DOI:
10.1039/D3RA02943C
(Paper)
RSC Adv., 2023,
13, 19477-19484
Antioxidative polyphenols attenuate pyocyanin-induced ROS production in neuronal HT22 cell lines†
Received
3rd May 2023
, Accepted 15th June 2023
First published on 28th June 2023
Abstract
Pyocyanin, a secreted virulence factor, plays an essential role during Pseudomonas aeruginosa infection. Infection of the central nervous system by this bacterium results in high mortality, but the studies on its mechanism are still rather limited. In this study, we first evaluate the neuronal damage caused by pyocyanin exposure in neuronal HT22 cells. Pyocyanin leads to mitochondrial syndrome and antioxidant defense disruption, therefore increasing intercellular reactive oxygen species (ROS) production. Several typical superior antioxidant polyphenols effectively protect against pyocyanin-induced neuronal cell damage. These findings suggest the neuronal protective activity more or less relies on the structure, rather than the residues. Pre-incubation of catechin activates the essential pathway, indicating inverse correlation of ERK and AMPK phosphorylation participates in this process. These data outline a novel strategy to eliminate intracellular generated ROS. The investigated candidates could be potentially used as therapeutic agents against various ROS-related neurological diseases.
1. Introduction
Pseudomonas aeruginosa is a multidrug resistant, Gram-negative pathogen with multiple niches in host bodies that mainly cause respiratory tract disease, urinary system disease, and even neurological disorder.1 P. aeruginosa is used to conquer various antibiotic attacks, classified as intrinsic, acquired and adaptive resistance, which makes it harder to explore therapeutic approaches for clinical infections.2 The main challenge is the batch of virulence factors manufactured, which are secreted by the bacterium, resulting in severe damage to the host. These virulence factors participate in the initial invasion and colorization, leading to either acute or chronic infection. The major pigment pyocyanin as one of the essential virulence factors, causes toxic effects, dysregulated or nonfunctional cell metabolism.3 Pyocyanin is a nitrogen-containing aromatic compound, which can easily permeate the host cell membrane. In addition, pyocyanin generates ROS, aided by its redox-active properties (Fig. 1A).4
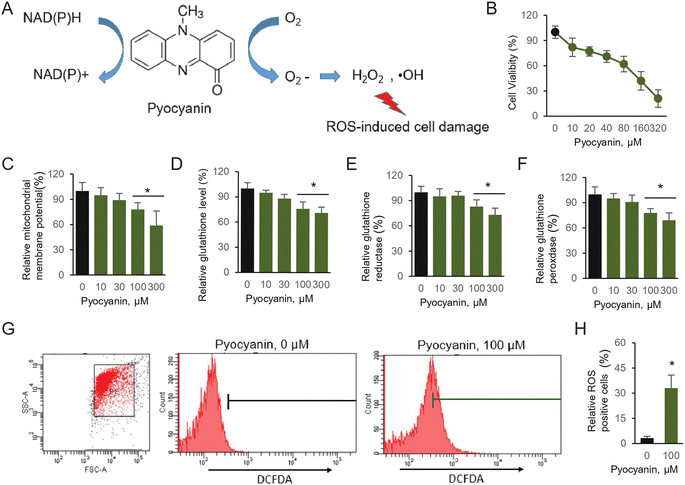 |
| Fig. 1 Pyocyanin-induced neural cell damage. (A) Mechanism of pyocyanin-induced oxidative stress (B) cell viability experiment was performed by CCK-8 kit. (C) Relative mitochondrial membrane potential, (D) intracellular glutathione, (E) glutathione reductase and (F) glutathione peroxidase. (G) The accumulated ROS was determined according to by FACS. (H) Evaluating ROS positive portion. The results are expressed as average ± SD (n = 3). Star marker indicates statistically difference of P < 0.05. | |
ROS are reactive chemical species containing oxygen. The normal metabolism balance of oxygen is essential in cell signaling. However, accumulated ROS production, caused by external chemicals or environmental stress, generates severe disruption of cell function. In addition, oxidative stress is a leading factor related with neurodegenerative disorders, which are clue of various neurological disease.5 The natural source polyphenols have been largely studied due to their antioxidants property, therefore further potentially utilized for therapeutic purpose against various neurodegenerative diseases.6 We have previously selected typical antioxidant polyphenols and proven their essential effect on excessive glutamate-induced abnormal cell physiology. The investigated compounds were mild cytotoxic, but behave effective on various radicals substrates owing to the antioxidative activity.7
It has been investigated earlier that the secreted virulence factors by P. aeruginosa participate central nervous system (CNS) infection, results in high mortality.8 Nevertheless, Pyocyanin-induced neuronal cytotoxicity is seldom studied. In addition, the CNS is highly susceptible to oxidative stress. Excess amount of ROS can damage neuronal cells and further lead to neurodegenerative issue.6a,9 The over-generated ROS is usually through inhibition of glutathione synthesis, which presents by two different states. One state is reduced glutathione (GSH) and the other one is oxidized glutathione (GSSG). The ratio between the two states of glutathione indicates cellular oxidative stress.10
The cellular oxidative stress generated by ROS can trigger various signaling pathways. The most essential protein among those pathway, which response for the accumulation of intercellular ROS, is adenosine monophosphate-activated protein kinase (AMPK).11 Three additional well-characterized mitogen-activated protein kinases (MAPK) also participated in vast array of ROS mediated physiological processes. The c-Jun amino-terminal kinase (JNK), extracellular signal-regulated kinase (ERK), and p38 are crucial transcript regulator and environmental stresses sensor. Those enzymes are regulated through characteristic phosphorelay system.12 Intense efforts have been done to illustrate the modulation during ROS production. During the pyocyanin stimulation in neural cell, how these components involved and activated are poorly understood. To this end, we set up a pyocyanin-induced neuronal cells model, exploring mouse hippocampal neuronal cell line HT22. The emergence of potential ROS damage have been investigated. The investigated natural derivate compounds, which have been studied before, potentially eliminate oxidative stress. Therefore, they are selected here to pursue research related to pyocyanin-induced cell damage.
In current study, our work explored attenuated effect towards pyocyanin-induced neurotoxicity. In current HT22 cell model, pyocyanin contributes excitotoxicity by decreasing the cell viability. Meanwhile, pyocyanin induced ROS production directly, associated with mitochondrial signal dysregulation and intracellular antioxidant system depletion. We take advantage of previous studied antioxidative compounds and utilize them to perform protective analysis.13 The mitochondrial function take effect abnormally by pyocyanin incubation, while pre-incubated with antioxidants extensively attenuated pyocyanin-induced cell dysfunction. Notably, catechin, one of the representative compound, triggers essential signal pathway. The data suggest that correlation of ERK and AMPK phosphorylation is involved in catechin protected neuronal damage. The results imply the protective effect mainly due to both chemical residues and structure. Those studies enlighten the application of natural anti-oxidative molecules against neurological issue.
2. Materials and methods
2.1. Materials and regents
HT-22, the immortalized mouse hippocampal cells is a lab stock. High glucose DMEM medium and Fetal Bovine Serum (FBS) are products from ATCC, USA. Cell Counting Assay Kit-8 (CCK-8) was purchased from Gold Biotechnology, China, which was used for cell viability assay. The fluorescence dye, including 2,7-dichlorofluorescein diacetate (DCFDA), rhodamine 123 (Rho123) and 5′,5′-dithiobis 2-nitrobenzoic acid (DTNB) were stock of Molecular Probe or Invitrogen, USA. Pyocyanin and antioxidative chemical polyphenols were all originally from Sigma or Fluka, USA. Antibodies used in this study came from Santa Cruz Biotechnology or Cell signal Technology. The other solutions are all analytical grade.
2.2. Cell viability assay
The mouse HT22 cells were maintained in DMEM medium supplemented to 10% FBS (v/v), with double antibiotics of penicillin at 100 units per mL and streptomycin at 100 μg ml−1. Unless indicated, the cells were cultured at 37 °C with 5% CO2. Once it is needed, they were passaged every three days at about 95% confluence by trypsinization. Unless otherwise indicated, the antioxidative compounds used in this study (Fig. 2) were added 12 hours prior to pyocyanin in all experiments.
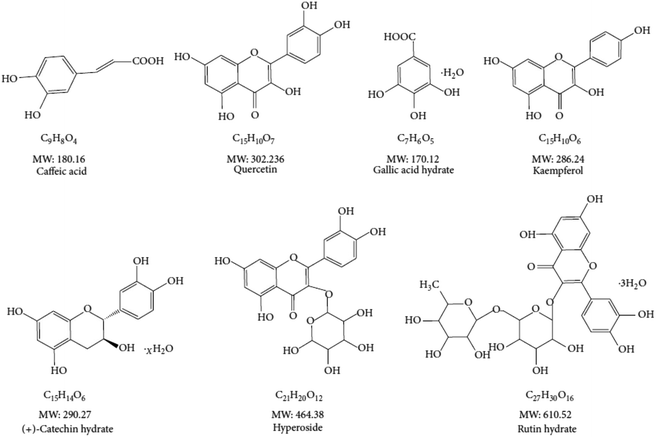 |
| Fig. 2 Chemical structure on selected antioxidant compounds. | |
The cell proliferation and cytotoxicity were determined by cell viability assay using CCK-8 kit, followed by the manufacturer's instruction. HT22 cells were seeded to 96-well flat bottom plate with 10
000 cells per well at initial volume 100 μl. Then, they were incubated for 24 hours. Pyocyanin with initial concentration at 320 μM was added into the medium and it is further diluted by two fold serial dilution and incubated for 12 hours. The wells without adding pyocyanin were set up as a positive control. Finally, 10 μl CCK-8 stock solution provided by the kit was added into each well, and mixed with medium by general shaking. The mixture was keep in the incubator for another 3 hours. The absorbance at a wavelength of 450 nm was determined. The result was expressed by OD450 relative to the control group.
2.3. Pyocyanin-induced HT22 cell damage
The mitochondrial membrane potential (ΔΨ) is frequently monitored by fluorescent probes rhodamine 123 (Rho123). It is employed to assess mitochondrial function, particularly in those neuronal cells involved in cell damage.14 HT22 cells were seeded into 96-well plates for 24 hours. Pyocyanin at indicated concentrations was incubated with cells for additional 12 hours. After incubation, the fluorescent dye, Rho123 at final concentration of 10 μg ml−1 was supplemented in the medium for 15 min at 37 °C and used to quantify mitochondrial membrane potential. After incubation, brief wash cells to remove excess dye. The Rho123 concentration was quantified by the intensity with excitation and emission at 488 nm and 520 nm, accordingly. The result was finally present by relative intensity compared to control group.
The glutathione, glutathione-dependent enzymes, including glutathione reductase and peroxidase were determined.15 HT22 cells pre-incubated at indicated concentration of pyocyanin for 12 hours were lysed with sulfosalicylic acid. Spin down cell lysate at 4 K rpm at 4 °C for 30 min, then supernatant was employed to set up the reaction, including glutathione disulfide reductase, NADPH and DTNB at final 5 units per mL, 0.3 mM and 0.5 mM, respectively. The wavelength at 412 nm was determined. The relative glutathione reductase was quantified according to the reduced oxidized glutathione when NADPH is supplied. Further, the activity of glutathione peroxidase was determined from the rate, between GSH and GSSG, which came from the absorbance at the wavelength 340 nm.
2.4. Pyocyanin-induced intercellular ROS production
The intercellular ROS production was quantified using fluorescence dye, 2,7-dichlorofluorescein diacetate (DCFDA) and performed by flow cytometry analysis.16 Transfer 4 × 105 per well HT22 cells into a 6-well plate and incubate for 24 hours. Then, gently add pyocyanin for another 12 hours. After incubation, the cells were digested by trypsin and resuspended at final concentration 1 μM DCFDA for 30 minutes in dark. BD Accuri™ C6 Plus flow cytometry was employed and at least 10
000 cells were analyzed in individual samples.
2.5. Western blotting assay
The method used for performing western blotting assay was described as before with minus modifications.17 The HT22 cells were scraped from culture dishes, and lysed in the buffer as described above. Whole cell lysate was clarified at 12 K rpm centrifugation at 4 °C for 25 minutes. The protein samples were loaded to 12% SDS-PAGE gel. The nitrocellulose membrane was used for transferring proteins and blocked with 5% nonfat milk for at least 1 hour, followed by incubating with individual primary antibodies at 4 °C overnight with general shaking. After a brief wash, the membrane was subjected to incubation with relative peroxidase-conjugated secondary antibodies. After incubation for 1 hour, and washing by TBST buffer, the bands were revealed in films according to ECL system (Amersham Pharmacia Biotech). The western blot band intensity was quantified by ImageJ software.18
2.6. Statistical analysis
The Student's t-test and the ANOVA-Tukey test were utilized here for statistical analysis, and evaluated by SPSS (IBM Corp., version 21.0, USA). Experiments, including flow cytometry assay and western blotting analysis, were repeated twice. All the other experiments were carried out by a minimum of three repeats. The data was expressed as mean ± standard deviation (SD). Where P < 0.05 was used to evaluate significant.
3. Results
3.1. Pyocyanin-induced HT22 cell defect and intracellular ROS generation
Pyocyanin, the major toxins secreted by thegram negative bacterium Pseudomonas aeruginosa, is responsible for severe infections in various systems, including central nervous system (CNS).19 However, the detailed evidence on pyocyanin-induced neuronal cell defect is rather limited. First of all, we utilize HT22 cells, establish a neuronal cell model to illustrate the potential damage caused by pyocyanin. It is a reversible redox-active pigment product at least partially associated with oxidative stress and accumulated intracellular ROS, mainly caused by hydrogen peroxide (H2O2) and superoxide (O2˙−) (Fig. 1A). Under aerobic conditions, those ROS products are generated from electrons donor.20
We first perform assay of pyocyanin on cytotoxicity. The CCK-8 kit will generate yellow dye in living cells, which is proportional to the amount of normal cells. The data suggest the HT22 cell viability significantly decreased to pyocyanin (Fig. 1B). Its viability is roughly half amount once 100 μM pyocyanin was incubated with HT22 cells for 12 hours. Next, we estimate the potential cell defect followed by pyocyanin exposure. When cells are supplemented with 100 and 300 μM pyocyanin, mitochondrial membrane potential dropped below normal levels. It is 78.3% and 59.1% respectively, compared with control group (Fig. 1C).
Due to the redox-active properties of pyocyanin, we next determine its exposure caused free radical damage as well as the oxidative enzyme targets inside the cells. The glutathione, GSH and GSSG activity were evaluated (Fig. 1D–F). Indeed, glutathione is proved to serve as an important antioxidant modulated by pyocyanin. Its relative level decreased, probably because of intermediate ROS products generation, such as hydrogen peroxide and superoxide, which cause oxidative stress. Therefore, the redox-active machinery enzymes were quantified. The activity of both glutathione reductase and glutathione peroxidase were affected by pyocyanin in HT22 cells. Finally, the relative ROS production induced by pyocyanin was quantified by flow cytometry using the fluorescence dye, DCFDA (Fig. 1G and 1H). Incubation with 100 μM pyocyanin, result in 33.3% ROS positive cells. Taken together, these results indicated pyocyanin exposure in HT22 neuronal cells, lead to ROS production directly, and further caused mitochondrial signal dysfunction and antioxidant system depletion inside cells.
3.2. Property of antioxidant polyphenols
The excess amount of ROS, either originate from intercellular oxygen or induced by exogenous chemicals, have a fatal effect on cytotoxicity and organism dysfunction. Previously, we have performed assays to prove the potential antioxidant activity employing chemicals with distinct size of molecular weight.7 The chemical structure of interested polyphenols are listed (Fig. 2). The peroxyl and hydroxyl radicals antioxidant capacity were investigated, with which those free radicals are directly correlated with oxidative damage. We found almost all investigated compounds exhibit more or less antioxidant ability on peroxyl and hydroxyl radicals, as well as the positive control trolox used in the assay. The half maximal inhibitory concentration (IC50) rates range from 2 μg ml−1 to 6 μg ml−1 in ABTS and DPPH assay.13 Notably, caffeic acid was the weakest one among the antioxidants to eliminate free radicals. In addition, the small size antioxidants investigated had lower IC50 in ROS scavenging analysis, indicating their essential function of high content of polyphenols. On the other hand, the effect on HT22 cell viability of the antioxidants was rather mild, no significant affection was found when HT22 cells was incubated with any of the antioxidants in 64 μg ml−1.7 The antiradical ability of compounds were varies, and depended on methods and environment conditions, however all of them were low cytotoxicity to neuronal cells, and therefore were used to characterize pyocyanin-induced cell damage.
3.3. Attenuated pyocyanin-induced HT22 cell dysfunction
The relationship between pyocyanin and mitochondrial membrane potential, as well as the redox-active properties of key electrons transfer enzymes, have strongly indicated the dysfunction induced by intercellular ROS. To this end, we hypothesize that the free radicals scavenging by antioxidants could contribute the attenuation of pyocyanin-induce neuronal cell dysfunction. Individual antioxidative compound used in this study (Fig. 2) was added 12 hours prior to pyocyanin, and then co-incubated with pyocyanin for additional 12 hours, where the pyocyanin incubation only cells was selected as mock group and normal culture HT22 cells were used as control. Most of the antioxidants restore mitochondrial membrane potential, except caffeic acid and gallic acid (Fig. 3A). The affection of pyocyanin on glutathione level, glutathione reductase and peroxidase could also be partially abolished by adding particular antioxidants (Fig. 3B–D). The neuronal protective ability of antioxidants on pyocyanin-induced HT22 cell damage was further quantified to compare the individual potential (Fig. 3E). The mitochondrial membrane potential, glutathione, GSH and GSSG results were compared. The data was generated by using increased percentage of antioxidant group to mock group, relative to control group. The bins of 25%, 50% and 75% were selected by employing green, blue, dark red and red color. It is obvious that caffeic acid is the weakest one to restore pyocyanin-induced cell dysfunction. Gallic acid had mild ability to protect the cell damage. Other than those, all the rest antioxidants restore more than 50% or even 75% of the mitochondrial membrane potential, glutathione level and glutathione peroxidase. Notably, the glutathione reductase level was hardly rescued. These results suggested pre-incubated with antioxidants attenuated pyocyanin-induced HT22 cell dysfunction.
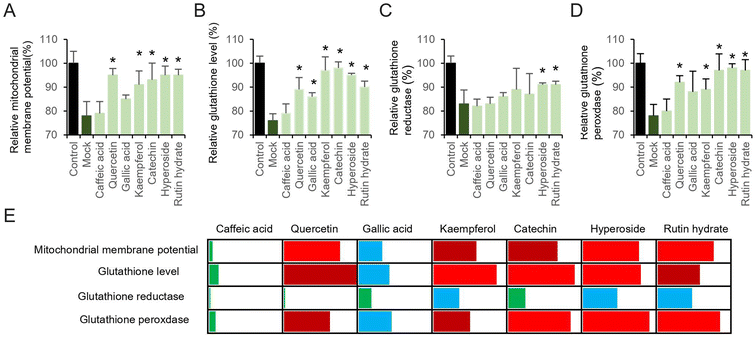 |
| Fig. 3 Antioxidant compounds attenuated pyocyanin-induced ROS production and cell dysfunction. (A) Relative mitochondrial membrane potential. (B) Glutathione, (C) glutathione reductase and (D) glutathione peroxidase amount. (E) Potential antioxidant comparison was analyzed by heat map. The relative increased percentage of data was used. The bins of 25%, 50% and 75% were selected by employing green, blue, dark red and red color. | |
3.4. Protection of catechin on pyocyanin-induced cell damage
It has been recognized that excess intercellular ROS potentially regulates signaling pathway, which subsequently mediates neurodegeneration and neuroprotection.21 Although numerous investigation have demonstrated the alternation of signaling pathways response to accumulated intracellular ROS, their functions and interactions remain unclear. Here, we focus two families of signing proteins. The first essential one is AMPK, which is involved into multiple metabolic process. The other group consists of ERK, JNK, and p38. All those regulator proteins belong to serine/threonine kinases, response by cellular stimuli.22 Since the protective effect of investigated antioxidants are similar, judged by the restore ability of cell dysfunction (Fig. 3E), a representative compound, catechin was selected to determine the mechanism of cell dysfunction attenuation. Here, we performed the western blotting assay, when pyocyanin was supplemented to HT22 cell, immediate response of phosphorylation on AMPK and ERK was appear after 0.5 and 3 hours (Fig. 4A–C), whereas longer incubation to 12 hours of pyocyanin did not active either proteins. Moreover, no significant change of phosphorylation of JNK and p38 were found (Fig. 4A, D and 4E). Next, the potential function of catechin on investigated signaling proteins was addressed. The HT22 cells were pre-incubated with catechin for 12 hours and then pyocyanin was added for additional 3 hours. Interestingly, catechin partially restored the AMPK and ERK phosphorylation to control level (Fig. 4F–H). At last, the relative ROS positive cells were also declined when catechin was used (Fig. 4I). Notably, U0126, the specific ERK pathway inhibitor, was also functional to attenuate pyocyanin-induced cell dysfunction. The data indicated an inverse correlation of ERK and AMPK phosphorylation was participated in catechin protected cell damage (Fig. 4B and F). The overall outline of the mechanism is shown as in Fig. 5.
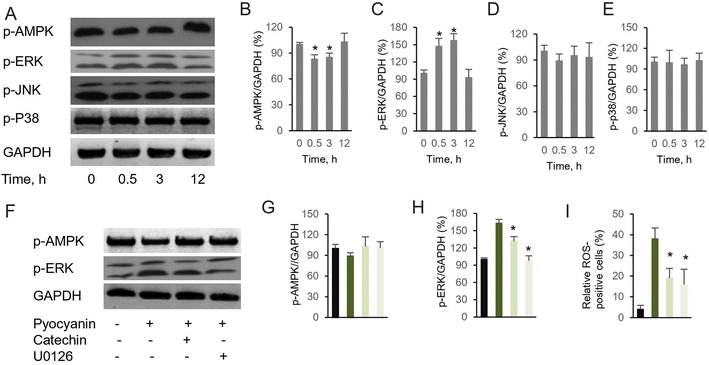 |
| Fig. 4 Pyocyanin-induced neuronal dysfunction activates AMPK and ERK pathway. (A) Western blot assay of essential kinases by their phosphorylation. Relative band intensity assay between phosphorylated (B) AMPK, (C) ERK, (D) JNK, (E) p38 and internal control. (F) Pre-incubation with catechin or U0126, the ERK specific inhibitor, partially abolish pyocyanin-induced AMPK and ERK phosphorylation. Relative band intensity assay between (G) p-AMPK, (H) p-ERK and internal control. (I) Pre-incubation with catechin or U0126 attenuated pyocyanin-induced ROS positive cells (n = 10 000 cells). The results are expressed as average ± SD (at least n = 3). Star marker indicates statistically difference of P < 0.05. | |
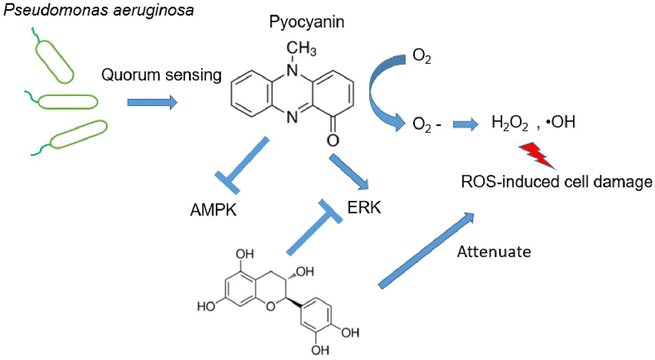 |
| Fig. 5 Catechin protective against pyocyanin-induced ROS production. | |
4. Discussion
Pyocyanin, an essential virulence factor, is easy to permeate cell membranes. Previous research work indicated its potential function during pseudomonas aeruginosa infection and its toxic effect.23 The most widely investigated pathogenicity of this bacterium is in respiratory models, only little is known about this virulence factor in the CNS.
In the current study, we provided in vitro evidence of pyocyanin toxicity in HT22 cells. As the key virulence factor, it was thus an inevitable phenomenon that the cell viability is strongly affected when HT22 cells were incubated with pyocyanin (Fig. 1B). In addition, the mitochondrial physiology was dominated in the redox environment, whereas the mitochondrial membrane potential declined (Fig. 1C). The glutathione and the detoxifying enzymes locating inside mitochondria emerged as the essential defense for normal functional maintenance to avoid mitochondrial dysfunction (Fig. 1D–F). Generation of mitochondrial ROS closed tied mitochondrial membrane abnormal function during the process of pyocyanin incubation (Fig. 1G and H). Taken together, we proved that the oxidative stress and its regulatory enzymes were involved in neuronal cell damage.
We have previously investigated the anti-oxidant effect of multiple natural source compounds (Fig. 2), which exhibit free radical scavenging activity, including ROS and RNS. The elimination on peroxyl and hydroxyl radical results indicated universal acticity of investigated candidates.7 Those compounds abolish pyocyanin-induced ROS production, through regulation of the internal mitochondrial membrane potential, glutathione level and key enzymes associated in this process (Fig. 3). Those cellular components are functional against ROS. The crucial energy sensor and regulator AMPK and ERK were identified in those process, whereas JNK and p-38 were mildly affected.
The common feature of those investigated compounds are polyphenols. It is interesting that those with larger molecular weight, including hyperoside and rutin hydrate regulate all tested glutathione enzymes (Fig. 3B and C). These larger compounds usually present in plants, especially citrus fruit. It is possible these compounds are responsible for mediating ROS-induced signaling cascades due to the significant permeation and bioavailability in our model. These data confirm our previous research work on free radical ROS scavenging activity in ABTS and DPPH assay and in various neuronal cells.7
On the other hand, our mechanism assay of essential pathways suggested protective role of selective compound for pyocyanin-induced toxicity (Fig. 4). It is not surprising since previously observed protective role of AMPK and ERK in development of neurodegenerative diseases.24 Indeed, phosphorylation of AMPK and ERK was regulated mildly at initial incubation process upto 3 hours (Fig. 4B and C), whereas the phosphorylation level of JNK and p-38 was almost identical with or without pyocyanin incubation (Fig. 4D and E). Furthermore, imbalance and loss of function of anti-oxidant defense has been rescued through glutathione participation at 12 hours. In contract, low glutathione is usually observed in negative ROS balance. It is hypothesized to associate with higher glycolytic activity, which result from reduced levels of oxidative phosphorylation.25 Notably, we select one typical compound catechin, which restored pyocyanin induced cell signaling phosphorylation and intercellular ROS production (Fig. 4F–I). These interesting findings demonstrated our investigated compounds are capable for helping regenerate glutathione levels. In all, the study suggest that correlation of ERK and AMPK phosphorylation is involved in catechin protected neuronal damage. The mechanism phenotypic assay indicated its association with mitochondrial membrane potential, as well as regulatory enzymes. Finally, the protection of antioxidant indeed rescue the ROS production which is potential damage to neural dysfunction, even cell death (Fig. 5).
It is now widely accepted that natural source polyphenols could be used for the prevention and treatment of disease. Potential mechanisms involved antioxidant, anti-inflammation, in which modulation of multiple signal events involved.26 We provide novel evidence that these compounds could potentially be used as mediators in neuronal cell damage. Its neuroprotective activity towards pyocyanin-induced cell dysfunction, as well as the subsequent effect on phosphorylation of AMPK and ERK further proved the attenuation of oxidative stress involves complex components.
5. Conclusion
To summary, the preliminary study provides the novel evidence on protecting effect of natural source polyphenols toward pyocyanin-induced neuronal cell damage. Pyocyanin cytotoxicity is associated to intracellular oxidative stress, as well as glutathione level from HT22 cells. Cell dysfunction suggested to subsequent neuronal degeneration. The screened candidates exhibit more or less protective effect, and abolish the ROS production through glutathione and its relative enzymes. The typical compounds catechin further suggested an opposite correlation between AMPK and ERK activation. These novel findings in this study warrant further investigation, provide clues on pyocyanin-induced CNS degradation disease. Those potential candidates are worth exploring further.
Data availability
Experimental data are available in the ESI.†
Contributions statement
HL. X. and Y. N. design the study, HL. X., Q. Y. and X. Z. performed cell viability, flow cytometry analysis, HL. X., performed western blot analysis, all author contributed to analyze the results. HL. X. wrote the initial manuscript, and all other authors helped draft the manuscript, and gave final approval for it.
Conflicts of interest
No conflicts of interest.
References
-
(a) A. Rodrigo-Troyano, V. Melo, P. J. Marcos, E. Laserna, M. Peiro, G. Suarez-Cuartin, L. Perea, A. Feliu, V. Plaza, P. Faverio, M. I. Restrepo, A. Anzueto and O. Sibila, Respiration, 2018, 96, 417–424 CrossRef CAS PubMed;
(b) V. M. Paz-Zarza, S. Mangwani-Mordani, A. Martinez-Maldonado, D. Alvarez-Hernandez, S. G. Solano-Galvez and R. Vazquez-Lopez, Rev. Chilena. Infectol., 2019, 36, 180–189 CrossRef PubMed;
(c) M. D. Baldissera, C. F. Souza, R. C. V. Santos and B. Baldisserotto, J. Fish Dis., 2018, 41, 209–213 CrossRef CAS PubMed.
-
(a) C. Gudiol, A. Albasanz-Puig, J. Laporte-Amargos, N. Pallares, A. Mussetti, I. Ruiz-Camps, P. Puerta-Alcalde, E. Abdala, C. Oltolini, M. Akova, M. Montejo, M. Mikulska, P. Martin-Davila, F. Herrera, O. Gasch, L. Drgona, H. Paz Morales, A. S. Brunel, E. Garcia, B. Isler, W. V. Kern, I. Morales, G. Maestro-de la Calle, M. Montero, S. S. Kanj, O. R. Sipahi, S. Calik, I. Marquez-Gomez, J. I. Marin, M. Z. R. Gomes, P. Hemmatti, R. Araos, M. Peghin, J. L. Del Pozo, L. Yanez, R. Tilley, A. Manzur, A. Novo, J. Carratala and I. S. Group, Antimicrob. Agents Chemother., 2020, 64, e02494 CAS;
(b) S. R. Coleman, T. Blimkie, R. Falsafi and R. E. W. Hancock, Antimicrob. Agents Chemother., 2020, 64, e01999 CrossRef CAS PubMed.
- B. Rada and T. L. Leto, Trends Microbiol., 2013, 21, 73–81 CrossRef CAS PubMed.
- L. A. Meirelles and D. K. Newman, Mol. Microbiol., 2018, 110, 995–1010 CrossRef CAS PubMed.
- J. M. Zolezzi, C. Silva-Alvarez, D. Ordenes, J. A. Godoy, F. J. Carvajal, M. J. Santos and N. C. Inestrosa, PLoS One, 2013, 8, e64019 CrossRef CAS PubMed.
-
(a) C. Li, S. Chai, Y. Ju, L. Hou, H. Zhao, W. Ma, T. Li, J. Sheng and W. Shi, Mol. Neurobiol., 2017, 54, 5286–5299 CrossRef CAS PubMed;
(b) S. N. A. Bukhari, Antioxidants, 2022, 11, antiox11030554 CrossRef PubMed.
- H. Xin, Y. Cui, Z. An, Q. Yang, X. Zou and N. Yu, RSC Adv., 2019, 9, 34735–34743 RSC.
- B. L. Wise, J. L. Mathis and E. Jawetz, J. Neurosurg., 1969, 31, 432–434 CAS.
- Z. Liu, T. Zhou, A. C. Ziegler, P. Dimitrion and L. Zuo, Oxid. Med. Cell. Longevity, 2017, 2017, 2525967 Search PubMed.
-
(a) D. Colle, D. B. Santos, J. M. Hartwig, M. Godoi, D. F. Engel, A. F. de Bem, A. L. Braga and M. Farina, Mol. Neurobiol., 2016, 53, 1280–1295 CrossRef CAS PubMed;
(b) S. C. Lu, Biochim. Biophys. Acta, 2013, 1830, 3143–3153 CrossRef CAS PubMed.
- A. Soltani, A. Salmaninejad, M. Jalili-Nik, A. Soleimani, H. Javid, S. I. Hashemy and A. Sahebkar, J. Cell. Physiol., 2019, 234, 2241–2251 CrossRef CAS PubMed.
-
(a) Y. L. Jia, X. J. Liu, H. Wen, Y. P. Zhan and M. H. Xiang, Int. J. Ophthalmol., 2019, 12, 1801–1806 CrossRef PubMed;
(b) X. W. Zhao, J. P. Zhou, Y. L. Bi, J. Y. Wang, R. Yu, C. Deng, W. K. Wang, X. Z. Li, R. Huang, J. Zhang and D. T. Tao, Mol. Biol. Rep., 2019, 46, 3149–3156 CrossRef CAS PubMed.
- H. Xin, Y. Cui, Z. An, Q. Yang, X. Zou and N. Yu, RSC Adv., 2019, 9, 34735–34743 RSC.
-
(a) S. W. Perry, J. P. Norman, J. Barbieri, E. B. Brown and H. A. Gelbard, Biotechniques, 2011, 50, 98–115 CrossRef CAS PubMed;
(b) A. Baracca, G. Sgarbi, G. Solaini and G. Lenaz, Biochim. Biophys. Acta, 2003, 1606, 137–146 CrossRef CAS PubMed.
-
(a) T. E. Tipple and L. K. Rogers, Methods Mol. Biol., 2012, 889, 315–324 CrossRef CAS PubMed;
(b) E. Kozer, S. Evans, J. Barr, R. Greenberg, I. Soriano, M. Bulkowstein, I. Petrov, Z. Chen-Levi, B. Barzilay and M. Berkovitch, Br. J. Clin. Pharmacol., 2003, 55, 234–240 CrossRef CAS PubMed.
- E. Eruslanov and S. Kusmartsev, Methods Mol. Biol., 2010, 594, 57–72 CrossRef CAS PubMed.
- M. Zhang, H. Zhao, X. Z. Shi, J. Wu, Q. Fu and W. Shi, Clin. Lab., 2014, 60, 1–8 CAS.
- C. A. Schneider, W. S. Rasband and K. W. Eliceiri, Nat. Methods, 2012, 9, 671–675 CrossRef CAS PubMed.
- Y. C. Chuang, W. N. Chang, C. H. Lu, H. S. Wu and H. W. Chang, Zhonghua Yufang Yixue Zazhi, 1999, 62, 300–307 CAS.
- S. Hall, C. McDermott, S. Anoopkumar-Dukie, A. J. McFarland, A. Forbes, A. V. Perkins, A. K. Davey, R. Chess-Williams, M. J. Kiefel, D. Arora and G. D. Grant, Toxins, 2016, 8, toxins8080236 CrossRef PubMed.
- J. Zhang, X. Wang, V. Vikash, Q. Ye, D. Wu, Y. Liu and W. Dong, Oxid. Med. Cell. Longevity, 2016, 2016, 4350965 Search PubMed.
-
(a) J. Liu, Y. Liu, J. Chen, C. Hu, M. Teng, K. Jiao, Z. Shen, D. Zhu, J. Yue, Z. Li and Y. Li, Toxicol. In Vitro, 2017, 45, 10–18 CrossRef PubMed;
(b) D. Xie, X. Wu, L. Lan, F. Shangguan, X. Lin, F. Chen, S. Xu, Y. Zhang, Z. Chen, K. Huang, R. Wang, L. Wang, X. Song, Y. Liu and B. Lu, Oncotarget, 2016, 7, 11609–11624 CrossRef PubMed.
-
(a) C. McDermott, R. Chess-Williams, K. A. Mills, S. H. Kang, S. E. Farr, G. D. Grant, A. V. Perkins, A. K. Davey and S. Anoopkumar-Dukie, Toxicol. In Vitro, 2013, 27, 1693–1698 CrossRef CAS PubMed;
(b) K. M. Smith, Y. Bu and H. Suga, Chem. Biol., 2003, 10, 81–89 CrossRef CAS PubMed.
-
(a) S. Z. A. Shah, D. Zhao, T. Hussain and L. Yang, Ageing Res. Rev., 2017, 40, 51–63 CrossRef CAS PubMed;
(b) L. Colucci-D'Amato, C. Perrone-Capano and U. di Porzio, Bioessays, 2003, 25, 1085–1095 CrossRef PubMed.
- W. Droge and E. Holm, FASEB J., 1997, 11, 1077–1089 CrossRef CAS PubMed.
-
(a) M. A. Moga, O. G. Dimienescu, C. A. Arvatescu, A. Mironescu, L. Dracea and L. Ples, Molecules, 2016, 21, molecules21081055 CrossRef PubMed;
(b) G. Bureau, F. Longpre and M. G. Martinoli, J. Neurosci. Res., 2008, 86, 403–410 CrossRef CAS PubMed.
|
This journal is © The Royal Society of Chemistry 2023 |
Click here to see how this site uses Cookies. View our privacy policy here.