DOI:
10.1039/D3RA02957C
(Paper)
RSC Adv., 2023,
13, 33514-33524
Effect of M-type reactive dyes on dyeing properties of twisted bamboo fiber bundles
Received
4th May 2023
, Accepted 6th October 2023
First published on 15th November 2023
Abstract
The dyeing properties of twisted bamboo fiber bundles were studied by using the combination of three primary colors in M-type reactive dyes. The study found that the three dyes of red, yellow and blue have good color rendering in the actual dyeing process, and because the molecular structures of the three are similar, the chemical reactions during the fixation are the same, so the final dyeing rate results are similar, which were 29–32%. Compared with the undyed twisted bamboo fiber bundle, the mechanical properties of the three-color twisted bamboo fiber bundle also changed significantly, and the tensile strength increased by 13.79% on average. The changes of elastic modulus and elongation at break showed that the three-color twisted bamboo fiber bundle had excellent flexibility. In addition, there are significant color differences between the samples of each color. When the color indexes of DB are used as the benchmark, the ΔE* of other dyed samples varies from 40 to 80, and the color of each sample is relatively uniform, without an obvious color flower phenomenon. This indicates that twisted bamboo fiber bundles with richer colors can be prepared by different combinations of three primary dyes, which can improve the ornamental value of bamboo fiber bundles after processing into large blocks and their application potential in home textiles, interior decoration and other fields.
Introduction
With the advancement and development of science and technology, synthetic fibers have gradually entered the public's field of vision and occupy an increasing proportion in the modern textile industry. However, due to the increasing shortage of oil resources, the high cost of traditional natural fiber cultivation and the global ecological environment changes, people began to try to extract and prepare new textile fibers from other renewable biomass materials.1–3 In this context, non-wood fiber materials began to enter the public view and gradually attracted people's attention. The structure and chemical composition of bamboo and wood are similar, but the growth cycle of wood is long and the mechanical properties of fast-growing wood are poor. The fast-growing, high-yield, renewable and widely distributed growth characteristics of bamboo make it have high ecological benefits and economic and social value. It is recognized as an energy bank for green environmental protection and sustainable development.4,5 In addition, bamboo is composed of three parts: the vascular system, basic system and epidermal system. The unique microstructure gives bamboo excellent mechanical properties.6–8 Therefore, bamboo is suitable as an alternative material for wood in many research fields, such as transparent bamboo,9 antibacterial bamboo,10 conductive bamboo,11 superhydrophobic bamboo12 and other bamboo-based materials with various properties.
Bamboo fiber fabric is one of the bamboo products that have gradually emerged in recent years. Because of its natural antibacterial properties and moisture permeability, bamboo fiber has been used in the production of textile fibers since ancient times. At present, bamboo fiber can be divided into natural bamboo fiber and chemical bamboo fiber. Natural bamboo fiber is mainly extracted by physical methods, also known as bamboo fiber. In the preparation, the fine filamentous bamboo fibers are obtained from the bulk bamboo mainly by mechanical rolling and dredging.13–16 The preparation method of chemical bamboo fiber is similar to that of viscose fiber, and it also basically follows the related manufacturing process of new regenerated cellulose fiber. Bamboo was crushed and combined with alkali treatment to process it into pulp, and then the bamboo cellulose fiber was transformed into a fiber that can be spun into bamboo yarn by wet spinning.17–19 In practical applications, bamboo pulp fibers can be blended with other natural plant fibers or animal fibers and the proportion of various fibers adjusted to obtain fabrics with different effects.19 However, the preparation and extraction process of these bamboo textile fibers are complicated, which makes bamboo fiber fabrics only occupy a very small market share in the actual production and application of textiles. Therefore, this study adopts a relatively simple process route, using 3–4 year-old bamboo as the substrate, through delignification and a twisting treatment to prepare twisted bamboo fiber bundles with excellent flexibility and spinnability.20
However, in the actual fabric dyeing production, it is often necessary to take into account the aesthetics and diversity of the products, which makes it rare for single-color fabrics to appear on the market. Those colorful and rich categories of yarns and fabrics are mostly completed by multi-color dyes. Reactive dyes have the characteristics of bright color, low cost and complete chromatography, and the active groups in them can be combined with cellulose by chemical bond, so that the dye molecules are firmly attached to the cellulose fiber. There are three main active groups in reactive dyes, namely, triazine, pyrimidine and vinyl sulfone. Different groups will cause different bonding reactions between dyes and fibers.21,22 In this experiment, the three primary colors of M-type reactive dyes were used to dye twisted fiber bundles and their physical and chemical properties were studied. Since the M-type reactive dye is a medium temperature dye, the fixation temperature is set to 60 °C. The dye molecule contains monochlorotriazine group and β-hydroxyethyl sulfone sulfate active group, which undergo nucleophilic substitution reaction and nucleophilic addition reaction with cellulose during dyeing, respectively.23–25 In this paper, the changes of the properties of dyed bamboo fiber bundles were investigated by the dyeing percentage, tensile test results, chemical composition and microstructure of the fiber bundles before and after dyeing. In addition, the color difference analysis of three primary colors and color matching bamboo fiber bundles was carried out by color difference test to explore the feasibility of dyeing twisted bamboo fiber bundles with three primary colors reactive dyes (Fig. 1).
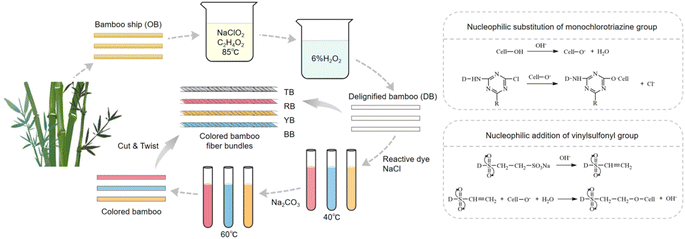 |
| Fig. 1 Preparation process of colored bamboo fiber bundles and reaction mechanism of M-type reactive dyes with cellulose. | |
Experimental and materials
Materials
The substrate used in this experiment was Phyllostachys heterocycla with three to four years old, which was taken from Anxi County, Quanzhou City, Fujian Province, China. The chemicals involved in the test are as follows: sodium chlorite, purity 80%, provided by China Shanghai McLean Co., Ltd; glacial acetic acid, 30% hydrogen peroxide, sodium chloride and sodium carbonate were all analytically pure and provided by China Nanjing Chemical Reagent Co., Ltd; the M-type trichromatic reactive dyes were reactive red M-3BE, reactive yellow M-3RE and reactive blue M-2GE, which were provided by Jiangsu Zhenqing Dye Factory.
The instruments used in the experiment are as follows: electric heating constant temperature blast drying box, model DHG-9643BS-III, provided by Shanghai Xinmiao Medical Device Manufacturing Co., Ltd; electronic balance, model JA21002, provided by Nanjing Xianou Instrument Manufacturing Co., Ltd; electro-thermal magnetic stirrer, model DF-101Z, provided by Nanjing Wozhong Instrument Equipment Co., Ltd; topPette manual single channel adjustable pipette, 20–200 μl, provided by Beijing Dalong Xingchuang Experimental Instrument Co., Ltd. Multifunctional grinder, model DE-100g, provided by Zhejiang Hongjingtian Industry & Trade Co., Ltd.
Experimental methods
Decolorization treatment of bamboo fiber bundles. The original bamboo was processed into 100 mm (length) × 6 mm (width) × 0.6 mm (thickness) of bamboo (original bamboo, OB), and dried in a blast oven at 60 °C for 24 h. Sodium chlorite solution with mass fraction of 4% was prepared, and glacial acetic acid was added to adjust the pH of the solution to 4.6. The dried bamboo pieces were put into a mixed solution and heated in a water bath at 85 °C for 4–5 h. In order to reduce the influence of the color of bamboo bundles on the dyeing effect, the delignification bamboo slices were immersed in a 6% hydrogen peroxide solution for 5 min. Delignified bamboo (DB) was obtained by washing with deionized water and drying at 60 °C for 12 h.
Preparation of three primary colors twisted bamboo fiber bundles. Reactive red M-3BE, reactive yellow M-3RE and reactive blue M-2GE were used to prepare 1.0% (o.w.f.) (on weight the fabric) dye solution. 30 g L−1 sodium chloride was added at 40 °C. After complete dissolution, decolored bamboo slices were added. The bath ratio was 1
:
20 and the dyeing time was 25 min. 10 g L−1 sodium carbonate was added to the dyeing bath, and the temperature was raised to 60 °C at a rate of 4°C min−1, and the fixation time was 35 min at this temperature. After dyeing, the bamboo pieces were taken out and washed with hot and cold water alternately until the washing solution was neutral. The bamboo pieces were dried at room temperature for 60 min. The bamboo slices before and after dyeing were liberated along the fiber direction into bamboo fiber bundles with a width of about 0.6 mm, and then twisted and dried. The resulting twisted bamboo fiber bundles are referred to as TB (twisted bamboo fiber bundle), RB (red twisted bamboo fiber bundle), YB (yellow twisted bamboo fiber bundle), BB (blue twisted bamboo fiber bundle).
Characterization method
Chemical composition test. The chemical composition of raw bamboo (OB), decolored bamboo (DB) and three primary color twisted bamboo fiber bundles (RB, YB, BB) were tested and characterized.Fourier transform infrared spectroscopy was measured using a VERTEX 80V FTIR spectrometer (Bruker Spectroscopy Instruments, USA). During the test, the infrared microscope selected the reflection mode, and the scanning range was 4000–500 cm−1.
The three major tests were performed according to the National Renewable Energy Laboratory (NREL) method.26
X-ray diffraction tests were performed using an X-ray single crystal diffractometer (D8 VENTURE type). During the test, 2θ is set to 5–80°, and the interval is 0.02°. The calculation formula of crystallinity is as follows, where I200 is the intensity of the diffraction peak at 2θ = 22°, and Iam is the intensity of the diffraction peak at 2θ = 16°.
|
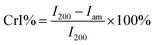 | (1) |
X-ray photoelectron spectroscopy was performed using an AXIS UltraDLD X-ray photoelectron spectrometer (AXIS UltraDLD, Shimadzu, U.K.).
Micro-morphology test. The microstructure of the samples was observed by FEI 200 scanning electron microscope (FEI, USA). Before the test, an ultra-thin slicer was used to cut the test sample with a suitable length, and the sample was fixed on the stage to facilitate the observation of the microstructure of the sample along the fiber direction and end face.
Dyeing rate test. The dye solution was prepared according to the dyeing formula, diluted to a certain multiple and diluted to a constant volume, and the absorbance of the diluent was measured using a U-3900 spectrophotometer. The Abs mode was selected for the determination, the spectral scanning range was set to 200–800 nm, and the scanning speed was 300 nm min−1. The standard working curve of the dye was drawn, and the maximum absorption wavelength (λmax) of each standard dye solution and the absorbance A at λmax were determined.27In the dyeing stage, a certain amount of dyeing residue was taken and diluted in the dyeing bath every 5 minutes.28 The absorbance Ai was measured at λmax and the dye uptake rate was calculated, so as to draw the dyeing rate curve. The formula for calculating the dye-uptake is as follows, where A0 is the absorbance of the initial dye solution diluted by n0 times, and Ai is the absorbance of the dye residue diluted by ni times.
|
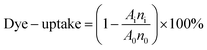 | (2) |
Tensile strength test. The tensile test of the samples was carried out by universal mechanical testing machine. The two ends of the dried sample were fixed with a fixture, the gauge was set to 40 mm, and the loading speed was 3 mm min−1. The tensile strength (N mm−2), elastic modulus (MPa) and elongation at break (%) are used as the evaluation indexes to evaluate the tensile properties of twisted bamboo fiber bundles. The calculation formulas of each index are as follows: |
Tensile strength: σ = Fb/So
| (3) |
|
Elastic modulus: E = σ/ε
| (4) |
|
Breaking elongation: e = (La − Lo)/Lo
| (5) |
Fb is the maximum force when the specimen is broken, and the unit is N; So is the original cross-sectional area of the sample, in mm2. E is elastic modulus, σ is stress, ε is strain; e is the elongation at break; Lo is the initial length of the sample; length of La specimen at break.
Color difference test. In order to explore the color matching performance of trichromatic reactive dyes in dyeing twisted bamboo fiber bundles, three reactive dyes were compounded in equal proportions according to the combination of red & yellow, red & blue, yellow & blue, red & yellow & blue, and dyed according to the above experimental steps. The resulting four groups of twisted bamboo fiber bundles are referred to as ORB (orange twisted bamboo fiber bundle), GB (green twisted bamboo fiber bundle), PB (purple twisted bamboo fiber bundle), GRB (grey twisted bamboo fiber bundle).The L*, a*, b* values of DB, RB, ORB, YB, GB, BB, PB and GRB samples under CIE Lab D65 10° standard were measured by color detector (RM200 type). Each group of samples was measured at three points, and the L*, a*, and b* values of the DB samples were used as benchmarks and the delta E (ΔE) color difference calculation formula was used to compare the color deviations between different color samples and take the average. The formula is as follows:
|
ΔE = (ΔL∗)2 + (Δa∗)2 + (Δb∗)2
| (6) |
|
 | (7) |
|
 | (8) |
|
 | (9) |
In the formula
,
and
represent the brightness value, red-green dimension and yellow-blue dimension of DB respectively.
,
and
represent the light and dark values, red and green dimensions and yellow and blue dimensions of each sample after staining and color matching, respectively. ΔL* represents the change of brightness before and after sample treatment. If it is positive, it is bright, and if it is negative, it is dark. Δa* indicates that if it is positive, it is red, and if it is negative, it is green; δb* indicates that if it is positive, it is yellow, and if it is negative, it is blue. ΔE* represents the total color difference, and the larger the value, the greater the color deviation.
Results and discussion
Chemical composition analysis
As shown in Fig. 2(b), OB has several characteristic peaks. The C–H stretching vibration peaks include O–H stretching vibration peak (3325 cm−1), C–H stretching vibration peak (2921 cm−1), C
O stretching vibration peak in hemicellulose (1734 cm−1), conjugated β-carbonyl group in lignin (1636 cm−1), aromatic ring skeleton group in lignin stretching vibration peak (1496 cm−1), bending vibration of asymmetric aromatic ethers (1032 cm−1), C–H in-plane bending vibration of cellulose and hemicellulose (1369 cm−1), aromatic ring C–H out-of-plane vibration peak (898 cm−1,825 cm−1). These peaks and peak shapes are closely related to lignin, cellulose, hemicellulose and some polysaccharides in bamboo. The chemical composition of bamboo after decolorization changed significantly, and mainly appeared near the functional groups of lignin, indicating that lignin was largely decomposed.29
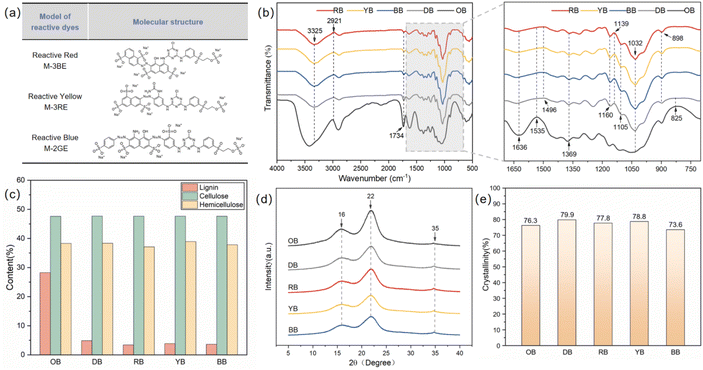 |
| Fig. 2 (a) Molecular structure of the dye; (b) analysis of characteristic functional groups of raw bamboo fiber bundles (OB), decolored bamboo fiber bundles (DB) and three primary color twisted bamboo fiber bundles (RB, YB, BB); (c) relative content of three major elements; (d) XRD pattern of the sample; (e) the crystallinity of the sample. | |
The three medium-temperature reactive dyes used in the dyeing process have similar structures and the same reaction mechanism. Therefore, the infrared spectra of the three groups of dyed bamboo fiber bundles also show a high degree of similarity. In dyed bamboo fiber bundles, the absorption peaks of C–O–C (1160 cm−1 and 1105 cm−1) were enhanced, indicating that the monochlorotriazine group in the dye structure reacted with bamboo-based cellulose and hemicellulose to form covalent bonds. In addition, the stretching vibration peaks of RB, YB and BB at 1139 cm−1 are enhanced, which is caused by S
O in the structure of vinylsulfone group. It can be basically proved that another active group in the dye molecule, vinylsulfone group, has also been successfully covalently bound to bamboo fiber, which further proves that the reactive dye molecules have been successfully attached to RB, YB and BB.
Fig. 2(c) shows the changes of the three major elements of bamboo fiber bundles before and after decolorization and dyeing. The lignin content of the original bamboo (OB) is 28.22%, the cellulose content is 47.61%, and the hemicellulose content is 38.29%, which is consistent with the current research on the three major elements of bamboo.30,31 The lignin content of decolorized bamboo fiber bundle (DB) pretreated by sodium chlorite method was significantly reduced by 4.87%, which was 82.74% lower than that of the original bamboo, while the content of cellulose and hemicellulose did not change significantly, indicating that the decolorization treatment did not have a significant effect on the structure of these two substances and lignin was largely decomposed. After dyeing the decolored bamboo fiber bundles with red, yellow and blue reactive dyes, the lignin of the three groups of samples showed different degrees of reduction, and the relative contents were 3.41%, 3.87% and 3.64%, respectively. Compared with DB further reduced. The content of cellulose and hemicellulose in the original bamboo, decolorized bamboo fiber bundles and dyed bamboo fiber bundles did not change much in the graph, especially the column height of cellulose was basically flat, indicating that the alkaline dye bath and the formation of new covalent bonds during the dyeing process did not cause too much damage to the cellulose structure.
In order to observe the crystal structure changes of bamboo fiber bundles before and after decolorization and dyeing, the samples were analyzed according to XRD test results. As shown in Fig. 2(d), the diffraction peaks of the five groups of samples appeared at 2θ = 16°, 2θ = 22°and 2θ = 35°, corresponding to the diffraction peaks of cellulose (101), (002) and (040), respectively.32 After decolorization treatment, the crystallinity of DB was significantly higher than that of OB. This was due to the removal of lignin, which increased the relative content of cellulose. The cellulose molecules in the amorphous region began to aggregate and rearrange and construct under the action of hydrogen bonds to form macromolecular aggregates, which increased the crystallinity. Combined with the results of infrared spectroscopy and three major elements test, it can be seen that dyeing treatment has no effect on the structure of cellulose in bamboo fiber bundles. It may be that the hydrogen bonds formed in the dyeing reaction strengthen the interaction between cellulose molecules, which improves the crystallinity of the tricolor bamboo fiber bundles to varying degrees. In addition, the crystalline region has a dense structure, which is closely related to the tensile properties of macromolecular fibers. During the preparation of dyed bamboo fiber bundles, the order and high strength of the crystalline region in the fiber are well retained, and the orientation of the fiber is also consistent with the direction of the molecular chain being pulled. Therefore, the increase of crystallinity indicates that the dyeing treatment has a promoting effect on the tensile properties of bamboo fiber bundles.33
In order to further explore the changes of chemical components in the preparation process of three-color twisted bamboo fiber bundles, XPS was used to quantitatively analyze the carbon and oxygen elements in the samples. In the existing research, it can be seen that C1, C2, C3, C4 and O1, O2 correspond to cellulose, hemicellulose, lignin and other extracts in bamboo. Therefore, the surface chemical properties of each group of samples can be characterized according to the content changes of C 1s and O 1s components and the change of O/C ratio. Combined with Fig. 3 and Table 1, it can be seen that the C1 and O1 in the bamboo fiber bundles treated with sodium chlorite were greatly reduced, and the O/C showed an increasing trend, indicating that a large amount of lignin was removed during the treatment process, which was consistent with the conclusions in the existing research. Compared with DB, the content of Cl in RB, YB and BB decreased by 6.80%, 11.31% and 30.24%, respectively. The content of other components and the value of O/C also changed accordingly. It is proved that some lignin is lost in the process of dyeing DB with M-type reactive dyes, which may be accompanied by a small amount of cellulose and hemicellulose structure destruction. This change corresponds to the three major test results in the previous article.34,35
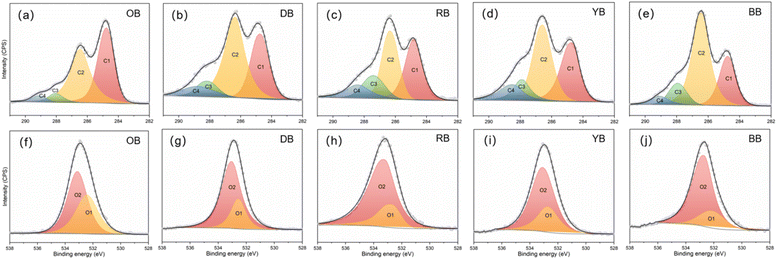 |
| Fig. 3 High resolution (a–e) C 1s and (f–j) O 1s fitting spectra. | |
Table 1 The component classification and peak separation results of C and O
Element component |
C (%) |
O (%) |
O/C (%) |
C1 |
C2 |
C3 |
C4 |
O1 |
O2 |
Binding type |
C–C, C–H |
C–O |
C–O |
O–C O |
O–C O |
C–O |
— |
Main resources |
Lignin and extracts |
Cellulose and hemicellulose |
Cellulose |
Hemicellulose and extracts |
Lignin |
Cellulose and hemicellulose |
— |
Content (%) |
OB |
45.62 |
45.05 |
4.82 |
4.51 |
34.87 |
65.13 |
33.01 |
DB |
37.93 |
36.98 |
14.25 |
10.84 |
27.66 |
72.34 |
40.16 |
RB |
35.35 |
37.34 |
16.17 |
11.14 |
21.91 |
78.09 |
47.29 |
YB |
33.64 |
42.85 |
11.62 |
11.89 |
24.82 |
75.18 |
45.71 |
BB |
26.47 |
55.92 |
12.23 |
5.38 |
20.52 |
79.48 |
46.32 |
Micromorphology analysis
In order to further explore the effect of reactive dye dyeing on the internal structure of bamboo fiber bundles, the microstructure of the samples was characterized. As shown in Fig. 4(a–d), orderly bamboo fibers, nearly round parenchyma cells and impurities on the surface of bamboo fiber bundles can be observed in the scanning electron microscope images of the end face and along the fiber direction of the original bamboo fiber bundles. There are starch granules in some cell cavities and many pits on the cell wall. Fig. 4(e–g) is a bamboo fiber bundle after decolorization treatment. Its end face is approximately circular. After amplification and display, it can be found that the impurities on the surface of TB are removed. The cells and fibers in the end face and along the fiber direction still maintain an orderly arrangement, but the starch granules in the cells have not been observed, which proves that they are decomposed during the decolorization process. The original round thin-walled cells shrink, the cell wall becomes thinner, the connection between cells is weakened, and the surface is prone to cracks under the action of twisting force during twisting.
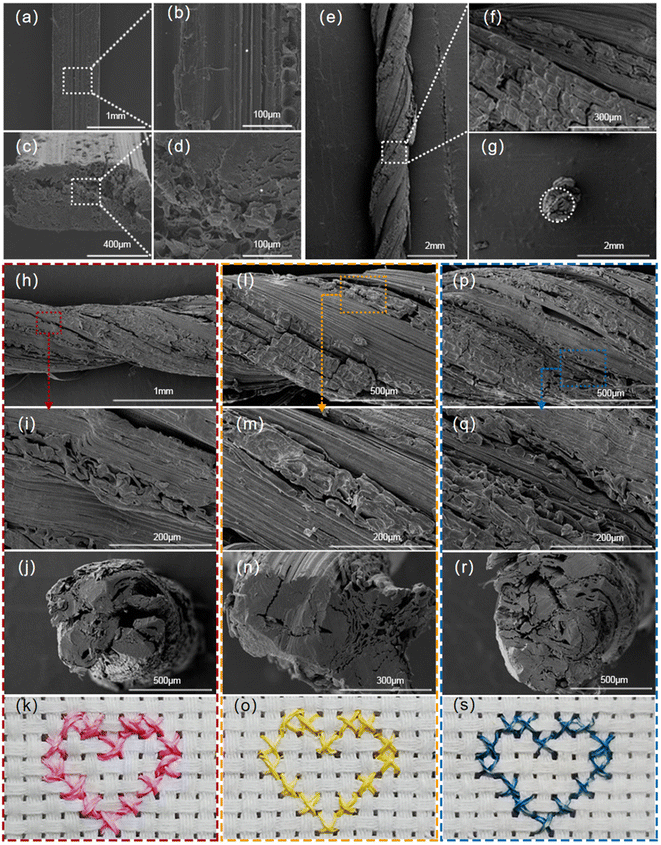 |
| Fig. 4 Scanning electron microscope images of the sample along the fiber direction and end face. (a–d) raw bamboo fiber bundles; (e–g) twisted bamboo fiber bundle; (h–j) red twisted bamboo fiber bundle; (l–n) yellow twisted bamboo fiber bundle; (p–r) blue twisted bamboo fiber bundle; (k,o,s) three primary colors twisted bamboo fiber bundles embroidered patterns on cross embroidered cloth. | |
In Fig. 4(h–p) are the microscopic morphology of bamboo fiber bundles with red, yellow and blue colors, respectively. Dyeing treatment further reduced the lignin content, the hydrogen bonds on cellulose and hemicellulose were combined with dye molecules, and the interaction between fibers and cells was weakened. In the figure, the cell shrinkage was more serious, the surface was rugged, and the internal pits were more obvious. The original neat cell sections were destroyed and showed a messy effect, the cell wall became thinner and partially dissolved, and the fiber bundles became loose and porous. Twisting is equivalent to the densification of dyed bamboo fiber bundles, which improves the defects of loose and easy dispersion of fiber bundles and increases the strength of fiber bundles. This is more intuitively reflected in the cross-sectional SEM diagram of the three-color bamboo fiber bundle. From Fig. 4(j), (m) and (p), it can be found that the vascular system can be seen on the cross-section of the three groups of samples, including the catheter, the fiber sheath and the parenchyma around the catheter. These structures show severe deformation, the internal space is squeezed, and the cross-sectional shape of some catheter cells changes from round to linear.
Dyeing rate analysis
As shown in Fig. 5, the UV-visible absorption spectrum shows that the three color dyes have the maximum absorption wavelength λmax at 543 nm, 419 nm and 599 nm, respectively. The absorbance value of each sample at λmax is determined to calculate the dye uptake value and draw the dye uptake rate curve.36–38 By analyzing the dyeing rate curve, it can be found that when dyeing to 5 min, the initial dyeing slope from large to small is reactive yellow, reactive red and reactive blue. During the dyeing period of 5–25 min, the dye was mainly adsorbed between the dye and the fiber. At 25 min, the fixing agent was added to the dyeing bath. The dye molecules were rapidly combined with cellulose under the action of OH−, and the colored substances in the dyeing bath were reduced. In the dyeing rate curve, the dyeing rate at this stage increased suddenly, and then tended to be gentle. The final dyeing rates of red, yellow and blue primary color bamboo fiber bundles were 29.7%, 32.0% and 29.2%, respectively. On the whole, the overall trend of the dyeing rate curve of the three groups of samples is similar, and the specific values of the dyeing rate and the dyeing rate in each stage are also similar, indicating that the bamboo fiber bundle is the same amount of color in the dyeing and fixing stage. It can be judged that the group of dyes has good compatibility and high synchronization in the dyeing process, and the probability of uneven color of the samples will be greatly reduced during the test of compound dyes.
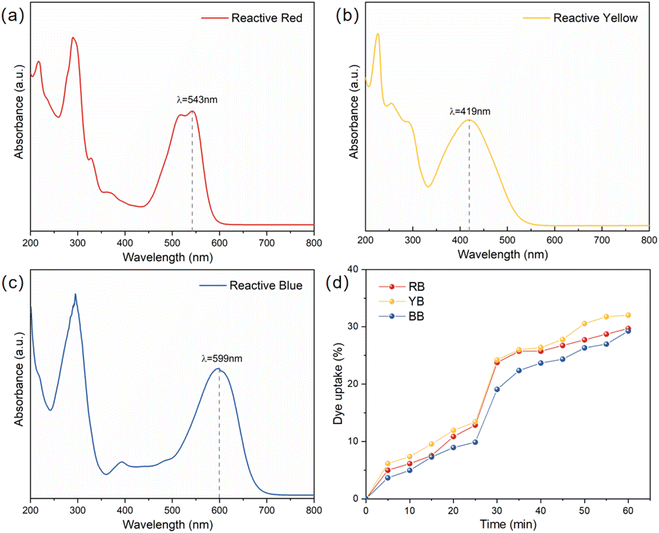 |
| Fig. 5 (a–c) UV absorption spectra of reactive red M-3BE, reactive yellow M-3RE, and reactive blue M-2GE; (d) dyeing rate curve of three twisted bamboo fiber bundles. | |
Analysis of tensile properties
As shown in Fig. 6(a), the original bamboo has excellent tensile strength, while the tensile strength of DB is only 28.2% of that of OB, indicating that the decolorization treatment will cause the bamboo bundle to lose some mechanical properties. Combined with Fig. 6(b–d), it can be seen that the brittleness of OB and DB is strong, which indicates that the sample is prone to fracture during bending. The tensile strength and elongation at break of TB are better than those of DB, and the elastic modulus is greatly reduced, indicating that TB has better tensile properties and excellent flexibility, which is due to twisting treatment. The tensile properties of bamboo fiber bundles dyed with trichromatic reactive dyes showed an overall improvement trend compared with TB. Through the analysis of Fig. 6(a–c), it can be seen that the tensile strength of RB, YB and BB is 2.44%, 11.36% and 27.57% higher than that of TB, respectively, while the elongation at break is slightly decreased, and the elastic modulus of the three groups is similar to that of TB. It was proved that more hydrogen bonds formed by the combination of dye molecules with double active groups and cellulose strengthened the interaction between cellulose molecules. In addition, decolorization treatment retained well-arranged cellulose nanofibers and porous structures in bamboo bundles while removing lignin. Twisting treatment made the internal of bamboo bundles more closely combined, which increased the external force required for cellulose nanofibers to slip until fracture when bamboo fiber bundles were pulled along the fiber direction. It can be seen from the stress–strain curve of the sample in Fig. 6(d) that TB experienced a longer elastic deformation stage, which corresponds to the highest elongation at break in Fig. 6(c), while RB, YB, and BB are longer in the stage of resisting plastic deformation. From the overall trend of the curve, the slope of the curve of the three-primary-color bamboo fiber bundle before reaching the breaking point is significantly lower than that of TB, and the breaking point is not as sharp as TB. The above shows that the rigidity of the fiber bundle after dyeing is reduced and has better toughness. According to the above analysis, the dyeing of medium temperature reactive dyes can give bamboo fiber bundles more excellent tensile properties.
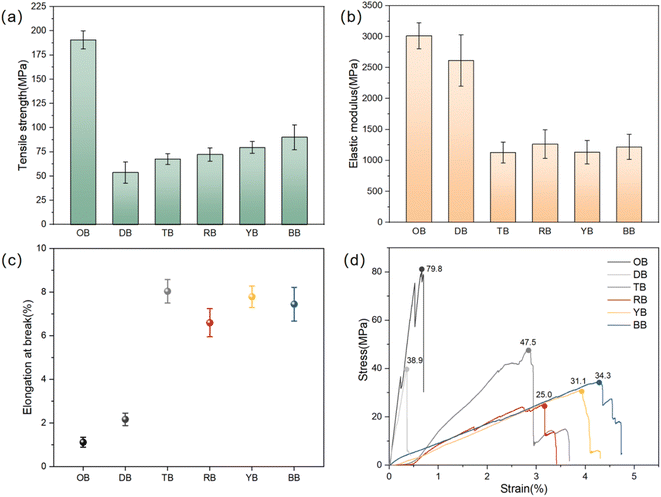 |
| Fig. 6 (a) Tensile strength; (b) tensile strength; (c) elongation at break; (d) stress–strain curve. | |
Color difference analysis
According to the RGB values measured by the colorimeter, the corresponding colors of each group of samples were found in the chromatography. As shown in Fig. 6, the original bamboo sheet was yellow, and the fiber and cell structure of the bamboo were retained after pretreatment with sodium chlorite. The lignin providing the chromophore group was effectively removed, and the bamboo was white. The bamboo textile fibers after dyeing and color matching show rich and bright colors.
In order to characterize the color change of the samples more accurately, the ΔE*, ΔL*, Δa
and Δb* of each dyed sample were calculated by CIE Lab D65 10° standard based on the color indexes of DB.39 According to the diagram, the color deviation (ΔE*) of the dyed samples ranged from 40 to 80. Except for the difference of yellow and green bamboo fiber bundles was 79 and 42, the color difference of the remaining color and gray bamboo fiber bundles was about 60. Compared with the white bamboo fiber bundles without lignin, the brightness of the colored bamboo fiber bundles dyed with reactive dyes showed a downward trend. The brightness value of the yellow bamboo bundles decreased the least, followed by the reactive red, reactive blue and related color bamboo bundles. Fig. 7(c and d) is the offset of dyed bamboo fiber bundles to red-green and yellow-blue directions. The surface colors of red, yellow, orange and purple samples all showed a reddening trend, while green and blue, one of the three primary colors, tended to be green. Although the Δa* of the compound gray was negative, the value was close to zero, which further proved that the three primary color dyes were equally dyed when dyeing bamboo fiber bundles. The Δb* of RB in the yellow-blue color system was less than zero, indicating that the apparent color of the bamboo fiber bundle after reactive red staining was red, but the color light was blue, and ORB and YB showed a yellowing trend in the coordinate system. Blue and other color samples obtained by blue compounding are negative in the coordinate system, that is, they all have a tendency to turn blue. The change of Δb* value of gray is relatively small, so the apparent color of the sample shows a dark gray with a cold tone.
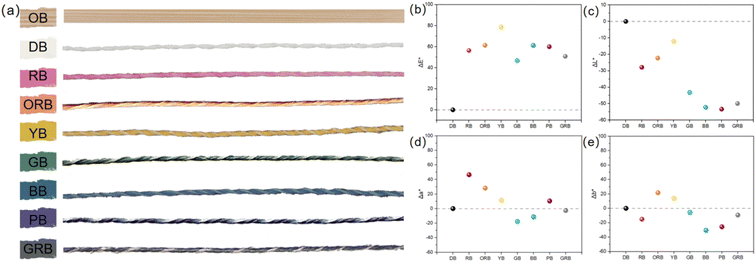 |
| Fig. 7 (a) The apparent color display of each group of samples before and after dyeing treatment; (b–e) the values of ΔE*, ΔL*, Δa* and Δb* of samples. | |
Conclusions
Due to its excellent flexibility and easy availability of raw materials, twisted bamboo fiber bundles can be used as a textile preparation material in the future textile industry. In this study, M-type reactive dyes were used to prepare colored twisted bamboo fiber bundles and their physicochemical properties were analyzed. The following conclusions were drawn:
(1) Through the analysis of the chemical composition and tensile properties of natural bamboo, decolored bamboo bundles and dyed bamboo bundles, it can be seen that the decolorization treatment can remove nearly 83% of lignin in bamboo, and the brittleness of bamboo fiber bundles is greatly reduced. M-type reactive dyes can covalently bond with decolored bamboo fiber bundles and improve the crystallinity of fibers. Combined with twisting treatment, the mechanical properties of bamboo fiber bundles can be improved. Compared with undyed twisted bamboo fiber bundles, the tensile strength of trichromatic twisted bamboo fiber bundles increased by 2.44%–27.57%. The texture is more soft and elastic, which is closer to the requirements of textile industry for flexibility and spinnability of textile fibers.
(2) In the dyeing test of twisted bamboo fiber bundles, the dye uptake of the red, yellow and blue three primary colors combination of M reactive dyes was 29.7%, 32.0% and 29.2%, respectively, showing the same amount of coloring characteristics and excellent color rendering. Compared with natural bamboo and undyed twisted bamboo fiber bundles, RB, YB and BB showed bright colors and shades. The four kinds of colored twisted bamboo fiber bundles prepared by compounding the three dyes in equal proportion also showed obvious color differences in color difference test results and visual effects, indicating that the use of reactive dyes to dye twisted bamboo fiber bundles has great controllability in color.
In a word, the mechanical properties of twisted bamboo fiber bundles dyed with medium-temperature reactive dyes have been significantly improved. The good dyeability, color rendering and color controllability provided more possibilities for their future applications in textile, home, decoration and other fields.
Abbrevations
OB | Original bamboo |
DB | Decolorized bamboo bundle |
TB | Twisted bamboo fiber bundle |
RB | Red twisted bamboo fiber bundle |
YB | Yellow twisted bamboo fiber bundle |
BB | Blue twisted bamboo fiber bundle |
ORB | Orange twisted bamboo fiber bundle |
GB | Green twisted bamboo fiber bundle |
PB | Purple twisted bamboo fiber bundle |
GRB | Grey twisted bamboo fiber bundle |
Author contributions
Conceptualization, S. H., F. Y., and Y. W.; writing-original draft preparation, S. H.; writing-review and editing, S. H., Y. W., and Y. Z.; methodology, S. H. and Y. W.; funding acquisition, Y. W. and F. Y. Notes the authors declare no competing financial interest.
Conflicts of interest
There are no conflicts to declare.
Acknowledgements
The authors gratefully acknowledge the financial support from the project funded by the National Natural Science Foundation of China (32001382 and 32071687).
Notes and references
- T. Rosén, B. S. Hsiao and L. D. Söderberg, Elucidating the Opportunities and Challenges for Nanocellulose Spinning, Adv. Mater., 2021, 33(28), 2001238 CrossRef PubMed.
- B. Pinlova, R. Hufenus and B. Nowack, Systematic study of the presence of microplastic fibers during polyester yarn production, Journal of Cleaner Production, 2022, 363, 132247 CrossRef CAS.
- W. Fan, G. Zhang and X. Zhang, et al., Superior Unidirectional Water Transport and Mechanically Stable 3D Orthogonal Woven Fabric for Human Body Moisture and Thermal Management, Small, 2022, 18(10), 2107150 CrossRef CAS PubMed.
- Z. Jiang, W. Yu and Y. Yu, Chemical composition analysis and surface property characterization of bamboo, J. Northeast For. Univ., 2006,(04), 1–2+6 Search PubMed.
- S. Tanaka, M. Seki and T. Miki, et al., Influence of pulsive pressure waves on liquid penetration into wood in semi-opened container, Journal of Wood Science, 2019, 65(1), 54 CrossRef.
- H. Chen, Z. Wu and B. Fei, Characterization of cross-sectional structure of bamboo fiber cell wall by atomic force microscopy, J. Nanjing For. Univ. (Nat. Sci. Ed.), 2016, 40(02), 139–143 Search PubMed.
- Y. Huang, B. Fei, Y. Yan, G. Wang and R. Zhao, Study on the mechanical properties of single bamboo fiber, China Pulp & Paper, 2009, 28(8), 10–12 Search PubMed.
- J. Yuan, X. Zhang and Y. Yan, Effects of vascular bundle distribution and structure on the macroscopic compressive properties of bamboo, J. Cent. South Univ. For. Technol., 2019, 39(06), 121–127 Search PubMed.
- Y. Wu, J. Wang and Y. Wang, et al., Properties of Multilayer Transparent Bamboo Materials, ACS Omega, 2021, 6(49), 33747–33756 CrossRef CAS PubMed.
- Q. Gao, J. Hu and Y. Huang, et al., Facile preparation of multifunctional bamboo with superhydrophobic, conductive, and flame-retardant properties, Ind. Crops Prod., 2022, 188, 115676 CrossRef CAS.
- O. G. Pandoli, R. J. G. Neto and N. R. Oliveira, et al., Ultra-highly conductive hollow channels guided by a bamboo bio-template for electric and electrochemical devices, J. Mater. Chem. A, 2020, 8(7), 4030–4039 RSC.
- K. Wang, T. Zhang and X. Xiao, et al., Nature-inspired phytic acid-based hybrid complexes for fabricating green and transparent superhydrophobic and anti-mildew coating on bamboo surface, Colloids Surf., A, 2022, 651, 129769 CrossRef CAS.
- Y. Yu, R. Zhu and B. Wu, et al., Fabrication, material properties, and application of bamboo scrimber, Wood Sci. Technol., 2015, 49(1), 83–98 CrossRef CAS.
- G. K. Prasad, R. Guruprasad and T. Senthilkumar, et al., Development of biodegradable tri-blended fabrics with improved moisture management properties using cotton/polylactic-acid/bamboo-viscose fibres, Indian J. Fibre Text. Res., 2022, 47(2), 161–165 CAS.
- A. Basit, W. Latif and S. A. Baig, et al., The Mechanical and Comfort Properties of Sustainable Blended Fabrics of Bamboo With Cotton and Regenerated Fibers, Cloth. Text. Res. J., 2018, 36(4), 267–280 CrossRef.
- C. Wang and Q. Zhang, Study on the physical extraction process and properties of bamboo fiber, J. Tianjin Univ. Technol., 2014, 33(5), 14–17 Search PubMed.
- B. P. Rocky and A. J. Thompson, Production of Natural Bamboo Fiber—2: Assessment and Comparison of Antibacterial Activity, AATCC J. Res., 2019, 6(5), 1–9 CrossRef CAS.
- X. Ye, E. Zhu and D. Wang, et al., Cationic functionalized bamboo fibers with spinnable and antibacterial properties prepared in chlorocholine chloride/urea deep eutectic solvent, Ind. Crops Prod., 2022, 188, 115607 CrossRef CAS.
- M. Yan and H. Cui, Effect of different spinning methods on the linear properties of bamboo fiber blended yarn [ J ], J. Shanghai Text. Technol., 2016, 44(08), 40–42 Search PubMed.
- Y. Wu, Y. Zheng and F. Yang, et al., Preparation process and characterization of mechanical properties of twisted bamboo spun fiber bundles, J. Mater. Res. Technol., 2021, 14, 2131–2139 CrossRef.
- S. Zhang, W. Ma and B. Tang, et al., Innovation and application of dyes with high fixation, Chin. J. Chem. Eng., 2022, 51, 146–152 CrossRef CAS.
- A. Y. L. Tang, C. H. Lee and Y. M. Wang, et al., A study of PEG-based reverse micellar dyeing of cotton fabric: reactive dyes with different reactive groups, Cellulose, 2019, 26(6), 4159–4173 CrossRef CAS.
- Y. Luo, K. Zhou and W. Hu, et al., Application of sodium oxalate as an accelerant in reactive yellow 3RS dyeing, J. Shaoxing Univ., 2015, 35(03), 15–19 Search PubMed.
- J. Wu, Y. Liu and L. Wang, Study on bonding stability of reactive dyes and bamboo fiber, Silk, 2008,(02), 24–25 Search PubMed.
- X. Dong, J. Liu and Y. Bai, et al., Reactive Red 3BS Dyeing of Banana Fiber, Dyeing and Dyeing, 2022, 59(01), 22–25 Search PubMed.
-
(a) X. Zhou, Oxidation of Lignin-Carbohydrate Complex by Laccase/Co(salen) One-Pot Catalysis, Sains Malays., 2022, 51(3), 723–732 CrossRef CAS;
(b) R. Ramful, T. P. M. Sunthar and E. Marin, et al., Investigating the Effect of Smoke Treatment on Hygroscopic Characteristics of Bamboo by FTIR and Raman Spectroscopy, Materials, 2022, 15(4), 1544 CrossRef CAS PubMed.
- A. Singh, A. Ahmed and A. Sharma, et al., Promising photocatalytic degradation of methyl orange dye via sol-gel synthesized Ag–CdS@Pr-TiO2 core/shell nanoparticles, Phys. B, 2021, 616, 413121 CrossRef CAS.
-
(a) A. Ahmed, A. Singh and B. Padha, et al., UV–vis spectroscopic method for detection and removal of heavy metal ions in water using Ag doped ZnO nanoparticles, Chemosphere, 2022, 303, 135208 CrossRef CAS PubMed;
(b) K. Toba, H. Yamamoto and M. Yoshida, Crystallization of cellulose microfibrils in wood cell wall by repeated dry-and-wet treatment, using X-ray diffraction technique, Cellulose, 2013, 20, 633–643 CrossRef CAS.
- R. Ramful, T. P. M. Sunthar and E. Marin, et al., Investigating the Effect of Smoke Treatment on Hygroscopic Characteristics of Bamboo by FTIR and Raman Spectroscopy, Materials, 2022, 15(4), 1544 CrossRef CAS PubMed.
- Y. Li, Q. Fu and S. Yu, et al., Optically Transparent Wood from a Nanoporous Cellulosic Template: Combining Functional and Structural Performance, Biomacromolecules, 2016, 17(4), 1358–1364 CrossRef CAS PubMed.
- H. Yu, C. Gui and Y. Ji, et al., Changes in Chemical and Thermal Properties of Bamboo after Delignification Treatment, Polymers, 2022, 14(13), 2573 CrossRef CAS PubMed.
- Y. Hu, F. Hu and M. Gan, et al., A rapid, green method for the preparation of cellulosic self-reinforcing composites from wood and bamboo pulp, Ind. Crops Prod., 2021, 169, 113658 CrossRef CAS.
- M. R. Rahman, M. Adamu and S. Hamdan, et al., Optimization and characterization of acrylonitrile/MAPE/nano-clay bamboo nanocomposites by response surface methodology, Polym. Bull., 2022, 79(5), 3031–3059 CrossRef CAS.
- K. M. Watling, J. F. Parr, L. Rintoul, C. L. Brownc and L. A. Sullivan, Raman, infrared and XPS study of bamboo phytoliths after chemical digestion, Spectrochim. Acta, Part A, 2011, 80(1), 106–111 CrossRef CAS PubMed.
- J. Gan, et al., UV-Filtering Cellulose Nanocrystal/Carbon Quantum Dot Composite Films for Light Conversion in Glass Windows, ACS Appl. Nano Mater., 2021, 4(11), 12552–12560 CrossRef CAS.
- B. Singh, et al., Electrochemical sensing and photocatalytic degradation of 2,4-dinitrophenol via bismuth (III) oxide nanowires, J. Mol. Struct., 2022, 1255, 132379 CrossRef CAS.
- B. Singh, et al., Effect of Pd concentration on the structural, morphological and photodiode properties of TiO2 nanoparticles, J. Mater. Sci.: Mater. Electron., 2020, 31(1), 65–74 CrossRef CAS.
- P. Mahajan, et al., Synthesis and characterization of ZnO@WO3 core/shell nanoparticles as counter electrode for dye-sensitized solar cell, Surf. Interfaces, 2022, 30, 101920 CrossRef CAS.
- Y. L. A. Tang, C. H. Lee and C. Y. Chan, et al., Alkyl Polyglucoside (APG) Nonionic Surfactant-Based Reverse Micellar Dyeing of Cotton Fabric - A Study of Reactive Dyes with Different Functional Groups, J. Nat. Fibers, 2023, 20(1), 1–22 Search PubMed.
|
This journal is © The Royal Society of Chemistry 2023 |