DOI:
10.1039/D3RA03215A
(Paper)
RSC Adv., 2023,
13, 20164-20178
Multicolor tunable bright photoluminescence in Ca2+/Mg2+ modified Eu3+ doped ZnGa2O4 phosphors under UV excitation for solid state lighting applications
Received
15th May 2023
, Accepted 26th June 2023
First published on 5th July 2023
Abstract
The Eu3+ doped and Mg2+/Ca2+ co-doped ZnGa2O4 phosphor samples were synthesized by solid-state reaction method and their structural and optical properties studied. The phase, crystallinity and particles size of the phosphor samples were studied by XRD and SEM measurements. EDS analyses were used to identify the elements present in the phosphor materials. The vibrational groups present in the phosphor samples were examined by Fourier transform infrared (FTIR) measurements. Pure ZnGa2O4 emits intense blue light under 260 nm excitation. However, Eu3+ doped and Mg2+/Ca2+ co-doped ZnGa2O4 phosphor samples exhibit intense red emission under 393 nm excitation. A bluish white color is observed in these samples under 290 nm excitation. The maximum PL emission intensity is found at 0.1 mol% Eu3+ doping concentration. For higher concentrations, concentration quenching was observed due to dipole–dipole interaction. The emission intensity is enhanced upto 1.20 and 2.91 times on co-doping of Mg2+ and Ca2+ via induced crystal field due to charge imbalance. The emission intensity of the phosphor is found to enhance further on annealing the samples at 873 K. Under various excitation wavelengths, color tunability was seen from blue to bluish-white to red regions. The lifetime of the 5D0 level of the Eu3+ ion improves via doping of Mg2+/Ca2+ ions and it increases appreciably on annealing. The temperature dependent photoluminescence study (TDPL) reveals a thermal quenching behavior of the sample with thermal stability ∼65% and activation energy ∼0.223 eV in the Eu3+/Ca2+ co-doped ZnGa2O4 phosphor sample.
1. Introduction
The rare earth doped inorganic phosphor materials are highly chemically, physically and thermally stable and yield large photoluminescence intensity on excitation with suitable wavelength. These materials have wide applications in different emerging fields, such as display devices, light emitting diodes (LEDs), color tunable devices, temperature sensing, development of new lasers, plant cultivation etc.1–7 This is possible due to the presence of a large number of meta-stable energy levels in the rare earth ions.8–12 The rare earth ions, such as Eu3+, Tb3+, Tm3+, Dy3+, etc., emit red, green, blue and yellow colors respectively, in different host matrices.2,5–7 Thus, a combination of these rare earth ions, such as Dy3+/Eu3+, Sm3+/Eu3+, Tb3+/Eu3+, etc. produces color tunable photoluminescence (PL) in different host matrices depending on their concentrations and excitation wavelengths.13–16 The concentration of these ions plays a very important role in achieving color tunable emissions. This occurs due to a variation in the intensity of the emitted colors from these ions as well as energy transfer between them. Out of these rare earth ions, the Eu3+ ion is very promising as it gives almost pure red emission.17–20 The Eu3+ doped phosphor materials are often used to fulfill the requirement of red components in white LEDs. However, the PL intensity of Eu3+ doped phosphor materials needs to be improved.
Efforts have been made by several groups and it is still required to enhance the PL emission intensity of Eu3+ ion in different hosts by co-doping the alkalis, alkaline earths, transition metals and rare earth ions.21–26 In these cases, the PL intensity of Eu3+ ion is enhanced not only due to increase in crystallinity of the materials but also due to crystal field effect of the other doped ions and energy transfer to Eu3+ ion by other ions.13–16,21–26 Yang et al. have prepared the novel red-emitting Sr7Sb2O12:Eu3+, M+ (M = Li, Na, K) phosphors and studied the effect of alkali ions on the PL intensity of Eu3+.21 Our group has also studied the impact of alkali doping on the PL intensity of Eu3+ ions in CaTiO3 phosphor.22 Singh et al. have reported luminescent characteristics of M3Y2Si3O12:Eu3+ (M = Ca, Mg, Sr and Ba) and found significant enhancement in the PL emission intensity in presence of these ions.23 Shi et al. have reported an enhancement in the PL intensity of Eu3+ ion in Y2O3:Eu3+ phosphors in presence of alkali and alkaline earth metal ions.27 The enhancement in PL intensity has been also observed due to charge compensation (crystal field effect), increase in crystallinity and asymmetric nature of the crystal field. Yang et al. have also observed that increasing the concentration of Bi3+ ions led to an improvement in the PL intensity of Eu3+ in ZnGa2O4 phosphor, which is caused by energy transfer from Bi3+ to Eu3+ ions.28 Rai et al. have observed enhancement in PL intensity of Eu3+ ion through energy transfer from Tb3+ to Eu3+ ions in LaVO4 phosphor.16 However, the effect of co-doping of Mg2+ and Ca2+ ions on the PL intensity in ZnGa2O4:Eu3+ phosphor has not been investigated to our knowledge. Our group has found that the PL intensity of LaVO4:Eu3+ phosphor was enhanced 4.5 times via co-doping of Ca2+ ion.24 In the present work, the PL intensity of Eu3+ doped ZnGa2O4 phosphor has been investigated in absence and presence of Mg2+/Ca2+ ions.
The thermal stability of phosphor material is one of the desirable conditions for practical applications as it is an important parameter for a photoluminescent phosphor. The variation of PL emission intensity with temperature is a function of thermal stability of the phosphor materials.29 The thermal stability of phosphor samples are compared in terms of photoluminescence emission at 423 K (150 °C) for LEDs applications as the phosphor materials deteriorate at higher temperatures and reduce its emission efficiency.30 The temperature dependent PL intensity has been studied by Rajendran et al. in Ba2YV3O11:Eu3+ phosphor and found the thermal stability of phosphor is 59.5% at 423 K.31 In the case of Ba2LaV3O11:Eu3+, this value was reported to be 62% at 423 K.32 The temperature-dependent PL in the Bi4Si3O12:Eu3+ phosphor was also studied by Zhang et al.33 They have found that the PL emission intensity is decreased to 50% at 398 K compared to its PL intensity at 298 K. It would be interesting to measure the thermal stability of Eu3+ doped and Eu3+/Ca2+ co-doped ZnGa2O4 phosphor material.
In this work, the Eu3+ doped and Mg2+/Ca2+ co-doped ZnGa2O4 phosphor materials have been synthesized through solid state reaction method at 1473 K. A small part of the prepared samples has been annealed at 873 K temperature to see the changes in structural and photoluminescence properties of the doped and co-doped samples. The X-ray diffraction (XRD), scanning electron microscopic (SEM) and energy dispersive X-ray spectroscopic (EDS) measurements have been carried out for the structural, morphological and elemental properties. The vibrational structures of the phosphor samples have been studied by Fourier transform infrared (FTIR) measurements. The Eu3+ doped ZnGa2O4 phosphor sample emits bright red color along with blue color on excitation with charge transfer band (CTB) of host at 260 nm and the charge transfer band (CTB) of Eu3+ at 290 nm. However, on excitation with n-UV wavelength at 393 nm (atomic line of Eu3+), only red emission is seen due to Eu3+ ion. The PL intensity of Eu3+ doped phosphor is enhanced on co-doping of Mg2+/Ca2+ ions. On annealing the samples at 873 K, the PL intensity of phosphor samples was further improved. The CIE coordinates of the phosphor samples were calculated for undoped and doped samples. The lifetime of 5D0 level of Eu3+ ion has been measured using 5D0 → 7F2 transition at 613 nm wavelength under the excitation with 393 nm. The thermal stability of the Eu3+ doped and Eu3+/Ca2+ co-doped ZnGa2O4 phosphor samples were demonstrated by the temperature dependent photoluminescence (TDPL) studies. These values in the two cases were found to be 58.43% and 64.88% with activation energies 0.198 eV and 0.223 eV, respectively at 423 K.
2. Experimental methods
2.1 Synthesis
The phosphor samples have been synthesized by a solid-state reaction method at 1473 K temperature. We have used base materials as ZnO (Otto, 99.99%), Ga2O3 (Alfa Aesar 99.9%), Eu2O3 (Molychem, 99.99%), MgO (Himedia, 99.9%) and CaCO3 (SDFCL, 99%). These materials were weighed carefully followed by mixing in agate mortar and acetone as mixing medium. The homogenously mixed samples were put in alumina crucible and heated in an electric furnace at 1473 K temperature for 4 hours. These samples were crushed into fine powder. A small part of these samples were further annealed at 873 K temperature for 4 hours separately to see the effect of further heating. Following compositions were used for the sample preparation: |
47ZnO + 53Ga2O3 → 47(ZnGa2O4) + 6Ga2O3
| (i) |
|
47ZnO + (53 − x)Ga2O3 + xEu2O3 → 47(ZnGa2−xO4+δ):xEu + 6Ga2O3
| (ii) |
where x was taken (0.05, 0.1, 0.2, 0.5 and 1.0 mol%) the concentration of Eu3+, respectively. In Mg2+/Ca2+ co-doped ZnGa2O4:Eu3+ phosphor samples, the compositions used were as follows: |
47ZnO + (53 − x − y)Ga2O3 + xEu2O3 + yMgO → 47(ZnGa2−x−yO4+δ):xEu:yMg + 6Ga2O3
| (iii) |
|
47ZnO + (53 − x − z)Ga2O3 + xEu2O3 + zCaCO3 → 47(ZnGa2−x−zO4+δ):xEu:zCa + 6Ga2O3 + CO2
| (iv) |
where x was fixed at 0.1 mol% concentration and the y and z were varied as 1, 2, 3 and 5 mol% concentrations to get the optimum PL intensity. The term ‘δ’ represents the excess of oxygen released during the synthesis. These phosphor samples were used for further analyses.
2.2 Instrumentation
The crystalline nature and phase purity of the phosphor samples were analyzed by monitoring the XRD patterns using Rigaku diffractometer (MiniFlex 600-unit and Cu Kα radiation with λ = 0.15406 nm). The scanning electron microscope (SEM) (Zeiss, Evo 18 Research unit) was used to study the morphological structure of the phosphor samples. The elements present in the phosphors were verified by the energy dispersive X-ray spectroscopic (EDS) studies. The Fourier transform infrared (FTIR) spectra were monitored to know the vibrational groups present in the phosphors using a PerkinElmer IR spectrometer (I-Frontier unit). The downshifting photoluminescence spectra of all the samples were recorded using Fluorolog-3 spectrophotometer (Horiba Jobin Yvon) attached with a 450 W Xenon lamp as a source of light (Horiba Jobin Yvon). We have also measured the lifetime of 5D0 level of Eu3+ ion using the same unit attached with a 25 W pulsed Xenon lamp.
3. Results and discussion
3.1 Structural studies
3.1.1 X-ray diffraction (XRD) measurements. The XRD patterns of ZnGa2O4:0.1Eu3+, ZnGa2O4:0.1Eu3+/3Mg2+ and ZnGa2O4:0.1Eu3+/3Ca2+ phosphor samples unannealed and annealed (at 873 K) are shown in Fig. 1(a–f). The phase of the spinel crystal is cubic. The diffraction peaks match well with standard JCPDS File No. 38-1240.2,4–7,35,36 Some weak impurity peaks were also observed in the XRD patterns due to monoclinic phase of β-Ga2O3. These impurity peaks are marked with asterisk ‘*’ in Fig. 1. The sharpness of the diffraction peaks indicates highly crystalline nature of the prepared phosphor. When the Mg2+/Ca2+ ions are co-doped in the ZnGa2O4:0.1Eu3+ phosphor at Ga3+ site, the XRD peaks are shifted towards lower 2θ angle side. The peaks are shifted as the ionic radii of Ca2+ (100 pm) and Mg2+ (72 pm) are higher as compared to Ga3+ (62 pm) ion.23 The shift in XRD peak position can be verified from the zoomed patterns shown in Fig. 1(a–f).
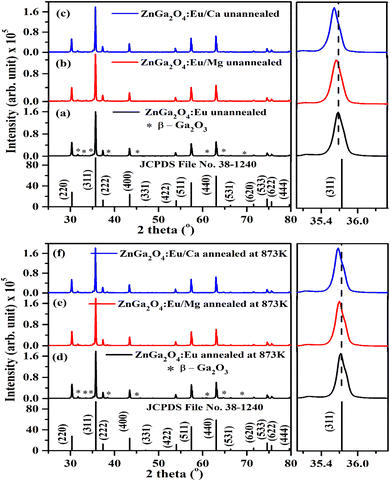 |
| Fig. 1 XRD patterns of (a) ZnGa2O4:0.1Eu3+ (b) ZnGa2O4:0.1Eu3+/3Mg2+ and (c) ZnGa2O4:0.1Eu3+/3Ca2+ phosphor samples and that of (d) ZnGa2O4:0.1Eu3+ (e) ZnGa2O4:0.1Eu3+/3Mg2+ and (f) ZnGa2O4:0.1Eu3+/3Ca2+ phosphor samples annealed at 873 K along with JCPDS File No. and their zoomed patterns of (311) lattice plane. | |
The phosphor samples annealed at 873 K temperature show an improvement in crystallinity of the materials. The average crystallite size (D) were calculated using Debye Scherrer's formula.34–37
|
 | (v) |
where
k is a constant (=0.89),
β is the full width at half maxima (FWHM) at a diffraction angle (
θ) and
λ is the wavelength of X-ray radiation. The obtained values of crystallite size are found to be 38.09, 39.06 and 41.27 nm for the ZnGa
2O
4:0.1Eu
3+, ZnGa
2O
4:0.1Eu
3+/3Mg
2+ and ZnGa
2O
4:0.1Eu
3+/3Ca
2+ phosphor samples, respectively. This shows that the crystallite size increases on co-doping of Ca
2+/Mg
2+ ions. The crystallite size is further improved on annealing the samples at 873 K and the values were found to be 41.29, 42.15 and 43.19 nm, respectively. The increase in crystallite size would be supportive for enhancing the PL intensity of the phosphor samples.
We have also analyzed the dislocation density for the ZnGa2O4:0.1Eu3+, ZnGa2O4:0.1Eu3+/3Mg2+ and ZnGa2O4:0.1Eu3+/3Ca2+ phosphor samples and the samples annealed at 873 K by using the following relation:7,35,36
|
 | (vi) |
The computed dislocation densities for the ZnGa2O4:0.1Eu3+, ZnGa2O4:0.1Eu3+/3Mg2+ and ZnGa2O4:0.1Eu3+/3Ca2+ phosphor samples are 6.8 × 10−4, 6.5 × 10−4 and 5.8 × 10−4 nm−2, respectively. In the case of annealed samples these values are 5.8 × 10−4, 5.6 × 10−4 and 5.3 × 10−4 nm−2, respectively. This shows that the dislocation density decreases in presence of Mg2+/Ca2+ ions thereby improve the local crystal structure, which is responsible for the enhancement of PL intensity of the phosphor materials.
The Rietveld refinements of XRD patterns for the ZnGa2O4:0.1Eu3+, ZnGa2O4:0.1Eu3+/3Mg2+ and ZnGa2O4:0.1Eu3+/3Ca2+ phosphor samples have been carried out using the FullProf program and they are shown in Fig. 2(a–c). The Fig. 2(a–c) shows that the observed and calculated XRD patterns match well with each other. The lower profile represents the difference between the observed and the calculated XRD patterns, whereas the vertical bars are Bragg's positions of ZnGa2O4 (cubic) and β-Ga2O3 (monoclinic) phases. The different crystallographic parameters, such as phase, space group, lattice parameters and unit cell volumes for the ZnGa2O4:0.1Eu3+, ZnGa2O4:0.1Eu3+/3Mg2+ and ZnGa2O4:0.1Eu3+/3Ca2+ phosphor samples are summarized in Table 1.
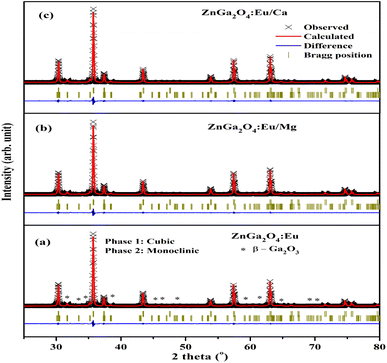 |
| Fig. 2 Rietveld fits of XRD patterns for (a) ZnGa2O4:0.1Eu3+ (b) ZnGa2O4:0.1Eu3+/3Mg2+ and (c) ZnGa2O4:0.1Eu3+/3Ca2+ phosphor. Asterisks ‘*’ represents the impurity peaks due to β-Ga2O3. | |
Table 1 Phase, space group, lattice parameters and unit cell volume for the ZnGa2O4:0.1Eu3+, ZnGa2O4:0.1Eu3+/3Mg2+ and ZnGa2O4:0.1Eu3+/3Ca2+ phosphor
Sample |
Phase (1) |
Space group |
Lattice parameters (ZnGa2O4) |
Volume (Å3) |
Phase (2) |
Space group |
Lattice parameters (β-Ga2O3) |
Volume (Å3) |
ZnGa2O4:0.1Eu3+ |
Cubic |
Fd m |
a = 8.3311 Å |
578.24 |
Monoclinic |
C2/m |
a = 12.216 Å |
209.38 |
|
|
|
b = 8.3311 Å |
|
|
|
b = 3.0395 Å |
|
|
|
|
c = 8.3311 Å |
|
|
|
c = 5.8084 Å |
|
|
|
|
α = β = γ = 90° |
|
|
|
α = 90° |
|
|
|
|
|
|
|
|
β = 103.876° |
|
|
|
|
|
|
|
|
γ = 90° |
|
ZnGa2O4:0.1Eu3+/3Mg2+ |
Cubic |
Fd m |
a = 8.3328 Å |
578.60 |
Monoclinic |
C2/m |
a = 12.222 Å |
209.51 |
|
|
|
b = 8.3328 Å |
|
|
|
b = 3.0389 Å |
|
|
|
|
c = 8.3328 Å |
|
|
|
c = 5.8101 Å |
|
|
|
|
α = β = γ = 90° |
|
|
|
α = 90° |
|
|
|
|
|
|
|
|
β = 103.865° |
|
|
|
|
|
|
|
|
γ = 90° |
|
ZnGa2O4:0.1Eu3+/3Ca2+ |
Cubic |
Fd m |
a = 8.3339 Å |
578.81 |
Monoclinic |
C2/m |
a = 12.222 Å |
209.63 |
|
|
|
b = 8.3339 Å |
|
|
|
b = 3.0398 Å |
|
|
|
|
c = 8.3339 Å |
|
|
|
c = 5.8117 Å |
|
|
|
|
α = β = γ = 90° |
|
|
|
α = 90° |
|
|
|
|
|
|
|
|
β = 103.861° |
|
|
|
|
|
|
|
|
γ = 90° |
|
3.1.2 SEM and EDS measurements. The SEM images of ZnGa2O4:0.1Eu3+, ZnGa2O4:0.1Eu3+/3Mg2+ and ZnGa2O4:0.1Eu3+/3Ca2+ phosphor samples are shown in Fig. 3(a–c). It is clear from the figure that the particles shape is nearly spherical and agglomerated to each other. The particles size distribution has been evaluated by plotting histogram for the different phosphor samples using ImageJ software and they are shown in Fig. 3(d–f). The average particles size is found to be 1.11 μm for ZnGa2O4:0.1Eu3+ phosphor, which is slightly increased on doping of Mg2+ (1.36 μm) and Ca2+ (1.54 μm) ions in the ZnGa2O4:Eu3+ phosphor, respectively.38 This clearly shows that the average particles size of the phosphor materials are increased in presence of Mg2+/Ca2+ ions. Maurya et al. have also observed an increase in particles size of the Ho3+/Yb3+ co-doped CaZrO3 phosphor after co-doping of Mg2+ ions and reported an enhancement in the emission intensity.38 An increase in the particles size of phosphor has been also reported by Rai et al. in the LaVO4:Eu3+ phosphor on incorporation of Ca2+ ion.24 They have also observed an increase in the PL intensity of phosphor via Ca2+ doping.
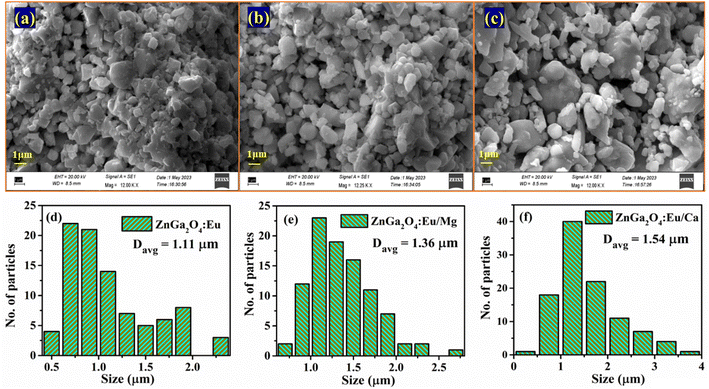 |
| Fig. 3 SEM images of (a) ZnGa2O4:0.1Eu3+ (b) ZnGa2O4:0.1Eu3+/3Mg2+ and (c) ZnGa2O4:0.1Eu3+/3Ca2+ and particles size distribution in (d) ZnGa2O4:0.1Eu3+ (e) ZnGa2O4:0.1Eu3+/3Mg2+ and (f) ZnGa2O4:0.1Eu3+/3Ca2+ phosphors. | |
Fig. 4(a–c) shows the energy dispersive X-ray spectroscopic (EDS) spectra of ZnGa2O4:0.1Eu3+, ZnGa2O4:0.1Eu3+/3Mg2+ and ZnGa2O4:0.1Eu3+/3Ca2+ phosphor samples, respectively. Fig. 4(a) clearly shows the presence of Zn, Ga, Eu and O elements in the phosphor samples. However, the incorporation of Mg and Ca elements along with these elements can be also verified by Fig. 4(b and c).
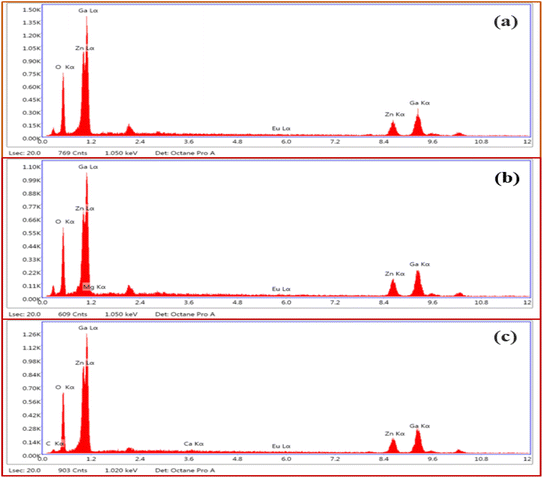 |
| Fig. 4 EDS spectra of (a) ZnGa2O4:0.1Eu3+ (b) ZnGa2O4:0.1Eu3+/3Mg2+ and (c) ZnGa2O4:0.1Eu3+/3Ca2+ phosphors. | |
3.2 Optical measurements
3.2.1 Fourier transform infrared (FTIR) measurements. FTIR spectra of ZnGa2O4, ZnGa2O4:0.1Eu3+, ZnGa2O4:0.1Eu3+/3Mg2+ and ZnGa2O4:0.1Eu3+/3Ca2+ phosphor samples were recorded in the 400–2000 cm−1 range and they are shown in Fig. 5. The spectra show two vibrational bands at 419 and 569 cm−1 and they are assigned to arise due to stretching vibrations of the Zn–O (419 cm−1) and Ga–O (569 cm−1) groups, respectively.7,35,36 No impurity peaks are seen in the phosphor samples. It has been observed that there is no change in vibrational band's positions however, the absorption intensity of the Zn–O and Ga–O bands are found to increase due to doping of Eu3+, Ca2+ and Mg2+ ions.
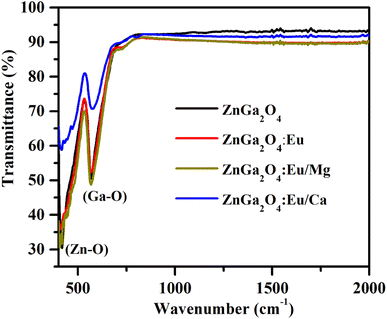 |
| Fig. 5 FTIR spectra of ZnGa2O4, ZnGa2O4:0.1Eu3+, ZnGa2O4:0.1Eu3+/3Mg2+ and ZnGa2O4:0.1Eu3+/3Ca2+ phosphor samples. | |
3.2.2 PL excitation (PLE) and PL emission measurements. The photoluminescence excitation (PLE) spectrum of ZnGa2O4 corresponding to λem = 434 nm and the photoluminescence (PL) emission spectrum under 260 nm excitation are shown in Fig. 6. The 260 nm band is due to charge transfer band of O2− → Ga3+. Upon 260 nm excitation, the emission spectrum of the ZnGa2O4 sample emits a broad intense band ranging from 350 to 550 nm with the peak position maxima at 434 nm, which can be attributed to the self-activation center of the octahedral Ga–O group in the spinel lattice.1,4 The emission of this self-activated phosphor sample matches well with the absorption spectrum of plant pigments, which shows usefulness of this material for plant growth applications.39
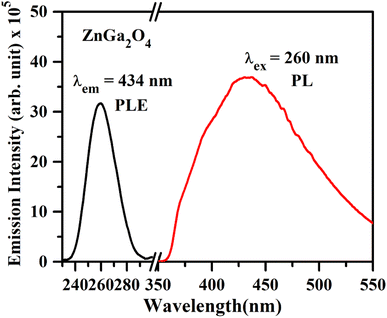 |
| Fig. 6 PL excitation spectrum of ZnGa2O4 under λem = 434 nm and PL emission spectrum of ZnGa2O4 phosphor under λex = 260 nm. | |
The PL excitation spectrum of ZnGa2O4:Eu3+ phosphor monitored in the 250–500 nm region with λem = 613 nm is shown in Fig. 7(a). The spectrum consists of a broad band ranging from 250–350 nm along with large number of sharp peaks due to intra-configurational forbidden 4f–4f transitions of Eu3+ ion.40–43 The broad band maxima observed at 290 nm is due to charge transfer band (CTB) of Eu3+ ion (O2− → Eu3+). The narrow peaks observed at 362, 375, 382, 393, 413 and 463 nm are ascribed to arise due to 7F0 → 5D4, 7F0 → 5L8, 7F0 → 5L7, 7F0 → 5L6, 7F0 → 5D3 and 7F0 → 5D2 transitions of Eu3+ ion, respectively.20–26,40–43 Among these peaks, the excitation peaks at 393 and 463 nm appear with relatively large intensity.
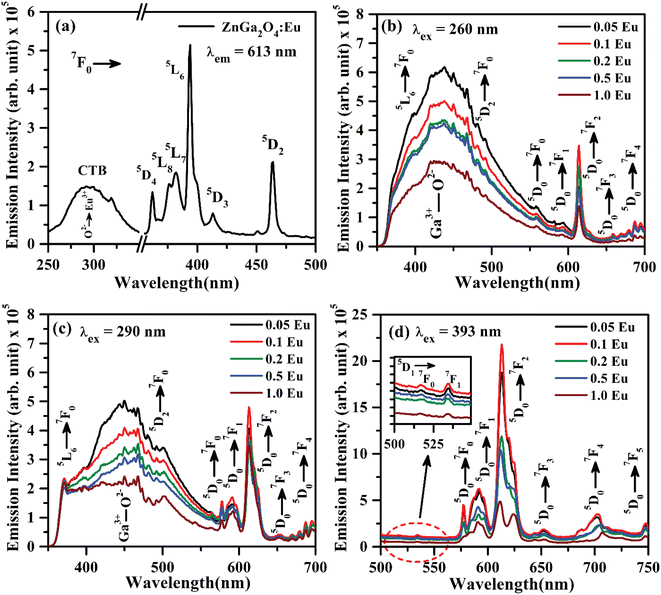 |
| Fig. 7 (a) PL excitation spectrum of ZnGa2O4:0.1Eu3+ phosphor with λem = 613 nm, PL emission spectra of ZnGa2O4:xEu3+ phosphors (where x = 0.05, 0.1, 0.2, 0.5 and 1.0 mol%) under (b) λex = 260 nm, (c) λex = 290 nm and (d) λex = 393 nm excitation. The inset in (d) is the zoomed PL emission spectra in the 500–549 nm range. | |
Fig. 7(b) shows the PL emission spectra of ZnGa2O4:xEu3+ phosphors (where x = 0.05, 0.1, 0.2, 0.5 and 1.0 mol%) recorded in 350–700 nm region under the excitation with 260 nm. The spectra show the broad band ranging from 350 to 550 nm due to self-activated emission of the ZnGa2O4 host with maxima at 434 nm superimposed with Eu3+ emission bands in which the bands in higher wavelength side from 550 to 700 nm are very intense. Similar results are also obtained under the excitation with CTB of Eu3+ at 290 nm, which is shown in Fig. 7(c). It is clear from the figure that the emission intensity of Eu3+ bands is better on excitation with charge transfer band (CTB) at 290 nm as compared to ZnGa2O4 excitation band at 260 nm. It is interesting to note that the emission peaks due to Eu3+ at 393 nm (5L6 → 7F0) and 463 nm (5D2 → 7F0) transitions are also superposed on the broad emission on excitation with 260 and 290 nm wavelengths.
The intense emission peaks positioned at 577, 592, 613, 652 and 696 nm are attributed to the 5D0 → 7F0, 5D0 → 7F1, 5D0 → 7F2, 5D0 → 7F3 and 5D0 → 7F4 transitions of Eu3+ ion, respectively, which are clearly shown in Fig. 7(b and c).20–26,40–43 Fig. 7(d) shows the PL emission spectra in the range of 500–750 nm under the excitation at 393 nm. The inset in Fig. 7(d) shows the zoomed emission spectra of Eu3+ in the range 500–549 nm. The emission peaks could be marked clearly at 519 and 534 nm due to 5D1 → 7F0 and 5D1 → 7F1 transitions of Eu3+ ion, respectively. The PL emission intensity of Eu3+ bands is maximum on excitation with 393 nm as compared to 290 and 260 nm. The band at 613 nm due to 5D0 → 7F2 transition exhibits the highest PL emission intensity for all excitation wavelengths. The emission intensity is optimum for 0.1 mol% concentration of Eu3+ ion.44,45
As is seen from Fig. 7, the intensity I5D0 → 7F2 ≫ I5D0 → 7F1. This clearly shows that the substitution of Eu3+ is at asymmetric site in the host lattice. Moreover, it is well known that the 5D0 → 7F2 transition of Eu3+ ion is due to electric dipole which obeys the selection rule ΔJ = ±2. Because of the absence of center of symmetry in this host matrix, such transitions are hypersensitive and affected by the local crystal field symmetry around the Eu3+ ion. On the other hand, the magnetic dipole transition (5D0 → 7F1) follows the selection rule ΔJ = ±1, and not affected by the local crystal field.21–27 The photoluminescence emission intensity of ZnGa2O4:xEu3+ has been monitored for different concentration of Eu3+ (where x = 0.05, 0.1, 0.2, 0.5 and 1.0 mol%). It is found that the emission intensity increases from 0.05 to 0.1 mol% and then decreases for higher concentrations due to concentration quenching. The variation of Eu3+ ion concentration versus the emission intensity for 613 nm band under the excitation at 393 nm is shown in Fig. 8(a). The concentration quenching has been observed above 0.1 mol% concentration of Eu3+ ion. On increasing the concentration of Eu3+ ion, the distance between two Eu3+ ions decreases, which increases the mutual interaction between the Eu3+ ions due to which the emission intensity of Eu3+ band is quenched.
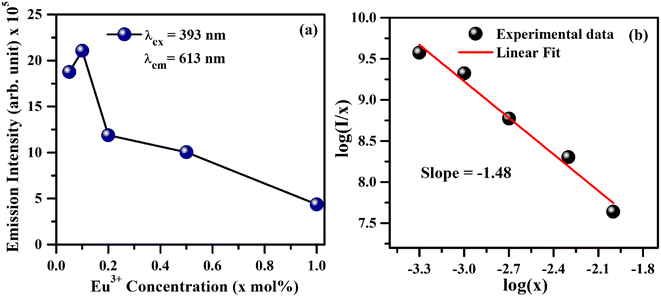 |
| Fig. 8 (a) Variation of PL emission intensity versus concentration of Eu3+ ion in the ZnGa2O4:xEu3+ phosphor samples (where x = 0.05, 0.1, 0.2, 0.5 and 1.0 mol%) and (b) a plot between log(I/x) and log(x). | |
The value of average critical distance between the two Eu3+ ions has been calculated using the relation:16,21,23,41,46,47
|
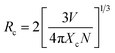 | (vii) |
where
V is the volume of unit cell,
Xc is the critical concentration of Eu
3+ ion and
N is the number of Eu
3+ ions occupying per unit cell in the host lattice. For the cubic spinel crystal structure,
V = 578.81 Å
3,
N = 8 and
Xc = 0.1 respectively.
46 The calculated value of
Rc using these parameters is found to be 11.13 Å. It is known that when the
Rc value is less than 5 Å, the nature of interaction between two Eu
3+ ions is exchange interaction. However, in the present case this distance is greater than 5 Å, therefore a multipolar interaction is the main cause of concentration quenching. The exact nature of this multipolar interaction for quenching the PL intensity can be confirmed by the following relation:
16,21,23,41,46,47 |
 | (viii) |
where
I/
x is the PL emission intensity per activator concentration.
k and
β terms are constants for a given phosphor. The value of
θ decides the actual nature of interaction between the activator ions. If this value is near to 6, the interaction is dipole–dipole. However, if this value is ∼8 or 10, the nature of interaction will be dipole–quadrupole or quadrupole–quadrupole, respectively. The simplified form of the
eqn (viii) can be written as:
21,41,46,47 |
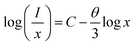 | (xi) |
where
C is a constant. The term −
θ/3 is the slope of the curve in between

and log
x.
Fig. 8(b) shows this plot for different concentrations of Eu
3+ ions for 613 nm under 393 nm excitation in the present case. A linear fit of this gives the slope value −1.48 from which the value of
θ is found to be 4.44, which is close to 6. This indicates that the dipole–dipole interaction is responsible for quenching of the PL intensity of Eu
3+ bands in ZnGa
2O
4 phosphor.
3.2.3 Effect of Mg2+ and Ca2+ doping on the PL intensity of ZnGa2O4:Eu3+. The PL emission spectra of ZnGa2O4:0.1Eu3+/yMg2+ and ZnGa2O4:0.1Eu3+/zCa2+ phosphors (where y/z = 1, 2, 3 and 5 mol%) monitored on excitation with 393 nm are shown in Fig. 9(a and b). From the Fig. 9(a and b), it is clear that the ZnGa2O4:0.1Eu3+/yMg2+ and ZnGa2O4:0.1Eu3+/zCa2+ phosphors emit similar emission bands as has been observed in the ZnGa2O4:0.1Eu3+ phosphor. The PL intensity of phosphor samples initially increases up to 3 mol% concentrations of Mg2+ and Ca2+ ions and then decrease for higher concentrations of these ions. The PL intensity is found to be maximum for 3 mol% concentrations of Mg2+/Ca2+ ions in the ZnGa2O4:0.1Eu3+ phosphor. The PL intensity of ZnGa2O4:0.1Eu3+ phosphor is increased upto 1.20 and 2.91 times on doping of 3 mol% Mg2+ and Ca2+ ions, respectively. Thus, the Ca2+ doping yields relatively larger PL emission intensity compared to Mg2+ in the ZnGa2O4:0.1Eu3+ phosphor. The enhancement in PL intensity of phosphor materials has been reported in several hosts via incorporation of Mg2+/Ca2+ ions.24,38,48
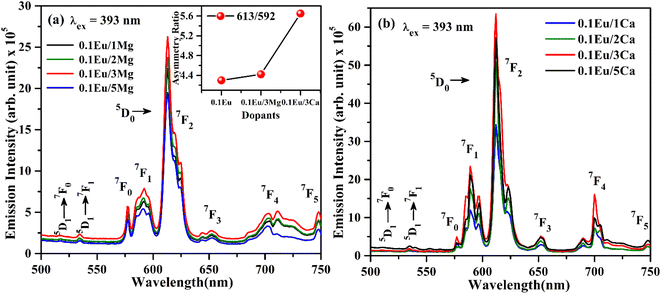 |
| Fig. 9 PL emission spectra of (a) ZnGa2O4:Eu3+/yMg2+ (b) ZnGa2O4:Eu3+/zCa2+ (where y/z = 1, 2, 3, 5 mol%) with λex = 393 nm. | |
The increase in PL intensity of the ZnGa2O4:0.1Eu3+ phosphor via Mg2+ and Ca2+ doping is due to charge imbalance in between the triply ionized Ga and doubly ionized Mg/Ca ions. This causes a crystal field around Eu3+ ion, which enhances its emission intensity. Since this field is larger in the case of Ca2+ ion than that of Mg2+ ion, the enhancement in PL intensity is more in the case of Ca2+ doping. The particles size of ZnGa2O4:0.1Eu3+ phosphor is improved from 1.11 to 1.36 and 1.54 μm through Mg2+ and Ca2+ doping, respectively. The larger particles have large number of activator ions which also contributes to this enhancement. Meetei et al. have also observed an enhancement in PL intensity in the YVO4:Dy3+ phosphor via doping of Ca2+ ion.48
The inset in Fig. 9(a) shows the asymmetry ratio of ZnGa2O4:0.1Eu3+, ZnGa2O4:0.1Eu3+/3Mg2+ and ZnGa2O4:0.1Eu3+/3Ca2+ phosphor samples upon 393 nm excitation, which clearly demonstrates to the enhancement in PL intensity. The asymmetry ratio of the electric dipole transition i.e. (5D0 → 7F2) to the magnetic dipole transition i.e. (5D0 → 7F1) versus 0.1 mol% Eu3+ doped and 3 mol% Mg2+ and Ca2+ co-doped phosphor samples for 613 nm emission band, respectively. The asymmetry ratio signifies the nature of crystal field around the Eu3+ ion, which is responsible for larger PL intensity. It is clear from the inset of figure that the asymmetry ratio is larger for Ca2+ doping compared to Mg2+ doping [see Table 2]. The larger value of asymmetry ratio induces larger photoluminescence in the Ca2+ co-doped ZnGa2O4:Eu3+ phosphor compared to the Mg2+ co-doped ZnGa2O4:Eu3+.
Table 2 Asymmetry ratio (I613 nm/I592 nm) for ZnGa2O4:Eu3+, ZnGa2O4:Eu3+/3Mg2+ and ZnGa2O4:Eu3+/3Ca2+ phosphors on excitation with 393 nm
Phosphor |
Asymmetric ratio (I613 nm/I592 nm) |
ZnGa2O4:0.1Eu3+ |
4.29 |
ZnGa2O4:0.1Eu3+/3Mg2+ |
4.42 |
ZnGa2O4:0.1Eu3+/3Ca2+ |
5.65 |
Fig. 10(a) shows the PL emission spectra of ZnGa2O4:0.1Eu3+, ZnGa2O4:0.1Eu3+/3Mg2+ and ZnGa2O4:0.1Eu3+/3Ca2+ phosphor samples at λex = 290 nm in the range 350–700 nm. It is clear from the figure that the PL emission intensity of host as well as of Eu3+ bands are enhanced in presence of Mg2+ and Ca2+ ions. This is due to crystal field of these ions.23,24,38,47,48 When these phosphor samples are excited with λex = 393 nm, the host is not excited. The emission bands are observed only due to Eu3+ ion in 500–750 nm range [see Fig. 10(b)]. A similar structure is also obtained in the case of 290 nm excitation; however, the PL intensity is relatively larger for Ca2+ doping. The PL intensity of Eu3+ band at 613 nm is enhanced upto 1.20 and 2.91 times for Mg2+ and Ca2+ doping, respectively.
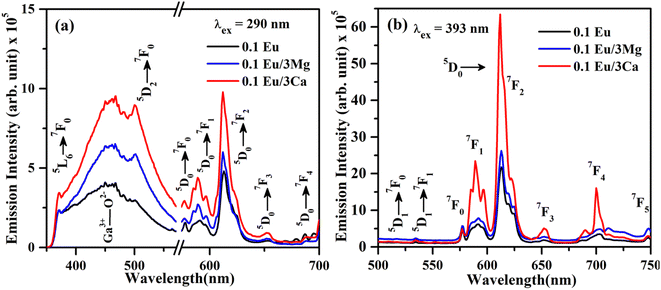 |
| Fig. 10 PL emission spectra of ZnGa2O4:Eu3+, ZnGa2O4:Eu3+/3Mg2+ and ZnGa2O4:Eu3+/3Ca2+ phosphor samples under (a) λex = 290 nm and (b) λex = 393 nm excitation. | |
3.2.4 Effect of annealing on the PL intensity. Fig. 11(a–c) shows the PL emission spectra of ZnGa2O4:0.1Eu3+, ZnGa2O4:0.1Eu3+/3Mg2+ and ZnGa2O4:0.1Eu3+/3Ca2+ phosphor samples unannealed and annealed (at 873 K) for 4 h upon 393 nm excitation. The PL intensity of the samples is found to enhance appreciably for the samples annealed at 873 K temperature. The PL intensity of ZnGa2O4:0.1Eu3+/3Ca2+ phosphor is enhanced more than the ZnGa2O4:0.1Eu3+/3Mg2+ and ZnGa2O4:0.1Eu3+ phosphor samples.24,38,47,48 Actually, the crystallinity of phosphor samples is improved on annealing at 873 K temperature, which affects the PL intensity. Sreena et al. have observed an improvement in the PL intensity of phosphor due to increase in crystallinity as the phosphor samples were calcined at 800 and 900 °C temperatures.49 Similarly, Kaewnuama et al. have also found an increase in PL intensity of the Eu3+ doped lithium lanthanum borate phosphor prepared at higher temperatures.50 A similar result has been also observed in our case.
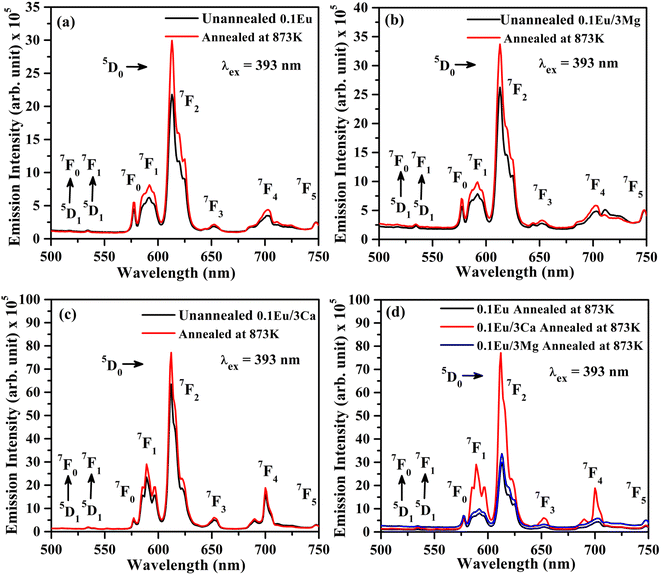 |
| Fig. 11 PL emission spectra of unannealed and annealed (at 873 K) (a) ZnGa2O4:0.1Eu3+, (b) ZnGa2O4:0.1Eu3+/3Mg2+ and (c) ZnGa2O4:0.1Eu3+/3Ca2+ phosphor samples on excitation with λex = 393 nm. (d) A comparison of the PL intensity of annealed samples at 873 K. | |
In order to understand the change in PL intensity due to annealing, we have also compared the PL intensity of ZnGa2O4:0.1Eu3+, ZnGa2O4:0.1Eu3+/3Mg2+ and ZnGa2O4:0.1Eu3+/3Ca2+ phosphor samples annealed at 873 K on excitation with 393 nm (see Fig. 11(d)). It is clear from the figure that the PL intensity of ZnGa2O4:0.1Eu3+ phosphor increases in presence of Mg2+ and Ca2+ ions on annealing.
3.2.5 CIE chromaticity diagram. The CIE (Commission Internationale de E'clarage) coordinates for the ZnGa2O4, ZnGa2O4:xEu3+ (where x = 0.05, 0.1, 0.2, 0.5 and 1.0 mol%), ZnGa2O4:0.1Eu3+/3Mg2+ and ZnGa2O4:0.1Eu3+/3Ca2+ phosphors on excitation with 260, 290 and 393 nm wavelengths have been plotted using GoCIE software and they are shown in Fig. 12(a–c). The obtained CIE coordinates for pure ZnGa2O4 phosphor under 260 nm excitation lies in deep blue region [see Fig. 12(a)]. On doping of different concentrations of xEu3+, 0.1Eu3+/3Mg2+ and 0.1Eu3+/3Ca2+ in the ZnGa2O4 phosphor, the CIE coordinates are tuned slightly in the blue region. The coordinates are shifted from pure blue to bluish-white regions on doping of xEu3+ and co-doping of 0.1Eu3+/3Mg2+ and 0.1Eu3+/3Ca2+ ions in the ZnGa2O4 phosphor upon 290 nm excitation35,36 [see Fig. 12(b)].
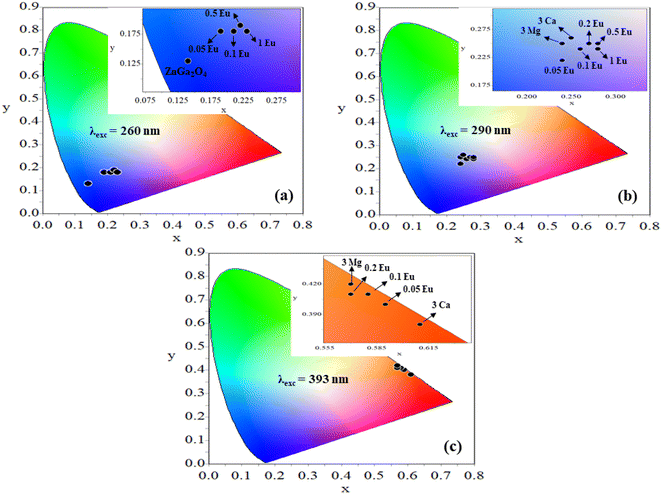 |
| Fig. 12 CIE diagrams of (a) ZnGa2O4 and ZnGa2O4:xEu3+ (where x = 0.05, 0.1, 0.2, 0.5 and 1.0 mol%) phosphors on excitation with 260 nm (b) ZnGa2O4:xEu3+, ZnGa2O4:0.1Eu3+/3Mg2+ and ZnGa2O4:0.1Eu3+/3Ca2+ phosphors on excitation with 290 nm (c) ZnGa2O4:xEu3+, ZnGa2O4:0.1Eu3+/3Mg2+ and ZnGa2O4:0.1Eu3+/3Ca2+ phosphors on excitation with 393 nm. | |
Further, the samples glow with bright red color on excitation with 393 nm, the CIE coordinates varying in the red region for different concentrations of Eu3+ ions [see Fig. 12(c)]. On co-doping of 3Mg2+ and 3Ca2+ ions in the ZnGa2O4:0.1Eu3+ phosphor, the CIE coordinates shift from (0.57, 0.42) to (0.61, 0.38). The CIE coordinates (0.61, 0.38), are close to the National Television System Committee (NTSC) standard value for a pure red color (0.67, 0.33). From this, it is clear that the co-doping of Mg2+ and Ca2+ ions in the ZnGa2O4:Eu3+ phosphor not only enhances the emission intensity but also improves the color perception. This shows that the emitted color is tunable with excitation wavelengths, which are useful in display devices. The calculated values of CIE coordinates are given in Table 3.
Table 3 CIE coordinates for the ZnGa2O4, ZnGa2O4:0.1Eu3+, ZnGa2O4:0.1Eu3+/3Mg2+ and ZnGa2O4:0.1Eu3+/3Ca2+ phosphors under 260, 290 and 393 nm excitations
Phosphor |
CIE coordinates (x,y) at λex = 260 nm |
CIE coordinates (x,y) at λex = 290 nm |
CIE coordinates (x,y) at λex = 393 nm |
ZnGa2O4 |
(0.14,0.13) |
|
|
ZnGa2O4:0.05Eu3+ |
(0.19,0.18) |
(0.24,0.22) |
(0.59,0.40) |
ZnGa2O4:0.1Eu3+ |
(0.21,0.18) |
(0.26,0.24) |
(0.58,0.41) |
ZnGa2O4:0.2Eu3+ |
(0.22,0.19) |
(0.27,0.25) |
(0.57,0.41) |
ZnGa2O4:0.5Eu3+ |
(0.22,0.19) |
(0.28,0.24) |
(0.57,0.42) |
ZnGa2O4:1.0Eu3+ |
(0.23,0.18) |
(0.28,0.25) |
(0.57,0.42) |
ZnGa2O4:0.1Eu3+/3Mg2+ |
|
(0.24,0.25) |
(0.57,0.42) |
ZnGa2O4:0.1Eu3+/3Ca2+ |
|
(0.25,0.26) |
(0.61,0.38) |
The color purity of the phosphor samples has been calculated by using the following relation.36
|
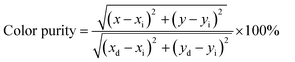 | (x) |
where (
x,
y), (
xi,
yi) and (
xd,
yd) are the CIE coordinates of phosphor, the standard light source for white light and the used dominant wavelength, respectively. The value of color purity of pure ZnGa
2O
4 is 85.87%. However, the color purity of the ZnGa
2O
4:0.1Eu
3+ was calculated to 93.62% upon 393 nm excitation.
4. Conclusions
The Eu3+ doped and Mg2+/Ca2+ co-doped ZnGa2O4 phosphor samples were successfully synthesized by solid-state reaction method at 1473 K. A small part of all the samples were further annealed for four hours at 873 K, which improves the PL intensity of the phosphor samples. The XRD, SEM, EDS, FTIR spectra of all the samples were monitored. The pure ZnGa2O4 emits broad blue emission (350–550 nm) on UV (260 nm) excitation. However, Eu3+ doped and Mg2+/Ca2+ co-doped samples emit blue and red bands on 260 and 290 nm excitations. However, excitation of doped samples with 393 nm emits intense pure red color. The PL intensity of emission bands is enhanced up to 1.20 and 2.91 times via co-doping of Mg2+ and Ca2+ ions in the Eu3+ doped phosphors due to increase in the crystallinity, particles size and crystal field due to charge imbalance. The blue emission due to pure ZnGa2O4 sample shifts slowly towards the bluish-white and red regions for Eu3+ doped and Ca2+/Mg2+ co-doped ZnGa2O4 phosphors on excitation with 290 and 393 nm wavelengths. For 393 nm excitation, it emits only red in all cases. The lifetime of 5D0 level of Eu3+ ion increases on Ca2+/Mg2+ doping and on annealing the sample. The temperature dependent photoluminescence (TDPL) study shows the thermal quenching behavior of the sample with thermal stability ∼65% and activation energy of 0.223 eV in the Eu3+/Ca2+ co-doped phosphor. Thus, the Eu3+ doped and Ca2+ co-doped ZnGa2O4 phosphor is highly thermally stable under external temperature, which may be useful for display devices, blue LEDs, warm red LEDs and plant growth lighting applications.
Conflicts of interest
Authors declare that there is no conflict of interest in the present study.
Acknowledgements
Monika acknowledges to Council of Scientific and Industrial Research, India for providing financial assistance of Senior Research Fellowship (Grant No. 09/013(0826)/2018-EMR-I).
References
- W. Yang, J. Li, B. Liu, X. Zhang, C. Zhang, P. Niu and X. Jiang, Multi-wavelength tailoring of a ZnGa2O4 nanosheet phosphor via defect engineering, Nanoscale, 2018, 10, 19039–19045 RSC.
- J.-H. Lee, H.-J. Park, K. Yoo, B.-W. Kim, J. C. Lee and S. Park, Characteristics of nano-sized ZnGa2O4 phosphor prepared by solution combustion method and solid-state reaction method, J. Eur. Ceram. Soc., 2007, 27, 965–968 CrossRef CAS.
- B. G. Tagiev, G. G. Guseinov, R. B. Dzhabbarov, O. B. Tagiev, N. N. Musaeva and A. N. Georgobiani, Synthesis and luminescent properties of ZnGa2S4: Eu, F and ZnGa2O4: Eu, F, Inorg. Mater., 2000, 36, 1189–1191 CrossRef CAS.
- Monika, R. S. Yadav, A. Bahadur and S. B. Rai, Concentration and pump power-mediated color tunability, optical heating and temperature sensing via TCLs of red emission in an Er3+/Yb3+/Li+ codoped ZnGa2O4 phosphor, RSC Adv., 2019, 9, 40092–40108 RSC.
- I. K. Jeong, H. L. Park and S. Mho, Two self-activated optical centers of blue emission in zinc gallate, Solid State Commun., 1998, 105, 179–183 CrossRef CAS.
- M. Vasile, P. Vlazan, P. Sfirloaga, I. Grozescu, N. M. Avram and E. Rusu, The luminescent properties of a ZnGa2O4 spinel doped with Eu3+ and Er3+ ions, Phys. Scr., 2009, 135, 014046 CrossRef.
- K.-H. Hsu, M.-R. Yang and K.-S. Chen, A study of ZnGa2O4 phosphor prepared by the solid method, J. Mater. Sci.: Mater. Electron., 1998, 9, 283–288 CrossRef CAS.
- L. Zhang, Y. Xu, X. Wu, S. Yin and H. You, Strong and pure red-emitting Eu3+-doped phosphor with excellent thermal stability for warm WLEDs, Mater. Adv., 2022, 3, 2591–2597 RSC.
- J. Zhao, H. Gao, H. Xu, Z. Zhao, H. Bu, X. Cao, L. He, Z. Yang and J. Sun, Structural and photoluminescence of Eu3+ doped Sr2lnTaO6 red phosphor with high color purity, RSC Adv., 2021, 11, 8282–8289 RSC.
- V. G. Nosov, A. A. Betina, T. S. Bulatova, P. B. Guseva, I. E. Kolesnikov, S. N. Orlov, M. S. Panov, M. N. Ryazantsev, N. A. Bogachev, M. Y. Skripkin and A. S. Mereshchenko, Effect of Gd3+, La3+, Lu3+ co-doping on the morphology and luminescent properties of NaYF4:Sm3+ phosphors, Mater, 2023, 16, 2157 CrossRef CAS PubMed.
- N. B. Adam, J. Pisarska, M. Kuwik, T. Goryczka, M. Zubko and W. A. Pisarski, Synthesis and photoluminescent characterization of ceramic phosphors Li2MgGeO4:Ln3+ (Ln3+ = Pr3+ or Tm3+) under different excitation wavelengths, RSC Adv., 2023, 13, 12386–12393 RSC.
- K. Riwotzki and M. Hasse, Wet-chemical synthesis of doped colloidal nanoparticles: YVO4:Ln (Ln = Eu, Sm, Dy), J. Phys. Chem. B, 1998, 50, 10129–10135 CrossRef.
- Y. R. Parauha and S. J. Dhoble, Color-tunable luminescence, energy transfer behavior and I–V characteristics of Dy3+, Eu3+ co-doped La(PO4) phosphors for WLEDs and solar applications, New J. Chem., 2022, 46, 6230–6243 RSC.
- M. Yan, G. Liu, J. Wen and Y. Wang, Blue-light-excited Eu3+/Sm3+ co-doped NaLa(MoO4)2 phosphors: synthesis, characterizations and red emission enhancement for WLEDs, Mater, 2018, 11, 1090 CrossRef PubMed.
- M. Xie, W. Ruan and J. Wang, Energy transfer, tunable photoluminescence of Sr3Lu(PO4)3:Tb3+, Eu3+ phosphors, J. Lumin., 2020, 224, 117278 CrossRef CAS.
- E. Rai, A. Roy, A. Rai, V. J. Fulari and S. B. Rai, Structural and luminescent properties and energy transfer from Tb3+ to Eu3+ in LaVO4:xTb3+/yEu3+ phosphors, J. Alloys Compd., 2023, 937, 168395 CrossRef CAS.
- R. L. Tranquilin, L. X. Lovisa, C. R. R. Almeida, C. A. Paskocimas, M. S. Li, M. C. Oliveira, L. Gracia, J. Andres, E. Longo, F. V. Motta and M. R. D. Bomio, Understanding the white-emitting CaMoO4 Co-Doped Eu3+, Tb3+ and Tm3+ phosphor through experiment and computation, J. Phys. Chem. C, 2019, 123, 18536–18550 CrossRef CAS.
- B. B. Niraula and C. Rizal, Photoluminescence property of Eu3+ doped CaSiO3 nano-phosphor with controlled grain size, Colloids Interfaces, 2018, 2(4), 52 CrossRef CAS.
- A. Dwivedi, M. Srivastava, R. Upadhyay, A. Srivastava, R. S. Yadav and S. K. Srivastava, A flexible photoluminescent nanoprobe for sensitive and rapid detection of arsenic ions concentration, Microchem., 2022, 127, 106969 CrossRef.
- K. Indumathi, S. Tamilselvan, L. Rajasekaran, A. Duke John David, G. Shakil Muhammad, G. Ramalingam and M. Biruntha, Structural and optical properties of Eu3+ doped Sr3Gd[PO4]3 phosphor white-LED application, Mater. Lett., 2022, 309, 131371 CrossRef CAS.
- W. B. Yang, F. B. Xiong, Y. Yang, H. F. Lin, X. G. Meng, E. Ma and W. Z. Zhu, A novel red-emitting Sr7Sb2O12:Eu3+, M+ (M=Li, Na, K) phosphor for warm white LED: synthesis, optical properties, and LED fabrication, Appl. Phys. A, 2022, 128, 584 CrossRef CAS.
- P. Singh, R. S. Yadav and S. B. Rai, Enhanced photoluminescence in a Eu3+ doped CaTiO3 perovskite phosphor via incorporation of alkali ions for white LEDs, J. Phys. Chem. Solids, 2021, 151, 109916 CrossRef CAS.
- D. Singh and S. Sheoran, Synthesis and luminescent characteristics of M3Y2Si3O12:Eu3+ (M = Ca, Mg, Sr and Ba) nanomaterials for display applications, J. Mater. Sci.: Mater. Electron., 2016, 27, 12 Search PubMed.
- E. Rai, R. S. Yadav, D. Kumar, A. K. Singh, V. J. Fullari and S. B. Rai, Improved photoluminescence in Eu3+ doped LaVO4 phosphor via co-doping of Li+/Ca2+ ions, J. Lumin., 2022, 241, 118519 CrossRef CAS.
- Q. Tang, N. Guo, Y. Xin, W. Li, B. Shao and R. Ouyang, Luminous tuning in Eu3+/Mn4+ co-doped double perovskite structure by designing the site-occupancy strategy for solid-state lighting and optical temperature sensing, Mater. Res. Bull., 2022, 149, 111704 CrossRef CAS.
- E. Rai, R. S. Yadav, D. Kumar, A. K. Singh, V. J. Fullari and S. B. Rai, Influence of Bi3+ ion on structural, optical, dielectric and magnetic properties of Eu3+ doped LaVO4 phosphor, Spectrochim. Acta, Part A, 2020, 243, 118787 CrossRef CAS PubMed.
- H. Shi, X. Y. Zhang, W. L. Dong, X. Y. Mi, N. L. Wang, Y. Li and H. W. Liu, Effect of co-doped metal cations on the properties of Y2O3:Eu3+ phosphors synthesized by gel-combustion method, Chin. Phys. B, 2016, 25, 047802 CrossRef.
- Z. Yang, J. Liao, S. Lai, H. Wu, Z. Fan, J. Qiu, Z. Song, Y. Yang and D. Zhou, Energy transfer and photoluminescence properties in Bi3+ and Eu3+ co-doped ZnGa2O4, Mater. Express, 2013, 3, 350–354 CrossRef CAS.
- J. Cui, Y. Zheng, Z. Wang, L. Cao, X. Wang, Y. Yao, M. Zhang, M. Zheng, Z. Yang and P. Li, Improving the luminescence thermal stability of Ca3Y2Ge3O12:Cr3+ based on cation substitution and its application in NIR LEDs, Mater. Adv., 2022, 3, 2772–2778 RSC.
- B. Yang, Y. Feng, Q. Zhao, M. He and Y. Lv, Novel tunable green-red luminescence in Mn2+ doped Ca9Tb(PO4)7 phosphors based on the Mn2+ regulation and energy transfer, Coatings, 2020, 10, 952 CrossRef CAS.
- M. Rajendran and S. Vaidyanathan, A novel highly efficient host-sensitized red emitting (Ba2YV3O11:Eu3+) phosphor for hybrid white LEDs, ChemistrySelect, 2020, 5, 5128–5136 CrossRef CAS.
- M. Rajendran, S. K. Samal and S. Vaidyanathan, A novel self-activated (bluish-green) and Eu3+ doped (red) phosphors for warm white LEDs, J. Alloys Compd., 2020, 815, 152631 CrossRef CAS.
- Y. Zhang, J. Xu, Q. Cui and B. Yang, Eu3+-doped Bi4Si3O12 red phosphor for solid state lighting: microwave synthesis, characterization, photoluminescence properties and thermal quenching mechanisms, Sci. Rep., 2017, 7, 42464 CrossRef CAS PubMed.
- S. Sheoran, S. Singh, A. Mann, A. Samantilleke, B. Mari and D. Singh, Novel synthesis and Optical investigation of trivalent Europium doped MGd2Si3O10 (M = Mg2+, Ca2+, Sr2+ and Ba2+) nanophosphors for full-color displays, J. Mater. NanoSci., 2019, 6, 73–81 CAS.
- Monika, R. S. Yadav, A. Bahadur and S. B. Rai, Near-Infrared light excited highly pure green upconversion photoluminescence and intrinsic optical bistability sensing in a Ho3+/Yb3+ co-doped ZnGa2O4 phosphor through Li+ doping, J. Phys. Chem. C, 2020, 124, 10117–10128 CrossRef CAS.
- Monika, R. S. Yadav, A. Rai and S. B. Rai, NIR light guided enhanced photoluminescence and temperature sensing in Ho3+/Yb3+/Bi3+ co-doped ZnGa2O4 phosphor, Sci. Rep., 2021, 11, 4148 CrossRef CAS PubMed.
- R. Kokate, P. Rohilla, S. Kaur, A. S. Rao and V. Singh, Visible emission characteristics in Tb3+ doped KNa3Al4Si4O16 phosphor, Optik, 2021, 243, 167391 CrossRef CAS.
- A. Maurya, R. S. Yadav, R. V. Yadav, S. B. Rai and A. Bahadur, Enhanced green upconversion photoluminescence from Ho3+/Yb3+ co-doped CaZrO3 phosphor via Mg2+ doping, RSC Adv., 2016, 6, 113469–113477 RSC.
- J. Xiang, J. Zheng, Z. Zhou, H. Suo, X. Zhao, X. Zhou, N. Zhang, M. S. Molokeev and C. Guo, Enhancement of red emission and site analysis in Eu2+ doped new-type structure Ba3CaK(PO4)3 for plant growth white LEDs, Chem. Eng. J., 2019, 356, 236–244 CrossRef CAS.
- H. Yao, Y. Zhang and Y. Xu, Dopant concentration-dependent morphological evolution of Zn2GeO4:Mn2+/Eu3+ phosphor and optical temperature sensing performance, J. Alloys Compd., 2019, 770, 149–157 CrossRef CAS.
- A. Luchechko, O. Kravets, L. Kostyk and O. Tsvetkova, Luminescence spectroscopy of Eu3+ and Mn2+ ions in MgGa2O4 spinel, Radiat. Meas., 2016, 90, 47–50 CrossRef CAS.
- M. Vasile, P. Vlazan and N. M. Avram, Characterization and optical properties of ZnGa2O4:Eu3+ nanophosphor grown by hydrothermal method, J. Alloys Compd., 2010, 500, 185–189 CrossRef CAS.
- Y.-M. Peng, Y.-K. Su and R.-Y. Yang, The charge transfer transition phenomenon and microstructure of Eu3+ -doped NaCaPO4 phosphors sintered with NH4Cl flux via solid-state reaction, Mater. Res. Bull., 2013, 48, 1946–1951 CrossRef CAS.
- M. M. Yawalkar, G. D. Zade, V. Singh and S. J. Dhoble, Investigation of luminescence processes in Li6Gd(BO3)3:Eu3+ phosphor, J. Mater. Sci.: Mater. Electron., 2017, 28, 180–189 CrossRef CAS.
- C. S. Vandana and B. H. Rudramadevi, The effect of Mg2+ concentration on structural and luminescent properties of CaTiO3: Eu3+ phosphor, Ferroelectrics, 2018, 524, 168–180 CrossRef CAS.
- M. K. Hussen and F. B. Dejene, Effect of Cr3+ doping on structural and optical property of ZnGa2O4 synthesized by sol gel method, Optik, 2019, 181, 514–523 CrossRef CAS.
- R. S. Yadav, S. J. Dhoble and S. B. Rai, Enhanced photoluminescence in Tm3+, Yb3+, Mg2+ tri-doped ZnWO4 phosphor: Three photon upconversion, laser induced optical heating and temperature sensing, Sens. Actuators, B, 2018, 273, 1425–1434 CrossRef CAS.
- S. D. Meetei, M. D. Singh and S. D. Singh, Facile synthesis, structural characterization, and photoluminescence mechanism of Dy3+ doped YVO4 and Ca2+ co-doped YVO4: Dy3+ nano-lattices, J. Appl. Phys., 2014, 115, 204910 CrossRef.
- T. S. Sreena, P. P. Rao, K. N. Ajmal and A. K. V. Raj, Influence of morphology on luminescence properties of xenotime-type phosphors NaYP2O7:Eu3+ synthesized via solid state and citrate-gel routes, J. Mater. Sci.: Mater. Electron., 2018, 9, 7458–7467 CrossRef.
- E. Kaewnuama and J. Kaewkhao, Synthesis-temperature effect on the luminescence under light and UV excitation of Eu3+ doped lithium lanthanum borate phosphor, Mater. Today: Proc., 2018, 5, 15086–15091 Search PubMed.
- Q. Zhang, X. Wang, X. Ding and Y. Wang, A potential red-emitting phosphor BaZrGe3O9:Eu3+ for WLED and FED applications: Synthesis, structure, and luminescence properties, Inorg. Chem., 2017, 56, 6990–6998 CrossRef CAS PubMed.
- G. Zhu, Z. Ci, Y. Shi, M. Que, Q. Wang and Y. Wang, Synthesis, crystal structure and luminescence characteristics of a novel red phosphor Ca19Mg2(PO4)14:Eu3+ for light emitting diodes and field emission displays, J. Mater. Chem. C, 2013, 1, 5960–5969 RSC.
|
This journal is © The Royal Society of Chemistry 2023 |