligand auxiliary.2 In recent years, brilliant achievements have been made in C–H bond functionalization reactions accomplished at the C-2,3 C-3,4 C-4,5 C-5 and C-8 (ref. 6) positions of quinoline and its derivatives. It is worth mentioning that the C-5 position functionalization reactions of N-(quinolin-8-yl)benzamide have a certain medicinal value with high activity and involve more types of reactions, such as amidation,7 sulfonylation,8 cyanoalkoxylation,9 allylation,10 alkylation,11 halogenation12 and so on.13
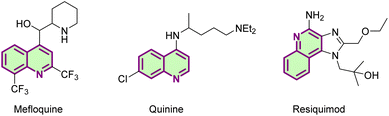 |
| Fig. 1 Representative drug candidates and natural products with quinoline skeleton. | |
In the past decades, with the boom in the area of inert C–H bond activation, cross-dehydrogenation coupling (CDC) reactions involving inert C–H bond activation have been developed,14 which was pioneered by Li in 2014.15 Currently, their group has not only constructed intermolecular C–C bond reactions by CDC of inert C(sp3)–H bonds,16 but also achieved CDC reactions of C(sp3)–H heteroarylation between alkanes and heteroarenes by visible light irradiation.17 Subsequently, CDC-type reactions have been widely used and rapidly developed (Scheme 1). Among the CDC reactions, there are even rarer dehydro-coupling reactions in which acetonitrile/acetone was used as a reaction substrate, for example, oxidant-promoted CDC reactions reported by Kianmehr,18 and Tan et al.19
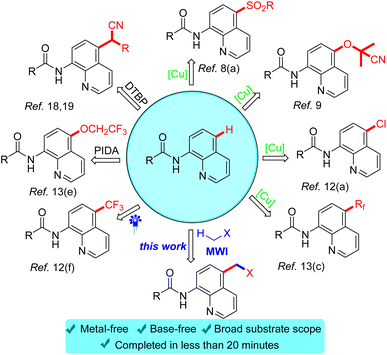 |
| Scheme 1 The C-5 position functionalization reactions of N-(quinolin-8-yl)amide. | |
Since microwave contain many advantages such as short reaction time and environmental friendliness which have been used in organic reactions, it has already attracted the attention of chemists and achieved remarkable results in the field of C–H bond activation.20 In the past few years, microwave-facilitated organic synthesis has developed into one of the most powerful tools in organic chemistry. Herein we wish to report a microwave-accelerated cross-dehydrogenative-coupling (CDC) of N-(quinolin-8-yl)amides with acetone/acetonitrile in the presence of benzoyl peroxide (BPO) as oxidant under metal-free conditions, delivering good yields of C-5-acetonated N-(quinolin-8-yl)amides as products.
We initiated our study with microwave irradiation of N-(quinolin-8-yl)benzamide (1a) and acetone (2a) in the presence of benzoyl peroxide (BPO). After 20 min at 100 °C, the desired acetonation product 3a was obtained in 62% yield (Table 1, entry 1). The reaction did not proceed with other oxidants such as H2O2, DTBP, TBHP and LPO (entries 2–5). Screening of oxidant loading indicated that 2.0 equiv. is the most effective choice for the reaction, afforded the product 3a in 71% yield (entries 6–9). Investigation of the reaction time and temperature gave no obvious improvement in the product yield of 3a (entries 10–16). Interestingly, when the model reaction was carried out in an oil bath at 100 °C for 12 h, only 18% yield of 3a was obtained (entry 17). For detailed optimization of reaction conditions, please see the (Tables S1–S4 in ESI).†
Table 1 Optimization of the reaction conditionsa

|
Entry |
Oxidant |
Time (min) |
T (°C) |
Yieldb (%) |
Reaction conditions: N-(quinolin-8-yl)benzamide (1a, 0.10 mmol), BPO, acetone (2a, 3.0 mL) under microwave irradiation. Isolated yield. In an oil bath at 100 °C for 12 h [DTBP = di-tert-butyl peroxide; TBHP = tert-butyl hydroperoxide; LPO = dilauroyl peroxide; n.r. = no reaction]. |
1 |
BPO |
20 |
100 |
62 |
2 |
H2O2 |
20 |
100 |
n.r. |
3 |
DTBP |
20 |
100 |
n.r. |
4 |
LPO |
20 |
100 |
Trace |
5 |
TBHP |
20 |
100 |
n.r. |
6 |
BPO (1.0 equiv.) |
20 |
100 |
41 |
7 |
BPO (2.0 equiv.) |
20 |
100 |
71 |
8 |
BPO (2.5 equiv.) |
20 |
100 |
73 |
9 |
BPO (3.0 equiv.) |
20 |
100 |
72 |
10 |
BPO (2.0 equiv.) |
30 |
100 |
69 |
11 |
BPO (2.0 equiv.) |
15 |
100 |
60 |
12 |
BPO (2.0 equiv.) |
10 |
100 |
47 |
13 |
BPO (2.0 equiv.) |
20 |
110 |
70 |
14 |
BPO (2.0 equiv.) |
20 |
120 |
71 |
15 |
BPO (2.0 equiv.) |
20 |
90 |
40 |
16 |
BPO (2.0 equiv.) |
20 |
80 |
32 |
17 |
BPO (2.0 equiv.) |
12 (h) |
100 |
18c |
Under optimal reaction conditions, we next investigated the substrate scope of acetonation reaction (Scheme 2). A variety of N-(quinolin-8-yl)arylamides with para-substituents, such as Me, MeO, tBu, CO2Me, F, Cl and Br groups, in differed electronic properties are well tolerated (3a–3h), affording the desired C-5-acetonated N-(quinolin-8-yl)arylamides in good yields. Some meta-substituted groups including Me, F and CF3 on the benzene ring in N-(quinolin-8-yl)arylamides were examined, and exhibited good reactivity in the reaction, providing the corresponding products (3i–3k) in 46–53% yields. When disubstituted N-(quinolin-8-yl)arylamide 1l was employed in this transformation, the corresponding product 3l was afforded in 40% yield. In addition, the reactions with an electron-donating group (Me or OMe) or an electron-withdrawing group (F or Cl) at the C2-position of the aryl rings generated the products 3m–3p with 49–71% yields. Notably, N-(quinolin-8-yl)-1-naphthamide reacted with acetone, affording the desired product 3q in 43% yield.
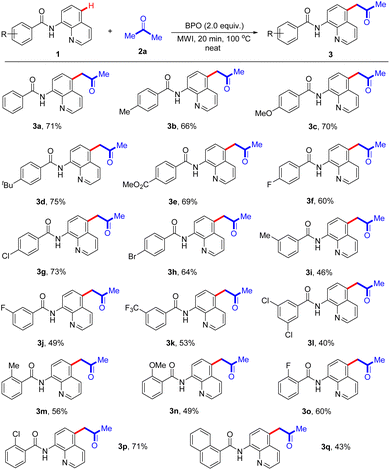 |
| Scheme 2 The substrates scope of N-(quinolin-8-yl)arylamides [reaction conditions: 1 (0.10 mmol), BPO (2.0 equiv.) and acetone (2a, 3.0 mL) under microwave irradiation at 100 °C for 20 min; isolated yield of the product]. | |
It is gratifying that the aliphatic-derived N-(quinolin-8-yl) amides reacted acetone under optimal conditions, indicating that it is not affected by the length of the aliphatic carbon chain, and the corresponding products 3r–3w were isolated in 69–81% yields (Scheme 3). It is also worth mentioning that the presence of a methyl substituent at the C-6 or C-3 position of the quinoline ring could also formed the desired products (3x and 3y) in 54% and 69%, respectively. Additionally, cyclopropanamide and cyclohexanamide also participated in the reaction and the acetonation products 3z and 3aa could be obtained in good yields (71% and 83%, respectively). It should be noted that N-(quinolin-8-yl)nicotinamide 1ab underwent the reaction smoothly with 2a to give the corresponding product 3ab in 60% yield. Encouragingly, when the quinoline moiety was replaced with naphthalene one, the obtained compounds were also compatible with this reaction, giving the anticipated products 3ac–3ae in 59–65% yields. Moreover, the optimized conditions were also suitable for the reaction of N-(5-bromonaphthalen-1-yl)picolinamide with acetone (2a), and the expected product (3af) was formed in 43% yield. Furthermore, when 1.0 g of 1a was subjected to the reaction, the targeted product 3a was obtained in 78% yield (Scheme 4).
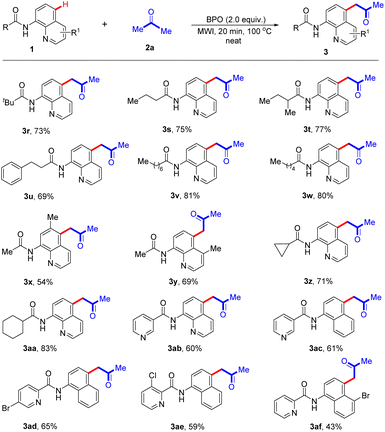 |
| Scheme 3 The substrates scope of N-(quinolin-8-yl)aliphatic amides and N-(quinolin-8-yl)heteroarylamides [reaction conditions: 1 (0.10 mmol), BPO (2.0 equiv.) and acetone (2a, 3.0 mL) under microwave irradiation at 100 °C for 20 min; isolated yield of the product]. | |
 |
| Scheme 4 Gram-scale reaction. | |
Remarkably, the reaction system was also capable of cyanomethylation reaction under optimal conditions, the results of which were summarized in Scheme 5. We were delighted to observe the acetonitrile was good coupling partner, enabling the production of 4a in 71% yield. Additionally, this reaction can tolerate functional groups such as N-(quinolin-8-yl)arylamides with para-Me (1b) and aliphatic-derived N-(quinolin-8-yl)amides can afford the corresponding products in 66%, 75%, and 67% yield, respectively.
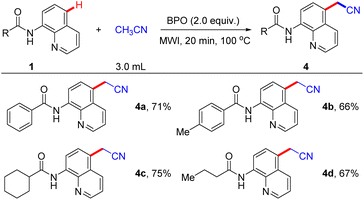 |
| Scheme 5 The substrates scope of N-(quinolin-8-yl)arylamides and N-(quinolin-8-yl)aliphaticamides [reaction conditions: 1 (0.10 mmol), BPO (2.0 equiv.) and acetonitrile (3.0 mL) under microwave irradiation at 100 °C for 20 min; isolated yield of the product]. | |
To gain insight into the reaction mechanism, the radical trapped experiments were conducted (Scheme 6). When a radical scavenger 2,2,6,6-tetramethyl-1-piperidinyloxy (TEMPO) or butylated hydroxytoluene (BHT) was added, no desired product was detected under the standard conditions, suggesting that a free radical was involved in this transformation. The TEMPO adduct 5 and BHT adduct 6 were detected by HRMS analysis (ESI†).
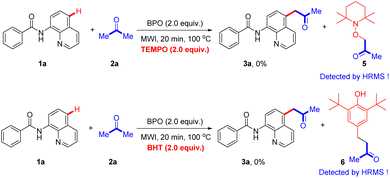 |
| Scheme 6 Radical trapped experiments. | |
Based on the control experiment, a plausible mechanism is proposed as in Scheme 7. First, the benzoate radical A is generated as a result of microwave irradiation from BPO, and it then captures a hydrogen atom from the substrate acetone 2a to form the carbon radical B, which is trapped by TEMPO or BHT to its adducts 5 or 6. The obtained acetone radical B reacts with substrate N-(quinolin-8-yl)benzamide 1a to afford an intermediate C, which further reacts with benzoate radical A to generate the final product 3a.
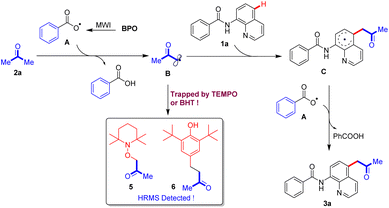 |
| Scheme 7 The proposed mechanism. | |
Conclusions
In summary, we have developed a microwave-accelerated cross-dehydrogenative-coupling (CDC) of N-(quinolin-8-yl)amides with acetone/acetonitrile under metal-free conditions. In the presence of benzoyl peroxide (BPO) as promoter, a cross-dehydrogenation coupling (CDC) of N-(quinolin-8-yl)amides with acetone underwent smoothly to generate the corresponding 5-acetonated/acetonitriled N-(quinolin-8-yl)amides in good yields. Compared with the traditional strategy for C-5 position coupling reactions, this reaction provides an environmentally friendly, efficient, and convenient access to C-5 position functionalization of N-(quinolin-8-yl)amides. Moreover, a plausible reaction mechanism is proposed based on a radical trapped experiment and HRMS studies. Further attempts to apply microwave acceleration coupling reactions are currently underway in our laboratory.
Conflicts of interest
There are no conflicts to declare.
Acknowledgements
We gratefully acknowledge the National Natural Science Foundation of China (21901081), University Natural Science Research Project of Anhui Province (KJ2021A0526, KJ2020A1199), Research Fund of Excellent Young Scientists in Higher Education Institution of Anhui Province, China (2022AH030060) and the Natural Science Foundation of Anhui Province (2008085QB90).
Notes and references
-
(a) J. Colomb, G. Becker, S. Fieux, L. Zimmer and T. Billard, J. Med. Chem., 2014, 57, 3884 CrossRef CAS PubMed;
(b) Y. C. Liu, J. H. Wei, Z. F. Chen, M. Liu, Y. Q. Gu, K. B. Huang, Z. Q. Li and H. Liang, Eur. J. Med. Chem., 2013, 69, 554 CrossRef CAS;
(c) S. Zhu, W. Lin and L. Yuan, Dyes Pigm., 2013, 99, 465 CrossRef CAS;
(d) Y. Lv, Z.-W. Hou, P. Li and L. Wang, Org. Chem. Front., 2023, 10, 990 RSC;
(e) V. R. Solomon and H. Lee, Curr. Med. Chem., 2011, 18, 1488 CrossRef CAS PubMed;
(f) T. Eicher, S. Hauptmann and A. Speicher, The Chemistry of Heterocycles: Structures, Reactions, Synthesis, and Applications 3rd, John Wiley & Sons, 2013 Search PubMed.
-
(a) G. Rouquet and N. Chatani, Angew. Chem., Int. Ed., 2013, 52, 11726 CrossRef CAS;
(b) M. Corbet and F. De Campo, Angew. Chem., Int. Ed., 2013, 52, 9896 CrossRef CAS;
(c) O. Daugulis, J. Roane and L. D. Tran, Acc. Chem. Res., 2015, 48, 1053 CrossRef CAS PubMed;
(d) X. Wang, R. Qiu, C. Yan, V. P. Reddy, L. Zhu, X. Xu and S. F. Yin, Org. Lett., 2015, 17, 1970 CrossRef CAS PubMed;
(e) X. Wang, L. Zhu, S. Chen, X. Xu, C. T. Au and R. Qiu, Org. Lett., 2015, 17, 5228 CrossRef CAS;
(f) J. Liu, G. Chen and Z. Tan, Adv. Synth. Catal., 2016, 358, 1174 CrossRef CAS;
(g) Z. Chen, B. Wang, J. Zhang, W. Yu, Z. Liu and Y. Zhang, Org. Chem. Front., 2015, 2, 1107 RSC.
-
(a) A. M. Berman, J. C. Lewis, R. G. Bergman and J. A. Ellman, J. Am. Chem. Soc., 2008, 130, 14926 CrossRef CAS PubMed;
(b) M. Tobisu, I. Hyodo and N. Chatani, J. Am. Chem. Soc., 2009, 131, 12070 CrossRef CAS PubMed;
(c) D. Zhao, W. Wang, F. Yang, J. Lan, L. Yang, G. Gao and J. You, Angew. Chem., Int. Ed., 2009, 48, 3296 CrossRef CAS.
- M. Wasa, B. Worrell and J.-Q. Yu, Angew. Chem., Int. Ed., 2010, 49, 1275 CrossRef CAS PubMed.
-
(a) C. C. Tsai, W. C. Shih, C. H. Fang, C. Y. Li, T. G. Ong and G. P. A. Yap, J. Am. Chem. Soc., 2010, 132, 11887 CrossRef CAS;
(b) Q. Chen, X. M. du Jourdin and P. Knochel, J. Am. Chem. Soc., 2013, 135, 4958 CrossRef CAS PubMed.
- J. Kwak, M. Kim and S. Chang, J. Am. Chem. Soc., 2011, 133, 3780 CrossRef CAS PubMed.
-
(a) D. Ji, X. He, Y. Xu, Z. Xu, Y. Bian, W. Liu, Q. Zhu and Y. Xu, Org. Lett., 2016, 18, 4478 CrossRef CAS;
(b) Y. Wang, Y. Wang, Z. Guo, Q. Zhang and D. Li, Asian J. Org. Chem., 2016, 5, 1438 CrossRef CAS;
(c) Y. Yin, J. Xie, F. Q. Huang, L. W. Qi and B. Zhang, Adv. Synth. Catal., 2016, 359, 1037 CrossRef.
-
(a) H. W. Liang, K. Jiang, W. Ding, Y. Yuan, L. Shuai, Y. C. Chen and Y. Wei, Chem. Commun., 2015, 51, 16928 RSC;
(b) H. Qiao, S. Sun, F. Yang, Y. Zhu, W. Zhu, Y. Dong, Y. Wu, X. Kong, L. Jiang and Y. Wu, Org. Lett., 2015, 17, 6086 CrossRef CAS PubMed;
(c) J. Xu, C. Shen, X. Zhu, P. Zhang, M. J. Ajitha, K. W. Huang, Z. An and X. Liu, Chem.–Asian J., 2016, 11, 882 CrossRef CAS PubMed;
(d) J. M. Li, J. Weng, G. Lu and A. S. C. Chan, Tetrahedron Lett., 2016, 57, 2121 CrossRef CAS;
(e) J. Wei, J. Jiang, X. Xiao, D. Lin, Y. Deng, Z. Ke, H. Jiang and W. Zeng, J. Org. Chem., 2016, 81, 946 CrossRef CAS;
(f) S. Liang and G. Manolikakes, Adv. Synth. Catal., 2016, 358, 2371 CrossRef CAS.
- C. Wen, R. Zhong, Z. Qin, M. Zhao and J. Li, Chem. Commun., 2020, 56, 9529 RSC.
- X. Cong and X. Zeng, Org. Lett., 2014, 16, 3716 CrossRef CAS PubMed.
-
(a) M. D. Reddy, F. R. Fronczek and E. B. Watkins, Org. Lett., 2016, 18, 5620 CrossRef CAS PubMed;
(b) M. Cui, J. H. Liu, X. Y. Lu, X. Lu, Z. Q. Zhang, B. Xiao and Y. Fu, Tetrahedron Lett., 2017, 58, 1912 CrossRef CAS.
-
(a) A. M. Suess, M. Z. Ertem, C. J. Cramer and S. S. Stahl, J. Am. Chem. Soc., 2013, 135, 9797 CrossRef CAS PubMed;
(b) H. Guo, M. Chen, P. Jiang, J. Chen, L. Pan, M. Wang, C. Xie and Y. Zhang, Tetrahedron, 2015, 71, 70 CrossRef CAS;
(c) B. Khan, R. Kant and D. Koley, Adv. Synth. Catal., 2016, 358, 2352 CrossRef CAS;
(d) Y. Wang, Y. Wang, K. Jiang, Q. Zhang and D. Li, Org. Biomol. Chem., 2016, 14, 10180 RSC;
(e) X. He, Y. Z. Xu, L. X. Kong, H. H. Wu, D. Z. Ji, Z. B. Wang, Y. G. Xu and Q. H. Zhu, Org. Chem. Front., 2017, 4, 1046 RSC;
(f) L. Zhao, P. Li, X. Xie and L. Wang, Org. Chem. Front., 2018, 5, 1689 RSC;
(g) Z. Wang, L. Wang, Z. Wang, P. Zhang and Y. Li, Chin. Chem. Lett., 2021, 32, 429 CrossRef CAS;
(h) C. Tian, L. M. Yang, H. T. Tian, G. H. An and G. M. Li, J. Fluorine Chem., 2019, 219, 23 CrossRef CAS.
-
(a) Z. Wu, Y. He, C. Ma, X. Zhou, X. Liu, Y. Li, T. Hu, P. Wen and G. Huang, Asian J. Org. Chem., 2016, 5, 724 CrossRef CAS;
(b) Y. Kuninobu, M. Nishi and M. Kanai, Org. Biomol. Chem., 2016, 14, 8092 RSC;
(c) L. K. Jin, G. P. Lu and C. Cai, Org. Chem. Front., 2016, 3, 1309 RSC;
(d) C. Shen, J. Xu, B. Ying and P. Zhang, ChemCatChem, 2016, 8, 3560 CrossRef CAS;
(e) N. Thrimurtulu, A. Dey, K. Pal, A. Nair, S. Kumar and C. M. R. Volla, ChemistrySelect, 2017, 2, 7251 CrossRef CAS;
(f) L. Ruyet, T. Poisson and T. Besset, Eur. J. Org. Chem., 2021, 2021, 3407 CrossRef CAS;
(g) H. Chen, P. Li, M. Wang and L. Wang, Org. Lett., 2016, 18, 4794 CrossRef CAS PubMed;
(h) L. Zhu, R. Qiu, X. Cao, S. Xiao, X. Xu, C. T. Au and S. F. Yin, Org. Lett., 2015, 17, 5528 CrossRef CAS PubMed;
(i) H. Sahoo, A. Mandal, J. Selvakumar and M. Baidya, Eur. J. Org. Chem., 2016, 2016, 4321 CrossRef CAS;
(j) Y. Dou, Z. Xie, Z. Sun, H. Fang, C. Shen, P. Zhang and Q. Zhu, ChemCatChem, 2016, 8, 3570 CrossRef CAS;
(k) C. J. Whiteoak, O. Planas, A. Company and X. Ribas, Adv. Synth. Catal., 2016, 358, 1679 CrossRef CAS;
(l) Y. He, N. Zhao, L. Qiu, X. Zhang and X. Fan, Org. Lett., 2016, 18, 6054 CrossRef CAS PubMed;
(m) C. Xia, K. Wang, J. Xu, C. Shen, D. Sun, H. Li, G. Wang and P. Zhang, Org. Biomol. Chem., 2017, 15, 531 RSC.
-
(a) C. Y. Huang, J. Li and C. J. Li, Nat. Commun., 2021, 12, 4010 CrossRef CAS PubMed;
(b) C. Y. Huang, J. Li, W. Liu and C.-J. Li, Chem. Sci., 2019, 10, 5018 RSC;
(c) T. Wu, X. Mu and G. Liu, Angew. Chem., 2011, 123, 12786 CrossRef;
(d) X. Q. Chu, H. Meng, Y. Zi, X. P. Xu and S. J. Ji, Org. Chem. Front., 2015, 2, 216 RSC;
(e) X. Q. Chu, Z. H. Xing, H. Meng, X. P. Xu and S. J. Ji, Org. Chem. Front., 2016, 3, 165 RSC;
(f) H. Su, L. Wang, H. Rao and H. Xu, Org. Lett., 2017, 19, 2226 CrossRef CAS PubMed;
(g) Y. R. Gu, X. H. Duan, L. Chen, Z. Y. Ma, P. Gao and L. N. Guo, Org. Lett., 2019, 21, 917 CrossRef CAS PubMed;
(h) Q. Wan, Z.-W. Hou, X.-R. Zhao, X. Xie and L. Wang, Org. Lett., 2023, 25, 1008 CrossRef CAS PubMed;
(i) S. Jin, H. Yao, S. Lin, X. You, Y. Yang and Z. Yan, Org. Biomol. Chem., 2020, 18, 205 RSC;
(j) X. Rong, L. Jin, Y. Gu, G. Liang and Q. Xia, Asian J. Org. Chem., 2020, 9, 185 CrossRef CAS;
(k) H. Y. Song, J. Jiang, C. Wu, J. C. Hou, Y. H. Lu, K. L. Wang, T. B. Yang and W. M. He, Green Chem., 2023, 25, 3292 RSC;
(l) Q. Q. Han, D. M. Chen, Z. L. Wang, Y. Y. Sun, S. H. Yang, J. C. Song and D. Q. Dong, Chin. Chem. Lett., 2021, 32, 2559 CrossRef CAS;
(m) J. Jiang, K. L. Wang, X. Li, C. Wu, H. T. Ji, X. Chen and W. M. He, Chin. Chem. Lett., 2023, 34, 108699 CrossRef.
-
(a) C.-J. Li, Acc. Chem. Res., 2008, 42, 335 CrossRef PubMed;
(b) S. A. Girard, T. Knauber and C.-J. Li, Angew. Chem., Int. Ed., 2013, 53, 74 CrossRef PubMed;
(c) C. Liu, J. Yuan, M. Gao, S. Tang, W. Li, R. Shi and A. Lei, Chem. Rev., 2015, 115, 12138 CrossRef CAS PubMed.
-
(a) G. Deng, L. Zhao and C.-J. Li, Angew. Chem., Int. Ed., 2008, 47, 6278 CrossRef CAS PubMed;
(b) X. Guo and C.-J. Li, Org. Lett., 2011, 13, 4977 CrossRef CAS;
(c) G. Deng and C. J. Li, Org. Lett., 2009, 11, 1171 CrossRef CAS.
-
(a) C. Y. Huang, J. Li and C.-J. Li, Nat. Commun., 2021, 12, 4010 CrossRef CAS;
(b) C. Y. Huang, J. Li, W. Liu and C.-J. Li, Chem. Sci., 2019, 10, 5018 RSC.
- F. Doraghi, E. Kianmehr and A. Foroumadi, Org. Chem. Front., 2021, 8, 5424 RSC.
- D. Liu, Z. Xia, Y. Xiao, Y. Yu, L. Yu, Z. Song, Q. Wu, J. Zhang and Z. Tan, Eur. J. Org. Chem., 2021, 5012 CrossRef CAS.
-
(a) S. Preciado, L. Mendive-Tapia, F. Albericio and R. Lavilla, J. Org. Chem., 2013, 78, 8129 CrossRef CAS;
(b) H. R. Schmidt, R. M. Betz, R. O. Dror and A. C. Kruse, Nat. Struct. Mol. Biol., 2018, 25, 981 CrossRef CAS PubMed;
(c) J. Huang, Y. Huang, T. Wang, Q. Huang, Z. Wang and Z. Chen, Org. Lett., 2017, 19, 1128 CrossRef CAS PubMed;
(d) J. Hou, X. Geng, W. Li, P. Zhang and L. Wang, Org. Lett., 2023, 25, 512 CrossRef PubMed;
(e) S. Ruan, C. Zhou, L. Li, L. Wang, J. Liu and P. Li, Org. Biomol. Chem., 2022, 20, 3817 RSC;
(f) P. Dhanush, P. V. Saranya and G. Anilkumar, Tetrahedron, 2022, 105, 132614 CrossRef CAS.
|
This journal is © The Royal Society of Chemistry 2023 |