DOI:
10.1039/D3RA01999C
(Review Article)
RSC Adv., 2023,
13, 19335-19355
Natural-based coagulants/flocculants as sustainable market-valued products for industrial wastewater treatment: a review of recent developments
Received
27th March 2023
, Accepted 13th June 2023
First published on 26th June 2023
Abstract
Industrial wastewater is categorized as a voracious consumer of fresh water and a high-strength source of pollution. Coagulation–flocculation is a simple and cost-effective technique for removing organic/inorganic compounds and colloidal particles from industrial effluents. Despite the outstanding natural properties, biodegradability, and efficacy of natural coagulants/flocculants (NC/Fs) in industrial wastewater treatment, their significant potential to remediate such effluents is underappreciated, particularly in commercial scale applications. Most reviews on NC/Fs focused on the possible application of plant-based sources such as plant seeds, tannin, certain vegetables/fruit peels, and their lab-scale potential. Our review expands the scope by examining the feasibility of using natural materials from other sources for industrial effluent decontamination. By analyzing the latest data on NC/Fs, we identify the most promising preparation techniques for making these materials stable enough to compete with traditional options in the marketplace. An interesting presentation of the results of various recent studies has also been highlighted and discussed. Additionally, we highlight the recent success of using magnetic-natural coagulants/flocculants (M-NC/Fs) in treating diverse industrial effluents, and discuss the potential for reprocessing spent materials as a renewable resource. The review also offers different concepts for suggested large-scale treatment systems used by MN-CFs.
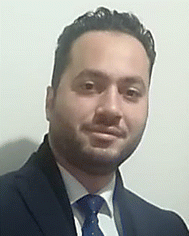 Ahmad K. Badawi | Dr Ahmad K. Badawi is a highly accomplished assistant professor of Environmental Engineering, renowned for his remarkable expertise in the field of wastewater treatment, particularly in addressing the challenges associated with municipal and industrial wastewater. Dr Badawi holds both MSc and PhD degrees in Environmental Engineering, and his exceptional achievements saw him appointed as a university professor at the remarkable age of 29. Throughout his career, Dr Badawi has dedicated his research and professional efforts to developing innovative approaches that significantly enhance the efficiency and sustainability of wastewater treatment processes. He combines various advanced treatment methods, including photocatalysis, adsorption filtration, biological processes, and chemical coagulation, in his quest for achieving optimal efficiency, cost-effectiveness, and environmental sustainability. Dr Badawi's research is highly regarded in the field, with a particular emphasis on the use of algal–bacterial photo-bioreactors for large-scale wastewater treatment. He has also conducted groundbreaking investigations into nano-sized advanced/hybrid materials for industrial wastewater treatment. Dr Badawi is a leading specialist in pilot plant design, fabrication, modelling, and implementation. He has designed and constructed several pilot plants across different WWTPs and factories, showcasing his expertise in the field. Dr Badawi is a prolific author, with numerous publications distributed between high-impact journals (Q1 & Q2) and international conferences. He is also an acting editor and reviewer at several reputable international journals, having edited and reviewed over 160 manuscripts. His expertise in the field has been acknowledged by several international journals, where he has been recognized as an editor/reviewer. Dr Badawi has also served as an acting PI/co-PI for many international and national funded grants, further highlighting his impact and leadership in the field. |
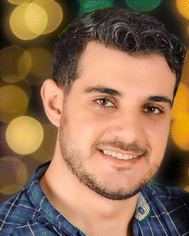 Reda S. Salama | Reda S. Salama is currently a full assistant professor at the Faculty of Engineering – Delta University for Science and Technology, Egypt. He received his BSc degree from Mansoura University, Egypt in 2010, MSc degree from Mansoura University in 2013 and his PhD also from Mansoura University in 2019. His research interests include: (i) synthesis and characterization of carbon-based materials for adsorption and catalysis; (ii) synthesis and applications of MOFs; (iii) water purification by advanced oxidation processes, especially photocatalysis; (iv) carbon monoxide oxidation and (v) preparation and characterization of metal-based materials from wastes for energy storage supercapacitors. He has presented over 30 studies to national and international journals and conferences. |
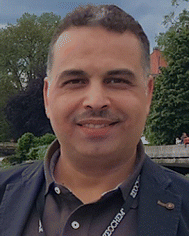 Mohamed Mokhtar M. Mostafa | Prof. Dr Mohamed Mokhtar M. Mostafa received his PhD from Cairo University, Cairo, Egypt in 1997. He held the position of Senior Research Fellow of DAAD, at the Institute of Chemical Engineering and Processing, Karlsruhe University, Germany in 1998–1999. He was invited as a Research Fellow to join the Surface Chemistry and Catalysis Department, Ulm University, Ulm, Germany in 2003 and to the Chemical Reaction Technology department, Erlangen-Nuremberg University (FAU), Germany in 2007. He was a Professor of physical chemistry at the National Research Centre (1990–2018) and he is currently a Professor of physical chemistry in the Chemistry Department, King Abdulaziz University, Saudi Arabia. Alternative catalytic techniques for organic synthesis are a prominent area of research for him. His focus is in advanced materials and heterogeneous catalysis. |
1. Introduction
Water pollution is a significant environmental concern that poses a threat on a global scale. Industrial effluents are considered as the primary source of water pollution and impurities due to their high chemical content and wide range of organic and inorganic contaminants.1,2 The common industrial wastewater sources are presented in Fig. 1.3,4 Industrial discharges are wreaking havoc on the ecosystem, potentially causing long-term harmful effects on the environment. Moreover, the chemical-intensive nature of industrial activities can have a detrimental impact on the environment at every stage of production.5,6 Generally, the characteristics of industrial effluents vary depending on the type of product made and the chemicals utilized. Most industrial effluents have significant levels of suspended and dissolved particles, organic and inorganic contaminants, toxic chemicals, non-biodegradable products and color, all of which are harmful to the environment and human health.7–9 The discharge of raw industrial effluents might cause the following environmental problems: (a) visible and irregular pigmentation of water surfaces. (b) Severe consequences on organisms/microorganisms affecting their organic activities according to pollutant concentration and exposure time. (c) Significant damage to the aquatic lifecycle due to the absorption of dissolved oxygen by pollutants.1,10–12 On the other hand, direct handling or exposure to contaminated media has been associated with various health concerns in humans. In this matter, numerous illness symptoms have been reported like skin irritation, cardiovascular diseases, eye and lung infections, and a variety of other harm issues.13–15 Many studies warned of the danger of “dioxin” contamination from the chemicals employed in most industrial activities. This substance pollutes farmed areas and waterways, and it may be harmful to health since it causes major illnesses such as cancer, renal failure, and fetal mutilation.16,17
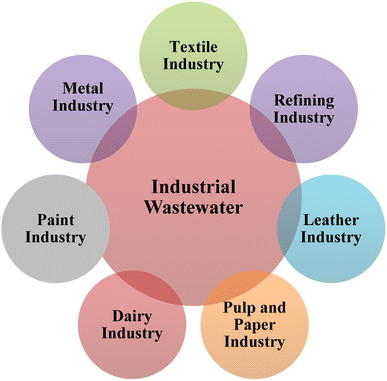 |
| Fig. 1 Common sources of industrial wastewaters. | |
Lately, many developing nations have experienced challenges caused by industrial wastewater and many health problems have been recorded for plants, animals and humans.18,19 This is due to the release of industrial effluents without adopting an effective treatment method. The conventional biological treatment processes have been reported insignificant by many researchers due to low biodegradation of many contaminants and dyes.20,21 Numerous researchers have reported the significance of coagulation/flocculation process in industrial wastewater treatment due to its efficacy, convenience of use, and low cost.10,22 The coagulation/flocculation treatment technology offers significant advantages in treating effluents that are frequently released, such as enhanced water quality. However, its full potential is restricted by some significant drawbacks. For instance, challenges concerning coagulant/flocculant recyclability can hinder efficiency, and the generation of large sludge volumes can create logistical and environmental issues. Additionally, the use of certain aluminum-based coagulants/flocculants has been associated with adverse health effects, further limiting the technology's potential.23,24 Nonetheless, ongoing research and innovation in this area hold the promise of overcoming these limitations, making coagulant/flocculant treatment technology an increasingly attractive option for industrial wastewater treatment. Recently, natural materials from various sources have been investigated as a sustainable alternative to chemical coagulants/flocculants. Most reviews on natural-based coagulants/flocculants (NC/Fs) focused on the possible application of plant-based sources such as plant seeds, tannin, and certain vegetables/fruit peels and their lab-scale potential relevance for water treatment. Our review expands the scope by examining the feasibility of using natural materials from other natural sources for industrial effluent decontamination. By analyzing the latest data on natural-based coagulants/flocculants, we identify the most promising techniques for making these materials stable enough to compete with traditional options in the marketplace. An adequate demonstration of diverse preparation methods has also been presented to highlight the exceptional chemical/physical properties, effectiveness, and recyclability of NC/Fs. An interesting presentation of the results of various recent studies have been highlighted and discussed. Additionally, we highlight the recent success of using magnetic-natural coagulants/flocculants (M-NC/Fs) in treating diverse industrial effluents, and discuss the potential for reprocessing spent materials as a renewable resource. The review also provides innovative ideas for proposed large-scale treatment systems employed by MN-CFs. Current challenges to the commercialization of NC/Fs that impede their application to industrial-scale wastewater treatment have also been identified.
2. Coagulation/flocculation process for industrial wastewater treatment
The coagulation/flocculation process is widely employed for industrial wastewater treatment to remove colloidal particles, dyes, heavy metals, and organic matter due to its ease of use, low cost, and upgradeability.25,26 This process involves the addition of coagulants/flocculants, which can be chemical or natural materials that destabilize and aggregate the suspended particles and colloids in the wastewater.27 Coagulants, such as aluminium sulfate, ferric chloride, and polyaluminum chloride, work by neutralizing the charge on the particles, while flocculants, such as polyacrylamide and chitosan, bind the destabilized particles together to form larger, denser flocs that can be easily separated from the wastewater.28,29 Generally, the performance of the coagulation/flocculation processes is affected by several environmental factors, including pH, coagulant/flocculant dosage, mixing rate, mixing time, temperature, and the physical characteristics of the coagulant/flocculant material, including density and particle size (refer to Fig. 2).29 pH is a critical parameter in the coagulation/flocculation process because it influences the charge on the particles and the activity of the coagulants/flocculants (C/Fs). The optimal pH for coagulation/flocculation is typically in the range of 6.5 to 7.5, but this can vary depending on the type of C/F used and the characteristics of the wastewater.30 The pH of the wastewater can be adjusted using acids or bases to achieve the desired pH range.30 On the other side, the dosage of C/Fs needs to be carefully controlled to ensure that the optimal amount is added for effective treatment.31 The physical and chemical characteristics of the C/Fs utilized also affect the efficiency of the process. Adding C/F with certain physical and chemical characteristics to wastewater might therefore improve coagulation performance and floc aggregation.24,32 The mixing time could also influence the rate and completeness of the reaction. It must be sufficient to guarantee that the C/Fs are thoroughly dispersed in the wastewater and that the particles have sufficient time to destabilize and agglomerate.33 The appropriate mixing time varies depending on the type of C/F utilized and the characteristics of the wastewater, but typically ranges between 5 and 30 minutes.24,28 Another critical component in the coagulation/flocculation process is the mixing rate. It influences the rate of dispersion as well as the shear forces that act on the particles. The appropriate mixing rate is also influenced by the characteristics of the wastewater and the type of C/F utilized; however, it typically varies between 200 and 600 rpm.14,24 In addition to these parameters, the design of the coagulation/flocculation system has a considerable impact on the process's efficiency. The type of mixing mechanism, the size and shape of the mixing tank, and the placement of the injection point for the C/Fs can all impact the effectiveness of the process.20 Overall, the coagulation/flocculation process is a multi-faceted process that requires careful optimization of several parameters to achieve efficient degree of treatment. The proper selection and control of these parameters can improve the quality of the treated water, reduce the environmental impact of industrial effluent, and reduce the overall costs of wastewater treatment. Several studies have investigated the coagulation/flocculation influencing parameters to acquire the most effective treatment. Karam et al. (2021) examined the viability of applying coagulation/flocculation process for real textile effluent decontamination with different dosages of FeCl3 coagulant (0.25–1.5) g L−1 at pH (1–11), rapid mixing (50–250) rpm for (1–5) min, slow mixing (10–50) rpm for (10–30) min and settling time (10–50) min. The optimum treatment rates were achieved at pH 9, 150 rpm for 1 min rapid mixing, 30 rpm for 20 min slow mixing and settling period of 30 min.33 Dehghani et al. (2016) also obtained considerable COD, turbidity, and TSS removals up to 38.6%, 63.7%, and 62.1%, respectively, after treating oil refinery effluent at 70 mg L−1 optimum of Moringa oleifera (MO) dose.34 In a study conducted by Jagaba et al. (2020), it was found that an optimal dosage of 2000 mg L−1 of MO resulted in significant removal rates of turbidity, COD, TSS, color, and oil & grease from palm oil mill effluents. Specifically, the removal rates were observed to be 88.3% for turbidity, 38.6% for COD, 95.4% for TSS, 90.2% for color, and 87.1% for oil & grease. It was also observed that contaminant removal rates rise as the C/F dose increases until the optimal C/F dosage is reached.35 An investigation into the effect of mixing rate on food industry wastewater treatment was conducted by Anteneh et al.36 In the study, a natural chitosan-based coagulant was used at different stirring rates (ranging from 50–200 rpm) to reduce COD and color. The results showed that mixing rates between 50 and 120 rpm resulted in COD and color reduction of 58–72% and 63–81%, respectively. The best outcomes were obtained at 150 rpm, with COD and color removal rates of 83% and 90%, respectively. However, the researchers reported a significant decrease in COD and color removal rates when the mixing speed was increased to 200 rpm.36 These findings suggest that the mixing rate plays a crucial role in food industry wastewater treatment, and identifying an optimal mixing speed is essential for achieving optimal results. Therefore, it is crucial to carry out jar testing on a laboratory scale to assess the impact of different operating parameters and determine the optimal conditions. This allows researchers and wastewater treatment professionals to identify the ideal dosages of C/Fs, mixing rates, settling time, and other parameters that can influence treatment efficiency. By optimizing these parameters, the treatment process can achieve the desired results in terms of contaminant removal rates and ensure that the effluent meets the required regulatory standards.10,33
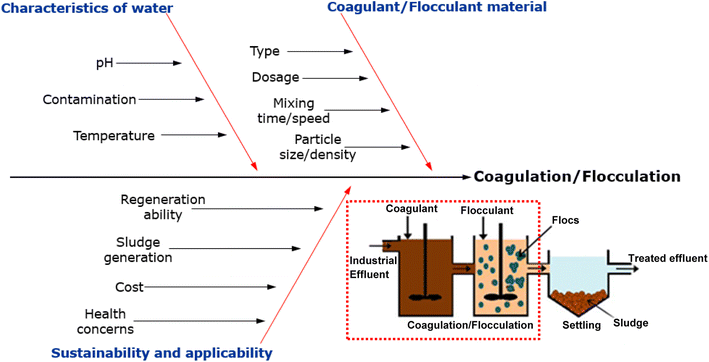 |
| Fig. 2 Main parameters affecting the coagulation/flocculation process. | |
In general, the selection of efficient C/F is critical for advancing treatment efficiency. As a result, researchers have presented numerous alternatives, including the utilization of chemical and natural coagulants/flocculants (C/Fs), such as chitosan, alginate, starch, and various organic polymers. The following sections present frequently utilized chemical and NC/Fs with a detailed analysis of their properties, preparation procedures, significance drawbacks and limitations. Subsequently, the use of MN-C/Fs and their ability to treat various industrial wastewaters are presented.
2.1. Chemical coagulants/flocculants
Chemical coagulants/flocculants are often employed in industrial wastewater treatment to remove suspended particles and other pollutants.33,37 Chemical coagulants are generally inorganic salts that are added to the wastewater to neutralize the electrostatic charges on the particles, destabilize the suspended solids, and promote aggregation into larger particles that can be more easily removed. Common chemical coagulants include aluminum sulphate (alum), ferric sulphate, ferric chloride, and polyaluminum chloride (PAC).38,39 Contrarily, chemical flocculants are generally organic polymers that are added to wastewater to make the particles larger and heavier so that they will settle or filter out more easily. These polymers possess a large molecular mass and a high concentration of electric charge, which allows them to adsorb onto the surfaces of the particles and create bridges between them.40 Larger flocs are created as a result, which are easier to remove from the wastewater. Polyacrylamide, polyethyleneimine, and polydiallyldimethylammonium chloride (polyDADMAC) are common chemical flocculants.41 Kaur et al. (2021) investigated the use of alum as a coagulant for the treatment of wastewater from a paper mill, and the results showed that alum was effective in reducing COD and TSS by up to 78% and 81%, respectively at 40 mg L−1 dose.25 Ribera-Pi et al. (2020) investigated the use of ferric chloride for the treatment of effluents from a petrochemical plant. The findings indicated that the use of ferric chloride was successful in decreasing COD levels, oil and grease, and heavy metal concentration.42 In general, the effectiveness of chemical coagulants/flocculants is well recognized because of their effectiveness pollutants from industrial effluents; however, further research suggests that long-term exposure to these substances can result in numerous health risks, such as Alzheimer's and senile dementia. Furthermore, producing large amounts of sludge since it is extremely difficult to regenerate or reuse. According to reports, a water treatment facility that employs alum and has the capacity to treat 190 million liters of water per day is expected to produce a minimum of 3 tonnes of solid waste daily, resulting in over 1000 tonnes annually.24,28 This finding has an indirect impact on the excess sludge entry to dumping sites, which are presently considered inadequate to handle the fast-increasing volume of sludge generated. Numerous types of particles, comprising carbon nanotubes,43 zeolites,44 bentonite,45 and silica27 have been introduced to advance coagulation/flocculation performance and reduce treatment time. It is possible that these additions aided to improve the coagulation/flocculation treatment performance and processing, but they are still limited to being easy to separate and regenerate. Additionally, the use of chemical coagulants/flocculants often generates a significant volume of sludge, increasing the cost of disposing of potentially hazardous sludge.32 Due to the negative impacts of chemical coagulants/flocculants on the environment and human health, recent research has focused on identifying alternative options for achieving water clarity. As a result, an increasing focus is being placed on the advancement of NC/Fs as a more environmentally friendly and sustainable substitute for chemical coagulants/flocculants.
2.2. Natural coagulants/flocculants (NC/Fs)
In contrast to chemical substances, NC/Fs are nontoxic, sustainable and generally harmless. Moreover, natural extracts are often locally available, making them affordable alternate to chemical substances.46,47 A comparative diagram between chemical and NC/Fs is shown in Fig. 3. Natural coagulants/flocculants are derived from natural sources, such as plants, fungi, and bacteria. They contain a wide range of active compounds, including soluble proteins, which have positive charges when it interacts with a solution. These proteins can bind to the negative ions in raw effluents, which cause turbidity.48–50 Fig. 4 shows the diverse main sources of NC/Fs representing their chemical composition compared to chemical coagulants/flocculants. Many researchers reported the superior performance of NC/Fs in water treatment and their potential decontamination rates for industrial wastewaters owing to their diverse intrinsic characteristics.34,50–52 These characteristics comprise long polymer chains, great cationic charges and efficient settling with large aggregate associating capacity. Table 1 lists the application of variously extracted NC/Fs for industrial wastewater decontamination. In many researches, NC/Fs have been reported to produce substantially smaller sludge volume (up to five times lesser than chemical C/Fs).53 As a result, the expenses of sludge treatment and management are reduced, making it a more viable alternative. Another advantage in terms of processing and operating costs is that NC/Fs are non-corrosive and do not devour alkalinity, therefore pipe erosions and pH adjustments may be avoided, allowing for more cost reserves. The main three sources of NC/Fs, namely plant, animal, and microorganism are thoroughly discussed in the following subsections.50,54
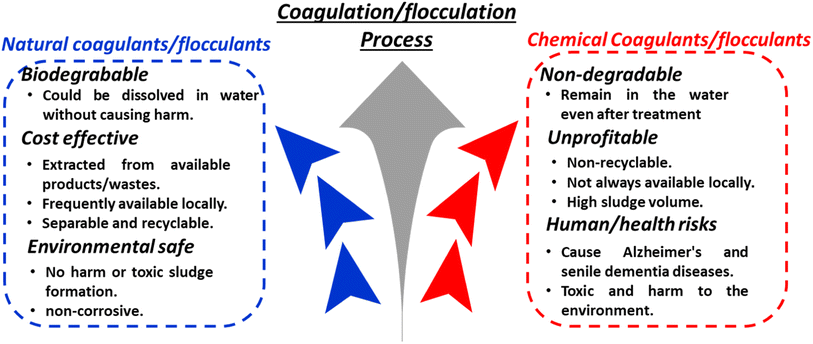 |
| Fig. 3 A comparative diagram between chemical and natural coagulants/flocculants. | |
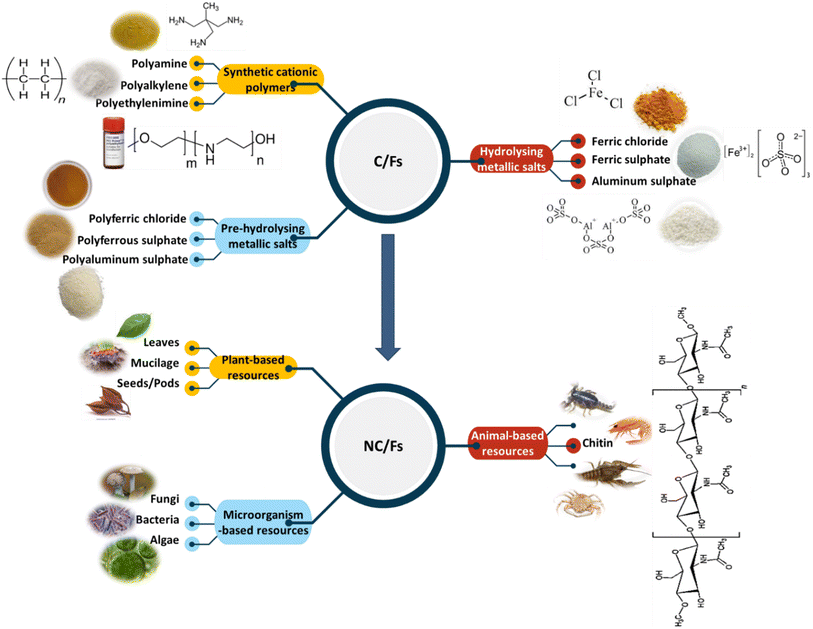 |
| Fig. 4 Most common sources of chemical and natural coagulants/flocculants. | |
Table 1 The effectiveness of variously extracted NC/Fs for industrial wastewater decontamination
NC/F extraction |
Effluent type |
Contaminant/initial concentration |
Operating conditions |
Removal |
Ref. |
Plantago ovata seeds |
Textile industry |
COD: 642 mg L−1 |
Dose: 1.5 mg L−1, pH < 8, settling time: 30 min |
89% |
74 |
Moringa oleifera |
Textile industry |
Turbidity: 250 NTU |
Dose: 60 mL |
48%, 70%, 57% |
57 |
TDS: 5750 mg L−1 |
TSS: 1250 mg L−1 |
Cassia obtusifolia seeds |
Pulp and paper industry |
TSS: 841 mg L−1, COD: 1453 mg L−1 |
Dose: 0.75 g L−1, pH 5, settling time: 1 min |
86.9%, 36.2% |
75 |
Cicer arietinum seed |
Petroleum industry |
COD: 2508 mg L−1 |
Dose: 1.5 mg L−1 |
95.2%, 98.9%, 83.8% |
76 |
Eggplant seed |
Turbidity: 435 NTU |
Dose: 2 mg L−1 |
92.1%, 99.4%, 81.8% |
Radish seed |
Oil: 100 mg L−1 |
Dose: 2 mg L−1 |
93.5%, 98.8%, 88.2% |
Date palm |
Pharmaceutical industry |
Color |
Dose: 100 mg L−1, pH 2, settling time: 50 min |
99.9% |
77 |
Chitosan |
Dairy industry |
TDS: 730 mg L−1 |
Dose: 10 mg L−1, pH 4 |
57%, 62% |
78 |
COD: 1532 mg L−1 |
Chitosan |
Paper industry |
TSS: 2029 mg L−1 |
Dose: 0.3 g L−1, pH 6 |
81%, 78% |
25 |
COD: 2816 mg L−1 |
Tannin |
Textile industry |
Color |
Dose: 180 mg L−1 |
100% |
59 |
Moringa oleifera |
Dairy industry |
Turbidity: 648 NTU |
Dose: 400 mg L−1 |
65.6%, 94.6%, 90.8%, 72.4%, 64% |
51 |
Total solids: 17 500 mg L−1 |
TSS: 5950 mg L−1 |
Sulphate: 1680 mg L−1 |
Chloride: 417 mg L−1 |
Cassia fistula seeds |
Textile industry |
Color |
Dose: 1.17 mg L−1 |
93.8% |
60 |
Tamarind seeds |
Detergent wastewater |
Turbidity: 1670 mg L−1 |
Dose: 400 mg L−1 pH−1: 7.25 |
97.7%, 39.6% |
8 |
COD: 1118.6 mg L−1 |
Devilfish invasive species |
Ceramic industry |
Turbidity: > 1000 ± 0.00 |
Dose: 800 mg L−1 |
74%, 79%, 94% |
65 |
COD: 318.14 ± 2.74 |
TSS: 0.80 ± 0.02 (mean values) |
Bacillus licheniformis |
Food industry |
BOD: 4.597 mg L−1 |
15 g L−1 molasses within 24 h incubation time |
75.9%, 80.2% |
72 |
COD: 9.152 mg L−1 |
Fenugreek seeds and okra |
Palm oil mill effluent |
Turbidity: 12 667 NTU |
Fenugreek dosage: 4.09 mg L−1 okra dosage: 57.69 mg L−1 pH−1: 3.17 |
94.9%, 92.7%, 63.1% |
73 |
TSS: 13 786 mg L−1 |
COD: 28 114 mg L−1 |
2.2.1. Plant-based coagulants/flocculants. Plant-based coagulants/flocculants are organic substances obtained from natural sources such as seeds, roots, and leaves of various plants. These substances are classified as both ionic and non-ionic polymers with varying molecular weights.55,56 They exhibit coagulation and flocculation properties due to the presence of active compounds such as proteins, polysaccharides, and tannins. Plant-based coagulants/flocculants are considered to be eco-friendly and sustainable alternatives to synthetic chemicals. Commonly used plant-based C/Fs include Moringa oleifera (MO), chitosan, and Tannins.46,49 Moringa oleifera, commonly known as the drumstick tree. The seeds of this plant contain cationic polyelectrolytes that can bind effectively with negatively charged particles such as dyes and heavy metals. When added to wastewater, the cationic polyelectrolytes present in MO seeds destabilize the suspended particles and facilitate their aggregation and settling, leading to the removal of contaminants.57,58 Tannins and lignin have also shown promising results in removing dyes and heavy metals from industrial wastewater. Tannins are naturally occurring polyphenolic compounds found in plants such as tea, grapes, and oak. These compounds have a high affinity towards heavy metals and can form insoluble complexes with them, leading to their removal from the wastewater.59 On the other hand, lignin is a complex aromatic polymer found in the cell walls of many plants. Its ability to remove heavy metals makes it a potential candidate for the treatment of industrial wastewater.49 Several studies have investigated the use of plant-based coagulants/flocculants in industrial wastewater treatment, with promising results. Tannin coagulant has been investigated for treating wastewater from the textile industry. The tannins were found to achieve 100% color removal at 180 mg L−1. The study also found that the use of tannins resulted in higher settleability and lower sludge production compared to the use of chemical coagulants.59 Moringa oleifera seeds were used as a coagulant for the treatment of dairy wastewater, and the results showed that it was effective in reducing total solids by 94.6%, sulphate by 72.4 and chloride by 64%.51 M.-T. Dao et al. (2021) investigated the use of natural coagulant obtained from Cassia fistula seeds for the treatment of wastewater from a textile dyeing industry. The results showed that the coagulant was effective in color removal with 93.8%.60 The use of a natural coagulant obtained from tamarind seeds for the treatment of detergent wastewater. The results showed that the tamarind seed coagulant was effective in reducing turbidity and COD, with a maximum removal efficiency of 97.7% and 39.6%, respectively.8
2.2.2. Animal-based coagulants/flocculants. Animal-based coagulants/flocculants are obtained from various animal sources, such as eggshells, bones, and blood. These C/Fs work by creating a positive charge, which attracts the negatively charged particles in the water, causing them to clump together and settle.44,48 Chitosan is a natural C/F often used in wastewater treatment. It is a naturally occurring biopolymer found in marine invertebrates, such as crustaceans and insects. It is a deacetylated derivative of chitin, which is considered the second most prevalent natural source of polysaccharide after cellulose. Chitosan possesses excellent coagulation and flocculation properties and can effectively remove pollutants from industrial wastewater. It is biodegradable and can be easily obtained from waste materials generated by the fishing industry, making it a sustainable and cost-effective option.61 It has been found to be effective in removing suspended solids and turbidity from wastewater due to its positively charged amino groups, which can bind with negatively charged particles and promote coagulation. Chitosan and chitin have an additional 6.89% of nitrogen content compared to other polysaccharides, which are typically rich in active chemicals like hydrogen, oxygen, and carbon. This makes them intriguing for marketing purposes.44 Since chitosan has amino groups, it may bind to a variety of metal ions.62 In addition, chitosan derived from crab shells contains 20% to 30% chitin, 30% to 40% proteins, and 40% to 50% calcium carbonate.63 To utilize these active chemicals in the coagulation–flocculation process to eliminate colloidal organic and inorganic contaminants from wastewater, their contents can be modified.64 Interparticle bridging, adsorption, charge neutralisation, and hydrophobic flocculation are coagulation-related processes.33 Gelatin is another frequently used animal-based coagulants/flocculants used for industrial wastewater treatment. It is a protein obtained from collagen-rich animal tissues, such as bones and hides. Gelatin has been found to be an effective coagulant/flocculant for the removal of dyes and heavy metals from wastewater due to its ability to form a stable gel-like structure. This gel-like structure has a high affinity for pollutants and is able to effectively remove them from wastewater. Some studies have reported promising results for the use of animal-based coagulants and flocculants in industrial wastewater treatment. M. M. Aguilera Flores et al. (2022) investigated the use of collagen extracted from devilfish invasive species as a coagulant in treating wastewater from ceramic industry wastewater. The results showed that the collagen effectively reduce turbidity, and remove COD and TSS by 74, 79, and 94%, respectively.65 Chitosan was used as a natural flocculant in the treatment of wastewater from a palm oil mill. The study found that chitosan was effective in reducing the concentration of solids and residual oil in the wastewater, with removal rates of up to 90% and 95%, respectively.66 In another study, gelatin extracted from meat & bone meal was used as a flocculant in treating wastewater. The results showed that the gelatin could effectively remove suspended solids and organic matter from the wastewater and promoted the settlement rate.67 N. Precious Sibiya et al. (2021) investigated the use of eggshell as a coagulant for treating high polluted wastewater. The study found that eggshell coagulant was effective in reducing TSS, turbidity, and color from the wastewater, with removal efficiencies of 98.52%, 98.89%, and 98.88%, respectively.14
2.2.3. Microorganism-based coagulants/flocculants. Microorganism-based coagulants and flocculants have emerged as a promising alternative to conventional chemical-based treatment methods for industrial wastewater. Microorganisms such as bacteria, fungi, and algae are known to produce a variety of biomolecules, including proteins, polysaccharides, and extracellular polymeric substances (EPS), which can act as coagulants or flocculants.52,68 These biologically-derived agents are capable of effectively removing suspended particles, organic matter, and other contaminants from wastewater, making it suitable for safe discharge into the environment. For instance, bacterial EPS have been reported to exhibit excellent adsorption capacity for cationic dyes due to the presence of amine, carboxyl, and hydroxyl groups.37,69 Moreover, fungal proteins can also act as coagulants to remove anionic dyes from wastewater by destabilizing the colloidal particles and aggregating them into larger flocs. Similarly, microorganism-based coagulants/flocculants can effectively remove heavy metals from wastewater by forming metal hydroxide precipitates or by adsorbing them onto the surface of the microorganisms. The species of the microorganism affects how much coagulant is produced from the bacterium strain. For instance, the proteoglycan coagulants produced by Bacillusmojavensis strain 32A include 98% polysaccharide and 1.6% protein, and they can attain up to 96% flocculating activity at pH 10.69,70 Consequently, it is important to construct a number of bacteria selection criteria in order to find a suitable microorganism that might be employed as a prospective biocoagulant generator. Four steps make up the procedure for obtaining biocoagulants from microorganisms: preliminary bacterial strain identification, bacterial screening, determining flocculating activity, and optimising culture conditions for NC/Fs synthesis.70,71 Microorganisms need some essential elements, such carbon and nitrogen to flourish.66 Sludge, which is rich in nutrients can be used to cultivate microorganisms to create sustainable NC/Fs. Agro-industrial wastes that contain primarily polysaccharides, such sugarcane, palm oil effluent, starch, sago molasses, maize steep liquor, and soybean juice, can also be utilized for microbial growth. However, each species' culture medium is distinct and need additional study. Ramadhani et al. (2022) investigated the use of a microbial flocculant produced by Bacillus licheniformis for treating food industry wastewater. The study found that the microbial flocculant was effective in reducing the BOD and COD of the wastewater, with a removal efficiency of 75.9% and 80.2% respectively.72 On the other side, the use of a bioflocculant produced by a bacterial strain of Serratia marcescens for treating real aquaculture effluent has been reported. The study found that the bioflocculant was effective in reducing the turbidity, and suspended solids of the water, with removal efficiencies of 83.95% and 78.82%, respectively.69 Natural coagulants and flocculants derived from fenugreek and okra, respectively, have been applied in the treatment of palm oil mill effluent. This approach resulted in removal efficiencies of 94.9%, 92.7%, and 63.1% for turbidity, TSS, and COD, respectively. Additionally, the sludge generated during the treatment process had a high calorific value, indicating that it could be employed as solid fuel briquettes.73
2.3. Common preparation methods of NC/Fs
Typically, NC/Fs are utilized either in powder form or as a stock solution; their specific preparation methods varying depending on their composition and source. The general processing stage of NC/Fs preparation/production is presented in Fig. 5. Various techniques can be employed to produce NC/Fs, which include extraction from botanical sources, chemical alteration, and biological procedures.34,75 An approach involves the recovery of coagulation and flocculation substances from plant materials, such as seeds, leaves, and roots, using methods such as grinding, sieving, and boiling, followed by centrifugation and filtration.56 These extracts can be utilized directly as coagulants and flocculants, or they can be refined through a range of techniques, including filtration or centrifugation.28,32 The effective use of these extractions depends on their characteristics, which need to be characterized and optimized for efficient applications. Characterization involves various techniques, such as Scanning electron microscopes (SEM), Energy-dispersive X-ray spectroscopy (EDS) analysis, and Fourier transform infrared (FTIR) spectroscopy, which are used to analyze the morphology, composition, and functional groups of natural coagulants and flocculants. SEM provides high-resolution images of the surface morphology of natural coagulants and flocculants, allowing for the observation of their particle size, shape, and distribution. EDS analysis, on the other hand, provides elemental composition information and identifies the presence of metals or other impurities. FTIR spectroscopy is used to identify the functional groups present in NC/Fs by measuring their vibrational energy. It also provides information on the type of bonds present, such as C–H, O–H, and N–H, which play a crucial role in the coagulation and flocculation process. Fig. 6 presents a comprehensive display of the various effective NC/Fs, featuring illustrative characterizations through SEM, EDS analysis, and FTIR spectra. A study on the elimination of color from textile wastewater, employing basil seed mucilage. Initially, the basil seeds were cleansed to eliminate any extraneous impurities and then immersed in water with a 0.9% NaCl solution at a temperature of 50 °C. The mixture was stirred for an hour until the seeds were fully swollen. Subsequently, a coarse plate was used to remove the gum layer from the seed surface, and the resulting mucilage was separated from the seeds using a 500-micron steel filter. The collected mucilage was utilized as a coagulant for treating wastewater.79
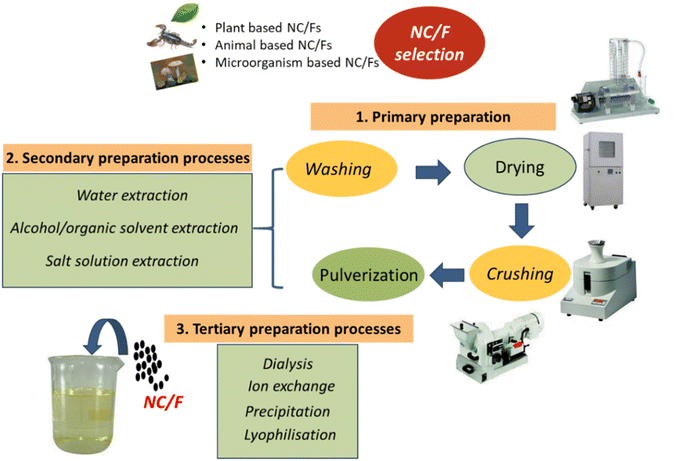 |
| Fig. 5 Common preparation methods and procedures of NC/Fs. | |
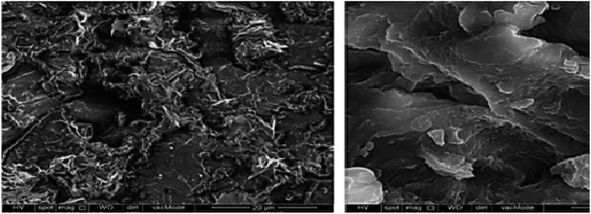 |
| Fig. 6 SEM microphotographs of flocs in both raw effluents and fenugreek natural coagulant, adapted with permission from ref. 73., Copyright, Elsevier, 2021. | |
Recently, the seeds of C. fistula were dried under sunlight and ground into powder. Hexane was used as a solvent in a Soxhlet system to extract the seeds. After 48 hours, the fibrous mass was obtained by ethanol precipitation. The solid material was then washed with distilled water and dried at 50 °C in air for 2 hours to produce C. fistula seed gum.60 Tamarindus indica (TI) seeds were utilized to produce a coagulant. The seeds were first washed with fresh water to eliminate any dirt and pulp, and then dried in the shade for 24 hours. The seed coat was removed by treating the seeds with hydrochloric acid, and the seeds were then dried once again. After drying, the seeds were ground into a fine TI powder using a grinding machine, and this powder was utilized as the coagulant.57 In another research,80 the peel was separated from fresh oranges, and the pith was removed from the peel using a laboratory knife to extract pectin from orange peel for use as a coagulant in wastewater treatment. The pith was then dried at room temperature until all the moisture had evaporated. Lemon-sourced citric acid was added to approximately 227 g of the dried pith, after which it was allowed to rest for an hour. Then, 2 cups of water were added to the mixture, and it was left for another hour. The mixture was heated for 10 minutes and allowed to cool before being filtered using a muslin cloth.80 A coagulant made from chestnut and acorn seeds was used to remove turbidity. To prepare the coagulant, the powder obtained from grinding the seeds was dissolved in either distilled water or NaCl solution. The active ingredient was then extracted with the assistance of a filter paper.81 The seeds of Sesamum indicum were carefully washed with de-ionized water to remove any impurities. After separating and removing any defective seeds, the samples were allowed to dry at room temperature for a period of two weeks to preserve their physicochemical properties. The dried seeds were then crushed to a particle size of approximately 300 μm. To prepare the coagulant, the oil was removed from the milled sample through n-Hexane. Next, 10 g of the resulting oil-free sample was dissolved in 250 mL of salt solution and heated to 70 °C while constantly stirred with a magnetic stirrer for one hour.
Following the reaction, the mixture was filtered, and the resulting filtrate was air-dried, ground again, and then kept in a sealed container for later utilization.82 Another study prepared a coagulant from Leucaena seeds for the removal of Congo red dye from synthetic wastewater. A powder of 5 g Leucaena seeds' kernels was combined with 100 mL of a solution containing 3 moles of sodium chloride per liter. The mixture was stirred for half an hour, and then the liquid was separated from the solids by using centrifugation (spinning at 5000 rpm for 10 minutes) and filtration. This liquid extract was used directly as a natural coagulant, without any additional processing. SEM analysis was utilized to examine the morphology of IONPs, which revealed that the IONPs were spherical iron nanoparticles that had aggregated. The purity of the IONPs was found to be high based on the EDS spectra, which showed that they contained 72.67 wt% Fe and 27.33 wt% O, with the C peak originating from the carbon tape used in the SEM-EDS analysis being excluded.83 To prepare the coagulant from Jatropha curcas seeds, the outer skin of the seeds was manually removed before selecting high-quality seeds. The kernel of the seeds was then ground into a fine powder (63–500 μm) using an electric blender. To extract the active ingredients, 5 g of the powder was blended with 100 mL of distilled water at ambient temperature for a few minutes, and then the resulting mixture was filtered via a muslin cloth.84 A study compared the coagulation efficacy of natural seed-based active coagulants (bean, lima bean, lentil, and fenugreek) extracted via diverse solvents (distilled water, NaCl, and NaOH). In the experiment, 5 g of seed powder was mixed with 50 mL of solvent for 1 hour at a temperature of 40 °C. After centrifugation, the clear solution was utilized as the coagulant in the water treatment. The coagulant extracted with 0.3 M NaCl was found to exhibit the highest coagulation efficiency of over 75%, compared to other solvents. The researchers hypothesized that the active coagulation agent was protein, and the increase in the concentration of the salts led to higher extraction of the protein. The protein's solubility was boosted in the presence of salt, and it was able to precipitate when exposed to an excessive amount of salt solution. The coagulation efficacy improved steadily up to a specific point, which suggests the potential of the extracted coagulant for wastewater treatment.13 In a recent study, natural coagulants derived from leguminous vegetables (such as green beans and pigeon pea) and fruit seeds (including Tamarindus indica and date palm) were utilized to eliminate turbidity. These seeds were pulverized and subjected to crude coagulant extraction using distilled water and a 1 M NaCl solution. The research results indicated that the distilled water extract of pigeon pea had the highest turbidity removal rate at 81%, whereas date palm performed the least effectively with a rate of 62.5%. When subjected to NaCl extraction, pigeon pea had the highest removal rate of 94.6%, followed by Tamarindus indica with a rate of 76.1%. The functional group peaks observed in the FTIR spectra (such as –OH, –NH, COOH, C
O, C–C, and C–H) confirmed the presence of protein-specific functional groups that support their potential application as coagulants.85 In another recent study, fenugreek seeds were subjected to a drying process at 70 °C in an oven for 24 hours. Once dried, the seeds were ground using a table top grinder for approximately two minutes to achieve a fine powder form. The powder was then sifted through a mesh with a range of 200 to 500 microns, and the remaining powder was ground and sifted again to ensure maximum utilization. The obtained powder was placed in a beaker, covered with parafilm and stored in a dry, cool area in the laboratory for use as a natural coagulant.73 Similarly, in the same study, okra was prepared for use as a natural flocculant in palm oil effluent treatment. The okra was cleaned with purified water to eliminate scums such as dust and remaining pesticides. The okra pods were trimmed at the stem end and diced into cubes measuring 2 to 4 mm. The diced okra was then submerged in distilled water in a food-grade plastic container using a 1
:
1 weight ratio of okra to water. The okra was left to soak for 24 hours. Then, the okra mucilage was extracted by passing it through a nylon cloth with added pressure to collect most of the mucilage. The strained mucilage was moved to a 500 mL beaker, sealed with parafilm, labelled, and kept in a refrigerator for storage at a temperature between 3 and 8 °C. A SEM analysis was conducted to study the formation of flocs in the sludge of untreated effluent (Fig. 6). The sludge was found to have fibrous web network structures that were asymmetrical and had random lengths of fibers with voids in the network. Additionally, the fenugreek natural coagulant was found to have a surface with sharp edges, numerous ridges, and deep void spaces.73 A microbial flocculant produced by B. licheniformis was used for food industry wastewater treatment. The preparation of B. licheniformis involved growing the culture on sterilized nutrient agar slant, followed by incubation at either 28 °C or 30 °C for 72 hours. Following the inoculation of 50 mL of pre-culture media, the solution was subjected to incubation on a rotary shaker at 200 rpm for 18 hours. Subsequently, the inoculated solution was moved to a 250 mL Erlenmeyer flask that contained 50 mL of fermentation media with a pH adjusted to neutral using either 1 M HCl or 1 M NaOH. Fermentation was performed for 72 hours on a 200 rpm orbital shaker at 37 °C, with sample assessments being taken every 24 hours.72
In general, the synthesis of NC/Fs has been widely explored as effective and sustainable alternatives to chemical coagulants/flocculants in industrial wastewater treatment. However, further research is needed to optimize the synthesis methods and evaluate the performance of these natural materials under different operating conditions. Magnetic natural coagulants/flocculants (MN-C/Fs) have been suggested to improve the coagulant/magnetic flocculant's responsiveness, which benefits treatment by improving superior solid–liquid separation efficiency, shortening hydraulic retention time, and achieving efficient, rapid settlement and lower energy consumption. The following part discusses the diverse syntheses methods of diverse MN-C/Fs with an interesting presentation of its application in industrial wastewater treatment highlighting the results of various recent studies.
3. Magnetic natural coagulants/flocculants (MN-C/Fs)
Magnetic coagulation/flocculation is a relatively novel method in which magnetic particles coagulate with other particles to generate magnetic flocs with increased settability. The MN-C/Fs has garnered loads of interest because it has more benefits than standard coagulation/flocculation processes.86,87 Combining magnetic materials with traditional coagulants/flocculants improves the coagulant/magnetic flocculant's responsiveness, which benefits treatment by improving solid–liquid separation efficiency, shortening hydraulic retention time, and achieving efficient, rapid settlement and lower energy consumption. In general, magnetic particles are coupled with a low-cost and widely applicable natural coagulants/flocculants.58 This method preserves the benefits of a little amount of coagulant/flocculant, robust decontamination ability, rapid settling time and speed. It also possesses the distinct magnetic separation properties of the recovered particles. To date, varied types of MN-C/Fs, with and without surface coating, have been employed for industrial wastewater treatment.88–90 Magnetic natural coagulants/flocculants have gained attention in recent years as an effective solution for industrial wastewater treatment. These materials are derived from natural sources such as plants, seeds, and microorganisms, and have magnetic properties that enable them to be easily separated from the treated wastewater using magnetic fields. One example of a magnetic natural coagulant/flocculant is magnetite, a naturally occurring mineral with a high magnetic susceptibility. Magnetite has been shown to be effective in removing various contaminants from industrial wastewater, including heavy metals, organic compounds, and bacteria. Moreover, magnetite has a high surface area and can act as an adsorbent, further enhancing its ability to remove contaminants from wastewater.91,92 Chitosan has been shown to have excellent flocculation properties and is effective in removing suspended solids and other pollutants from wastewater. When modified with magnetic particles, chitosan can be easily separated from the treated wastewater using magnetic fields.66,88 Fig. 7 shows the processing of common MN-C/Fs extractions assisted by ferric oxide particles. Furthermore, Table 2 lists diverse MN-C/Fs and their performance for industrial wastewater decontamination. A study investigated a magnetic Moringa oleifera (MMO) coagulant for the removal of TSS, color and COD from palm oil mill wastewater. The findings indicated that MMO was able to achieve a removal efficiency of 83.3%, 28.1% and 85.2%, respectively as a sustainable magnetic natural-based coagulant.58 In a recent study, researchers examined the effectiveness of two magnetized coagulants, chitosan magnetite (CF) and rice starch magnetite (RF), which were synthesized by co-precipitation in 3 changed proportions of natural coagulants (chitosan or rice starch) and magnetite nanoparticles. The tested wastewater exhibited an initial turbidity of 45.6 NTU, a color of 315 Pt. Co, a phosphate concentration of 1.18 mg L−1, a COD of 352 mg L−1, and an absorbance of 73.4%. The best magnetized coagulant was found to be RF with a ratio of 1
:
1, which removed over 80% of the contaminants and achieved 90% absorbance.61 Other researchers examined the potential of magnetic-chitosan nanocomposite (MCN) as a coagulant for treating palm oil mill effluent. The findings indicated that the magnetic chitosan particles (CMNP) were highly effective in removing turbidity, TSS, and COD from the effluent, with removal rates of 98.8%, 97.6%, and 62.5%, respectively. Compared to natural chitosan, the magnetic-chitosan particles exhibited better parameter reductions at much lower dosage consumption, even at the original pH of the palm oil mill effluent. The researchers also highlighted that the magnetic properties of the particles facilitated their recovery and reuse, providing a significant advantage over traditional coagulants.93 In another study, a magnetic lime-based coagulant (MLC) was integrated with a membrane distillation process for pre-treatment of desulfurization wastewater. The results revealed full suspended solids removal and magnetic seed expressively accelerated the settling rates.94 A recent magnetic flocculant (CPAMF) was created by coating Fe3O4 with a positively charged form of polyacrylamide (CPAM). This was done to treat high level of turbid effluents. The findings of the study showed that CPAMF was effective in flocculating high turbidity water achieving optimal transmittance of 92.4% at dosage of 0.24 g L−1 and rapid mixing conditions 300 rpm and 20 min.89 To summarize, the synthesis and preparation of MN-C/Fs offer a sustainable and cost-effective approach for the treatment of various types of wastewaters. The use of natural and waste materials as a functionalizing agent for magnetic nanoparticles, combined with their magnetic properties, makes them highly effective for wastewater treatment, while also providing an opportunity for the valorisation of waste materials.
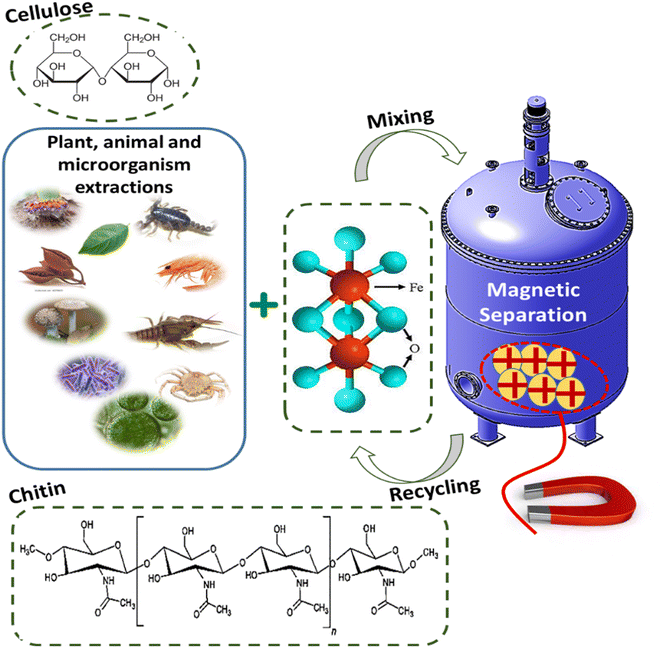 |
| Fig. 7 Processing of common MN-C/Fs extractions assisted by ferric oxide particles. | |
Table 2 The effectiveness of variously extracted MN-C/Fs for industrial wastewater decontamination
MC/Fs |
MN-C/Fs |
Industrial effluent type |
Contaminant |
pH |
Dosage |
Removal |
Ref. |
MC |
MN-F |
Synthetic wastewater |
Cu(II) |
6 |
100 mg L−1 |
93.4%, 88.6%, 61.4% |
90 |
MC-g-PAM |
MC-g-PAA |
CMNP |
MN-C |
Palm oil mill effluent |
Turbidity, TSS, COD |
6 |
25 mg L−1 |
98.8%, 97.6%, 62.5% |
93 |
Fe3O4 Leucocephala seed extrication |
MN-C |
Congo red synthetic wastewater |
Color |
3 |
20 mL L−1 |
90% |
83 |
MMO albumin fractionation |
MN-C |
Reactive black 5 synthetic wastewater |
Color |
6 |
115 mg L−1 |
94% |
95 |
CPAMF |
MN-F |
High turbid effluent |
Turbidity |
5 |
240 mg L−1 |
92.4% |
89 |
Fe3O4 MMO extraction (NaCl 1 M; 1 : 100) |
MN-C |
Synthetic dairy wastewater |
Turbidity |
7 |
1600 mg L−1 |
50.5%, 81.5% |
92 |
Color |
α-Fe2O3-MMO |
MN-C |
Textile effluent |
Turbidity |
9.27 |
400 mg L−1 |
91.4%, 92.4% |
86 |
Color |
Fe3O4 MMO extraction (CaCl2 0.03 M; 1 : 100) |
MN-C |
Reactive black 5 synthetic wastewater |
Color |
2 |
100 mg L−1 |
93.7%, 72% |
87 |
Total organic compound (TOC) |
3.1. Synthesis of MN-C/Fs
The synthesis and preparation of magnetic natural coagulants/flocculants (MN-C/Fs) involve several important steps, each of which must be carefully optimized to ensure the desired performance of the material.32 The first step in the synthesis of MN-C/Fs is the selection of suitable natural materials. These can be obtained from various sources, such as plants, animals, and microorganisms as previously discussed. The natural materials are typically rich in functional groups, such as carboxylic, hydroxyl, and amine groups, that can aid in the coagulation and flocculation process. Once the natural materials are identified, they are subjected to various processing steps, such as drying, grinding, and sieving, to obtain a fine powder. Next, the natural materials are modified to introduce magnetic properties. This can be achieved by incorporating magnetic nanoparticles into the natural materials, either through physical or chemical methods.88,93,96 The most commonly used magnetic nanoparticles are iron oxide nanoparticles (MNPs), which have excellent magnetic properties and biocompatibility.1 In addition to the synthesis of MN/Fs, there are also various methods for their preparation, such as emulsion polymerization and sol–gel synthesis.58,95 The modified natural materials are then subjected to various characterization techniques, such as X-ray diffraction, SEM, and FTIR, to confirm the successful incorporation of the magnetic nanoparticles. Finally, the MN-C/Fs are prepared by dispersing the modified natural materials in water and optimizing the conditions for coagulation and flocculation.97 The parameters that can be optimized include pH, temperature, dosage, and mixing speed. Once the optimum conditions are identified, the magnetic natural coagulants/flocculants can be used to treat industrial wastewater, leading to the efficient removal of suspended particles and contaminants.95,97
Sun et al. (2021) successfully prepared and modified three chitosan based magnetic flocculants MC, MC-g-PAM, and MC-g-PAA, for the optimum removal of Cu(II) from synthetic wastewater. The MC, MC-g-PAM, and MC-g-PAA material characterization including SEM, FTIR, and XRD reveal excellent magnetic induction, attaining outstanding removal rates of Cu(II) reached 93.4%, 88.6%, and 61.4%, respectively.90 In another study,88 a sequence of quaternized chitosan (QC)-grafted MNPs (Fe3O4-MNPs) were effectively prepared for diesel oil separation using a co-precipitation method, followed by surface coating with silica and aminopropyl to provide a surface for advance grafting of QC molecular chains (Fig. 8). The separation capacity was calculated to be greater than 105 mg diesel oil per mg magnetic flocculant. The flocculant's performance revealed to the huge amount of amino and hydroxyl groups on the surface of chitosan molecules, which contain lone pairs of electrons that can coordinate with metal ions.88,90 Other researchers used crosslinking method to prepare magnetic cellulose flocculant. A predetermined quantity of cellulose was combined with a suspension of magnetite powder in 100 mL of distilled water, and then heated to 65 °C using a water bath shaker for six hours. The mixture was allowed to cool to room temperature, and then 1 mL of glutaraldehyde (with 25% purity) was slowly added to the solution while stirring at room temperature for one hour to promote crosslinking. Afterward, the solution was filtered, rinsed with ethanol and distilled water, and dried in an oven at 50 °C until a constant weight was reached. The prepared magnetic cellulose flocculants showed high efficiency in the removal of turbidity, color, TSS, and COD from palm oil mill effluent, with removal rates of 74.6%, 63.9%, 77.2%, and 55.8%.96
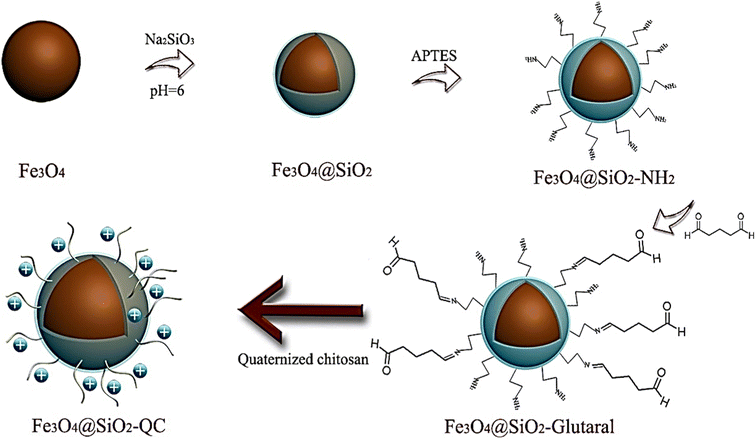 |
| Fig. 8 Synthetic scheme of the QC-grafted MNPs, adapted with permission from ref. 88, Copyright, Elsevier, 2018. | |
In a recent study researchers developed a magnetic coagulant by applying a co-precipitation method on Leucaena leucocephala seed extract with iron oxide nanoparticles, and evaluated its effectiveness in removing Congo red dye from synthetic wastewater. The coagulant exhibited a remarkable removal efficiency of 90% and demonstrated excellent recyclability, with a 30 mL L−1 sludge volume. Furthermore, the magnetic property of the coagulant enabled easy recovery after the treatment process, making it a promising and sustainable solution for dye wastewater treatment.83 Another study synthesized magnetic MO-Fe3O4 (MMO) nanoparticles coagulant via ultrasonic-assisted technique. The researchers first created a solution using MO seed powder and 0.1 L of 0.5 M CaCl2. This mixture was then stirred at 100 rpm for 30 minutes to allow for the extraction of the active components. After filtering the mixture, the resulting 3 wt% MO extract solution was stored in a refrigerator with a temperature range of 7–12 °C. Next, they mixed different masses of MNPs with 0.1 L of the 3 wt% MO extract solution and subjected the mixture to varying sonication times and temperatures. After completing the ultra-sonication process, the researchers filtered the blend via qualitative filter paper. Then, the powder of MMO coagulant was dried by using an air-drying oven at 60 °C until a stable weight was achieved. The study characterized the preparation conditions and appearance of both MMO and MNPs powder using FTIR and SEM analysis as shown in Fig. 9. The IR-spectra for MNPs showed a sharp intensity at 569 cm−1 indicating the Fe–O bond of magnetic material. The same band around 582 cm−1 was observed in MMO spectra, but with slight differences due to the introduction of other materials during synthesis (Fig. 9a). The characteristics of the biomass source, mostly MO, were still found in the MMO spectra. MNPs tended to agglomerate due to their ferromagnetic characteristics, while MMO had reduced agglomeration, possibly due to the introduction of ultrasonic force during synthesis. The study found that the ultrasonic force prevented MNPs from agglomerating, resulting in smaller, well-dispersed clusters in the MMO coagulant (Fig. 9b).58
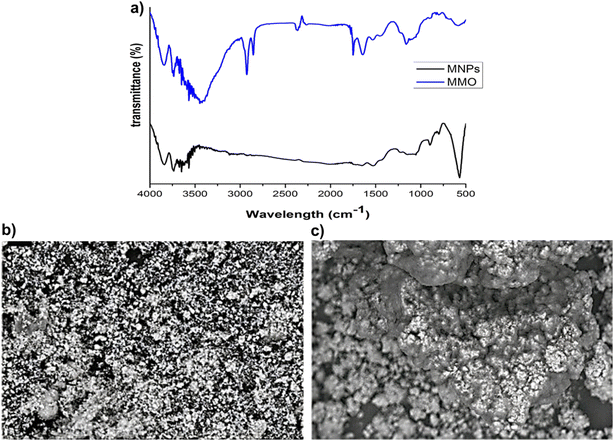 |
| Fig. 9 Characterization and appearance of MMO coagulant and MNPs (a) FTIR analysis of MNPs and MMO, SEM analysis of (b) MNPs and (c) MMO, Adapted with permission from ref. 58, Copyright, Elsevier, 2022. | |
3.2. Recycling and regeneration of MN-C/F
One of the key advantages of using MN-C/Fs is the ability to recycle and regenerate the material. This is particularly important in industrial applications where large volumes of water are treated and the cost of disposal is significant. By using magnetic separation, the coagulant/flocculant can be easily removed from the treated water and regenerated for reuse.83,98 There are several methods for recycling and regenerating MN-C/Fs. One common approach is to use a magnetic field to separate the coagulant/flocculant from the treated water. The material can then be washed with a regeneration solution to remove any remaining impurities and restore its magnetic properties.81,99 Another approach involves the use of acids or bases to regenerate the coagulant/flocculant. This method has been shown to be particularly effective for biopolymer-based coagulants/flocculants, where the material can be easily dissolved and then re-precipitated through the addition of a suitable counter-ion.100 Fig. 10 provides a comprehensive display of the effective MN-C/Fs, showcasing their potential for recycling and regeneration through many cycles. Several studies have demonstrated the successful retrieval of MPs from flocs utilizing a magnetic field, resulting in a smaller volume of sludge containing MPs compared to other particle types.32,88,89,100 The recovery performance of three chitosan based magnetic flocculants; MC, MC-g-PAM, and MC-g-PAA has been explored by researchers.90 All recycling/reuse trials reveal significant recovery rates reached 77.24%, 68.66%, and 72.97% for MC, MC-g-PAM, and MC-g-PAA, respectively after five times of use (Fig. 10b). Noor et al. (2022) investigated the recycling of MMO-Fe3O4 nanoparticles for the treatment of palm oil effluent. The study found that the nanoparticles could be effectively regenerated and reused up to three times, maintaining a high removal efficiency for pollutants (Fig. 10a).58 The use of ethanol to wash the MNPs helped eliminate any remaining impurities from the synthesis process. As a result, more of the MNPs' surface area was available for functionalization with the active compounds in MO extract. This likely prevented a gradual decrease in the performance of MMO in removing pollutants from palm oil wastewater.58,101 Lü et al. (2018) explored the reuse of Quaternized chitosan (QC)-grafted MNPs flocculants for the removal of diesel oil from wastewater. The study found that Fe3O4@SiO2-QC could be regenerated and reused up to eight times under acidic condition and seven cycles under both neutral and alkaline conditions without significant loss of their removal efficiency (Fig. 10c).88 In a study by Reck et al. (2020), MMO-albumin fractionation-Fe3O4 nanoparticles were used for the removal of color from Reactive Black 5 synthetic wastewater. The study found that the MN-C showed a high removal efficiency for color and could be reused up to five times.95
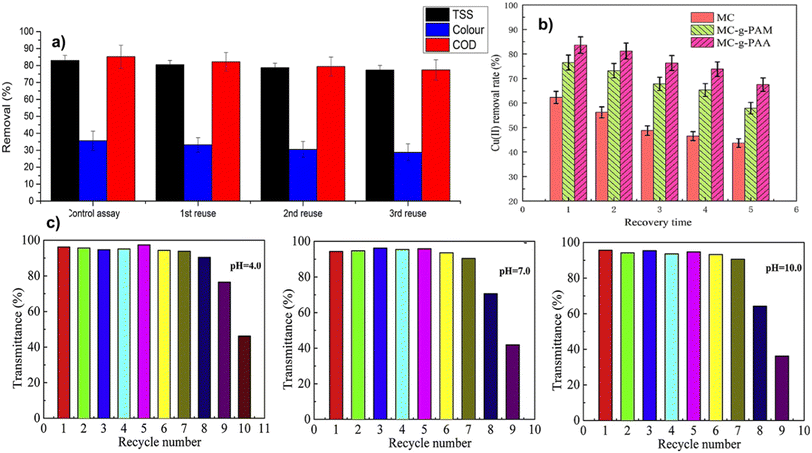 |
| Fig. 10 Recycling and regeneration of (a) MMO-Fe3O4 nanoparticles on the removal of TSS and color from palm oil effluent, adapted with permission from ref. 58, Copyright, Elsevier, 2022, (b) MC, MC-g-PAM, and MC-g-PAA on the removal of Cu(II), adapted with permission from ref. 90, Copyright, Elsevier, 2022, and (c) Fe3O4@SiO2-QC on the removal of Cu(II) at various pH levels, adapted with permission from ref. 88, Copyright, Elsevier, 2018. | |
4. Disposal concerns and treatment options for coagulant sludge
After the wastewater treatment process, the coagulation and flocculation processes often result in the generation of coagulant sludge. This sludge consists of the residual solids, including both contaminants and the coagulant material itself. It is essential to properly manage and dispose of this coagulant sludge due to its potential environmental impact.10,20 Coagulant sludge has the potential to release contaminants into the environment if not adequately contained.102 In general, implementing appropriate treatment methods and exploring potential uses can contribute to sustainable waste management practices, minimizing environmental impacts and promoting resource recovery. Leaching of pollutants into soil and water bodies can lead to adverse effects on ecosystems and human health.103 Dewatering is a common treatment method used to reduce the moisture content of coagulant sludge. This process involves mechanical methods such as filtration, centrifugation, or pressing to separate the liquid phase from the sludge, reducing its volume for further treatment or disposal.104 On the other side, stabilization and solidification processes involve treating the coagulant sludge to immobilize or encapsulate the contaminants, reducing their mobility and leachability.105 This can be achieved by adding binding agents, such as cement or lime, to the sludge to form a solidified mass that can be safely disposed of.106 Thermal treatment methods, such as incineration or pyrolysis, can be also employed to reduce the volume of coagulant sludge and destroy organic contaminants. These processes utilize high temperatures to convert the sludge into ash, gases, and heat, reducing the overall waste volume and destroying harmful substances.107
Treated coagulant sludge, after undergoing stabilization processes, can be applied to land as a soil conditioner or fertilizer. The sludge's nutrient content, organic matter, and binding properties can improve soil quality and promote plant growth when applied in appropriate quantities and following regulatory guidelines.107,108 Some treatment methods, such as incineration, can generate heat and energy from the coagulant sludge. The heat produced can be utilized for on-site energy needs or even converted into electricity, contributing to the overall sustainability of the wastewater treatment plant. Furthermore, coagulant sludge may contain valuable resources like metals or minerals that can be recovered and reused. Extraction and recycling processes can be employed to recover these resources, reducing the dependency on virgin materials and promoting circular economy principles.102,105,107
5. Proposed large-scale MN-CFs based systems
This review highlights diverse concepts for suggested large-scale treatment systems used by MN-CFs. Here are five proposed large-scale magnetic natural coagulants/flocculants systems:
(1) Magnetic chitosan nanoparticles-based system: magnetic chitosan nanoparticles refer to a class of magnetic NC/Fs which utilize chitosan, a natural polymer extracted from crustacean shells, as the coagulation/flocculation material. The chitosan could be chemically modified with magnetic nanoparticles to facilitate magnetic separation of the coagulated/flocculated pollutants. This methodology has exhibited encouraging outcomes in eliminating heavy metals, dyes, and organic compounds from wastewater.44,48,61
(2) Magnetic moringa seeds-based system: magnetic moringa seeds are another type of magnetic natural coagulant/flocculant that utilizes the seeds of the moringa tree, which contain cationic proteins that can coagulate/flocculate contaminants in water. The seeds are functionalized with magnetic nanoparticles for magnetic separation. This system has been shown to be effective in the removal of turbidity, organic matter, and bacteria from wastewater.34,58,81,98
(3). Magnetic starch flocculants-based system: magnetic starch-based flocculants are a type of magnetic natural flocculant that uses starch, a natural polymer derived from plants, as the flocculant material. The starch is modified with magnetic nanoparticles for magnetic separation. This system has been shown to be effective in the removal of suspended solids, turbidity, and heavy metals from water.109–111
(4) Magnetic tannin-based system: tannins are a group of natural polyphenols that can be extracted from plants and have been shown to have coagulating properties. Magnetic tannin-based coagulants utilize the coagulating properties of tannins and are functionalized with magnetic nanoparticles for easy separation of the coagulated contaminants from treated wastewater.29,49,59,76
(5) Magnetic cellulose flocculants-based system: cellulose is a natural polymer that can be extracted from plants and has been used in various wastewater treatment applications. Magnetic cellulose-based flocculants have the ability to utilize the flocculating properties of cellulose and facilitate the magnetic separation when be modified by magnetic nanoparticles.112,113
These proposed large-scale magnetic natural coagulants/flocculants systems are reported to offer several advantages over conventional water treatment methods, such as the use of natural materials, low cost, and high efficiency. Additionally, the magnetic properties of these systems make them easy to separate from the treated water, which reduces the need for additional treatment steps and minimizes waste. Particularly, these systems have the potential to provide a more sustainable and cost-effective solution for industrial wastewater treatment, mainly in developing countries where access to clean water is limited. Fig. 11 provides a visual representation of the key components and requirements of the proposed large-scale treatment systems employed by effective MN-CFs. This includes the shape/design of the various units within the system, which have been to be carefully engineered to ensure their effectiveness in treating the intended waste effluents.
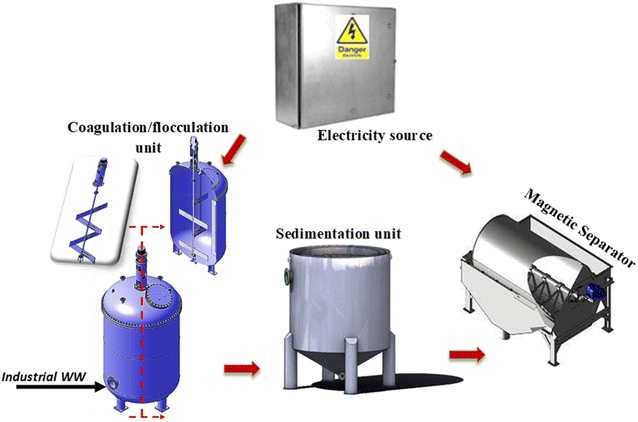 |
| Fig. 11 Main proposed large-scale treatment units employed by MN-CFs for industrial effluent decontamination. | |
6. Limitations and future prospects
There are some limitations to the commercialization of NC/Fs. Each of natural sources of coagulants/flocculants has its unique advantages and disadvantages. For example, plant-based coagulants/flocculants are readily available and inexpensive, but their effectiveness may vary depending on the source and the quality of the material.13,76 The composition and quality of plant substances can vary depending on several factors, such as climate, soil, and harvesting techniques. Moreover, some plant-based substances are also seasonal, and their availability is limited to certain periods.49,55 This inconsistency can result in variations in the coagulation and flocculation performance, making it difficult to achieve consistent results. On the other side, the lack of standardization in the production and application of NC/Fs makes it challenging for companies to use them on a large scale.55 In contrast, animal-based coagulants/flocculants may be more effective, but they may be less readily available and more expensive. Moreover, they may not be suitable for use in industries where there are concerns about the spread of animal-borne diseases. Additionally, there are ethical concerns around the use of animal products in industrial applications.44,48,61,67 Similarly, micro-organism-based coagulants/flocculants may be effective, but their production and use may be more complex.68,113 Additionally, the extraction/production of most NC/Fs can be complicated, time-consuming and expensive, making it difficult to meet the high demand for these products. Despite numerous investigations on the coagulation mechanism utilizing NC/Fs, there are still limitations. Additionally, identifying the chemical compound accountable for particle agglomeration is a formidable task due to the intricacy of the process and the potential synergistic impacts among the various components involved.29,55 “Additional investigation is necessary to identify the active compounds that could aid in discovering the primary compound responsible for the coagulation–flocculation process. The barriers that prevent the commercialization of NC/Fs are typically related to financial, research and development, market awareness, and regulatory approval issues. These concerns can be addressed by enhancing the properties of NC/Fs, comprehending the risks and limitations, promoting the use of a combination of chemical and NC/Fs, examining the economic, social, and environmental aspects, and garnering support from local governing bodies. Since this process requires time and effort, gradual measures must be taken to bridge the gaps and overcome the constraints.
One major limitation of using MN-C/Fs is their relatively low coagulation/flocculation efficiency compared to synthetic chemicals. This is due to the lower charge density and smaller size of natural materials, which can result in lower binding capacity with waterborne contaminants.81,98 Additionally, the stability of MN-C/Fs under various environmental conditions, such as pH, temperature, and salinity, can be a challenge that needs to be addressed. Another limitation is the lack of standardized protocols for the synthesis, characterization, and application of MN-C/Fs, which can make it difficult to compare results between different studies.58,88,95,110 There is also a need for further research on the mechanisms of action of MN-C/Fs, which can improve their efficiency and enhance their applicability for a wide range of industrial wastewater treatment applications. Despite these limitations, there are promising future prospects for the use of MN-C/Fs in water treatment.
7. Conclusions
Natural-based coagulants and flocculants have several advantages over chemical ones, including lower cost, biodegradability, and lower potential for producing harmful by-products. Moreover, some NC/Fs, such as chitosan and Moringa oleifera seeds extract, have been found to have antimicrobial properties, which can reduce the risk of bacterial growth in the treated wastewater. However, there are some challenges associated with the use of NC/Fs, including variability in their performance due to differences in their composition, and difficulties in their extraction and preservation. Animal-based coagulants/flocculants offer a promising avenue for the development of sustainable and effective wastewater treatment technologies. However, their use in industrial wastewater treatment is limited due to issues such as cost, ethical concerns, and the potential risk of transmitting diseases. Further research is needed to optimize the use of these materials and to better understand their mechanisms of action. Microorganism-based coagulants and flocculants have several advantages over chemical and natural coagulants and flocculants, including their low cost, environmental friendliness, and potential for producing value-added products such as biofuels and bioplastics. However, there are also challenges associated with their use, including variability in production and performance, and difficulties in scale-up and commercialization. The synthesis and preparation of MN-C/Fs involve a variety of methods and materials, depending on the specific application and wastewater treatment requirements. The use of magnetic nanoparticles as a functionalizing agent allows for easy recovery and reuse of the coagulants/flocculants, making them a more sustainable and cost-effective option compared to traditional coagulants and flocculants moreover, makes them a suitable option for large-scale industrial wastewater treatment applications. There is limited research and development of NC/Fs, which hinders their commercialization. Research in this area is essential to improve the understanding of NC/Fs' mechanisms and optimize their application. The lack of research limits the potential for their wider use in different industrial sectors, including water treatment and food processing. While natural coagulants and flocculants offer numerous benefits over synthetic chemicals, several limitations hinder their commercialization. Addressing these limitations requires a concerted effort from researchers, industries, and governments to ensure the standardization, availability, and optimization of NC/Fs' performance.
Data availability
The data presented in this study are available in the article.
Author contributions
Conceptualization, A. K. B. and T. S. S., software, A. K. B and R. S. S; validation, A. K. B., R. S. S., M. M. M. M., T. S. S.; investigation, M. M. M. M.; resources, A. K. B. and M. M. M. M.; data curation, R. S. S.; writing-original draft preparation, A. K. B.; writing-review and editing, A. K. B, R. S. S., M. M. M. M., T. S. S; supervision, A. K. B, M. M. M. M, T. S. S.; project administration, T. S. S.; funding acquisition, T. S. S.
Conflicts of interest
The authors declare no conflict of interest.
References
- A. K. Badawi, M. Abd Elkodous and G. A. M. Ali, Recent advances in dye and metal ion removal using efficient adsorbents and novel nano-based materials: an overview, RSC Adv., 2021, 11(58), 36528–36553 RSC.
- A. Kane, et al., Advanced Photocatalytic Treatment of Wastewater Using Immobilized Titanium Dioxide as a Photocatalyst in a Pilot-Scale Reactor: Process Intensification, Materials, 2022, 15(13), 4547 CrossRef CAS PubMed.
- M. Kamali, et al., Sustainability criteria for assessing nanotechnology applicability in industrial wastewater treatment: Current status and future outlook, Environ. Int., 2019, 125, 261–276 CrossRef CAS PubMed.
- R. Chakraborty, et al., Adsorption of heavy metal ions by various low-cost adsorbents: a review, Int. J. Environ. Anal. Chem., 2020, 1–38 Search PubMed.
- S. M. Abdelbasir and A. E. Shalan, An overview of nanomaterials for industrial wastewater treatment, Korean J. Chem. Eng., 2019, 36(8), 1209–1225 CrossRef CAS.
- V. S. Hakke, et al., Hybrid Treatment Technologies for the Treatment of Industrial Wastewater, in Water Pollution and Remediation: Heavy Metals, Springer, 2021, pp. 211–241 Search PubMed.
- L. Bilińska, et al., Catalytic ozonation of textile wastewater as a polishing step after industrial scale electrocoagulation, J. Environ. Manage., 2020, 265, 110502 CrossRef PubMed.
- R. A. Ronke, O. G. Saidat and G. Abdulwahab, Coagulation-flocculation treatment of industrial wastewater using tamarind seed powder, Int. J. ChemTech Res., 2016, 9(5), 771–780 Search PubMed.
- A. Guesmi, et al., Disinfection of corona and myriad viruses in water by non-thermal plasma: a review, Environ. Sci. Pollut. Res., 2022, 29(37), 55321–55335 CrossRef CAS PubMed.
- A. Dalvand, et al., Application of Chemical Coagulation Process for Direct Dye Removal from Textile Wastewater, J. Environ. Health Sci. Eng., 2017, 2(3), 333–339 CAS.
- A. K. Badawi and I. S. Fahim, A critical review on green corrosion inhibitors based on plant extracts: Advances and potential presence in the market, Int. J. Corros. Scale Inhib., 2021, 10(4), 1385–1406 CAS.
- A. K. Badawi, et al., Advanced wastewater treatment process using algal photo-bioreactor associated with dissolved-air flotation system: A pilot-scale demonstration, J. Water Process. Eng., 2022, 46, 102565 CrossRef.
- E. M. Elsayed, et al., Comparison of coagulation performance using natural coagulants against traditional ones, Sep. Sci. Technol., 2021, 56(10), 1779–1787 CrossRef CAS.
- N. Precious Sibiya, S. Rathilal and E. Kweinor Tetteh, Coagulation treatment of wastewater: kinetics and natural coagulant evaluation, Molecules, 2021, 26(3), 698 CrossRef PubMed.
- T. M. Alasri, et al., Band-structure engineering of TiO2 photocatalyst by AuSe quantum dots for efficient degradation of malachite green and phenol, J. Inorg. Organomet. Polym. Mater., 2023, 1–12 Search PubMed.
- N. T. H. Nhung, et al., A review of soil contaminated with dioxins and biodegradation technologies: current status and future prospects, Toxics, 2022, 10(6), 278 CrossRef CAS PubMed.
- E. Hoffman, et al., Characterization and spatial distribution of organic-contaminated sediment derived from historical industrial effluents, Environ. Monit. Assess., 2019, 191, 1–19 CrossRef PubMed.
- T. S. Saleh, et al., Design and development of novel composites containing nickel ferrites supported on activated carbon derived from agricultural wastes and its application in water remediation, Materials, 2023, 16(6), 2170 CrossRef CAS PubMed.
- H. Islam, et al., Geospatial analysis of wetlands based on land use/land cover dynamics using remote sensing and GIS in Sindh, Pakistan, Sci. Prog., 2021, 104(2), 00368504211026143 Search PubMed.
- A. K. Badawi and K. Zaher, Hybrid treatment system for real textile wastewater remediation based on coagulation/flocculation, adsorption and filtration processes: Performance and economic evaluation, J. Water Process. Eng., 2021, 40, 101963 CrossRef.
- A. Aouni, R. Lafi and A. Hafiane, Feasibility evaluation of combined electrocoagulation/adsorption process by optimizing operating parameters removal for textile wastewater treatment, Desalin. Water Treat., 2017, 60, 10890 Search PubMed.
- B. Mella, et al., Treatment of leather dyeing wastewater with associated process of coagulation-flocculation/adsorption/ozonation, Ozone: Sci. Eng., 2018, 40(2), 133–140 CrossRef CAS.
- Z. Al-Qodah, Y. Al-Qudah and E. Assirey, Combined biological wastewater treatment with electrocoagulation as a post-polishing process: A review, Sep. Sci. Technol., 2020, 55(13), 2334–2352 CrossRef CAS.
- A. K. Verma, R. R. Dash and P. Bhunia, A review on chemical coagulation/flocculation technologies for removal of colour from textile wastewaters, J. Environ. Manage., 2012, 93(1), 154–168 CrossRef CAS PubMed.
- B. Kaur, R. K. Garg and A. P. Singh, Treatment of wastewater from pulp and paper mill using coagulation and flocculation, J. Environ. Treat. Tech., 2021, 9(1), 158–163 Search PubMed.
- M. Z. B. Mukhlish, et al., Removal of reactive dye from aqueous solution using coagulation–flocculation coupled with adsorption on papaya leaf, J. Mech. Eng. Sci., 2016, 10(1), 1884–1894 CAS.
- S. Wang, et al., Preparation and coagulation-flocculation performance of covalently bound organic hybrid coagulant with excellent stability, Colloids Surf., A, 2020, 600, 124966 CrossRef CAS.
- C. Zhao, et al., Application of coagulation/flocculation in oily wastewater treatment: A review, Sci. Total Environ., 2021, 765, 142795 CrossRef CAS PubMed.
- H. N. P. Dayarathne, et al., Removal of natural organic matter from source water: Review on coagulants, dual coagulation, alternative coagulants, and mechanisms, J. Water Process. Eng., 2021, 40, 101820 CrossRef.
- Y. Sun, et al., Evaluation and optimization of enhanced coagulation process: Water and energy nexus, Water-Energy Nexus, 2019, 2(1), 25–36 CrossRef.
- S. Dubey, M. Agrawal and A. B. Gupta, Advances in coagulation technique for treatment of fluoride-contaminated water: a critical review, Rev. Chem. Eng., 2019, 35(1), 109–137 CrossRef CAS.
- M. H. Mohamed Noor, et al., Assessing the effectiveness of magnetic nanoparticles coagulation/flocculation in water treatment: a systematic literature review, Int. J. Environ. Sci. Technol., 2022, 19(7), 6935–6956 CrossRef CAS.
- A. Karam, E. S. Bakhoum and K. Zaher, Coagulation/flocculation process for textile mill effluent treatment: experimental and numerical perspectives, Int. J. Sustain. Eng., 2021, 14(5), 983–995 CrossRef.
- M. Dehghani and M. H. Alizadeh, The effects of the natural coagulant Moringa oleifera and alum in wastewater treatment at the Bandar Abbas Oil Refinery, Environ. Health Eng. Manage. J., 2016, 3(4), 225–230 CrossRef.
- A. H. Jagaba, et al., Sustainable use of natural and chemical coagulants for contaminants removal from palm oil mill effluent: A comparative analysis, Ain Shams Eng. J., 2020, 11(4), 951–960 CrossRef.
- W. Anteneh and O. P. Sahu, Natural coagulant for the treatment of food industry wastewater, International Letters of Natural Sciences, 2014 Search PubMed.
- N. Das, N. Ojha and S. K. Mandal, Wastewater treatment using plant-derived bioflocculants: green chemistry approach for safe environment, Water Sci. Technol., 2021, 83(8), 1797–1812 CrossRef CAS PubMed.
- O. P. Sahu and P. K. Chaudhari, Review on chemical treatment of industrial waste water, J. Appl. Sci. Environ. Manage., 2013, 17(2), 241–257 CAS.
- N. K. Shammas, et al., Fundamentals of chemical coagulation and precipitation, Environmental flotation engineering, 2021, pp. 95–142 Search PubMed.
- C. S. Lee, J. Robinson and M. F. Chong, A review on application of flocculants in wastewater treatment, Process Saf. Environ. Prot., 2014, 92(6), 489–508 CrossRef CAS.
- V. H. Dao, N. R. Cameron and K. Saito, Synthesis, properties and performance of organic polymers employed in flocculation applications, Polym. Chem., 2016, 7(1), 11–25 RSC.
- J. Ribera-Pi, et al., Coagulation-flocculation and moving bed biofilm reactor as pre-treatment for water recycling in the petrochemical industry, Sci. Total Environ., 2020, 715, 136800 CrossRef CAS PubMed.
- P. Pal, J. P. Pandey and G. Sen, Synthesis, characterization and flocculation studies of a novel graft copolymer towards destabilization of carbon nano-tubes from effluent, Polymer, 2017, 112, 159–168 CrossRef CAS.
- A. H. Jagaba, et al., Wastewater treatment using alum, the combinations of alum-ferric chloride, alum-chitosan, alum-zeolite and alum–Moringa oleifera as adsorbent and coagulant, Int. J. Manag. Sci. Eng. Manag., 2018, 2(3), 67–75 Search PubMed.
- S. M. R. Shaikh, et al., Investigation of the effect of polyelectrolyte structure and type on the electrokinetics and flocculation behavior of bentonite dispersions, Chem. Eng. J., 2017, 311, 265–276 CrossRef CAS.
- B. Othmani, M. G. Rasteiro and M. Khadhraoui, Toward green technology: a review on some
efficient model plant-based coagulants/flocculants for freshwater and wastewater remediation, Clean Technol. Environ. Policy, 2020, 22, 1025–1040 CrossRef CAS.
- M. M. Alnawajha, et al., Plant-based coagulants/flocculants: characteristics, mechanisms, and possible utilization in treating aquaculture effluent and benefiting from the recovered nutrients, Environ. Sci. Pollut. Res., 2022, 29(39), 58430–58453 CrossRef CAS PubMed.
- E. B. Holmes, et al., Evaluation of Chitosans as Coagulants—Flocculants to Improve Sand Filtration for Drinking Water Treatment, Int. J. Mol. Sci., 2023, 24(2), 1295 CrossRef CAS PubMed.
- H. Kristianto, The potency of Indonesia native plants as natural coagulant: a mini review, Water Conserv. Sci. Eng., 2017, 2(2), 51–60 CrossRef.
- M. Al-Sahari, A. A. S. Al-Gheethi, and R. M. S. Radin Mohamed, Natural coagulates for wastewater treatment; A review for application and mechanism, Prospects of fresh market wastes management in developing countries, 2020, pp. 17–31 Search PubMed.
- D. Deepa, et al., Primary treatment of dairy wastewater using bio based natural coagulants, Mater. Today: Proc., 2022, 60, 616–621 CAS.
- J. El-Gaayda, et al., Natural flocculants for the treatment of wastewaters containing dyes or heavy metals: a state-of-the-art review, J. Environ. Chem. Eng., 2021, 9(5), 106060 CrossRef CAS.
- C. Y. Teh, et al., Recent advancement of coagulation–flocculation and its application in wastewater treatment, Ind. Eng. Chem. Res., 2016, 55(16), 4363–4389 CrossRef CAS.
- C. Y. Teh and T. Y. Wu, The potential use of natural coagulants and flocculants in the treatment of urban waters, Chem. Eng. Trans., 2014, 39, 1603–1608 Search PubMed.
- B. U. Okoro, et al., Natural organic matter (NOM) and turbidity removal by plant-based coagulants: A review, J. Environ. Chem. Eng., 2021, 9(6), 106588 CrossRef CAS.
- S. B. Kurniawan, et al., Challenges and opportunities of biocoagulant/bioflocculant application for drinking water and wastewater treatment and its potential for sludge recovery, Int. J. Environ. Res. Public Health, 2020, 17(24), 9312 CrossRef CAS PubMed.
- S. Ramesh and L. Mekala, Treatment of Textile Wastewater Using Moringa Oleifera and Tamarindus indica, Int. Res. J. Eng. Technol., 2018, 6(171), 3891–3895 Search PubMed.
- M. H. M. Noor, et al., Optimization of sonication-assisted synthesis of magnetic Moringa oleifera as an efficient coagulant for palm oil wastewater treatment, Environ. Technol. Innovation, 2022, 25, 102191 CrossRef.
- E. C. Lopes, et al., Evaluation of a tannin-based coagulant on the decolorization of synthetic effluents, J. Environ. Chem. Eng., 2019, 7(3), 103125 CrossRef CAS.
- M.-T. Dao, et al., Pilot-scale study of real domestic textile wastewater treatment using Cassia fistula seed-derived coagulant, J. Chem., 2021, 2021, 7608856 Search PubMed.
- N. P. Sibiya, et al., Effect of Magnetized Coagulants on Wastewater Treatment: Rice Starch and Chitosan Ratios Evaluation, Polymers, 2022, 14(20), 4342 CrossRef CAS PubMed.
- N. M. Mahmoodi, et al., Dye removal from colored textile wastewater using chitosan in binary systems, Desalination, 2011, 267(1), 64–72 CrossRef CAS.
- H. E. Knidri, et al., Rapid and efficient extraction of chitin and chitosan for scale-up production: Effect of process parameters on deacetylation degree and molecular weight, Int. J. Biol. Macromol., 2019, 139, 1092–1102 CrossRef PubMed.
- C. K. Coleman, et al., Chitosan Coagulation Pretreatment to Enhance Ceramic Water Filtration for Household Water Treatment, Int. J. Mol. Sci., 2021, 22(18), 9736 CrossRef CAS PubMed.
- M. M. Aguilera Flores, et al., Evaluation of a biocoagulant from devilfish invasive species for the removal of contaminants in ceramic industry wastewater, Sci. Rep., 2022, 12(1), 1–12 CrossRef PubMed.
- E. Lichtfouse, et al., Chitosan for direct bioflocculation of wastewater, Environ. Chem. Lett., 2019, 17, 1603–1621 CrossRef CAS.
- G. J. Piazza and R. A. Garcia, Meat & bone meal extract and gelatin as renewable flocculants, Bioresour. Technol., 2010, 101(2), 781–787 CrossRef CAS PubMed.
- S. B. Kurniawan, et al., Challenges and Opportunities of Biocoagulant/Bioflocculant Application for Drinking Water and Wastewater Treatment and Its Potential for Sludge Recovery, Int. J. Environ. Res. Public Health, 2020, 17(24), 9312 CrossRef CAS PubMed.
- S. B. Kurniawan, et al., Treatment of real aquaculture effluent using bacteria-based bioflocculant produced by Serratia marcescens, J. Water Process. Eng., 2022, 47, 102708 CrossRef.
- F. Ben Rebah, W. Mnif and S. M. Siddeeg, Microbial flocculants as an alternative to synthetic polymers for wastewater treatment: a review, Symmetry, 2018, 10(11), 556 CrossRef CAS.
- A. Ahmad, et al., Exploring the extraction methods for plant-based coagulants and their future approaches, Sci. Total Environ., 2022, 818, 151668 CrossRef CAS PubMed.
- A. N. Ramadhani, A. N. K. W. Sarosa and L. H. Al Rosyad, The potency of microbial flocculant produced by B. licheniformis using molasses as the carbon source and its application in food industry wastewater treatment, Mater. Today: Proc., 2022, 63, S244–S247 CAS.
- F. A. B. M. Lanan, et al., Utilisation of natural plant-based fenugreek (Trigonella foenum-graecum) coagulant and okra (Abelmoschus escluentus) flocculant for palm oil mill effluent (POME) treatment, J. Environ. Chem. Eng., 2021, 9(1), 104667 CrossRef CAS.
- B. Ramavandi and S. Farjadfard, Removal of chemical oxygen demand from textile wastewater using a natural coagulant, Korean J. Chem. Eng., 2014, 31(1), 81–87 CrossRef CAS.
- W. Subramonian, T. Y. Wu and S.-P. Chai, A comprehensive study on coagulant performance and floc characterization of natural Cassia obtusifolia seed gum in treatment of raw pulp and paper mill effluent, Ind. Crops Prod., 2014, 61, 317–324 CrossRef CAS.
- E. H. Khader, T. H. J. Mohammed and N. Mirghaffari, Use of natural coagulants for removal of COD, oil and turbidity from produced waters in the petroleum industry, J. Pet. Environ. Biotechnol., 2018, 9(3), 374 Search PubMed.
- I. M. Iloamaeke and C. O. Julius, Treatment of Pharmaceutical Effluent Using seed of Phoenix Dactylifera as a Natural Coagulant, J. Basic Phy. Res., 2019, 9(1), 91–100 Search PubMed.
- B. Sarkar, et al., Wastewater treatment in dairy industries—possibility of reuse, Desalination, 2006, 195(1–3), 141–152 CrossRef CAS.
- S. Shamsnejati, et al., Mucilaginous seed of Ocimum basilicum as a natural coagulant for textile wastewater treatment, Ind. Crops Prod., 2015, 69, 40–47 CrossRef CAS.
- S. M. Mohan, Use of naturalized coagulants in removing laundry waste surfactant using various unit processes in lab-scale, J. Environ. Manage., 2014, 136, 103–111 CrossRef CAS PubMed.
- T. R. T. Santos, et al., Development of a magnetic coagulant based on Moringa oleifera seed extract for water treatment, Environ. Sci. Pollut. Res., 2016, 23, 7692–7700 CrossRef CAS PubMed.
- C. A. Igwegbe and O. D. Onukwuli, Removal of total dissolved solids (TDS) from aquaculture wastewater by coagulation-flocculation process using Sesamum indicum extract: effect of operating parameters and coagulation-flocculation kinetics, Pharm. Chem. J., 2019, 6(4), 32–45 CAS.
- H. Kristianto, et al., Magnetically assisted coagulation using iron oxide nanoparticles-Leucaena leucocephala seeds' extract to treat synthetic Congo red wastewater, Int. J. Environ. Sci. Technol., 2020, 17, 3561–3570 CrossRef CAS.
- Z. Z. Abidin, et al., A preliminary study on Jatropha curcas as coagulant in wastewater treatment, Environ. Technol., 2011, 32(9), 971–977 CrossRef CAS PubMed.
- A. A. Owodunni, S. Ismail and N. G. Olaiya, Parametric study of novel plant-based seed coagulant in modeled wastewater turbidity removal, Environ. Sci. Pollut. Res., 2022, 1–9 Search PubMed.
- T. R. T. dos Santos, et al., Evaluation of magnetic coagulant (α-Fe2O3-MO) and its reuse in textile wastewater treatment, Water, Air, Soil Pollut., 2018, 229, 1–17 CrossRef.
- C. S. Miyashiro, et al., Synthesis and performance evaluation of a magnetic biocoagulant in the removal of reactive black 5 dye in aqueous medium, Mater. Sci. Eng., C, 2021, 119, 111523 CrossRef CAS PubMed.
- T. Lü, et al., Enhanced demulsification from aqueous media by using magnetic chitosan-based flocculant, J. Colloid Interface Sci., 2018, 518, 76–83 CrossRef PubMed.
- J. Ma, et al., Magnetic flocculants synthesized by Fe3O4 coated with cationic polyacrylamide for high turbid water flocculation, Environ. Sci. Pollut. Res., 2018, 25, 25955–25966 CrossRef CAS PubMed.
- Y. Sun, et al., Magnetic flocculation of Cu(II) wastewater by chitosan-based magnetic composite flocculants with recyclable properties, Carbohydr. Polym., 2021, 261, 117891 CrossRef CAS PubMed.
- R. Singh and R. Bhateria, Experimental and Modeling Process Optimization of Lead Adsorption on Magnetite Nanoparticles via Isothermal, Kinetics, and Thermodynamic Studies, ACS Omega, 2020, 5(19), 10826–10837 CrossRef CAS PubMed.
- A. M. d. Oliveira, et al., Functionalized magnetite nanoparticles with Moringa oleifera with potent antibacterial action in wastewater, Environ. Technol., 2021, 42(27), 4296–4305 CrossRef CAS PubMed.
- N. Saifuddin and S. Dinara, Pretreatment of palm oil mill effluent
(POME) using magnetic chitosan, E-J. Chem., 2011, 8(S1), S67–S78 CrossRef CAS.
- L. Zheng, et al., Insight into the magnetic lime coagulation-membrane distillation process for desulfurization wastewater treatment: From pollutant removal feature to membrane fouling, J. Hazard. Mater., 2020, 391, 122202 CrossRef CAS PubMed.
- I. M. Reck, et al., Protein fractionation of Moringa oleifera Lam. seeds and functionalization with magnetic particles for the treatment of reactive black 5 solution, Can. J. Chem. Eng., 2019, 97(8), 2309–2317 CrossRef CAS.
- M. H. M. Noor, N. Ngadi and W. S. Luing, Synthesis of magnetic cellulose as flocculant for pre-treatment of anaerobically treated palm oil mill effluent, Chem. Eng. Trans., 2018, 63, 589–594 Search PubMed.
- A. Heiras-Trevizo, et al., Al-doped Fe3O4 hollow nanostructured particles: a promising material for application in the coagulation-flocculation process, Mater. Lett., 2023, 330, 133404 CrossRef CAS.
- G. A. P. Mateus, et al., Evaluation of a magnetic coagulant based on Fe3O4 nanoparticles and Moringa oleifera extract on tartrazine removal: coagulation-adsorption and kinetics studies, Environ. Technol., 2018, 41, 1648–1663 CrossRef PubMed.
- K. Q. Jabbar, A. A. Barzinjy and S. M. Hamad, Iron oxide nanoparticles: Preparation methods, functions, adsorption and coagulation/flocculation in wastewater treatment, Environ. Nanotechnol. Monit. Manag., 2022, 17, 100661 CAS.
- T. R. T. dos Santos, et al., Magnetic coagulant based on Moringa oleifera seeds extract and super paramagnetic nanoparticles: optimization of operational conditions and reuse evaluation, Desalin. Water Treat., 2018, 106, 226–237 CrossRef CAS.
- S. Kumar, et al., Chitosan nanocomposite coatings for food, paints, and water treatment applications, Appl. Sci., 2019, 9(12), 2409 CrossRef CAS.
- J. Kluczka, et al., Assessment of aluminum bioavailability in alum sludge for agricultural utilization, Environ. Monit. Assess., 2017, 189, 1–8 CrossRef CAS PubMed.
- A. Suman, T. Ahmad and K. Ahmad, Dairy wastewater treatment using water treatment sludge as coagulant: a novel treatment approach, Environ. Dev. Sustain., 2018, 20, 1615–1625 CrossRef.
- H. Wei, et al., Coagulation/flocculation in dewatering of sludge: a review, Water Res., 2018, 143, 608–631 CrossRef CAS PubMed.
- O. A. Johnson and A. C. Affam, Petroleum sludge treatment and disposal: A review, Environ. Eng. Res., 2019, 24(2), 191–201 CrossRef.
- M. I. Ejimofor, I. G. Ezemagu and M. C. Menkiti, Physiochemical, Instrumental and thermal characterization of the post coagulation sludge from paint industrial wastewater treatment, S. Afr. J. Chem. Eng., 2021, 37, 150–160 Search PubMed.
- L. Cai, et al., Stabilization of heavy metals in piggery wastewater sludge through coagulation-hydrothermal reaction–pyrolysis process and sludge biochar for tylosin removal, J. Cleaner Prod., 2020, 260, 121165 CrossRef CAS.
- L. Zhou, et al., Study on polymer-bridging flocculation performance of ultrafine specular hematite ore and its high gradient magnetic separation behavior: Description of floc microstructure and flocculation mechanism, Sep. Purif. Technol., 2021, 276, 119304 CrossRef CAS.
- M. H. Mohamed Noor, et al., Phytotoxicity and Cost-effectiveness Analysis of Palm Oil Wastewater Treated with Magnetic Cationic Starch Flocculant, Water, Air, Soil Pollut., 2022, 233(12), 482 CrossRef CAS.
- M. H. M. Noor, K. J. Lee and N. Ngadi, Starch engineered with Moringa oleifera seeds protein crosslinked Fe3O4: A synthesis and flocculation studies, Int. J. Biol. Macromol., 2021, 193, 2006–2020 CrossRef PubMed.
- M. H. M. Noor, et al., Synthesis and application of polyacrylamide grafted magnetic cellulose flocculant for palm oil wastewater treatment, J. Environ. Chem. Eng., 2020, 8(4), 104014 CrossRef.
- P. Maćczak, H. Kaczmarek and M. Ziegler-Borowska, Recent achievements in polymer bio-based flocculants for water treatment, Materials, 2020, 13(18), 3951 CrossRef PubMed.
- G. Guan, et al., A cost-effective anionic flocculant prepared by grafting carboxymethyl cellulose and lignosulfonate with acrylamide, Cellulose, 2021, 28, 11013–11023 CrossRef CAS.
|
This journal is © The Royal Society of Chemistry 2023 |